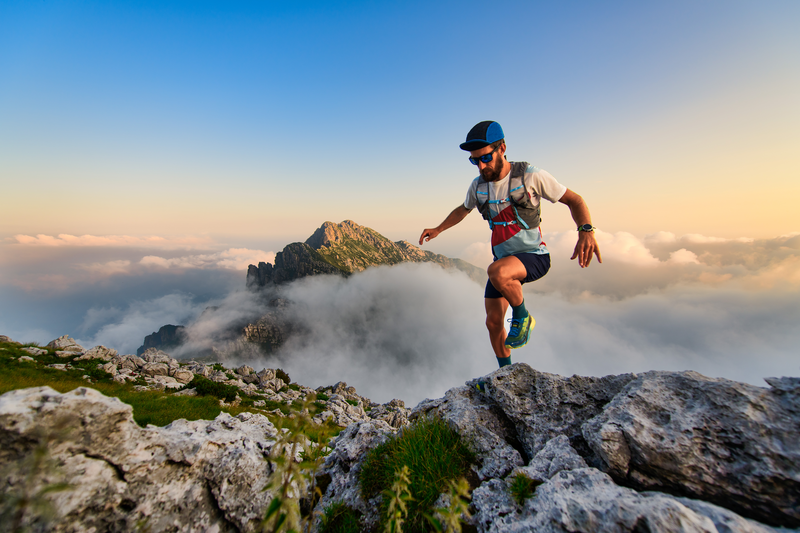
94% of researchers rate our articles as excellent or good
Learn more about the work of our research integrity team to safeguard the quality of each article we publish.
Find out more
ORIGINAL RESEARCH article
Front. Oncol. , 26 August 2021
Sec. Gastrointestinal Cancers
Volume 11 - 2021 | https://doi.org/10.3389/fonc.2021.731144
This article is part of the Research Topic Hepatocellular Carcinoma: from Basic Research to Clinical Trials View all 24 articles
Purpose: Gamma-glutamyl transpeptidase (GGT) family genes play crucial roles in the formation and progression of several solid tumors. However, the expression patterns and the prognostic significance of GGT members in hepatocellular carcinoma (HCC) remain unknown. This study was designed to determine the expression profiles of GGT family members in HCC and validate the prognostic value of serum GGT protein in patients with HCC.
Method: We comprehensively searched public resources based on the LIHC dataset to determine the expression patterns, prognostic significance, DNA methylation status, immune infiltration, and biological pathways of GGT family genes in HCC. Subsequently, we validated the prognostic value of serum GGT protein in 85 patients with early-stage HCC subjected to curative hepatectomy from the Renmin Hospital of Wuhan University.
Results: Except for GGT1, other GGT family members (GGT5, GGT6, and GGT7) were found to be differentially expressed in primary HCC samples (N = 371) and normal control tissues (N = 50). Furthermore, a positive relationship was not only observed between GGT1 and GGT5 (Spearman coefficient: 0.24, P = 5.143 × 10−6) but also between GGT5 and GGT6 (Spearman coefficient: 0.38, P = 1.24 × 10−13). The expression of GGT1, GGT5, and GGT7 was correlated with overall survival (OS), and GGT7 was associated with disease-free survival (DFS) in patients with HCC. Negative associations between DNA methylation and expression of mRNA were observed for GGT1 (Spearman coefficient: −0.38, P = 6.24e-14), GGT6 (Spearman coefficient: −0.29, P = 1.23e-8), and GGT7 (Spearman coefficient: −0.34, P=6.7e-11). GGT family genes were well correlated with the infiltration levels of immune cells in HCC, especially CD4+ T cells, macrophages, and dendritic cells. Finally, when validated with clinical data from the Renmin cohort, a high expression of serum GGT protein was identified as a strong prognostic element of unfavorable OS (HR = 3.114, P = 0.025), but not of DFS (HR = 1.198, P = 0.05) in patients with HCC subjected to curative hepatectomy.
Conclusion: To our knowledge, this is the first comprehensive analysis of the expression patterns and clinical value of GGT family genes in patients with HCC. Our study laid the foundation for the clinical application of the GGT protein in the survival assessment of patients with HCC.
Liver cancer is the fourth leading cause of cancer-related mortalities worldwide, after lung cancer, colorectal cancer, and stomach cancer (1). Hepatocellular carcinoma (HCC) is the most common pathological type of primary liver cancer, followed by cholangiocarcinoma. The major risk factors of HCC include viral hepatitis (hepatitis B virus, hepatitis C virus), metabolic factors (non-alcoholic fatty liver disease), behavioral factors (alcoholism, frequent smoke), and aflatoxins. Although considerable achievements have been attained in the field of anti-HCC, including surgical removal, molecular targeted therapy, and chemoradiotherapy, the long-term survival outcomes of individuals with HCC have not met our expectations owing to early metastasis (2). Therefore, identifying serum biomarkers that are specific to the survival outcomes of patients with HCC is quite desirable.
α-Fetoprotein (AFP) has long been recognized as a diagnostic biomarker for HCC but was recently excluded from the surveillance criteria of several authoritative HCC management consensus (3, 4). As the overexpression of AFP is only observed in approximately 50% of patients with HCC, identifying other serum biomarkers is imperative. Gamma-glutamyl transpeptidase (GGT) is a crucial liver enzyme involved in glutathione metabolism and is responsible for the extracellular cleavage of glutathione (5). GGT is a common liver enzyme located on the surface of most cells but is the most commonly found in hepatocytes (6). GGT is routinely utilized in clinical practice to assist clinicians in identifying the presence of liver injury. However, increased serum GGT is reported to be associated with metabolic syndrome (7), chronic kidney disease (8, 9), dementia (10, 11), and even malignant tumors (12). Recently, GGT has been demonstrated as the hallmark of oxidative stress; this enzyme can induce pro-oxidant reactions, which play an essential role in tumor formation and cell proliferation (13). Although several clinical studies have revealed the association between serum GGT and overall survival (OS) in patients with HCC (14–17), the overall prognostic effect of serum GGT remains uncertain in patients with HCC. Moreover, the expression profiles of GGT family genes in liver tissues and the prognostic values of GGT family genes in patients with HCC have never been investigated. Hence, the primary goal of this study was to investigate the expression profiles, prognostic role, DNA methylation, immune infiltration, and potential biological pathways of GGT family members in HCC via mining data from publicly accessible datasets. The second aim was to validate the prognostic value of serum GGT protein in patients with HCC based on our cohort.
