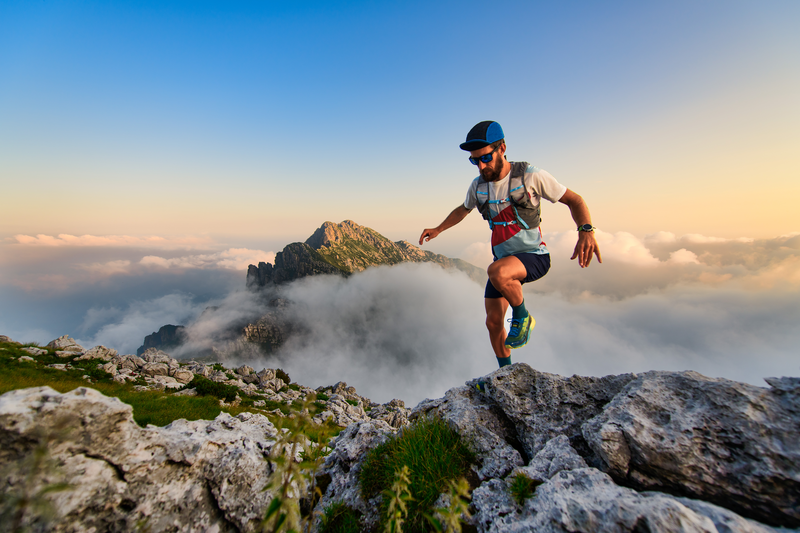
94% of researchers rate our articles as excellent or good
Learn more about the work of our research integrity team to safeguard the quality of each article we publish.
Find out more
ORIGINAL RESEARCH article
Front. Oncol. , 13 September 2021
Sec. Molecular and Cellular Oncology
Volume 11 - 2021 | https://doi.org/10.3389/fonc.2021.732743
This article is part of the Research Topic The Role of Exosomes in Cancers Progression and Related Therapeutic Strategies View all 8 articles
Background: Patient participation in colorectal cancer (CRC) screening via a stool test and colonoscopy is suboptimal, but participation can be improved by the development of a blood test. However, the suboptimal detection abilities of blood tests for advanced neoplasia, including advanced adenoma (AA) and CRC, limit their application. We aimed to investigate the proteomic landscape of small extracellular vesicles (sEVs) from the serum of patients with colorectal neoplasia and identify specific sEV proteins that could serve as biomarkers for early diagnosis.
Materials and Methods: We enrolled 100 patients including 13 healthy subjects, 12 non-AAs, 13 AAs, and 16 stage-I, 15 stage-II, 16 stage-III, and 15 stage-IV CRCs. These patients were classified as normal control, early neoplasia, and advanced neoplasia. The sEV proteome was explored by liquid chromatography-tandem mass spectrometry. Generalized association plots were used to integrate the clustering methods, visualize the data matrix, and analyze the relationship. The specific sEV biomarkers were identified by a decision tree via Orange3 software. Functional enrichment analysis was conducted by using the Ingenuity Pathway Analysis platform.
Results: The sEV protein matrix was identified from the serum of 100 patients and contained 3353 proteins, of which 1921 proteins from 98 patients were finally analyzed. Compared with the normal control, subjects with early and advanced neoplasia exhibited a distinct proteomic distribution in the data matrix plot. Six sEV proteins were identified, namely, GCLM, KEL, APOF, CFB, PDE5A, and ATIC, which properly distinguished normal control, early neoplasia, and advanced neoplasia patients from each other. Functional enrichment analysis revealed that APOF+ and CFB+ sEV associated with clathrin-mediated endocytosis signaling and the complement system, which have critical implications for CRC carcinogenesis.
Conclusion: Patients with colorectal neoplasia had a distinct sEV proteome expression pattern in serum compared with those patients who were healthy and did not have neoplasms. Moreover, the six identified specific sEV proteins had the potential to discriminate colorectal neoplasia between early-stage and advanced neoplasia. Collectively, our study provided a six-sEV protein biomarker panel for CRC diagnosis at early or advanced stages. Furthermore, the implication of the sEV proteome in CRC carcinogenesis via specific signaling pathways was explored.
Colorectal cancer (CRC) ranks as the third most common cancer and the second leading cause of cancer-related deaths worldwide; therefore, it is a critical public health issue (1). In Taiwan, CRC incidence was 42.9 cases per 100,000 residents in 2017 and higher than lung cancer, breast cancer, and hepatoma. Early detection and robust screening for CRC could significantly reduce mortality from CRC (2). Considerable efforts have substantially improved CRC screening participation, which has resulted in a 3.3% decrease per year in CRC incidence rates from 2011 to 2016 (3). The most commonly used modalities for screening CRC are stool-based tests and colonoscopy. Among the various screening modalities, colonoscopy offers confirmatory evidence of disease and reduces mortality rates by 67% (4, 5). However, the inconvenience, invasiveness, and cost of colonoscopy limit patient compliance to screening. Thus, a population-based randomized control trial demonstrated that fecal immunochemical tests (FITs) detected as many CRCs as colonoscopy as a first-line screening modality (6). Currently, stool-based FIT and DNA tests are available for screening CRC. Although FIT profoundly contributes to reducing CRC mortality, low sensitivity to precancerous lesions has hampered its clinical utility (7–9). Although stool DNA tests show a better detection for precancerous lesions and early cancers than FIT, participation in stool-based screening remains suboptimal because of the negative acceptance of fecal specimen collection, handling, and transportation to the clinic (10, 11). A blood test could provide an additional 6.5% participation than FIT for screening (12). Not surprisingly, there is a preference for blood-based screening to improve the limited acceptance of either colonoscopy or stool-based tests, highlighting the need and potential merit for developing highly robust blood-based tests for identifying colorectal neoplasia.
Small extracellular vesicles (sEVs) are membrane-bound vesicles ranging from 30 to 150 nm in diameter that are secreted by various cell types. They encapsulate multiple proteins, nucleic acids, and other biomolecules with either the physiological or pathological status of parental cells (13). sEVs transport biomolecules between cells and play an essential role in intercellular communication locally and systematically. Moreover, they contribute to cancer formation and progression by facilitating tumor angiogenesis and metastasis. The contents of sEVs are protected from external proteases and other enzymes and remain biologically stable. sEVs isolated from breast cancer demonstrated high levels of stable phosphorylated proteins and provided a window into the molecular mechanism underlying cancer progression and metastasis (14). Thus, sEVs are helpful biomarkers for the early detection of cancer. Previous studies have explored Glypican-1-containing sEVs that may play a role in diagnosing pancreatic cancer (15) and CRC (16). Moreover, CD147-containing sEVs in plasma from CRC patients are also higher than those from healthy subjects (17).
