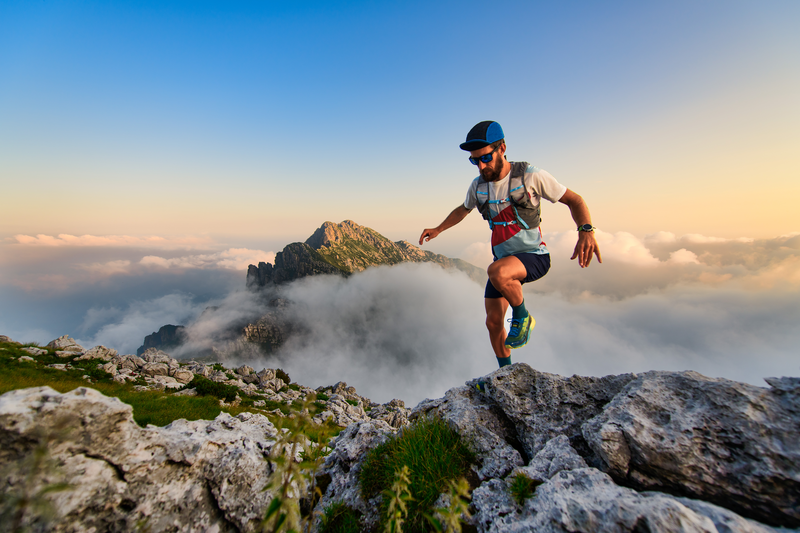
94% of researchers rate our articles as excellent or good
Learn more about the work of our research integrity team to safeguard the quality of each article we publish.
Find out more
REVIEW article
Front. Oncol. , 09 November 2021
Sec. Radiation Oncology
Volume 11 - 2021 | https://doi.org/10.3389/fonc.2021.728452
This article is part of the Research Topic Principles and Clinical Applications of Interstitial Brachytherapy View all 9 articles
The three-dimensional iridium-192 (192Ir) high-dose-rate (HDR) brachytherapy manifests itself as a high-precision, hypofractionated, dose-escalating, minimally invasive method in the armamentarium of contemporary radiation oncology clinical applications. In this study, the physical aspects of the 192Ir radionuclide are presented. Its dosimetric application in HDR brachytherapy for different anatomical sites (prostate, gynecological malignancies, liver, and intrathoracic tumors) as well as the corresponding dosimetric comparison with the stereotactic body radiation therapy (SBRT) techniques based on a representative selection of dosimetric publications is reviewed and illustrated.
Iridium-192 (192Ir) has been extensively used for high-dose-rate (HDR) brachytherapy (BT) for more than four decades in the remote afterloading BT systems. Its physical properties are presented in Table 1 (1–5). Its medium energy range of X- and gamma-rays (mean energy: 370 keV) in its energy spectrum and its beneficial reference air kerma rate (RAKR) of 0.1091 μGym2h-1MBq-1 in comparison with other HDR source radionuclides such as 60Co (energy: 1.173 and 1.332 MeV, RAKR: 0.308 μGym2h-1MBq-1) have always required definitively less shielding than any external beam radiation modality. The tenth value layer (TVL) for 192Ir is 16 and 152 mm for lead and concrete, respectively, while for 60Co, the TVL is 41 and 218 mm for lead and concrete, respectively (4). This enables the construction of 192Ir HDR BT treatment rooms in a more cost-effective way and storage of the source inside the remote afterloader an easier task. The half-life of 192Ir is 73.81 days, enabling a relatively economic application of this radionuclide in remote afterloading HDR systems for radiation therapy, by implementing four source exchanges per year, in order to maintain the HDR of greater or equal to 12 Gy/h. Its main advantage is especially the high specific activity (340 GBqmg-1), which makes feasible the manufacturing and the distribution of high-activity 192Ir miniaturized size sources (370–480 GBq). The typical length of an encapsulated 192Ir HDR BT source is in the order of 5–11 mm, and the typical outer diameter lies in the order of 0.8–1 mm. For distances from the source center, r, greater than the double of the 192Ir active core length (r ≥ 2L ≈ 8 mm), the source can be considered a point source in its dosimetry (2, 3), and the dose rate distribution in the periphery of the source—hence the dose rate in the planning target volume (PTV) and in the surrounding healthy tissue—is determined by the inverse square law (r-2). The immediate contact of the implanted catheter/applicator inside the PTV, where the miniaturized 192Ir source is remotely driven, along with the predominance of the inverse square law of a single source in its dwell position, facilitates the characteristic steep dose fall-off, which in comparison with a single megavoltage external X-ray or even a proton beam (with the characteristic Bragg peak) is unbeatable.
Table 1 Physical characteristics of 192Ir radionuclide and 192Ir sources used in brachytherapy (1–4).
The characteristic dosimetric property of the 192Ir BT source with its sharp dose gradient justifies reasonably the name “Iridium-Knife” in correspondence to the high-precision, steep-dose gradient Linear Accelerator (LINAC)-based multi-leaf collimator and the robotic radiosurgery device-based external-beam radiotherapy, which are also known as X-knife (6) and Cyberknife (7), respectively.