We retrospectively gathered clinical data of patients with early-stage HCC (TNM I–II) from Renmin Hospital of Wuhan University from 2012 to 2016. The inclusion criteria were (1) patients with early-stage HCC (2), patients that underwent curative resection, and (3) patients who were tested for serum GGT protein. The exclusion criteria were (1) patients with cholangiocarcinoma (2), patients with other malignant tumors (3), patients without follow-up data, and (4) patients with advanced TNM stage HCC (TNM III–IV). The detailed clinicopathological data obtained from the Electronic Medical Record System were age at diagnosis, gender, levels of serum GGT, histological stage, tumor size, TNM stage, HBV infection, and serum AFP. All patients with HCC in our cohort were required to complete regular follow-ups after surgical resection. Patients with HCC who did not visit our outpatient department as scheduled were called for follow-ups to determine the living or recurrent status. OS and disease-free survival (DFS) were recorded during the follow-up. Based on the above criteria, 85 cases with primary HCC from the Renmin cohort were finally included in our analysis. Our study plan was strictly screened by the Ethical Committee of Renmin Hospital of Wuhan University (No. WDRY-2019-K104).
UALCAN (http://ualcan.path.uab.edu) is an interactive and comprehensive web tool for analyzing cancer OMICS data (TCGA, CPTAC, and MET500) that provide users with easy access to public cancer data (18). In this study, we employed this database via its methylation module to gain information about the differential expression of four GGT family genes and the methylation status between liver cancerous tissues and pathological normal tissues.
GEPIA (http://gepia.cancer-pku.cn) is a newly developed webpage for analyzing the RNA sequencing expression data from the GTEx and TCGA projects (19). GEPIA provides users with various functions, including differential expression analysis, survival analysis, and correlation analysis. We utilized the survival module via the GEPIA webpage to assess the associations between GGT family genes (GGT1, GGT5, GGT6, and GGT7) and survival outcomes (OS and DFS) of patients with HCC.
The cBioPortal for Cancer Genomics, a very powerful database (http://www.cbioportal.org/), is designed to provide consumers with visual analysis of large-scale cancer genomic datasets (20). This powerful database was employed to determine the association between two GGT family members and the correlation between DNA methylation and mRNA expression of the GGT family genes. Moreover, this genetic database was used to investigate the correlations between the expression of GGT family genes and copy number, as well as mutations.
UCSC Xena (http://xena.ucsc.edu), designed by the University of California–Santa Cruz, is an online exploration webpage for visualizing tumor data (21). We used this database to retrieve the clinical information and RNA-sequencing data of GGT family genes in TCGA-LIHC dataset. This powerful database was also employed, via its visualization module, to determine the distribution of the CpG sites of four GGT family genes in HCC.
TIMER database (https://cistrome.shinyapps.io/timer/) is a comprehensive resource for systemic analysis of six immune infiltrates (CD4+ T cells, B cells, CD8+ T cells, macrophages, neutrophils, and dendritic cells) across diverse cancer types (22). TIMER database allows users to freely input specific parameters, resulting in a picture display to conveniently assess tumor immunological, clinical, and genomic features. We utilized the gene module to determine the correlations between four GGT family genes and immune infiltrates in HCC.
The gene expression values of the LIHC dataset were downloaded from TCGA database (https://www.cancer.gov/). All individuals with primary HCC were divided into high- or low-expression group based on the median value of GGT1, GGT5, GGT6, and GGT7. Thereafter, the cluster profiler R package (https://www.r-project.org) in R software (version 3.0) was employed to identify the most likely biological pathways in HCC.
Whole data analysis was implemented with SPSS software (version 21.0) and GraphPad Prism (version 7.0). GGT1, GGT5, GGT6, and GGT7 were transformed into categorical variables (low expression or high expression) according to the median value. Serum GGT protein and serum AFP were also transformed into categorical variables (normal concentration or high concentration) based on the reference value of Renmin Hospital of Wuhan University. The difference in serum GGT levels between two groups was determined by the Student’s t test. For analysis of survival, the log-rank test was used to compare the median survival time between two groups. Multivariate analysis combined with univariate surviving analysis was conducted to identify the independent prognostic factor in patients with HCC. A two-tailed P value less than 0.05 was regarded as statistically significant.
The mRNA expression data for GGT family genes (GGT1, GGT5, GGT6, and GGT7) from 371 primary HCC samples and 50 normal control samples were analyzed via UALCAN web tool. Except for GGT1 (Figure 1A), the other GGT family members (Figures 1B–D) were found to be differentially expressed in primary HCC samples (N = 371) and normal control tissues (N = 50). Furthermore, we browsed the cBioPortal webpage to determine the correlations between two GGT family genes (Figure S1). Interestingly, a positive relationship was observed not only between GGT1 and GGT5 (Spearman coefficient: 0.24, P = 5.143 × 10−6) but also between GGT5 and GGT6 (Spearman coefficient: 0.38, P = 1.24 × 10−13).
Figure 1 Expression profiles of GGT family genes in HCC specimens and normal tissues from TCGA-LIHC dataset. (A) GGT1; (B) GGT5; (C) GGT6; (D) GGT7.