Proteins, not only genes composed of nucleic acids, are responsible for the phenotype of cancer cells. Therefore, it is crucial to elucidate the mechanisms of disease by investigating the proteome. Proteomics is a large-scale investigation of proteins that can comprehensively map the biological processes of carcinogenesis (18). The proteome is more complex than the genome because of alternative transcription initiation, alternative splicing, RNA editing, proteolytic processing, and posttranslational modifications. Proteins in the blood have the potential to serve as biomarkers for detecting CRC. However, the detection of low abundance proteins in the blood remains challenging (10). sEVs encapsulate proteins or present proteins on the surface, which provides a window for amplifying the low abundance proteins in the blood through the purification and enrichment of sEVs. As mentioned above, growing evidence supports that proteins contained within sEVs could be used to detect various cancers, including CRC (14–16).
In this study, we explored the proteomic landscape of sEVs specific to advanced adenoma (AA) and CRC and their potential as biomarkers for detecting colorectal neoplasia.
Patients who underwent colonoscopy at National Taiwan University Hospital for screening, surveillance, or symptoms and received endoscopic treatment or surgery were included prospectively. The patients were categorized based on colonoscopic findings and histologic diagnosis. Blood samples were collected chronologically within 6 years (from 2014 to 2019). Participants who had a history of inflammatory bowel disease or hereditary CRC, such as Lynch syndrome, familial adenomatous polyposis, or hyperplastic polyposis, were excluded. Participants with active malignancy within 5 years before the diagnosis of CRC were also excluded. This study protocol was approved by the Institutional Review Board of the National Taiwan University Hospital (No. 201912055RINB). All data used in this study were anonymous without any identifiable personal information.
Colorectal neoplasms were classified as non-AA, AA, and CRC according to the 2019 WHO classification system for tumors of the digestive system (19). AA was defined as a lesion 10 mm or larger in diameter or with a villous component or high-grade dysplasia. Healthy controls were defined as subjects with a negative colonoscopic finding. Each subcategory was defined as follows: normal control including healthy control and non-AA; early neoplasia including AA and stage-I CRC; advanced neoplasia including stage II–IV CRC. The cecum to splenic flexure was defined as the proximal colon, and the descending colon to the rectum was defined as the distal colon.
Whole blood was collected in a BD Vacutainer™ SST™ II Advanced tube and processed within 2 h after blood was drawn. We centrifuged the blood SST tubes for 10 min at 3000 g in a prechilled centrifuge set at 25°C, and we used a swing-out rotor with appropriate buckets. We carefully pipetted off the serum supernatant, the serum was aliquoted and kept at -80°C until use, and freeze-thawing cycles were avoided as much as possible after frozen storage. sEVs were purified from human serum samples using size exclusion chromatography on a drip with extracellular vesicle isolation by size exclusion chromatography on an EVSecond L70® drip column (GL Sciences, Tokyo, Japan). The column was initially equilibrated with 700 μl of fetal bovine serum twice, followed by three washing steps using 1500 μl of PBS. After washing, 100 μl of the collected human serum sample was loaded onto the column, followed by the collection of 12 consecutive fractions in 100 μl of PBS. CD9 expression in these fractions was analyzed using Western blotting, and CD9-positive fractions were recognized as the sEV-rich portion. The sEVs obtained using this system were previously authenticated with transmission electron microscopy and Western blotting. The diameters of the particles in the sEV fractions were analyzed with a NanoSight™ LM10.
The sEV-containing eluates of EVSecond L70 columns (GL Sciences, Tokyo, Japan) were dried and resolved in 20 mM HEPES-NaOH (pH 8.0), 12 mM sodium deoxycholate, and 12 mM sodium N-lauroyl sarcosinate. In the elution step, we collected eluate fractions continuously (100 µl/fraction). Then, the 4th to 6th fractions were combined (300 µl) and dried by vacuum evaporation. Following reduction with 20 mM dithiothreitol at 100°C for 10 min and alkylation with 50 mM iodoacetamide at ambient temperature for 45 min, proteins were digested with 5 μl of immobilized trypsin (Thermo Fisher Scientific, Inc., Waltham, MA, USA) on a plate shaker (TAITEC, M-BR-024) at 1000 rpm at 37°C for 6 h to mix the trypsin beads. After removing sodium deoxycholate and sodium N-lauroyl sarcosinate by ethyl acetate extraction, the resulting peptides were desalted by an Oasis HLB μElution plate (Waters Corp., Milford, MA, USA) and subjected to mass spectrometric analysis. The Oasis plate was prewetted with 500 µl 70% acetonitrile and equilibrated with 500 µl 0.1% TFA in 2% acetonitrile at 250 µl/min. Following sample loading at 100 µl/min, plates were washed twice with 500 µl 0.1% TFA in 2% acetonitrile at 250 µl/min. Peptides were eluted with 100 μl 70% acetonitrile at 100 μl/min and subsequently dried by vacuum evaporation. Peptides were analyzed using an LTQ-Orbitrap-Velos mass spectrometer (Thermo Fisher Scientific, Inc.) combined with an UltiMateTM 3000 RSLCnano-flow HPLC system (Thermo Fisher Scientific, Inc.). Using 0.1% formic acid as Solvent A and 0.1% formic acid in acetonitrile as Solvent B, peptides were separated on a C18 Chip-column (75 µm × 200 mm, Nikkyo Technos, Tokyo, Japan) by a gradient of Solvent B 2–30% for 95 min and 30–95% for 15 min at a flow rate of 250 nl/min. The eluted peptides were ionized with a spray voltage of 2000 V, and MS data were acquired in a data-dependent fragmentation method in which the survey scan was acquired between m/z 400 and 1600 at a resolution of 60,000 with an automatic gain control (AGC) target value of 1.0 × 106 ion counts. The top 20 intense precursor ions in each survey scan were subjected to low-resolution MS/MS acquisitions using normal CID scan mode with an AGC target value of 5,000 ion counts in the linear ion trap. Protein identification and quantification were performed using MaxQuant software (https://www.biochem.mpg.de/5111795/maxquant). The MS/MS spectra were searched against the Homo sapiens protein sequence database in SwissProt using Proteome Discoverer 2.4 software (Thermo Scientific), in which peptide identification filters were set at a false discovery rate <1%. The minimum peptide length was 6. The single peptide identification was used. Only “Razor + unique peptides” were used for the calculation of relative protein concentration. Candidate proteins were selected using the following criteria: commonly expressed in sEVs from all of the subjects with colorectal neoplasia; plasma membrane protein expressed on the sEV membrane; and higher expression level in patients with colorectal neoplasia than in healthy individuals.
Protein identification and label-free quantification were performed using Proteome Discoverer 2.2 software (Thermo Fisher Scientific). For protein identification, the LC-MS dataset was searched against the SwissProt Human Database with the Mascot (Matrix Science) or Sequest HT (Thermo Fisher Scientific) database search engine, where FDR <1% was set as the peptide identification threshold. For label-free quantification and data normalization, the Minora Feature Detector node in the Processing workflow and the Feature Mapper node followed by the Precursor Ions Quantifier node in the Consensus workflow were used with default parameters in Proteome Discoverer 2.2 software.