The purpose of this mini-review is to illustrate the capability of the three-dimensional (3D) HDR BT with 192Ir for the delivery of conformal dose coverage of the PTV by concomitant dose escalation inside them and the steep dose fall-off in their periphery, acting thus as another precision dose knife, due to its physical properties. Furthermore, by reviewing representative published data for four different anatomical sites, a dosimetric comparison with the stereotactic body radiation therapy (SBRT) method is presented and the advantages as well as disadvantages of the 192Ir HDR BT are highlighted.
In this article, we review dosimetric studies for prostate, liver, endometrium, and intrathoracic malignancies either clinically performed with HDR BT and afterward virtually planned with SBRT or vice versa (8–15). The dosimetric comparison for PTV coverage, organs at risk (OARs) dose sparing, plan conformity, dose heterogeneity, dose fall-off sharpness, and low dose spill are presented with the aid of dose-volume and physical quantities presented in Table 2. The clinical evaluation and the medical follow-up of the patients subjected to these radiotherapy modalities are beyond the scope of this article.
Table 2 Dose-volume and physical quantities used for the dosimetric assessment of the radiation therapy modalities.
For high- and intermediate-risk prostate cancer patients, a small number of planning studies have been carried out for the comparison of the established prostate HDR BT with the SBRT modality (8–10). It should be mentioned that the SBRT in the prostate was initially termed “virtual HDR” because of the similar characteristics to HDR BT, namely, hypofractionation and high dose per fraction administration.
In the first study (8), the patients were treated with 192Ir HDR BT with transrectal ultrasound (TRUS)-guided plastic needle insertion with a dose of 4 × 9.5 Gy as monotherapy. Following the recommendations of the American Brachytherapy Society, the dose parameters applied in the optimization phase were for the urethra Dmax less than 120% of the prescribed dose (PD), for rectum Dmax less than 100% of the PD and D2ccm less than 70% of the PD, for the bladder D2ccm less than 75% of the PD, and for the PTV coverage V100% greater than 90%. Spratt et al. used two approaches to dosimetrically compare the HDR dosimetry with the virtual SBRT plans. The first was the “normal tissue-prioritized” plan, where the primary goal was to maintain the OAR constraints by simultaneously trying to maximize target coverage, and the second approach was the “PTV-prioritized” plan, where the coverage of the PTV was the primary goal by allowing the constraints for the OARS to be violated in an as minimal as possible extent.
The dosimetric comparison results of the “normal tissue-prioritized” plans are presented in Table 3 [Table 1 (8)] and reveal that all dose metrics for the rectum and the bladder were lower for the HDR BT, but only the rectal Dmax reached statistical significance, representative of the sharper dose fall-off outside of the PTV for the Iridium-Knife. As expected, dose metrics for the urethra were always higher for the HDR BT in comparison with SBRT due to the predominant inhomogeneity of the dose distributions around the source dwell positions inside the PTV and in the proximity of the urethra, a characteristic that is evident with the dose escalation feature and the resulting value of V200% for the HDR BT inside the PTV, a value that cannot be reached by SBRT.
Table 3 The results of the dosimetric comparison of the normal tissue-prioritized plans from the study of Spratt et al. (8) [courtesy of Spratt et al. (8), reproduced with permission].
For the case of “PTV-prioritized” plans, where an attempt was made for SBRT to match the PTV V200% of the HDR BT by relaxing the OAR constraints, the PTV V150% was significantly higher (67%) for SBRT than for the HDR BT plan (40%) (p = 0.045). Furthermore, rectum Dmax and Dmean for the SBRT were 111% and 33%, respectively, while in HDR BT, they were significantly lower 94% (p = 0.045) and 27% (p = 0.028), respectively, thus violating the dosimetric constraints for rectum. Additionally, the bladder and urethral doses were higher for SBRT in this approach compared to HDR BT, without showing statistical significance. Another important finding of this study is that, for this plan approach, the surrounding healthy tissue total body dose was significantly greater for SBRT than for HDR BT (V10% = 2,206 cm3 for SBRT vs. V10% = 1,250 cm3 for HDR BT, p = 0.01), implying that the dose escalation with SBRT inside the prostate PTV to approach HDR BT comes with a cost of increased dose spillage in the surrounding healthy tissue.
In the second study (9), six consecutive prostate patients have been treated HDR BT with a dose prescription of 45.5 Gy in seven fractions. Metallic needles were placed with real-time TRUS guidance, and the plan was created on a CT image acquired after needle insertion. The clinical target volume (CTV) comprised the prostate gland plus 5 mm in all directions to cover possible extracapsular extensions, except for the posterior rectal margin, which varied from 2 to 5 mm depending on the distance from the rectal wall. The planning goal in that study was to achieve a V100% of partially lower than 100% (because V100% = 100% is impossible due to the interference of the metallic needle positions with the urethra shape in the anterior ventral part of the prostate that cannot be intersected, thus leaving a cold spot in that area), with urethra Dmax lower than 150% of the PD and rectum Dmax lower than 100% of the PD.
The virtual SBRT (CyberKnife) planning was performed with the CT images and structures from the HDR BT plan, except for the PTV that was adjusted with an additional margin of 2 mm. The SBRT dosimetric constraints were for the PTV: dose within 100% and 150% of the PD, Dmax for rectum, urethra, and bladder to be less or equal to 100% of the PD. For each SBRT plan, the most common prescription method of D95% was used as the prescription dose to perform the dosimetric comparison of SBRT with HDR BT.