We utilized GEPIA web tool to determine the prognostic values of GGT family genes in 364 patients with HCC with available follow-up data from TCGA LIHC dataset. A total of 248 patients were males, and 120 were females. The mean age of patients was 59.71 years. A total of 169 patients had TNM I stage, 86 patients had TNM II stage, 85 patients had TNM III stage, and 4 patients had TNM IV stage. Among them, 68 cases were assessed as grade A, 17 cases were assessed as grade B, and no patients were assessed as grade C. The mean follow-up time was 26.44 months. High expression of GGT1 mRNA was correlated with inferior OS (HR = 1.4, P = 0.049, Figure 2A) in patients with HCC; however, this correlation disappeared for DFS (HR = 1.2, P = 0.15, Figure 2B). For the GGT5 mRNA, no positive association was observed between high levels of GGT5 and worse OS (HR = 0.72, P = 0.066, Figure 2C). Fortunately, high expression of GGT5 was significantly associated with more favorable DFS in patients with HCC (HR = 0.73, P = 0.04, Figure 2D). GGT6 was found to have little prognostic value for OS (HR = 1, P = 1.00, Figure 2E) and DFS (HR = 0.83, P = 0.3, Figure 2F) in patients with HCC. In addition, high expression of GGT7 was identified as a promising predictor of both poor OS (HR = 1.7, P = 0.0048, Figure 2G) and less favorable DFS (HR = 1.4, P = 0.02, Figure 2H) in HCC.
Figure 2 Survival analysis of HCC patients stratified by median expression value of GGT family genes, which were downloaded from GEPIA database. Association between GGT1 mRNA expression and overall survival (A). Association between GGT1 mRNA expression and disease-free survival (B). Association between GGT5 mRNA expression and overall survival (C). Association between GGT5 mRNA expression and disease-free survival (D). Association between GGT6 mRNA expression and overall survival (E). Association between GGT6 mRNA expression and disease-free survival (F). Association between GGT7 mRNA expression and overall survival (G). Association between GGT7 mRNA expression and disease-free survival (H).
As serum GGT protein, encoded by GGT1, is routinely detected in the clinical setting as a common index reflecting liver injury, we assessed the clinical significance of serum GGT protein in 85 patients with early-stage HCC who received curative hepatectomy. Eight patients were females, and 77 patients were males. The mean age of patients was 52.27 years. A total of 26 cases were defined as TNM I stage, while 59 cases were identified as TNM II stage. The mean follow-up time was 43.13 months. As shown in Figure 3, high levels of serum GGT protein were observed in older (age, >55 years) patients with HCC (P = 0.0395), large tumor size (>5 cm, P = 0343), tumor recurrence (P = 0.0037), and Child Pugh grade (P < 0.001). Additionally, we evaluated the prognostic performance of serum GGT protein in patients with early-stage HCC by using Kaplan–Meier curves. As shown in Figure 4, patients with early-stage HCC with high levels of serum GGT protein had shorter OS time (HR = 2.921, P = 0.012, Figure 4A) and DFS time (HR = 1.859, P = 0.0485, Figure 4B) than those with low expression. Finally, Cox regression analysis was performed to determine the independent risk element of early-stage HCC. High expression of serum GGT was identified as a strong prognostic element for inferior OS (HR = 3.114, P = 0.025, (Table S1), but not for DFS (HR = 1.198, P = 0.05, Table S2) in patients with HCC subjected to curative hepatectomy.
Figure 3 Correlations of serum GGT protein with common clinical variables in 85 cases of early-stage HCC patients from Renmin cohort. (A) age (P = 0.0395); (B) gender (P = 0.0968); (C) G stage (P = 0.4273); (D) HBV infection (P = 0.673); (E) tumor size (P = 0.0343); (F) AFP levels (P = 0.8182); (G) TNM stage (P = 0.2548); (H) tumor recurrence (P = 0.037); (I) Child Pugh grade (P < 0.001).
Figure 4 Kaplan-Meier curves of survival in 85 cases of early-stage HCC patients from Renmin cohort stratified by median level of serum GGT protein. High expression of serum GGT protein predicted inferior OS (A), while not for DFS (B) in HCC patients with curative hepatectomy.
First, the UALCAN database was employed to determine whether differential DNA methylation status of GGT family genes existed between liver tumors and normal controls. DNA methylation of GGT1 (P = 0.0067, Figure 5A), GGT5 (P < 0.0001, Figure 5B), and GGT7 (P = 0.046, Figure 5C) was significantly higher in normal tissues than in the corresponding HCC specimens, with the exception of GGT6 (P = 0.09, Figure 5D). The cBioPortal database was also used to determine the potential association between the expression of GGT family genes and DNA methylation. Encouragingly, negative associations between DNA methylation and expression of mRNA were observed for GGT1 (Spearman coefficient: −0.38, P = 6.24e-14, Figure 6A), GGT6 (Spearman coefficient: −0.29, P = 1.23e-8, Figure 6B), and GGT7 (Spearman coefficient: −0.34, P=6.7e-11, Figure 6C); however, this negative relationship disappeared for GGT5 (Spearman coefficient: −0.09, P = 0.0788, Figure 6D). The detailed distribution of CpG sites in GGT family genes in HCC, downloaded from the UCSC Xena database, is listed in Figure S2. We also analyzed the copy number status (Figure S3) and DNA mutations (Figure S4) of GGT family genes in TCGA-LIHC dataset; however, no DNA mutations were found for GGT6 in TCGA-LIHC dataset.
Figure 5 DNA methylation patterns of GGT family genes in HCC tumors and normal controls. DNA methylation of GGT1 (A), GGT5 (B), and GGT7 (C) was significantly higher in normal tissues than the corresponding HCC specimens, with the exception of GGT6 (D).
Figure 6 Correlation analysis of DNA methylation with mRNA expression of GGT family members in HCC revealed by cBioPortal webpage. The negative associations between DNA methylation and expression of mRNA were observed in GGT1 (A), GGT6 (B), and GGT7 (C), while this negative relationship disappeared in GGT5 (D).