A generalized association plot (GAP, http://gap.stat.sinica.edu.tw/Software/GAP/index.htm) was used to visualize the expression patterns and identify the outliers in patient proteins (20, 21). We chose the proximity measure of rows with Euclidean distance and Pearson correlation for the column to create the data matrix and two proximity matrices. After deciding on the parameters, GAP used matrix visualization (MV) to present three matrix data. Because the data matrix was standardized, a symmetric blue (negative) to white (zero) to red (positive) color spectrum (Display Condition set to Center: Matrix) was used to represent the range (−9.78, 9.78). In this study, three MV maps were sorted by HCT–R2E dendrograms (22) for 1921 protein expression on 98 patient’s data. The two proximity matrices are shown in Supplementary Figure S1.
Ingenuity Pathway Analysis (IPA) (QIAGEN company, Redwood City, CA, USA), a web-based computational platform, was used to conduct functional enrichment analysis of genes. A total of 1921 differentially expressed proteins (>2-fold change) were analyzed by the core analysis enrichment tool based on the IPA database. Canonical pathways and upstream regulator analysis found by core analysis in IPA were given a p-value.
In this study, we constructed a decision tree in Orange3 (https://orangedatamining.com/) to extract six essential proteins. The parameters of the decision tree in Orange3 were set to induce a binary tree which splits parent node into two child nodes, a minimum number of instances in leaves equal to 1, forbids the algorithm to split the nodes with less than 1 (do not split subsets smaller than = 1), and limit the maximal tree depth set to default. The visualization for the result also used the component of Tree Viewer in Orange3.
A total of 100 subjects were enrolled in the present study. The clinical demographics are listed in Table 1. The mean age was 62.2 years old, and 50% of them were male. The indications for colonoscopy included screening (67%), surveillance (3%), and symptomatic (30%). Thirteen healthy controls, 12 non-AAs, 13 AAs, 16 stage-I CRCs, 15 stage-II CRCs, 16 stage-III CRCs, and 15 stage-IV CRCs were eligible and were included in the study. Among all colorectal neoplasias, 33 (37.5%) lesions were located in the proximal colon, as confirmed by histological diagnosis.
The raw data of the sEV proteome containing 3353 proteins were obtained from the 100 patients. To minimize the influence of missing data on the correlation between proteins and patients, patients (rows) or proteins (columns) with missing data of more than 50% were excluded. Accordingly, a total of 1921 proteins from 98 patients were subjected to analysis as a sEV proteome dataset. The flowchart of protein selection is demonstrated in Figure 1. Because the expression difference in each of the 1921 sEV proteins was significant, standardization was used to normalize the protein expression data. Then, we used GAP, which integrated clustering methods and visualization of the data matrix, and two proximity matrices to analyze the relationship between patients and proteins. MV was employed for the demonstration of the sEV protein data matrix to achieve a better understanding of the association between the patients and proteins, and the result is illustrated in Figure 2A. Data from the normal subjects were mostly concentrated at the bottom of the data matrix plot, and approximately 40% of the analyzed proteins had prominently high expression. Apparently, data from the patients with early and advanced neoplasia were presented at the top of the data matrix plot; nevertheless, the data was mixed and difficult to distinguish from each other. To minimize the influence of the missing value (yellow), the missing values in the data matrix were imputed with the average expression value of each protein accordingly. The result after imputation is shown in Figure 2B. The patients with early and advanced neoplasia continued to display a different expression pattern of the sEV proteins from normal subjects. Based on these GAP results, we were confident in identifying the specific sEV proteins that distinguished patients with colorectal neoplasia from those who were classified as healthy and/or nonneoplastic.
Figure 2 Matrix visualization for exosome proteins also sorted by HCT–R2E seriations: 98 patients by 1921 proteins. The Euclidean distance map for 98 patients and the Pearson correlation map for 1921 proteins are not shown due to space limitations. (A) Matrix visualization before imputation. (B) Matrix visualization after imputation.
Next, we calculated the fold change (FC) and p-value < 0.05 of the 1921 sEV proteins from 98 normal and tumor patients and drew a volcano map, as shown in Figure 3. The blue dots in the figure represent proteins with a p-value less than 0.05 and log2 (FC) absolute value greater than 1. The proteins whose log2 (FC) was positive had higher expression in tumor status. Since we wanted to analyze the proteins with significant differences, 1054 proteins with a p-value less than 0.05 were selected for further examination. Among them, 170 were upregulated, and 884 proteins were downregulated in CRC neoplasia-derived sEVs.
To identify critical proteins capable of distinguishing three subcategories, normal, early neoplasia and advanced neoplasia, we used a decision tree to classify the sEV protein data (98 patients by 1054 proteins). The visualization of the decision tree result is shown in Figure 4A. We found that 6 sEV proteins (GCLM, KEL, APOF, CFB, PDE5A, and ATIC) can perfectly distinguish patients from the three different subcategories in a differential expression manner. Figure 4B presents the expression values of these 6 sEV proteins from 98 patients by MV. In Figure 4, the patients of normal group (red) can be distinguished by GCLMhigh and APOFlow, and GCLMlow, ATIClow/GCLMhigh, CFBlow can distinguish most patients with early neoplasia (green) and advanced neoplasia (blue), and additional predictors of early or advanced neoplasia are PED5Alow, KELhigh and PED5Ahigh, KELlow, respectively. Consistently, these six essential sEV proteins indicated by the red dots on the volcano map in Figure 3 are shown to be statistically significant.
Figure 4 Three status types were classified by an exosome protein data matrix for 98 patients. (A) The result of the decision tree. (B) Matrix visualization based on decision tree results.
The mechanistic properties and functions of sEVs in the serum from patients with colorectal neoplasia have not been widely investigated. To further investigate the oncogenic effects of sEV proteins, we performed a functional analysis of the sEV proteome, which included a total of 1921 sEV proteins that showed a statistically significant 2-fold expression difference between the normal and tumor groups. Using IPA for canonical pathway analysis, we conducted functional enrichment analysis of these differentially expressed proteins. The results showed that these differentially expressed proteins were enriched in clathrin-mediated signaling [-log10(p-value) = 28.2], integrin signaling [-log10(p-value) = 27.7], signaling by Rho family GTPases [-log10(p-value) = 21.4], ERK/MAPK signaling [-log10(p-value) = 11.7], and FAK signaling [-log10(p-value) = 11.7] (Figure 5). The cellular functions of the six proteins identified by IPA are shown in Table 2. The cellular functions and major pathways associated with these six proteins were the clathrin-mediated endocytosis signaling pathway and the complement system in CRC carcinogenesis.
The current study identified six differentially expressed sEV-associated proteins in the serum of patients with or without colorectal neoplasia, which may serve as biomarkers to diagnose these patients as early as possible. Moreover, several specific signaling pathways intimately related to the progression and development of CRC malignancies were consistently regulated by sEV-associated proteins. Conclusively, we explored the proteomic landscape of sEVs in the serum from patients with colorectal neoplasia with the goal of developing a novel blood test for early diagnosis and shedding new insight into the role of sEVs in CRC progression.