The results for dose volume parameters of the PTV [Table 1 (9)] revealed that, in terms of dose escalation inside the PTV, the HDR BT is significantly better than the SBRT modality. However, the D99%, the D100%, and the V100% SBRT values are higher than the corresponding ones of HDR BT due to the inherent limitation of the HDR BT to dosimetrically fully cover with the PD the anterior part of the PTV, where the urethra is located, because the urethra should not be penetrated with needles. Considering the dose received by lower volume fractions of the PTV, such as the D90%, HDR BT performed significantly better than SBRT. Moreover, the V125%, the V150%, and the V200% values of HDR BT are significantly higher than the ones of SBRT, and this dose escalation could lead to a better outcome (16).
The dosimetric comparison for the rectum revealed that HDR BT had a higher Dmax and a steeper dose fall-off inside the rectum than SBRT in the intermediate to high dose range. The average D1ccm, D2ccm, D5ccm, D10ccm, and D20ccm were significantly lower for HDR BT by 5.1, 7.1, 7.6, 6.1, and 2.4 Gy, respectively. Moreover, the average V40%–100% values were also significantly lower in HDR BT, even though they were relatively small (1.6–4.5 cm3). For the urethra, the HDR BT was inferior to SBRT with statistical significance. The average D0.1ccm, D0.2ccm, D0.5ccm, D10%, and D20% were considerably higher than for SBRT by 12.6, 8.9, 4.5, 10.2, and 7.0 Gy, respectively. For the bladder, HDR BT resulted in higher doses than the SBRT modality, with no statistical significance however, except for the D0.5ccm, which was equal to 96.2 Gy for HDR BT and 51.1 Gy for SBRT (p = 0.02). This might be attributed to the fact that, for the HDR BT, source dwell positions of catheters inside the bladder pouch were activated in order to be able to cover the anterior cranial part of the prostate PTV with the 100% of the PD, resulting in very high doses in the bladder.
In the third study (10), 15 patients were treated with SBRT (CyberKnife) with a dose prescription of 35 Gy in five fractions. TRUS-based fiducial marker implantation was performed at least 5 days before treatment planning CT acquisition, and a TRUS dataset was acquired immediately before implantation. For the SBRT plans, GTV was defined as the prostate on MRI in low risk and prostate with base of seminal vesicles (1 cm proximal) for all other patients. CTV was generated by uniform expansion of the CTV by 2 mm, while PTV was generated by anisotropic expansion of the CTV (1 mm posterior and 3 mm in all other directions). The planning goal was to achieve V100% of more than 95% for the PTV and V37.5Gy of more than 95% for the GTV. The dosimetric constraints for rectum Dmax were lower than 38 Gy, and V36Gy, V29Gy, and V18Gy encompassing less than 1, 15, and 25 cm3, respectively. For bladder, the Dmax was lower than 38 Gy, with V36Gy less than 10 cm3 and V18Gy covering less than 40% of its volume. For urethra, Dmax was lower than 44 Gy and V44Gy less than 20% of its volume.
The virtual HDR BT plans were created on the aforementioned TRUS images with a dose prescription of 35 Gy in a single fraction in order to allow direct dosimetric comparison to the clinical SBRT plan. The PTV encompassed the entire prostate gland without margins. The planning goal was to achieve D90% of more than 100% and D150% less than 35% for the PTV. The dosimetric constraints for rectum D0.1ccm were less than 80% of PD for rectum and bladder and less than 120% of the PD for urethra.
The dosimetric comparison for the prostate (i.e., SBRT GTV or HDR BT PTV) showed that the dose delivered to 98% of the volume, the V35Gy and V37.5Gy were significantly higher for SBRT compared to HDR BT plans, while V42Gy and V52.5Gy were significantly higher for HDR BT compared to SBRT plans. The maximum dose to the rectum and bladder was significantly lower, while the maximum dose to the urethra was significantly higher in HDR BT plans compared to SBRT plans.
A small number of studies thus far attempted to dosimetrically compare brachytherapy plans against SBRT plans in the treatment of primary or secondary malignancies of the liver (11, 12).
Pennington et al. (11) investigated the differences of brachytherapy and SBRT plans in a retrospective study of 10 patients with liver metastasis, originally treated with SBRT, and for which virtual 192Ir HDR BT plans were created. Both plans were designed to deliver five fractions of 12 Gy to the same PTV, while no precise information is given for the generation of this volume. The stated HDR BT planning goal was to match the PTV receiving 100% of the PD to the SBRT plan. In terms of target coverage, they found that the mean PTV V100% was comparable between HDR BT and SBRT (94.1% vs. 93.9%, p = 0.8), while mean PTV V150% was significantly higher in HDR BT plan (63.6% vs. 0%), revealing significant dose escalation. On the other hand, significantly lower minimum dose as a percentage of the prescription dose within the PTV was exposed for HDR BT compared to SBRT plans (66% vs. 88%, p = 0.0002). They found no statistically significant differences (p = 0.109) in dose fall-off as estimated by R50%. The authors concluded that HDR BT can achieve higher target dose, similar dose to OARs but potentially lower target coverage in comparison with SBRT (11). A limitation to this study is the retrospective virtual HDR BT planning of the data, coupled with the fact that the same PTV was irradiated as for the SBRT plans. This is typically not the case in clinical practice where the CTV is considered to be the PTV in HDR BT treatments, since due to the fact that needles are fairly stable within the tumor, the uncertainties addressed by the PTV expansion are deemed negligible. Therefore, even though it is a fact that in HDR BT clinical practice, it is often difficult for the prescription dose to cover the entirety of the PTV, a fairer comparison of the two modalities would compare the coverage of the prescription dose for the respective PTV employed for each modality.