TIMER web tool was executed to gain insights into the associations between GGT family genes and infiltration levels of several immune cells in HCC. As shown in Figure 7A, expression of GGT1 was negatively correlated with tumor purity (r = −0.236, P = 9.03e-6) and positively associated with infiltration of CD4+ T cells (r = 0.148, P = 6.11e-3), macrophages (r = 0.163, P = 2.54e-3), and dendritic cells (r = 0.116, P = 3.25e-2). As for GGT5 (Figure 7B), expression of GGT5 was strongly correlated with tumor purity (r = −0.526, P = 5.39e-26) and positively associated with infiltration of CD8+ T cells (r = 0.193, P = 3.36e-4), CD4+ T cells (r = 0.352, P=1.85e-11), macrophages (r = 0.304, P = 9.57e-9), neutrophils (r = 0.213, P = 6.45e-5), and dendritic cells (r = 0.212, P = 7.935e-5). With regard to GGT6 (Figure 7C), its expression was positively related to CD4+ T cells (r = 0.26, P = 1.05e-6), macrophages (r = 0.328, P = 5.48e-10), neutrophils (r = 0.216, P = 5.17e-5), and dendritic cells (r = 0.193, P = 3.33e-4). Finally, expression of GGT7 was positively correlated with tumor purity (r = 0.317, P = 1.53e-9), CD8+ T cells (r = 0.254, P = 2.02e-6), CD4+ T cells (r = 0.216, P = 5.54e-5), macrophages (r = 0.323, P = 1.0e-9), neutrophils (r = 0.233, P=1.27e-5), and dendritic cells (r = 0.245, P = 5.0e-6) (Figure 7D). Collectively, we found that the four GGT family genes were well correlated with the infiltration levels of immune cells in HCC, especially with CD4+ T cells, macrophages, and dendritic cells.
Figure 7 Association between GGT1 (A), GGT5 (B), GGT6 (C), GGT7 (D), and immune cells (B cell, CD8+ T cell, CD4+ T cell, macrophage, neutrophil, and dendritic cell) in HCC tissues revealed by TIMER database.
TIMER web tool was executed to gain insights into the associations between GGT family genes and infiltration levels of several immune cells in HCC. As shown in Figure 8A, expression of GGT1 was negatively correlated with tumor purity (r = −0.236, P = 9.03e-6) and positively associated with infiltration of CD4+ T cells (r = 0.148, P = 6.11e-3), macrophages (r = 0.163, P = 2.54e-3), and dendritic cells (r = 0.116, P = 3.25e-2). As for GGT5 (Figure 8B), expression of GGT5 was strongly correlated with tumor purity (r = −0.526, P = 5.39e-26) and positively associated with infiltration of CD8+ T cells (r = 0.193, P = 3.36e-4), CD4+ T cells (r = 0.352, P = 1.85e-11), macrophages (r = 0.304, P = 9.57e-9), neutrophils (r = 0.213, P = 6.45e-5), and dendritic cells (r = 0.212, P = 7.935e-5). With regard to GGT6 (Figure 8C), its expression was positively related to CD4+ T cells (r = 0.26, P = 1.05e-6), macrophages (r = 0.328, P = 5.48e-10), neutrophils (r = 0.216, P = 5.17e-5), and dendritic cells (r = 0.193, P = 3.33e-4). Finally, expression of GGT7 was positively correlated with tumor purity (r = 0.317, P = 1.53e-9), CD8+ T cells (r = 0.254, P = 2.02e-6), CD4+ T cells (r = 0.216, P = 5.54e-5), macrophages (r = 0.323, P = 1.0e-9), neutrophils (r = 0.233, P = 1.27e-5), and dendritic cells (r = 0.245, P = 5.0e-6) (Figure 8D). Collectively, we found that the four GGT family genes were well correlated with the infiltration levels of immune cells in HCC, especially with CD4+ T cells, macrophages, and dendritic cells.
Figure 8 Enrichment analysis of biological pathways of GGT family genes in HCC via R software. (A) GGT1; (B) GGT5; (C) GGT6; (D) GGT7.
GGT family genes, including GGT1, GGT5, GGT6, and GGT7, encode enzymes involved in the metabolism of glutathione and the transpeptidation of amino acids. GGT family genes have been reported to be closely correlated to tumor formation and progression in several malignant neoplasms. In our analysis, we investigated the correlation between the expression of GGT family genes and the survival outcomes of patients with HCC. We demonstrated that the expression levels of GGT5 mRNA, GGT6 mRNA, and GGT7 mRNA were significantly different between normal liver specimens and HCC tissues. Moreover, based on survival analysis, high expression of GGT7 mRNA was a prognostic factor for less favorable OS and poor DFS in patients with HCC. For the first time, we gained insights into the relationship between GGT family genes and the tumor immune microenvironment and found that GGT family members were positively correlated with several immune cells, such as CD8+ T cells, macrophages, and dendritic cells. To our knowledge, this is also the first study to evaluate the DNA methylation of GGT family genes in HCC.
GGT1, a key gene encoding the GGT protein, plays a critical role in maintaining the homeostasis of GSH. Recently, GGT1 was implicated in the progression and metastasis of several cancers. According to Coradini et al. (23), the expression of GGT1 was significantly higher in breast tumors than in histologically normal tissues and correlated negatively with BCL2 and positively with TP53. GGT1 could promote the initiation and progression of clear-cell renal cell carcinoma. Further, its inhibition significantly decreased the migration of tumor cells, suggesting that GGT1 might be of therapeutic interest for patients with renal cancer (24). Another study further revealed that serum exosomal GGT1 could be a useful marker for advanced clinical features of patients with renal cancer (25). Kawakami et al. (26) found that the activity of serum exosomal GGT1 was significantly higher in individuals with prostate cancer than those with benign prostatic hyperplasia. In the present analysis, no differential expression of GGT1 mRNA was found between liver cancerous specimens and pathological normal tissues; however, low expression of GGT1 predicted relatively favorable OS in patients with HCC.