A number of previous studies have evaluated the presence of protein biomarkers in serum for detecting cancer by liquid chromatography coupled to tandem mass spectrometry (23–26). This high-throughput proteomic technique has become a powerful approach for biomarker discovery because it enables the identification and quantification of thousands of proteins within the same experiment. To minimize interference from the high abundance of common proteins in the blood, we focused our attention on serum sEVs, which may contain proteins specific to CRC, to identify meaningful biomarkers of CRC. The present study identified six sEV proteins, namely, GCLM, KEL, APOF, CFB, PDE5A, and ATIC, that could distinguish early neoplasia, advanced neoplasia, and normal controls from each other well. Thus, these six serum sEV proteins are capable of serving as components of a novel blood test to detect precancerous lesions (AAs) and CRC. In contrast, FIT detected CRC with a sensitivity of 79% (27), but the sensitivity decreased to 21–28% for detecting AA (7–9, 28). Moreover, methylated SEPT9 DNA (mSEPT9) is a plasma test approved by the Food and Drug Administration of the United States for detecting CRC. However, its sensitivity for detecting CRC and AA was only 48.2% and 11.2%, respectively (29). Given the suboptimal sensitivity for both CRC and precancerous lesions, the current guidelines, including the US Preventive Services Task Force and American College of Gastroenterology, suggest against the use of mSEPT9 for CRC screening (30, 31). Both FIT and mSEPT9 have limitations in detecting precancerous neoplasms. On the other hand, sEV proteins may distinguish early neoplasia, including AA and stage-I CRC, from normal control cells, thereby providing an opportunity for the development of a new blood test to resolve unmet clinical needs.
The present study identified six sEV proteins differentially expressed between patients with and without colorectal neoplasia and could distinguish AA and CRC from normal controls. The six sEV proteins included GCLM, APOF, ATIC, PDE5A, KEL, and CFB. Understanding the function of these proteins may help to clarify the role of EV in CRC carcinogenesis. GCLM is associated with glutathione biosynthesis and CRC formation. Because GCLM is a sensor of oxidative stress, this association is consistent with the notion that oxidative stress is related to CRC progression (32). APOF is a subtype of apolipoprotein and an essential regulator of cholesterol transport. APO is not only beneficial for diagnosis and prognosis prediction but also a potential therapeutic target in cancer. APO can be abnormally expressed in various cancers, including CRC. In combination with other molecules, APO is a possible biomarker for cancer detection (33). ATIC is a bifunctional enzyme that catalyzes the last two steps of purine biosynthesis. According to a report from The Human Protein Atlas, ATIC has a strong immunoreactivity in CRC tissue (https://www.proteinatlas.org/ENSG00000138363-ATIC). Moreover, ATIC is one of the differentially expressed proteins associated with drug response in CRC (34). PDE5A plays an essential role in signal transduction by regulating the intracellular concentration of cyclic nucleotides (35). Mutation of the PDE5A gene confers a survival advantage in patients with CRC (36). KEL is a zinc endopeptidase with endothelin-3-converting enzyme activity (37). A higher expression of KEL in rectal cancer is associated with worse survival (The Human Protein Atlas, https://www.proteinatlas.org/ENSG00000197993-KEL/pathology/colorectal+cancer/READ). CFB, complement factor B, is a component in the alternative and lectin pathway of the complement system. CFB affects the control of tumor growth, and the activation of the lectin pathway was increased significantly in patients with CRC compared to healthy subjects (38). These abovementioned proteins are encapsulated within sEVs and play a role in CRC carcinogenesis. It would be worthwhile to investigate their role as therapeutic targets in the future.
sEVs play an essential role in cell-cell communication locally and systemically. Growing evidence suggests that the reciprocal relationship between cancer cells and the surrounding nonneoplastic cells, called the tumor microenvironment, is critical for tumor development (39). Thus, great attention has been given to molecules that mediate the cross-talk between cancer cells and the surrounding microenvironment. The role of sEVs in the intercellular exchange of proteins within the cancer microenvironment may play a pivotal role in cancer development, progression, metastasis, and drug resistance in CRC (40, 41). Given the nature of sEVs in intercellular communication, the proteins carried by sEVs have biological functions and actively involved in carcinogenesis. The present study explored whether sEV proteins are involved in CRC carcinogenesis via clathrin-mediated endocytosis signaling, the complement system, integrin signaling, ERK/MAPK signaling, FAK signaling, and the complement system. The first two signaling pathways are significantly associated with two of the six EV proteins specific to colorectal neoplasia: APOF and CFB. The other three pathways have been proven to play a critical role in CRC carcinogenesis and progression (42–44).
The functions of the differentially expressed sEV proteins were enriched in the clathrin-mediated endocytosis signaling pathway. The sEV protein specific to colorectal neoplasia, APOF, indeed plays an important role in this related signaling pathway. The clathrin-mediated pathway is the primary pathway for internalizing nutrients, hormones, and other signaling molecules from the plasma membrane into the intracellular compartment and is partially responsible for the cellular immune response and pathogen-influenced signaling. In addition, MACC1 promoted cell proliferation and metastasis via a clathrin-mediated pathway in CRC (45). Taken together, clathrin-mediated signaling is associated with cellular maintenance and infectious disease. Consistently, the present study demonstrated that this pathway might play a role in CRC carcinogenesis through sEV proteins.
The other signaling pathway associated with the sEV proteins specific to colorectal neoplasia, namely, CFB, is the complement system. The complement system has traditionally been considered to be related to innate immune responses. Complement components can regulate the tumor microenvironment (TME), functioning as a bridge between tumor-promoting and tumor-suppressing immune responses. Indeed, the balance of the complement components is lost in the presence of cancer (46, 47). In other words, the expression of complement proteins increases in cancer cells, and complement activation in the TME may promote tumorigenesis and progression (48). The mechanism involved in complement activation in the TME remains unclear. The present study explored whether C1q+ sEVs increased significantly in subjects with colorectal neoplasia compared with those without. Complement C1q is the activator of the classical pathway. C1q is expressed in several human malignant tumor stroma and vascular endothelium and has been proven to promote cancer cell adhesion, migration, and proliferation (49). The complement proteins could interact with stromal cells in the TME. Tumor-associated macrophages (TAMs) have been reported to contribute to tumor progression (50). The complement proteins could activate and recruit macrophages into tumor tissues. C1q could induce macrophage polarization and suppress macrophage NLRP3 inflammasome activation (51). Complement proteins and TAMs mutually regulate each other. Moreover, recent studies showed that C1q-polarized macrophages expressed elevated levels of programmed death-ligand 1 (PD-L1) and PD-L2 and then suppressed the proliferation of human allogeneic inflammatory T cells (52). Given that PD-L1 is a target molecule for immunotherapy (53), it will be intriguing to investigate the implications of C1q+ sEVs in immunotherapy.