In a recent study, Hass et al. (12) performed a similar comparison of HDR BT vs. SBRT plans for liver lesions while addressing the aforementioned limitations of previous studies. In their article, 85 patients previously treated for liver malignancies with HDR BT were used, and for each, a retrospective virtual SBRT plan was generated. The GTV was generated based on contrast-enhanced breath-hold CT scanning with a 3–5-mm margin for the generation of the CTV, depending on the visualization quality of the GTV. No additional margin was added for HDR BT planning, and therefore, CTV = PTV for this case. In contrast, a margin of 5 mm in lateral direction and 10 mm along the craniocaudal axis was added to the GTV for the generation of the SBRT-specific PTVs. The prescription dose for both treatments was the same for both plans (15 or 20 Gy in one fraction, depending on type of tumor) and was prescribed for both treatment modalities to be 99.9% of the PTV. The same OAR constraints were employed. The dosimetric results of this investigation are summarized in Table 4. They revealed significantly better coverage PTV D99.9% by the prescribed HDR BT plans compared to SBRT plans in both the 15 Gy (p < 0.001) and 20 Gy (p = 0.003) groups. Similarly, mean PTV D90% was significantly higher in the HDR BT plans in both the 15-Gy (p < 0.001) and 20 Gy (p < 0.001) groups. Regarding the exposure of the remaining liver volume, the study found no statistically significant differences in V5Gy in the 15 Gy group (p = 0.095), but statistical significance was found in the 20 Gy group (p = 0.001) in favor of the HDR BT plans. The authors concluded that HDR BT revealed superior outcomes both in terms of target coverage (PTV D99.9% and D90%) and exposure to the remaining healthy liver. They also discussed further advantages to each of the examined modalities. Namely, HDR BT allows for irradiation of larger liver tumors (SBRT is typically limited to sizes with diameter less than 4–6 cm) and more centrally located tumors (for which SBRT is typically avoided) while also being less affected by uncertainties related to respiratory breathing motion. On the other hand, HDR BT is a minimally invasive procedure while SBRT is non-invasive. The authors point out some limitations in their study. The main one is the fact that their planning procedure for SBRT was aiming for a relatively homogeneous dose distribution inside the target, avoiding central dose escalation. This would typically lead to a shallower dose gradient than would otherwise be achieved if the authors allowed the SBRT treatment planning optimizer the flexibility to have a higher dose in the central region of the PTV. Therefore, steeper dose fall-off would be expected with current SBRT planning strategies that allow up to 125%–140% of the prescription dose within the target.
Table 4 The dosimetric results (mean ± standard deviation) of the different brachytherapy (BT) and stereotactic body radiotherapy (SBRT) from the study of Hass et al. (12) [courtesy of Hass et al. (12), reproduced with permission].
A number of investigators performed retrospective studies to dosimetrically compare HDR BT and SBRT sequential boost in the treatment of gynecologic malignancies, and two representative investigations are discussed herein (13, 14).
Georg et al. (13) attempted a comparison of HDR BT against SBRT in cervical cancer using high-tech techniques for both methods. Nine patients with locally advanced cervical cancer that were previously treated with HDR BT boost under MRI guidance were employed. Additional SBRT plans were created with step-and-shoot intensity-modulated radiotherapy (IMRT) and intensity-modulated proton therapy (IMPT) for each patient. Both SBRT treatment plans were created under the assumptions that (a) MRI-guided beam delivery was available, (b) dedicated applicator for cervix immobilization would be used, (c) online adaptive planning would be employed, and (d) sufficient patient immobilization would exist. For HDR BT plans, the PTV was taken to be the same as the CTV, while for the SBRT plans, two PTV scenarios were created, with 5- and 3-mm expansion of the GTV for the generation of the SBRT-specific PTV. For all cases, the plans created to deliver four fractions of 7 Gy. HDR BT plans were prescribed such that the prescription dose would fully cover the PTV, while SBRT plans aimed to deliver the highest possible dose to the GTV and PTVs [intermediate-risk PTV (IR-PTV) and high-risk PTV (HR-PTV)] while maintaining the same OAR DVH parameters previously achieved by the HDR BT plans. Since the SBRT planning constraints adopted the HDR BT-achieved OAR doses, these were very similar. The authors revealed that for IMRT plans limited to the HDR BT-achieved OAR constraints, GTV or PTV D90% was in general lower than the respective HDR BT plans. For IMPT plans, D90% was mostly similar or lower to that of HDR BT plans. When ratios of volumes receiving 3 and 3.5 Gy were compared, IMRT plans revealed volumes twice as large, and volumes receiving 5 Gy were 1.5 times as large as HDR BT plans, regardless of the PTV margin size. Volumes receiving 7 Gy were on average smaller in IMRT than HDR BT plans. For IMPT, volumes receiving 3, 3.5, and 5 Gy were approximately 1.5 times larger compared to HDR BT plans. They concluded that for image-guided cervical cancer sequential boost treatments, both IMRT and IMPT seem to be inferior to HDR BT (13).