GGT5, formerly known as GGT-like activity 1 (GGTLA1), plays a critical role in redox regulation, immune function, and drug metabolism (27). Wei and coworkers (12) discovered that GGT5 was highly expressed in cancer-associated fibroblasts in lung cancer, predicting the poor survival of patients with lung cancer. GGT5 was also regarded as the key metabolism-related gene in gastric cancer (28) and colon cancer (29). In our study, we found an association between high expression of GGT5 mRNA and relatively favorable progression-free survival rather than OS. Studies related to GGT6 in the field of cancer are very few. Nonetheless, two recent studies highlighted the prognostic role of GGT6 mRNA in head and neck squamous cell carcinoma (30) and papillary renal cell carcinoma (31). However, these situations differ from those in HCC as revealed by our analysis. GGT7, formerly known as GGTL3, is a novel member of the GGT family. GGT7 was reported to interact with key proteins associated with the progression of lung cancer (32) and is minimally expressed in gliomas compared to that in normal brain tissues (33). Another study (34) related to GGT7 and glioblastoma revealed that GGT7 might play a key role in promoting glioblastoma growth by regulating reactive oxygen species (ROS) levels. To our knowledge, the present study is the first to explore the oncogenic role of GGT7 in HCC and demonstrated that the expression of GGT7 mRNA is higher in liver cancerous tissues than in normal liver specimens. Among the four GGT family genes, only the high expression of GGT7 mRNA was correlated with poor OS and inferior DFS, indicating that GGT7 might be a promising biomarker for predicting survival in patients with HCC.
GGT protein is a type of membrane-bound enzyme that plays an important role in regulating the production of intracellular glutathione, which is regarded as a classical antioxidant element against ROS (35). In addition, a prior study reported that ROS, a recognized carcinogenic factor, could contribute to the expression of GGT through the redox regulation of various key genes (36). Several preliminary studies have demonstrated that the immune microenvironment could lead to the progression and metastasis of cancer cells through the upregulation of oxidative stress (37–39). Our study also delved into the potential association between GGT family members and the immune microenvironment using the TIMER database. We found that four GGT family members were well correlated with the infiltration abundance of CD8+ T cells, macrophages, and dendritic cells. Biological pathway analysis by GSEA also revealed that GGT1 was enriched in the T-cell receptor signaling pathway. Abnormal expression of GGT family genes could induce the abnormal production of endogenous ROS, causing cells to be subjected to persistent oxidative stress, subsequently triggering aberrant methylation of the CpG island (6). Consistent with previous findings, the present study also revealed the aberrant CpG island methylation of GGTs between normal liver specimens and liver cancerous tissues. Moreover, a negative relationship was found between the mRNA levels of GGT family genes and DNA methylation. Hence, it is reasonable that elevated serum GGT protein reflects the persistent oxidative stress that is associated with the unfavorable prognosis of patients with HCC.
Sustained oxidative stress by high levels GGT protein would lead to increased risk of gastrointestinal cancer (40), including HCC. Change in activity of GGT is a very useful biomarker in the identification of patients with elevated risk of HCC (41). Moreover, serum GGT combined with other serum biomarkers were effective diagnostic markers of AFP-negative HCC, especially in individuals with early stage, small size or good liver function (42). More researchers continue to assess the prognostic role of serum GGT in cervical cancer (43), esophageal squamous cell carcinoma (44), ovarian cancer (45), renal cell carcinoma (25), and HCC (46). By carrying out a clinical analysis, Zhang et al. (15) found that a high concentration of serum GGT was positively associated with advanced TNM stage and large tumor size and was an independent element for predicting the OS rate of patients with primary HCC. Wang et al. (16) concluded that serum GGT was also an independent risk index for worse OS in individuals with AFP-negative HCC. Another clinical study (47) with 285 patients with HCC who underwent liver transplantation drew a similar conclusion that elevated serum GGT levels were correlated with inferior OS and larger tumor. The strong association between high levels of serum GGT and inferior OS was also observed in patients with HCC subjected to chemoembolization (48). A recent meta-analysis (49) with 9,238 patients with primary HCC demonstrated that preoperative serum GGT protein is a predictive index of unfavorable prognosis for patients with primary HCC. Consistent with the results of previous studies, our analysis based on a cohort of 85 patients with HCC indicated that increased GGT level seems to be associated with aggressive HCC features and is also a strong risk factor for unfavorable OS in patients with HCC. Unlike previous studies, we also determined the association between elevated GGT level and DFS. Unfortunately, this correlation was not observed after multivariate analysis, which might be owing to the limited sample size.
Three limitations of this study must be mentioned. First, the results of this study were mainly derived from systematic bioinformatic analyses. No experimental studies were performed to explore the biological functions of GGT family members. Therefore, basic experiments with regard to GGT family members in HCC cell lines are imminently needed to provide the basis for their clinical application. Besides the limited sample size (N = 85), the clinical study was retrospective and comprised of a single-center cohort. Because of this retrospective nature, we did not obtain serum GGT data at different time points. As a result, we could not assess the dynamic effect of serum GGT, which might be more valuable than that of preoperative serum GGT. Hence, prospective clinical trials with sufficient sample size are also needed to address these issues and promote the clinical utility of GGT protein.
To our knowledge, this is the first comprehensive analysis of the expression patterns and clinical value of GGT family genes in patients with HCC, providing insights for the experimental exploration of GGT family members as potential targets in HCC. Our study also lays the foundation for the clinical application of the GGT protein in prognostic assessment of patients with HCC.
The original contributions presented in the study are included in the article/Supplementary Material. Further inquiries can be directed to the corresponding authors.
The studies involving human participants were reviewed and approved by Ethics Committee of Renmin Hospital of Wuhan University. The patients/participants provided their written informed consent to participate in this study.