Integrin signaling, ERK/MAPK signaling, and FAK signaling are well known to be associated with carcinogenesis. Among these three pathways, integrins work as upstream signaling molecules of the other two pathways. Integrins belong to heterodimeric cell surface adhesion receptors, which are composed of noncovalently associated α and β subunits, and the integrin family consists of 18α and 8β members (54). Each integrin has multiple activation states and exerts effects via a cascade of downstream amplification pathways (55). Growing evidence reveals that integrins enable signaling through the cell membrane bidirectionally in addition to physically linking the intracellular cytoskeleton and extracellular matrices (56), thereby controlling cell attachment, movement, growth, differentiation, and survival (57). As cell adhesion receptors, integrins have been reported to be associated with various cancers, including CRC (42). In the present study, we found that the expression of the integrin subunits ITGAM and ITGB2 was increased in the sEVs of patients with colorectal neoplasia. Gong et al. demonstrated that ITGAM was highly expressed in CRC lesions, indicating that the expression of ITGAM is suitable for the diagnosis and prognosis of CRC (42). In addition, Benedicto et al. found that reduced ITGB2 expression was associated with reduced proliferation, adhesion, and migration in various CRC cell lines. This finding suggested a vital role of ITGB2 during CRC metastasis (58). In agreement with the above observations, sEVs, which contain ITGAM and ITGB2, were increased in the serum of patients with colorectal neoplasia, implying that sEVs play a specific role in the expression of these two proteins that participate in the development of cancer malignancies, such as metastasis. Moreover, it is worth investigating whether ITGB2+ EVs in serum can be useful for the diagnosis of metastatic CRC.
The ERK/MARK signaling pathway is one of the most important pathways for cell proliferation (59). This pathway is located downstream of many growth factor receptors, including epidermal growth factor and integrins. Activation of this signaling pathway is essential in intestinal epithelial differentiation (60). There is growing evidence that indicates that the activation of the ERK/MAPK pathway is involved in the pathogenesis, progression, and oncogenic characteristics of CRC (43). Our functional proteomic analysis indicated that the expression of sEVs containing the PP1 subunits PPP1CA and PPP1R12A was decreased in subjects with colorectal neoplasia. The decrease in PP1+ EVs may minimize their inhibitory effect on ERK/MARK signaling and subsequently promote CRC progression. This hypothesis is supported by a previous study, which demonstrated that lower expression of PPP1R12A was associated with worse recurrence-free survival in CRC (61). FAK, a key mediator of integrin and growth factor signal transduction, is activated directly by SRC kinase (62). The kinase complex of FAK-SRC is reportedly attributed to the activation of downstream pathways, such as PI3K, AKT, and ERK/MARK (63, 64). For example, FAK signaling through the ERK/MARK pathway is needed to promote cancer cell development (65). Moreover, the FAK-SRC complex might induce MMP2 and MMP9 and subsequently increase the invasiveness of cancer cells (66). The present study demonstrated that the expression of the FAK-SRC complex was increased in serum sEVs in patients with colorectal neoplasia. In summary, we investigated whether the differentially expressed sEV proteins may promote the proliferation and metastasis of CRC through upstream integrin signaling, followed by FAK signaling and ERK/MARK signaling. These differentially expressed sEV proteins in patients with colorectal neoplasia will be potential therapeutic targets and worth further investigation in the future.
The strength of the present study is that we explored the proteomic landscape of sEVs in the serum of patients with colorectal neoplasia. The exploration of the proteome in CRC tissue has been conducted in a previous study (67). However, proteomic investigations in serum have been less well-studied in CRC. The advantage of the blood-based approach is that it is more feasible to use the specific sEV proteins as biomarkers for the establishment of a diagnostic test than those from tissue samples. Moreover, six sEV proteins specific to colorectal neoplasia were identified, and these molecules prominently distinguished colorectal neoplasia from the normal control. Our results provide an opportunity to develop a novel blood test for detecting CRC. However, the diagnostic performance, including the sensitivity, specificity, and accuracy of the specific sEV proteins, needs to be validated in the next stage. Thus, one of the limitations of the present study is the lack of validation experiments to support the diagnostic performance of the blood panel test containing the six sEV proteins. Furthermore, the true or false positive rate of the blood panel compared with FIT should be investigated. Another limitation of the present study is that IPA was used to identify the signaling pathways. The biological function of the differentially expressed sEV proteins in the signaling pathways needs to be further validated in the future.
In conclusion, the present study explored whether patients with colorectal neoplasia had distinct expression patterns in the sEV proteome from the serum of patients with colorectal neoplasia compared with those without. Furthermore, six of the differentially expressed sEV proteins were shown to behave as biomarkers to distinguish early neoplasia, advanced neoplasia, and normal controls from each other. These specific sEV proteins have the potential to be used in the development of a novel blood test for detecting colorectal neoplasia at early stages. Finally, the association of the sEV proteome with colorectal neoplasia in CRC carcinogenesis via specific signaling pathways was explored. These findings will help improve our understanding of the role of sEV proteins in CRC development and identify candidate therapeutic targets in the future.
The original contributions presented in the study are included in the article/Supplementary Material. Further inquiries can be directed to the corresponding authors.
The studies involving human participants were reviewed and approved by National Taiwan University Hospital, No. 201906020RINC. The patients/participants provided their written informed consent to participate in this study.
Conceptualization: T-LS, M-SW, and H-MC. Data curation: KU and H-MC. Formal analysis: Y-CH and C-HK. Funding acquisition: H-MC, T-LS, and L-CC. Investigation: T-LS, KU, and L-CC. Methodology: T-LS, Y-CH, C-HK, and KU. Resources: T-LS, H-MC, and M-SW. Supervision: T-LS. Writing: LC, TS, Y-CH, and C-HK. All authors contributed to the article and approved the submitted version.
This work was supported by grants from the Ministry of Science and Technology, Taiwan (MOST109-2314-B-002-085 to L-CC; 110-2321-B-002-014 to M-SW; 105-2320-B-002-058-MY3 to T-LS).
The authors declare that the research was conducted in the absence of any commercial or financial relationships that could be construed as a potential conflict of interest.
All claims expressed in this article are solely those of the authors and do not necessarily represent those of their affiliated organizations, or those of the publisher, the editors and the reviewers. Any product that may be evaluated in this article, or claim that may be made by its manufacturer, is not guaranteed or endorsed by the publisher.
The Supplementary Material for this article can be found online at: https://www.frontiersin.org/articles/10.3389/fonc.2021.732743/full#supplementary-material
CRC, colorectal cancer; FIT, fecal immunochemical test; EV, extracellular vesicle; AA, advanced adenoma; LC-MS, liquid chromatography-tandem mass spectrometry; AGC, automatic gain control; GAP, generalized association plot; MV, Matrix visualization; IPA, Ingenuity Pathway Analysis; mSEPT9, methylated SEPT9 DNA; TME, tumor microenvironment; TAM, tumor-associated macrophage.