Yildirim et al. (14) recently performed a similar retrospective dosimetric study comparing HDR BT plans against SBRT plans designed with VMAT technique on a conventional linear accelerator (Axesse, Elekta AB, Stockholm, Sweden) and on a Hi-Art Tomotherapy system (TomoTherapy Inc., Madison, WI). Twelve patients with early-stage endometrial cancer previously treated postoperatively with HDR BT were included and for which SBRT plans were retrospectively created with the two abovementioned technologies. The PTV was defined as a uniform 3D 5-mm expansion of the cylinder volume at the upper 3–5 mm of the vagina and was the same for all planning cases. The PD was 25 Gy in five fractions. The goal of the plan in both HDR BT and SBRT cases was that 95% of the PTV to receive at least 95% of the PD and 100% of the target volume to receive at least 90% of the PD. The authors reported total coverage of target volumes with 150%–250% of the PD for the HDR BT plans. The mean PTV D98% was 24.71 ± 0.36 Gy for the TomoTherapy plans and 24.42 ± 0.38 Gy for the VMAT plans, i.e., slightly lower than the prescription dose and were deemed adequate. Regarding the OAR doses, bladder D2cc was found significantly lower in HDR BT plans than in VMAT and TomoTherapy plans, with no significant differences observed in the rectum D2cc between the three plans. The authors concluded that their investigation demonstrated comparable PTV coverage between SBRT and HDR BT plans with lower inhomogeneity in the SBRT compared to the HDR BT plans and claimed that this study showed the feasibility of using SBRT as an alternative to HDR BT in endometrial cancer patients treated postoperatively (14). A limitation to this study was the use of the same PTV in both SBRT and HDR BT plans, created with the expansion of the CTV, even though it is unlikely to be performed clinically due to the inherent positioning uncertainties associated with external beam radiotherapy treatments.
As a result of a growing recent utilization of SBRT treatments instead of the established HDR BT option in clinical settings, Gill et al. (17) utilized the United States National Cancer Database for the evaluation of the potential impact of SBRT usage in cervical cancer treatments. A total of 7,654 patients with stage IIB–IVA cervical cancer for which boost modality information was available were used. Of these patients, 90.3% received HDR BT and the rest received IMRT or SBRT. It was observed that from 2004 to 2011, the use of brachytherapy decreased from 96.7% to 86.1%, while use of IMRT and SBRT increased from 3.3% to 13.9% (p < 0.01). The comparative survival analysis between the two modalities revealed that IMRT or SBRT boost resulted in inferior overall survival (hazard ratio, 1.86; 95% confidence interval, 1.35–2.55; p = 0.01) when compared to HDR BT boost. The authors concluded that the increased use of IMRT and SBRT techniques for delivering the boost dose in cervical cancer patients with the concurrent apparent increase in mortality risk should raise concerns when deciding on the use of these modalities over HDR BT outside of clinical trials.
Addressing the concern raised by the analysis by Gill et al. (17), the Society of Gynecologic Oncology and the American Brachytherapy Society recently published a review article (18) that includes a discussion on the comparison of brachytherapy with IMRT or SBRT. They conclude with the statement “(…) conformal external beam therapies such as IMRT or SBRT should not be used as alternatives to brachytherapy in patients undergoing primary curative-intent radiation therapy for cervical cancer”.
In the study of Milickovic et al. (15), five patients with intrathoracic malignancies (IMs) of different sizes, that received X knife SBRT, were selected for 192Ir HDR BT comparative plan analysis. These patients were planned with the treatment planning system (TPS) Oncentra MasterPlan v4.5 (Elekta, Veenendaal, Netherlands) with 9–10 non-coplanar 6 MV X-ray beams delivering doses from 5 Gy up to 20 Gy per fraction. In order to ensure healthy tissue sparing, the planned dose encompassing the PTV was set equal to 80% of the isocenter dose. By using the same structures (PTV and OARs), they generated HDR BT virtual plans with the Oncentra Brachytherapy v4.5 TPS, with the planning goal to achieve the same PTV with the PD of the SBRT and the aid of the hybrid inverse optimization algorithm installed in the TPS (19). The number of virtual catheters for the BRT plans ranged from 6 to 10, and the number of activated dwell positions pro cm3 was 3. For the comparative dosimetric analysis, DVH was calculated and the paired Student’s t-test with a p-value of 0.05 as significance threshold was applied.
The results of this study show a significantly better PTV coverage (p = 0.030) with the HDR BT for the V100% (93.04% of the PD) vs. the SBRT (88.94% of the PTV), while the other VX% and DX% parameters revealed no significant benefit of the HDR BT over SBRT, although the values are always greater for the HDR BT in comparison to the corresponding SBRT ones, except for the Dmin. The value of V150% for HDR BT equals to 24.67, while for SBRT is 0.0, as expected, indicating the ability of HDR BT for significant dose escalation inside the PTV (p = 4.84 × 10-4).