WD and XZ designed the research. ST performed the bioinformatic analysis. JL and YG collected the clinical data. ST wrote the manuscript. JL revised the manuscript. All authors contributed to the article and approved the submitted version.
This study was supported by the Independent Research Project of Wuhan University (No. 413000342).
The authors declare that the research was conducted in the absence of any commercial or financial relationships that could be construed as a potential conflict of interest.
All claims expressed in this article are solely those of the authors and do not necessarily represent those of their affiliated organizations, or those of the publisher, the editors and the reviewers. Any product that may be evaluated in this article, or claim that may be made by its manufacturer, is not guaranteed or endorsed by the publisher.
The Supplementary Material for this article can be found online at: https://www.frontiersin.org/articles/10.3389/fonc.2021.731144/full#supplementary-material
1. Akinyemiju T, Abera S, Ahmed M, Alam N, Alemayohu MA, Allen C, et al. The Burden of Primary Liver Cancer and Underlying Etiologies From 1990 to 2015 at the Global, Regional, and National Level: Results From the Global Burden of Disease Study 2015. JAMA Oncol (2017) 3(12):1683–91. doi: 10.1001/jamaoncol.2017.3055
2. Anwanwan D, Singh SK, Singh S, Saikam V, Singh R. Challenges in Liver Cancer and Possible Treatment Approaches. Biochim Biophys Acta Rev Cancer (2020) 1873(1):188314. doi: 10.1016/j.bbcan.2019.188314
3. Llovet JM, Ducreux JB, Lencioni R, DI Bisceglie AM, Galle PR, Dufour JF, et al. EASL-EORTC Clinical Practice Guidelines: Management of Hepatocellular Carcinoma. J Hepatol (2012) 56(4):908–43. doi: 10.1016/j.jhep.2011.12.001
4. Bruix J, Sherman M. Management of Hepatocellular Carcinoma: An Update. Hepatology (2011) 53(3):1020–2. doi: 10.1002/hep.24199
5. Whitfield JB. Gamma Glutamyl Transferase. Crit Rev Clin Lab Sci (2001) 38(4):263–355. doi: 10.1080/20014091084227
6. Kunutsor SK. Gamma-Glutamyltransferase-Friend or Foe Within? Liver Int (2016) 36(12):1723–34. doi: 10.1111/liv.13221
7. Lee MY, Hyon DS, Huh JH, Kim HK, Han SK, Kim JY, et al. Association Between Serum Gamma-Glutamyltransferase and Prevalence of Metabolic Syndrome Using Data From the Korean Genome and Epidemiology Study. Endocrinol Metab (Seoul) (2019) 34(4):390–7. doi: 10.3803/EnM.2019.34.4.390
8. Kunutsor SK, Laukkanen JA. Gamma-Glutamyltransferase and Risk of Chronic Kidney Disease: A Prospective Cohort Study. Clin Chim Acta (2017) 473:39–44. doi: 10.1016/j.cca.2017.08.014
9. Shibata M, Sato KK, Uehara S, Koh H, Oue K, Kambe H, et al. Serum Gamma-Glutamyltransferase, Daily Alcohol Consumption, and the Risk of Chronic Kidney Disease: The Kansai Healthcare Study. J Epidemiol (2020) 30(4):163–9. doi: 10.2188/jea.JE20180240
10. Han E, Lee JY, Han KD, Cho H, Kim KJ, Lee BW, et al. Gamma Glutamyltransferase and Risk of Dementia in Prediabetes and Diabetes. Sci Rep (2020) 10(1):6800. doi: 10.1038/s41598-020-63803-0
11. Lee YB, Han K, Park S, Kim SM, Kim NH, Choi KM, et al. Gamma-Glutamyl Transferase Variability and Risk of Dementia: A Nationwide Study. Int J Geriatr Psychiatry (2020) 35(10):1105–14. doi: 10.1002/gps.5332
12. Wei JR, Dong J, Li L. Cancer-Associated Fibroblasts-Derived Gamma-Glutamyltransferase 5 Promotes Tumor Growth and Drug Resistance in Lung Adenocarcinoma. Aging (Albany NY) (2020) 12(13):13220–33. doi: 10.18632/aging.103429
13. Albhaisi S, Qayyum R. The Association Between Serum Liver Enzymes and Cancer Mortality. Clin Exp Med (2021). doi: 10.1007/s10238-021-00733-9
14. Gong G, Zheng K, Xue S, Hou J, Zhang Q. Serum AFU, GGT and TK1 Levels in PHC Patients and Their Correlation With Clinicopathology and Diagnostic Value. Cell Mol Biol (Noisy-le-grand) (2020) 66(5):111–6. doi: 10.14715/cmb/2020.66.5.20
15. Zhang LX, Lv Y, Xu AM, Wang HZ. The Prognostic Significance of Serum Gamma-Glutamyltransferase Levels and AST/ALT in Primary Hepatic Carcinoma. BMC Cancer (2019) 19(1):841. doi: 10.1186/s12885-019-6011-8
16. Wang X, Mao M, He Z, Zhang L, Li H, Lin J, et al. Development and Validation of a Prognostic Nomogram in AFP-Negative Hepatocellular Carcinoma. Int J Biol Sci (2019) 15(1):221–8. doi: 10.7150/ijbs.28720
17. Ozcelik F. Prognostic Value of Gamma-Glutamyl Transpeptidase in Liver Cirrhosis and Hepatocellular Cancer Regardless of Other Parameters. Clin Res Hepatol Gastroenterol (2021) 45(5):101708. doi: 10.1016/j.clinre.2021.101708
18. Chandrashekar DS, Bashel B, Balasubramanya S, Creighton CJ, Ponce-Rodriguez I, Chakravarthi B, et al. UALCAN: A Portal for Facilitating Tumor Subgroup Gene Expression and Survival Analyses. Neoplasia (2017) 19(8):649–58. doi: 10.1016/j.neo.2017.05.002