1. Deshields TL, Wells-Di Gregorio S, Flowers SR, Irwin KE, Nipp R, Padgett L, et al. Addressing Distress Management Challenges: Recommendations From the Consensus Panel of the American Psychosocial Oncology Society and the Association of Oncology Social Work. CA Cancer J Clin (2021). doi: 10.3322/caac.21672
2. Edwards BK, Ward E, Kohler BA, Eheman C, Zauber AG, Anderson RN, et al. Annual Report to the Nation on the Status of Cancer, 1975-2006, Featuring Colorectal Cancer Trends and Impact of Interventions (Risk Factors, Screening, and Treatment) to Reduce Future Rates. Cancer (2010) 116:544–73. doi: 10.1002/cncr.24760
3. Siegel RL, Miller KD, Goding Sauer A, Fedewa SA, Butterly LF, Anderson JC, et al. Colorectal Cancer Statistics, 2020. CA Cancer J Clin (2020) 70:145–64. doi: 10.3322/caac.21601
4. Singh H, Nugent Z, Demers AA, Kliewer EV, Mahmud SM, Bernstein CN. The Reduction in Colorectal Cancer Mortality After Colonoscopy Varies by Site of the Cancer. Gastroenterology (2010) 139:1128–37. doi: 10.1053/j.gastro.2010.06.052
5. Kahi CJ, Imperiale TF, Juliar BE, Rex DK. Effect of Screening Colonoscopy on Colorectal Cancer Incidence and Mortality. Clin Gastroenterol Hepatol (2009) 7:770–5; quiz 711. doi: 10.1016/j.cgh.2008.12.030
6. Quintero E, Castells A, Bujanda L, Cubiella J, Salas D, Lanas A, et al. Colonoscopy Versus Fecal Immunochemical Testing in Colorectal-Cancer Screening. N Engl J Med (2012) 366:697–706. doi: 10.1056/NEJMoa1108895
7. Imperiale TF, Ransohoff DF, Itzkowitz SH, Levin TR, Lavin P, Lidgard GP, et al. Multitarget Stool DNA Testing for Colorectal-Cancer Screening. N Engl J Med (2014) 370:1287–97. doi: 10.1056/NEJMoa1311194
8. Chiu HM, Lee YC, Tu CH, Chen CC, Tseng PH, Liang JT, et al. Association Between Early Stage Colon Neoplasms and False-Negative Results From the Fecal Immunochemical Test. Clin Gastroenterol Hepatol (2013) 11:832–8.e1-2. doi: 10.1016/j.cgh.2013.01.013
9. Hernandez V, Cubiella J, Gonzalez-Mao MC, Iglesias F, Rivera C, Iglesias MB, et al. Fecal Immunochemical Test Accuracy in Average-Risk Colorectal Cancer Screening. World J Gastroenterol (2014) 20:1038–47. doi: 10.3748/wjg.v20.i4.1038
10. Ahn SB, Sharma S, Mohamedali A, Mahboob S, Redmond WJ, Pascovici D, et al. Potential Early Clinical Stage Colorectal Cancer Diagnosis Using a Proteomics Blood Test Panel. Clin Proteomics (2019) 16:34. doi: 10.1186/s12014-019-9255-z
11. Wolf AMD, Fontham ETH, Church TR, Flowers CR, Guerra CE, LaMonte SJ, et al. Colorectal Cancer Screening for Average-Risk Adults: 2018 Guideline Update From the American Cancer Society. CA Cancer J Clin (2018) 68:250–81. doi: 10.3322/caac.21457
12. Symonds EL, Hughes D, Flight I, Woodman R, Chen G, Ratcliffe J, et al. A Randomized Controlled Trial Testing Provision of Fecal and Blood Test Options on Participation for Colorectal Cancer Screening. Cancer Prev Res (Phila) (2019) 12:631–40. doi: 10.1158/1940-6207.CAPR-19-0089
13. Tkach M, Thery C. Communication by Extracellular Vesicles: Where We Are and Where We Need to Go. Cell (2016) 164:1226–32. doi: 10.1016/j.cell.2016.01.043
14. Chen IH, Xue L, Hsu CC, Paez JS, Pan L, Andaluz H, et al. Phosphoproteins in Extracellular Vesicles as Candidate Markers for Breast Cancer. Proc Natl Acad Sci USA (2017) 114:3175–80. doi: 10.1073/pnas.1618088114
15. Melo SA, Luecke LB, Kahlert C, Fernandez AF, Gammon ST, Kaye J, et al. Glypican-1 Identifies Cancer Exosomes and Detects Early Pancreatic Cancer. Nature (2015) 523:177–82. doi: 10.1038/nature14581
16. Li J, Li B, Ren C, Chen Y, Guo X, Zhou L, et al. The Clinical Significance of Circulating GPC1 Positive Exosomes and its Regulative miRNAs in Colon Cancer Patients. Oncotarget (2017) 8:101189–202. doi: 10.18632/oncotarget.20516
17. Tian Y, Ma L, Gong M, Su G, Zhu S, Zhang W, et al. Protein Profiling and Sizing of Extracellular Vesicles From Colorectal Cancer Patients via Flow Cytometry. ACS Nano (2018) 12:671–80. doi: 10.1021/acsnano.7b07782
18. Wilkins MR, Sanchez JC, Gooley AA, Appel RD, Humphery-Smith I, Hochstrasser DF, et al. Progress With Proteome Projects: Why All Proteins Expressed by a Genome Should be Identified and How to do it. Biotechnol Genet Eng Rev (1996) 13:19–50. doi: 10.1080/02648725.1996.10647923
19. Nagtegaal ID, Odze RD, Klimstra D, Paradis V, Rugge M, Schirmacher P, et al. The 2019 WHO Classification of Tumours of the Digestive System. Histopathology (2020) 76:182–8. doi: 10.1111/his.13975
20. Chen CH. Generalized Association Plots: Information Visualization via Iteratively Generated Correlation Matrices. Statistica Sin (2002) 12:7–29.