The CI, HTCI, CN, and COIN parameters did not show significant differences. For the high-dose spillage, there was no significant difference shown for both treatment methods because the amount of healthy tissue receiving doses more than 105% of the PD is low. For the intermediate-dose spillage, the R50% of the HDR BT treatment plans was significantly (p = 0.002) better (2.47) than the corresponding one of the SBRT (21.14), D2cm was significantly higher for the SBRT treatment plans than for the HDR BT (p < 0.001), and V20%–V80% was significantly in favor of HDR BT (p = 0.003). In the same study, it has been illustrated that this volume difference favors the usage of HDR BT with increasing PTV. The comparison of the dose indices of the OARs exhibited no statistically significant difference of the two methods, except for the Dmax in the spinal cord, where for HDR BT, the average value equals to 12.85Gy ± 7.42% and for the SBRT equals to 21.14Gy ± 5.72% of the PD (p = 0.022).
The study of Milickovic et al. (15) showed that SBRT is not dosimetrically superior to HDR BT and furthermore that HDR BT can deliver higher dose escalation inside the PTV in intrathoracic cases. Regarding larger lesions, where SBRT might not be able to deliver a sufficient dose due to normal tissue dose constraints, HDR BT is shown to manifest itself as a therapeutic option, considering the dosimetric aspect, although the results of the study might differ, if real implants are considered, because catheter placement alters the PTV anatomy.
Milickovic et al. (15) also selected five patients with cerebral malignancies (recurrent glioblastoma multiform) who received X knife stereotactic radiosurgery (SRS) for retrospective dosimetric comparison against 192Ir HDR BT. SRS and HDR BT plans were generated with the same considerations described above for the intrathoracic malignancies in the same publication.
The results show that similar coverage was achieved by the two modalities (HDR BT: 96.09%, SRS: 94.73%) with no statistically significant differences (p = 0.227). As mentioned above, VX% and DX% parameters revealed no statistically significant differences besides V150%, which was significantly higher for HDR BT compared to SRS plans.
The HTCI, CN, and COIN parameters did not show significant differences besides CI, which was significantly better (p = 0.026) for HDR BT than for SBRT. No significant differences were observed for R50%, while D2cm and V20%–V80% revealed significant differences in favor of HDR BT (p = 0.032 and p = 0.035, respectively). Dose incidence for OARs did not reveal any significant differences either between the two treatment modalities.
Milickovic et al. (15) conclude that HDR BT is at least as good as SRS in cerebral malignancies—when the anatomical position of the target allows needle placement—the steep dose fall-off was shown to be sharper in HDR BT plans, while intermediate- and low-dose spillage was as good or significantly lower in HDR BT plans.
This article deals with the illustration of the basic 192Ir physical properties, which justify its steep dose fall-off in its application for the interventional radiation oncology. Additionally, it reviews representative dosimetric comparison studies of the SBRT vs. the HDR BT modality (summary in Table 5). The clinical comparison and analysis review are beyond the scope of this article, as it is also for the assessment of the impact of the uncertainties each of the two modalities is linked to.
Table 5 Summary of study year, original clinical plan, quantitative treatment plan analysis metrics employed, and number of patient cases utilized for the studies mentioned in current review.
For prostate, two groups (8, 9) performed dosimetric comparison of real HDR BT plans with virtual SBRT plans, while the third group (10) compared real SBRT plans against virtual HDR BT plans. In general, SBRT revealed significantly better D100% and D99% than HDR BT due to the inherent limitation of HDR BT to fully cover areas inside the PTV that are anteriorly intersecting with the urethra. In terms of dose escalation, HDR BT showed significantly higher average V150% and V200% values compared to SBRT. HDR BT performed significantly better for the dose to the rectum and the bladder but not for the dose inside the urethra in the first (8) and third (10) studies. In the second study (7), HDR BT performed significantly better for rectum but worse for bladder and urethra than SBRT. Both modalities have their advantages and disadvantages regarding the PTV coverage and the sparing of the OARs, with only one dosimetric feature that might be clinically decisive in future clinical trials, namely, the dose escalation inside the prostate PTV, which HDR BT can provide.
In liver malignancies, Pennington et al. (11) dosimetrically compared SBRT and virtual HDR BT, treating the same PTV with both techniques, and concluded that HDR BT could achieve higher target dose, similar dose to OARs, but potentially lower minimum dose to the target. Hass et al. (12) concluded that HDR BT revealed superior outcomes in terms of both target coverage and exposure to the remaining healthy liver.
In gynecologic malignancies, Yildirim et al. (14) used the same PTV to generate plans for both modalities and demonstrated slightly lower but comparable PTV coverage for SBRT plans compared to HDR BT plans and similar doses to the OARs, except the bladder D2cc, which was found significantly lower in HDR BT plans. Georg et al. (13) used different PTVs for each modality and attempted to use the high-tech techniques each modality has to offer and revealed inferior performance for SBRT in terms of both target coverage and steepness of dose fall-off. A retrospective survival analysis based on the United States National Cancer Database (17), triggered by an apparent growing clinical implementation of SBRT replacing HDR BT, revealed an apparent increase in mortality risk associated with an increased use of IMRT and SBRT techniques, instead of HDR BT, for delivering the boost dose in cervical cancer patients.