19. Tang Z, Li C, Kang B, Gao G, Li C, Zhang Z. GEPIA: A Web Server for Cancer and Normal Gene Expression Profiling and Interactive Analyses. Nucleic Acids Res (2017) 45(W1):W98–102. doi: 10.1093/nar/gkx247
20. Jiao XD, Qin BD, You P, Cai J, Zang YS. The Prognostic Value of TP53 and its Correlation With EGFR Mutation in Advanced non-Small Cell Lung Cancer, an Analysis Based on Cbioportal Data Base. Lung Cancer (2018) 123:70–5. doi: 10.1016/j.lungcan.2018.07.003
21. Goldman MJ, Craft B, Hastie M, Repecka K, McDade F, Kamath A, et al. Visualizing and Interpreting Cancer Genomics Data via the Xena Platform. Nat Biotechnol (2020) 38(6):675–8. doi: 10.1038/s41587-020-0546-8
22. Li B, Severson E, Pignon JC, Zhao H, Li T, Novak J, et al. Comprehensive Analyses of Tumor Immunity: Implications for Cancer Immunotherapy. Genome Biol (2016) 17(1):174. doi: 10.1186/s13059-016-1028-7
23. Coradini D, Gambazza S, Oriana S, Ambrogi F. Body Mass Index and Gamma-Glutamyl Transferase Expression in Normal and Cancerous Breast Tissue. Breast Cancer-Tokyo (2020) 27(5):850–60. doi: 10.1007/s12282-020-01080-5
24. Bansal A, Sanchez DJ, Nimgaonkar V, Sanchez D, Riscal R, Skuli N, et al. Gamma-Glutamyltransferase 1 Promotes Clear Cell Renal Cell Carcinoma Initiation and Progression. Mol Cancer Res (2019) 17(9):1881–92. doi: 10.1158/1541-7786.MCR-18-1204
25. Horie K, Kawakami K, Fujita Y, Matsuda Y, Arai T, Suzui N, et al. Serum Exosomal Gamma-Glutamyltransferase Activity Increased in Patients With Renal Cell Carcinoma With Advanced Clinicopathological Features. Oncology (2020) 98(10):734–42. doi: 10.1159/000508688
26. Kawakami K, Fujita Y, Matsuda Y, Arai T, Horie K, Kameyama K, et al. Gamma-Glutamyltransferase Activity in Exosomes as a Potential Marker for Prostate Cancer. BMC Cancer (2017) 17(1):316. doi: 10.1186/s12885-017-3301-x
27. Wickham S, West MB, Cook PF, Hanigan MH. Gamma-Glutamyl Compounds: Substrate Specificity of Gamma-Glutamyl Transpeptidase Enzymes. Anal Biochem (2011) 414(2):208–14. doi: 10.1016/j.ab.2011.03.026
28. Wen F, Huang J, Lu X, Huang W, Wang Y, Bai Y, et al. Identification and Prognostic Value of Metabolism-Related Genes in Gastric Cancer. Aging (Albany NY) (2020) 12(17):17647–61. doi: 10.18632/aging.103838
29. Ren J, Feng J, Song W, Wang C, Ge Y, Fu T. Development and Validation of a Metabolic Gene Signature for Predicting Overall Survival in Patients With Colon Cancer. Clin Exp Med (2020) 20(4):535–44. doi: 10.1007/s10238-020-00652-1
30. Reddy RB, Khora SS, Suresh A. Molecular Prognosticators in Clinically and Pathologically Distinct Cohorts of Head and Neck Squamous Cell Carcinoma-A Meta-Analysis Approach. PloS One (2019) 14(7):e218989. doi: 10.1371/journal.pone.0218989
31. Liu Z, Wan Y, Yang M, Qi X, Dong Z, Huang J, et al. Identification of Methylation-Driven Genes Related to the Prognosis of Papillary Renal Cell Carcinoma: A Study Based on The Cancer Genome Atlas. Cancer Cell Int (2020) 20:235. doi: 10.1186/s12935-020-01331-7
32. He X, Di Y, Li J, Xie Y, Tang Y, Zhang F, et al. Molecular Cloning and Characterization of CT120, a Novel Membrane-Associated Gene Involved in Amino Acid Transport and Glutathione Metabolism. Biochem Biophys Res Commun (2002) 297(3):528–36. doi: 10.1016/s0006-291x(02)02227-1
33. Pang X, Panee J. Roles of Glutathione in Antioxidant Defense, Inflammation, and Neuron Differentiation in the Thalamus of HIV-1 Transgenic Rats. J Neuroimmune Pharmacol (2014) 9(3):413–23. doi: 10.1007/s11481-014-9538-0
34. Bui TT, Nitta RT, Kahn SA, Razavi SM, Agarwal M, Aujla P, et al. Gamma-Glutamyl Transferase 7 is a Novel Regulator of Glioblastoma Growth. BMC Cancer (2015) 15:225. doi: 10.1186/s12885-015-1232-y
35. Luo C, Xu B, Fan Y, Yu W, Zhang Q, Jin J. Preoperative Gamma-Glutamyltransferase Is Associated With Cancer-Specific Survival and Recurrence-Free Survival of Nonmetastatic Renal Cell Carcinoma With Venous Tumor Thrombus. BioMed Res Int (2017) 2017:3142926. doi: 10.1155/2017/3142926
36. Corti A, Franzini M, Paolicchi A, Pompella A. Gamma-Glutamyltransferase of Cancer Cells at the Crossroads of Tumor Progression, Drug Resistance and Drug Targeting. Anticancer Res (2010) 30(4):1169–81. doi: 10.1097/CAD.0b013e32833d19f0
37. Kou L, Sun R, Jiang X, Lin X, Huang H, Bao S, et al. Tumor Microenvironment-Responsive, Multistaged Liposome Induces Apoptosis and Ferroptosis by Amplifying Oxidative Stress for Enhanced Cancer Therapy. ACS Appl Mater Interfaces (2020) 12(27):30031–43. doi: 10.1021/acsami.0c03564
38. Maj T, Wang W, Crespo J, Zhang H, Wang W, Wei S, et al. Oxidative Stress Controls Regulatory T Cell Apoptosis and Suppressor Activity and PD-L1-Blockade Resistance in Tumor. Nat Immunol (2017) 18(12):1332–41. doi: 10.1038/ni.3868