21. Wu HM, Tien YJ, Chen CH. GAP: A Graphical Environment for Matrix Visualization and Cluster Analysis. Comput Stat Data Anal (2010) 54:767–78. doi: 10.1016/j.csda.2008.09.029
22. Tien YJ, Lee YS, Wu HM, Chen CH. Methods for Simultaneously Identifying Coherent Local Clusters With Smooth Global Patterns in Gene Expression Profiles. BMC Bioinf (2008) 9:155. doi: 10.1186/1471-2105-9-155
23. Arbelaiz A, Azkargorta M, Krawczyk M, Santos-Laso A, Lapitz A, Perugorria MJ, et al. Serum Extracellular Vesicles Contain Protein Biomarkers for Primary Sclerosing Cholangitis and Cholangiocarcinoma. Hepatology (2017) 66:1125–43. doi: 10.1002/hep.29291
24. Ong ES, Len SM, Lee AC. Differential Protein Expression of the Inhibitory Effects of a Standardized Extract From Scutellariae Radix in Liver Cancer Cell Lines Using Liquid Chromatography and Tandem Mass Spectrometry. J Agric Food Chem (2005) 53:8–16. doi: 10.1021/jf049172w
25. Ralhan R, Desouza LV, Matta A, Tripathi SC, Ghanny S, Datta Gupta S, et al. Discovery and Verification of Head-and-Neck Cancer Biomarkers by Differential Protein Expression Analysis Using iTRAQ Labeling, Multidimensional Liquid Chromatography, and Tandem Mass Spectrometry. Mol Cell Proteomics (2008) 7:1162–73. doi: 10.1074/mcp.M700500-MCP200
26. Coskun E, Jaruga P, Jemth AS, Loseva O, Scanlan LD, Tona A, et al. Addiction to MTH1 Protein Results in Intense Expression in Human Breast Cancer Tissue as Measured by Liquid Chromatography-Isotope-Dilution Tandem Mass Spectrometry. DNA Repair (Amst) (2015) 33:101–10. doi: 10.1016/j.dnarep.2015.05.008
27. Lee JK, Liles EG, Bent S, Levin TR, Corley DA. Accuracy of Fecal Immunochemical Tests for Colorectal Cancer: Systematic Review and Meta-Analysis. Ann Intern Med (2014) 160:171. doi: 10.7326/M13-1484
28. Brenner H, Tao S. Superior Diagnostic Performance of Faecal Immunochemical Tests for Haemoglobin in a Head-to-Head Comparison With Guaiac Based Faecal Occult Blood Test Among 2235 Participants of Screening Colonoscopy. Eur J Cancer (2013) 49:3049–54. doi: 10.1016/j.ejca.2013.04.023
29. Church TR, Wandell M, Lofton-Day C, Mongin SJ, Burger M, Payne SR, et al. Prospective Evaluation of Methylated SEPT9 in Plasma for Detection of Asymptomatic Colorectal Cancer. Gut (2014) 63:317–25. doi: 10.1136/gutjnl-2012-304149
30. Shaukat A, Kahi CJ, Burke CA, Rabeneck L, Sauer BG, Rex DK. ACG Clinical Guidelines: Colorectal Cancer Screening 2021. Am J Gastroenterol (2021) 116:458–79. doi: 10.14309/ajg.0000000000001122
31. Force U.S.P.S.T., Bibbins-Domingo K, Grossman DC, Curry SJ, Davidson KW, Epling JW Jr., et al. Screening for Colorectal Cancer: US Preventive Services Task Force Recommendation Statement. JAMA (2016) 315:2564–75. doi: 10.1001/jama.2016.5989
32. Tatebe S, Unate H, Sinicrope FA, Sakatani T, Sugamura K, Makino M, et al. Expression of Heavy Subunit of Gamma-Glutamylcysteine Synthetase (Gamma-GCSh) in Human Colorectal Carcinoma. Int J Cancer (2002) 97:21–7. doi: 10.1002/ijc.1574
33. Ren L, Yi J, Li W, Zheng X, Liu J, Wang J, et al. Apolipoproteins and Cancer. Cancer Med (2019) 8:7032–43. doi: 10.1002/cam4.2587
34. Chen Y, Ni J, Gao Y, Zhang J, Liu X, Chen Y, et al. Integrated Proteomics and Metabolomics Reveals the Comprehensive Characterization of Antitumor Mechanism Underlying Shikonin on Colon Cancer Patient-Derived Xenograft Model. Sci Rep (2020) 10:14092. doi: 10.1038/s41598-020-71116-5
35. Loughney K, Hill TR, Florio VA, Uher L, Rosman GJ, Wolda SL, et al. Isolation and Characterization of cDNAs Encoding PDE5A, a Human cGMP-Binding, cGMP-Specific 3',5'-Cyclic Nucleotide Phosphodiesterase. Gene (1998) 216:139–47. doi: 10.1016/S0378-1119(98)00303-5
36. Lehrer S, Rheinstein PH, Rosenzweig KE. Mutations of the PDE5A Gene Confer a Survival Advantage in Patients With Colon Cancer. Cancer Prev Res (Phila) (2018) 11:439–40. doi: 10.1158/1940-6207.CAPR-18-0105
37. Lee S, Lin M, Mele A, Cao Y, Farmar J, Russo D, et al. Proteolytic Processing of Big Endothelin-3 by the Kell Blood Group Protein. Blood (1999) 94:1440–50. doi: 10.1182/blood.V94.4.1440
38. Ytting H, Jensenius JC, Christensen IJ, Thiel S, Nielsen HJ. Increased Activity of the Mannan-Binding Lectin Complement Activation Pathway in Patients With Colorectal Cancer. Scand J Gastroenterol (2004) 39:674–9. doi: 10.1080/00365520410005603
39. Bissell MJ, Hines WC. Why Don't We Get More Cancer? A Proposed Role of the Microenvironment in Restraining Cancer Progression. Nat Med (2011) 17:320–9. doi: 10.1038/nm.2328
40. Siveen KS, Raza A, Ahmed EI, Khan AQ, Prabhu KS, Kuttikrishnan S, et al. The Role of Extracellular Vesicles as Modulators of the Tumor Microenvironment, Metastasis and Drug Resistance in Colorectal Cancer. Cancers (Basel) (2019) 11(6):746. doi: 10.3390/cancers11060746
41. Howcroft TK, Zhang HG, Dhodapkar M, Mohla S. Vesicle Transfer and Cell Fusion: Emerging Concepts of Cell-Cell Communication in the Tumor Microenvironment. Cancer Biol Ther (2011) 12:159–64. doi: 10.4161/cbt.12.3.17032
42. Gong YZ, Ruan GT, Liao XW, Wang XK, Liao C, Wang S, et al. Diagnostic and Prognostic Values of Integrin Alpha Subfamily mRNA Expression in Colon Adenocarcinoma. Oncol Rep (2019) 42:923–36. doi: 10.3892/or.2019.7216
43. Wang X, Wang Q, Hu W, Evers BM. Regulation of Phorbol Ester-Mediated TRAF1 Induction in Human Colon Cancer Cells Through a PKC/RAF/ERK/NF-kappaB-Dependent Pathway. Oncogene (2004) 23:1885–95. doi: 10.1038/sj.onc.1207312
44. Huang K, Gao N, Bian D, Zhai Q, Yang P, Li M, et al. Correlation Between FAK and EGF-Induced EMT in Colorectal Cancer Cells. J Oncol (2020) 2020:5428920. doi: 10.1155/2020/5428920
45. Imbastari F, Dahlmann M, Sporbert A, Mattioli CC, Mari T, Scholz F, et al. MACC1 Regulates Clathrin-Mediated Endocytosis and Receptor Recycling of Transferrin Receptor and EGFR in Colorectal Cancer. Cell Mol Life Sci (2021) 78:3525–42. doi: 10.1007/s00018-020-03734-1