For intrathoracic malignancies, SBRT has been shown by Milickovic et al. (15) to be non-superior to HDR BT in its dosimetric aspect. Especially with regard to larger PTVs, HDR BT holds value in covering locations, where SBRT is unable to deliver sufficient dose because of normal tissue dose constraints, because the low-dose regions around the PTV increase much faster for SBRT than in the case of the HDR BT (example in Figure 1). The dose fall-off sharpness of the “192Ir-knife” is moreover capable of boosting dose inside the PTV with its inherent inhomogeneous dose distribution, leading to a dose escalation that cannot be reached by SBRT for intrathoracic PTVs.
Figure 1 Comparison of a high-dose-rate brachytherapy (left) and stereotactic body radiotherapy (right) plans for lung malignancies in different patients.
In cerebral malignancies, Milickovic et al. (15) demonstrated that HDR BT can be at least as good as SRS when the anatomical location of the target allows for needle placement. Significant dose escalation with significantly lower intermediate- and low-dose spillage was also demonstrated to be achievable with HDR BT plans.
While the 192Ir BT (Iridium-Knife) with its invasive nature requires additional expertise, experience, and logistics in comparison with external beam radiotherapy ablative options (SBRT, SRS), it manifests itself as an extremely “sharp knife” in terms of delivering higher dose gradients and simultaneously reducing the delivered dose to adjacent OARs. Furthermore, the integral dose delivered to the patient can be significantly reduced in comparison to SBRT and SRS treatment options, according to the studies presented herein. Its ability to significantly escalate dose inside the target region may have a key role in the era of personalized treatments, aiding the investigations toward optimal combinations of ablative radiation doses combined with immunotherapy (20) or present a practical solution for tumors with radioresistant hypoxic regions (21). HDR BT and external beam ablative options should be considered equivalent and complementary radiotherapy techniques and chosen based on their merits for each anatomical region and individual patient and tumor characteristics.
YR and GA conceived and designed the review, collected and analyzed the representative studies from the literature, and wrote and approved the submitted version.
The authors declare that the research was conducted in the absence of any commercial or financial relationships that could be construed as a potential conflict of interest.
All claims expressed in this article are solely those of the authors and do not necessarily represent those of their affiliated organizations, or those of the publisher, the editors and the reviewers. Any product that may be evaluated in this article, or claim that may be made by its manufacturer, is not guaranteed or endorsed by the publisher.
1. Baltas D, Sakelliou L, Zamboglou N. The Physics of Modern Brachytherapy for Oncology. Boca Raton: CRC Press, Taylor & Francis Group (2007), ISBN: ISBN: 0-7503-0708-0.
2. Rivard MJ, Coursey BM, DeWerd LA, Hanson WF, Huq MS, Ibbott GS, et al. Update of AAPM Task Group No. 43 Report: A Revised AAPM Protocol for Brachytherapy Dose Calculations. Med Phys (2004) 31:633–74. doi: 10.1118/1.1646040
3. Perez-Calatayud J, Ballester F, Das RK, Dewerd LA, Ibbott GS, Meigooni AS, et al. Dose Calculation for Photon-Emitting Brachytherapy Sources With Average Energy Higher Than 50kev: Report of the AAPM and ESTRO. Med Phys (2012) 39:2904–29. doi: 10.1118/1.3703892
4. International atomic energy agency. Radiation Protection in Design of Radiotherapy Facilities, Safety Reports Series No. 47. Vienna: IAEA (2006).
5. Strohmaier S, Zwierzchowski G. Comparison of 60Co and 192Ir Sources in HDR Brachytherapy. J Contemp Brachyther (2011) 3(4):199–208. doi: 10.5114/jcb.2011.26471
6. Wang L, Li J, Paskalev K, Hoban P, Luo W, Chen L, et al. Commissioning and Quality Assurance of a Commercial Stereotactic Treatment-Planning System for Extracranial IMRT. J Appl Clin Med Phys (2006) 7:21–34. doi: 10.1120/jacmp.v7i1.2125
7. Bibault JE, Prevost B, Dansin E, Mirabel X, Lacornerie T, Lartigau E. Image-Guided Robotic Stereotactic Radiation Therapy With Fiducial-Free Tumor Tracking for Lung Cancer. Radiat Oncol (2012) 7:102. doi: 10.1186/1748-717X-7-102
8. Spratt DE, Scala LM, Folkert M, Voros L, Cohen GN, Happersett L, et al. A Comparative Dosimetric Analysis of Virtual Stereotactic Body Radiotherapy to High-Dose-Rate Monotherapy for Intermediate-Risk Prostate Cancer. Brachytherapy (2013) 12(5):428–33. doi: 10.1016/j.brachy.2013.03.003