39. Scharping NE, Menk AV, Moreci RS, Whetstone RD, Dadey RE, Watkins SC, et al. The Tumor Microenvironment Represses T Cell Mitochondrial Biogenesis to Drive Intratumoral T Cell Metabolic Insufficiency and Dysfunction. Immunity (2016) 45(2):374–88. doi: 10.1016/j.immuni.2016.07.009
40. Hong SW, Lee HJ, Han K, Moon JM, Park S, Soh H, et al. Risk of Gastrointestinal Cancer in Patients With an Elevated Level of Gamma-Glutamyltransferase: A Nationwide Population-Based Study. PloS One (2021) 16(2):e245052. doi: 10.1371/journal.pone.0245052
41. Orzechowska D, Klimowicz K, Stepien A, Mikula T, Sapula M, Wiercinska-Drapalo A. Change in Gamma-Glutamyl Transpeptidase Activity as a Useful Tool in Identifying A Group of Patients With Elevated Risk of Hepatocellular Carcinoma Development After DAA Treatment of Chronic Hepatitis C. Clin Exp Hepatol (2021) 7(1):93–100. doi: 10.5114/ceh.2021.104466
42. Li J, Tao H, Zhang E, Huang Z. Diagnostic Value of Gamma-Glutamyl Transpeptidase to Alkaline Phosphatase Ratio Combined With Gamma-Glutamyl Transpeptidase to Aspartate Aminotransferase Ratio and Alanine Aminotransferase to Aspartate Aminotransferase Ratio in Alpha-Fetoprotein-Negative Hepatocellular Carcinoma. Cancer Med (2021) 10(14):4844–54. doi: 10.1002/cam4.4057
43. Zhu Y, Zhang AJ, Wu DB, Shen Z, Chen G, Shi YY, et al. Prognostic Significance of the Pretreatment Serum Gamma-Glutamyltransferase Levels in Chinese Patients With non-Metastatic Cervical Cancer. Oncotarget (2017) 8(70):115701–8. doi: 10.18632/oncotarget.22273
44. Huang H, Wang XP, Li XH, Chen H, Zheng X, Lin JH, et al. Prognostic Value of Pretreatment Serum Alanine Aminotransferase/Aspartate Aminotransferase (ALT/AST) Ratio and Gamma Glutamyltransferase (GGT) in Patients With Esophageal Squamous Cell Carcinoma. BMC Cancer (2017) 17(1):544. doi: 10.1186/s12885-017-3523-y
45. Grimm C, Hofstetter G, Aust S, Mutz-Dehbalaie I, Bruch M, Heinze G, et al. Association of Gamma-Glutamyltransferase With Severity of Disease at Diagnosis and Prognosis of Ovarian Cancer. Br J Cancer (2013) 109(3):610–4. doi: 10.1038/bjc.2013.323
46. Yang JG, He XF, Huang B, Zhang HA, He YK. Rule of Changes in Serum GGT Levels and GGT/ALT and AST/ALT Ratios in Primary Hepatic Carcinoma Patients With Different AFP Levels. Cancer Biomark (2018) 21(4):743–6. doi: 10.3233/CBM-170088
47. Ince V, Carr BI, Bag HG, Koc C, Usta S, Ersan V, et al. Gamma Glutamyl Transpeptidase as a Prognostic Biomarker in Hepatocellular Cancer Patients Especially With >5 Cm Tumors, Treated by Liver Transplantation. Int J Biol Markers (2020) 35(2):91–5. doi: 10.1177/1724600820921869
48. Guo J, Liu S, Gao S, Kou F, Zhang X, Liu P, et al. Gamma-Glutamyltranspeptidase as a Prognostic Biomarker in Advanced Hepatocellular Carcinoma Treated With Transarterial Chemoembolization. J Vasc Interv Radiol (2021) 32(3):419–28. doi: 10.1016/j.jvir.2020.07.020
Keywords: gamma-glutamyl transpeptidase, family genes, hepatocellular carcinoma, survival analysis, biomarker
Citation: Tian S, Li J, Guo Y, Dong W and Zheng X (2021) Expression Status and Prognostic Significance of Gamma-Glutamyl Transpeptidase Family Genes in Hepatocellular Carcinoma. Front. Oncol. 11:731144. doi: 10.3389/fonc.2021.731144
Received: 26 June 2021; Accepted: 30 July 2021;
Published: 26 August 2021.
Edited by:
Xin Chen, University of California, San Francisco, United StatesReviewed by:
Yi Liu, University of Texas MD Anderson Cancer Center, United StatesCopyright © 2021 Tian, Li, Guo, Dong and Zheng. This is an open-access article distributed under the terms of the Creative Commons Attribution License (CC BY). The use, distribution or reproduction in other forums is permitted, provided the original author(s) and the copyright owner(s) are credited and that the original publication in this journal is cited, in accordance with accepted academic practice. No use, distribution or reproduction is permitted which does not comply with these terms.
*Correspondence: Weiguo Dong, ZHdnQHdodS5lZHUuY24=; Xin Zheng, eGluMTFAaG90bWFpbC5jb20=
†These authors have contributed equally to this work
Disclaimer: All claims expressed in this article are solely those of the authors and do not necessarily represent those of their affiliated organizations, or those of the publisher, the editors and the reviewers. Any product that may be evaluated in this article or claim that may be made by its manufacturer is not guaranteed or endorsed by the publisher.
Research integrity at Frontiers
Learn more about the work of our research integrity team to safeguard the quality of each article we publish.