46. Ajona D, Ortiz-Espinosa S, Pio R. Complement Anaphylatoxins C3a and C5a: Emerging Roles in Cancer Progression and Treatment. Semin Cell Dev Biol (2019) 85:153–63. doi: 10.1016/j.semcdb.2017.11.023
47. Bajic G, Degn SE, Thiel S, Andersen GR. Complement Activation, Regulation, and Molecular Basis for Complement-Related Diseases. EMBO J (2015) 34:2735–57. doi: 10.15252/embj.201591881
48. Markiewski MM, DeAngelis RA, Benencia F, Ricklin-Lichtsteiner SK, Koutoulaki A, Gerard C, et al. Modulation of the Antitumor Immune Response by Complement. Nat Immunol (2008) 9:1225–35. doi: 10.1038/ni.1655
49. Bulla R, Tripodo C, Rami D, Ling GS, Agostinis C, Guarnotta C, et al. C1q Acts in the Tumour Microenvironment as a Cancer-Promoting Factor Independently of Complement Activation. Nat Commun (2016) 7:10346. doi: 10.1038/ncomms10346
50. Mantovani A, Marchesi F, Malesci A, Laghi L, Allavena P. Tumour-Associated Macrophages as Treatment Targets in Oncology. Nat Rev Clin Oncol (2017) 14:399–416. doi: 10.1038/nrclinonc.2016.217
51. Benoit ME, Clarke EV, Morgado P, Fraser DA, Tenner AJ. Complement Protein C1q Directs Macrophage Polarization and Limits Inflammasome Activity During the Uptake of Apoptotic Cells. J Immunol (2012) 188:5682–93. doi: 10.4049/jimmunol.1103760
52. Clarke EV, Weist BM, Walsh CM, Tenner AJ. Complement Protein C1q Bound to Apoptotic Cells Suppresses Human Macrophage and Dendritic Cell-Mediated Th17 and Th1 T Cell Subset Proliferation. J Leukoc Biol (2015) 97:147–60. doi: 10.1189/jlb.3A0614-278R
53. Gou Q, Dong C, Xu H, Khan B, Jin J, Liu Q, et al. PD-L1 Degradation Pathway and Immunotherapy for Cancer. Cell Death Dis (2020) 11:955. doi: 10.1038/s41419-020-03140-2
54. Arnaout MA. Integrin Structure: New Twists and Turns in Dynamic Cell Adhesion. Immunol Rev (2002) 186:125–40. doi: 10.1034/j.1600-065X.2002.18612.x
55. Goodman SL, Picard M. Integrins as Therapeutic Targets. Trends Pharmacol Sci (2012) 33:405–12. doi: 10.1016/j.tips.2012.04.002
56. Hynes RO. Integrins: Bidirectional, Allosteric Signaling Machines. Cell (2002) 110:673–87. doi: 10.1016/S0092-8674(02)00971-6
57. Legate KR, Wickstrom SA, Fassler R. Genetic and Cell Biological Analysis of Integrin Outside-in Signaling. Genes Dev (2009) 23:397–418. doi: 10.1101/gad.1758709
58. Benedicto A, Marquez J, Herrero A, Olaso E, Kolaczkowska E, Arteta B. Decreased Expression of the Beta2 Integrin on Tumor Cells is Associated With a Reduction in Liver Metastasis of Colorectal Cancer in Mice. BMC Cancer (2017) 17:827. doi: 10.1186/s12885-017-3823-2
59. Troppmair J, Bruder JT, Munoz H, Lloyd PA, Kyriakis J, Banerjee P, et al. Mitogen-Activated Protein Kinase/Extracellular Signal-Regulated Protein Kinase Activation by Oncogenes, Serum, and 12-O-Tetradecanoylphorbol-13-Acetate Requires Raf and is Necessary for Transformation. J Biol Chem (1994) 269:7030–5. doi: 10.1016/S0021-9258(17)37478-1
60. Taupin D, Podolsky DK. Mitogen-Activated Protein Kinase Activation Regulates Intestinal Epithelial Differentiation. Gastroenterology (1999) 116:1072–80. doi: 10.1016/S0016-5085(99)70010-7
61. Zhang C, Li A, Li H, Peng K, Wei Q, Lin M, et al. PPP1R12A Copy Number Is Associated With Clinical Outcomes of Stage III CRC Receiving Oxaliplatin-Based Chemotherapy. Mediators Inflamm (2015) 2015:417184. doi: 10.1155/2015/417184
62. Dunty JM, Gabarra-Niecko V, King ML, Ceccarelli DF, Eck MJ, Schaller MD. FERM Domain Interaction Promotes FAK Signaling. Mol Cell Biol (2004) 24:5353–68. doi: 10.1128/MCB.24.12.5353-5368.2004
63. Yoon H, Dehart JP, Murphy JM, Lim ST. Understanding the Roles of FAK in Cancer: Inhibitors, Genetic Models, and New Insights. J Histochem Cytochem (2015) 63:114–28. doi: 10.1369/0022155414561498
64. Bianconi D, Unseld M, Prager GW. Integrins in the Spotlight of Cancer. Int J Mol Sci (2016) 17(12):2037. doi: 10.3390/ijms17122037
65. Zheng Y, Xia Y, Hawke D, Halle M, Tremblay ML, Gao X, et al. FAK Phosphorylation by ERK Primes Ras-Induced Tyrosine Dephosphorylation of FAK Mediated by PIN1 and PTP-PEST. Mol Cell (2009) 35:11–25. doi: 10.1016/j.molcel.2009.06.013
66. Van Slambrouck S, Grijelmo C, De Wever O, Bruyneel E, Emami S, Gespach C, et al. Activation of the FAK-Src Molecular Scaffolds and p130Cas-JNK Signaling Cascades by Alpha1-Integrins During Colon Cancer Cell Invasion. Int J Oncol (2007) 31:1501–8. doi: 10.3892/ijo.31.6.1501
Keywords: colorectal cancer, blood test, small extracellular vesicle, proteome, biomarker
Citation: Chang L-C, Hsu Y-C, Chiu H-M, Ueda K, Wu M-S, Kao C-H and Shen T-L (2021) Exploration of the Proteomic Landscape of Small Extracellular Vesicles in Serum as Biomarkers for Early Detection of Colorectal Neoplasia. Front. Oncol. 11:732743. doi: 10.3389/fonc.2021.732743
Received: 29 June 2021; Accepted: 24 August 2021;
Published: 13 September 2021.
Edited by:
Dianzheng Zhang, Philadelphia College of Osteopathic Medicine (PCOM), United StatesReviewed by:
Edouard Nice, Monash University, AustraliaCopyright © 2021 Chang, Hsu, Chiu, Ueda, Wu, Kao and Shen. This is an open-access article distributed under the terms of the Creative Commons Attribution License (CC BY). The use, distribution or reproduction in other forums is permitted, provided the original author(s) and the copyright owner(s) are credited and that the original publication in this journal is cited, in accordance with accepted academic practice. No use, distribution or reproduction is permitted which does not comply with these terms.
*Correspondence: Chiun-How Kao, MTU3Mjk0QG1haWwudGt1LmVkdS50dw==; Tang-Long Shen, c2hlbnRsQG50dS5lZHUudHc=
Disclaimer: All claims expressed in this article are solely those of the authors and do not necessarily represent those of their affiliated organizations, or those of the publisher, the editors and the reviewers. Any product that may be evaluated in this article or claim that may be made by its manufacturer is not guaranteed or endorsed by the publisher.
Research integrity at Frontiers
Learn more about the work of our research integrity team to safeguard the quality of each article we publish.