9. Fukuda S, Seo Y, Shiomi H, Yamada Y, Ogata T, Morimoto M, et al. Dosimetry Analyses Comparing High-Dose-Rate Brachytherapy, Administered as Monotherapy for Localized Prostate Cancer, With Stereotactic Body Radiation Therapy Simulated Using CyberKnife. J Radiat Res (2014) 55(6):1114–21. doi: 10.1093/jrr/rru048
10. Chatzikonstantinou G, Keller C, Scherf C, Bathen B, Kohn J, Tselis N. Real-Word Dosimetric Comparison Between CyberKnife SBRT and HDR Brachytherapy for the Treatment of Prostate Cancer. Brachytherapy (2020) 20(1):44–9. doi: 10.1016/j.brachy.2020.07.011
11. Pennington JD, Park SJ, Abgaryan N, Banerjee R, Lee PP, Loh C, et al. Dosimetric Comparison of Brachyablation and Stereotactic Ablative Body Radiotherapy in the Treatment of Liver Metastasis. Brachytherapy (2015) 14:537–42. doi: 10.1016/j.brachy.2015.04.002
12. Hass P, Mohnike K, Kropf S, Brunner TB, Walke M, Albers D, et al. Comparative Analysis Between Interstitial Brachytherapy and Stereotactic Body Irradiation for Local Ablation in Liver Malignancies. Brachytherapy (2019) 18(6):823–8. doi: 10.1016/j.brachy.2019.08.003
13. Georg D, Kirisits C, Hillbrand M, Dimopoulos J, Potter R. Image-Guided Radiotherapy for Cervix Cancer: High-Tech External Beam Therapy Versus High-Tech Brachytherapy. Int J Radiat Oncol Biol Phys (2008) 71(4):1272–8. doi: 10.1016/j.ijrobp.2008.03.032
14. Yildirim BA, Dolek Y, Guler OC, Arslan G, Onal C. Dosimetric Comparison of Vaginal Vault Brachytherapy vs Applicator-Guided Stereotactic Body Radiotherapy With Volumetric Modulated Arc Therapy and Helical Tomotherapy for Endometrium Cancer Patients. Med Dosim (2019) 44(4):332–8. doi: 10.1016/j.meddos.2018.11.005
15. Milickovic N, Tselis N, Karagiannis E, Ferentinos K, Zamboglou N. Iridium Knife: Another Knife in Radiation Oncology. Brachytherapy (2017) 16(4):884–92. doi: 10.1016/j.brachy.2017.03.005
16. Kuban DA, Tucker SL, Dong L, Starkschall G, Huang EH, Cheung MR, et al. Long-Term Results of the M.D. Anderson Randomized Dose-Escalation Trial for Prostate Cancer. Int J Radiat Oncol Biol Phys (2008) 70:1003–7. doi: 10.1016/j.ijrobp.2007.06.054
17. Gill BS, Lin JF, Krivak TC, Sukumvanich P, Laskey RA, Ross MS, et al. National Cancer Data Base Analysis of Radiation Therapy Consolidation Modality for Cervical Cancer: The Impact of New Technological Advancements. Int J Radiat Oncol Biol Phys (2014) 90(5):1083–90. doi: 10.1016/j.ijrobp.2014.07.017
18. Holschneider CH, Petereit DG, Chu C, Hsu IC, Ioffe YJ, Klopp AH, et al. Brachytherapy: A Critical Component of Primary Radiation Therapy for Cervical Cancer. Brachytherapy (2019) 152(3):540–7. doi: 10.1016/j.brachy.2018.11.009
19. Karabis A, Giannouli S, Baltas D. HIPO: A Hybrid Inverse Treatment Planning Optimisation Algorithm in HDR Brachytherapy. Radiother Oncol (2005) 76:S29. doi: 10.1016/S0167-8140(05)81018-7
20. Filatenkov A, Baker J, Mueller AMS, Kenkel J, Ahn GO, Dutt S, et al. Ablative Tumor Radiation can Change the Tumor Immune Cell Microenvironment to Induce Durable Complete Remissions. Clin Cancer Res (2015) 21(16):3727–39. doi: 10.1158/1078-0432.CCR-14-2824
Keywords: iridium knife, brachytherapy, dosimetry, high-dose-rate (HDR) brachytherapy, stereotactic ablative radiotherapy (SABR), stereotactic body radiation therapy (SBRT), stereotactic radiosurgery (SRS), iridium-192
Citation: Roussakis Y and Anagnostopoulos G (2021) Physical and Dosimetric Aspects of the Iridium-Knife. Front. Oncol. 11:728452. doi: 10.3389/fonc.2021.728452
Received: 21 June 2021; Accepted: 19 October 2021;
Published: 09 November 2021.
Edited by:
Valdir Carlos Colussi, University Hospitals Cleveland Medical Center, United StatesReviewed by:
Wei Zhao, Beihang University, ChinaCopyright © 2021 Roussakis and Anagnostopoulos. This is an open-access article distributed under the terms of the Creative Commons Attribution License (CC BY). The use, distribution or reproduction in other forums is permitted, provided the original author(s) and the copyright owner(s) are credited and that the original publication in this journal is cited, in accordance with accepted academic practice. No use, distribution or reproduction is permitted which does not comply with these terms.
*Correspondence: Yiannis Roussakis, eWlhbm5pcy5yb3Vzc2FraXNAZ29jLmNvbS5jeQ==
†These authors have contributed equally to this work
Disclaimer: All claims expressed in this article are solely those of the authors and do not necessarily represent those of their affiliated organizations, or those of the publisher, the editors and the reviewers. Any product that may be evaluated in this article or claim that may be made by its manufacturer is not guaranteed or endorsed by the publisher.
Research integrity at Frontiers
Learn more about the work of our research integrity team to safeguard the quality of each article we publish.