- 1Department of Rheumatology and Clinical Immunology – Charité–Universitätsmedizin Berlin, Corporate Member of Freie Universität Berlin, Humboldt-Universität zu Berlin, and the Berlin Institute of Health (BIH), Berlin, Germany
- 2Deutsches Rheuma-Forschungszentrum (DRFZ Berlin) – a Leibniz Institute, Berlin, Germany
- 3Department of Haematology, Sheffield Teaching Hospitals Foundation NHS Trust, Sheffield, United Kingdom
- 4Department of Oncology and Metabolism, University of Sheffield, Sheffield, United Kingdom
- 5Department of Neuroscience, Uppsala University, Uppsala, Sweden
- 6Institute of Biotechnology, Technische Universität Berlin, Berlin, Germany
- 7Scleroderma Clinic, Dip. Reumatologia, ASST G. Pini-CTO, Milano, Italy
- 8Unité de Médecine Interne: (UF 04) CRMR MATHEC, Maladies Auto-Immunes et Thérapie Cellulaire, Paris, France
- 9Universite de Paris, IRSL, Recherche Clinique Appliquee `à l’´hématologie, Paris, France
- 10Department of Medicine, McGill University, Montreal, QC, Canada
- 11Centre for Immunobiology, Blizard Institute, Barts and the London School of Medicine, Queen Mary University of London, London, United Kingdom
- 12Service d’hématologie Clinique et de Thérapie Cellulaire, Hôpital Saint Antoine, APHP, Sorbonne Université, INSERM UMRs 938, Paris, France
- 13Department of Brain Sciences, Imperial College London, London, United Kingdom
- 14Unit of Hematology and Bone Marrow Transplantation, IRCCS San Raffaele Scientific Institute, Vita-Salute San Raffaele University, Milan, Italy
- 15IDIBAPS, CIBER-EHD, Barcelona, Spain
- 16Department of Neuroscience, Sheffield Teaching Hospitals NHS, Foundation Trust, Sheffield, United Kingdom
- 17NIHR Neurosciences Biomedical Research Centre, University of Sheffield, Sheffield, United Kingdom
Over the past decades, hematopoietic stem cell transplantation (HSCT) has been evolving as specific treatment for patients with severe and refractory autoimmune diseases (ADs), where mechanistic studies have provided evidence for a profound immune renewal facilitating the observed beneficial responses. The intestinal microbiome plays an important role in host physiology including shaping the immune repertoire. The relationships between intestinal microbiota composition and outcomes after HSCT for hematologic diseases have been identified, particularly for predicting the mortality from infectious and non-infectious causes. Furthermore, therapeutic manipulations of the gut microbiota, such as fecal microbiota transplant (FMT), have emerged as promising therapeutic approaches for restoring the functional and anatomical integrity of the intestinal microbiota post-transplantation. Although changes in the intestinal microbiome have been linked to various ADs, studies investigating the effect of intestinal dysbiosis on HSCT outcomes for ADs are scarce and require further attention. Herein, we describe some of the landmark microbiome studies in HSCT recipients and patients with chronic ADs, and discuss the challenges and opportunities of microbiome research for diagnostic and therapeutic purposes in the context of HSCT for ADs.
Introduction
Intestinal microbiota may positively affect many aspects of the host physiology, including absorption of nutrients, prevention of overgrowth by potential pathogens, maintenance of epithelial barrier function, and shaping the immune system (1). Studies of the microbiome in the setting of hematopoietic stem cell transplantation (HSCT) demonstrated that intestinal flora are of particular importance in determining treatment outcomes, influencing immune reconstitution, and impacting complications such as infections or graft-versus-host disease (GvHD) (2, 3). In addition, changes in the microbial composition and function have been associated with various autoimmune diseases (ADs), and, although the precise mechanistic links between the microbiome and ADs remain largely unknown, increasing evidence suggests that disturbed gut microbiota contribute to pathogenesis (4). Among the potential mechanisms in the complex interplay between gut microbiota and host immune system, abnormal microbial translocation, molecular mimicry, and dysregulation of local and systemic immunity have been postulated.
This article will summarize the current evidence supporting the relationship between the microbiome and specific ADs, its impact on transplant outcomes, and potential therapeutic interventions, such as fecal microbiota transplantation (FMT). Moving forward, we propose how we may evaluate and influence the microbiome in the setting of HSCT for ADs to affect immune reconstitution and potentially improve clinical outcomes.
Interaction Between Gut Microbiota and the Host Immune System
While the primary function of the intestinal microbiota for the host has been considered to be the digestion of complex sugars and the provision of essential vitamins, it has become clear that the microbiota play an important role in the education and shaping of a functioning immune system. Evidence for this comes from the analysis of germ-free mice, which in the absence of any microbiota have underdeveloped lymph organs and reduced innate immune competence resulting in increased susceptibility to infection (5). Most likely for similar reasons, germ-free mice are resistant to genetic and induced models of autoimmunity. While the molecular mechanisms are still poorly understood, several pathways involved in the microbiota–host interaction have been identified, ranging from provision of ligands for innate receptors, such as Toll-like receptors for “trained” immunity (6), to the production of short-chain fatty acids, a product of the metabolizing of dietary fibers by certain bacteria, which have been described to enhance immune regulation (7, 8). Reciprocally, the host controls the microbiota through the production of antimicrobial peptides by intestinal epithelial cells and copious amounts of IgA antibodies, which are actively transported into the gut lumen by the intestinal epithelial cells, controlling the growth, mobility and attachment of intestinal bacteria (9). Alterations to this intricate microbiota – host interaction, e.g. genetic defects disrupting microbial sensing of the host or loss of bacterial diversity, often summarized under the term dysbiosis, resulting in loss of microbial functions for the host, has been associated with the development of chronic inflammatory diseases (10). Mechanistically, several pathways have been discussed by which intestinal microbiota might contribute to the development or perpetuation of autoimmune diseases (11). They include gut dysbiosis, which disrupts local gut homeostasis and may promote translocation of commensal or pathobionts to tissues where they facilitate chronic inflammation. In addition, microbiota may trigger autoimmunity directly by providing antigenic stimuli resulting in cross-reactivity of autoreactive lymphocytes and autoantibodies with bacterial orthologues. Finally, microbiota may modulate the immune system through their metabolites and may facilitate immune regulation by stimulating regulatory immune elements (summarized in Figure 1).
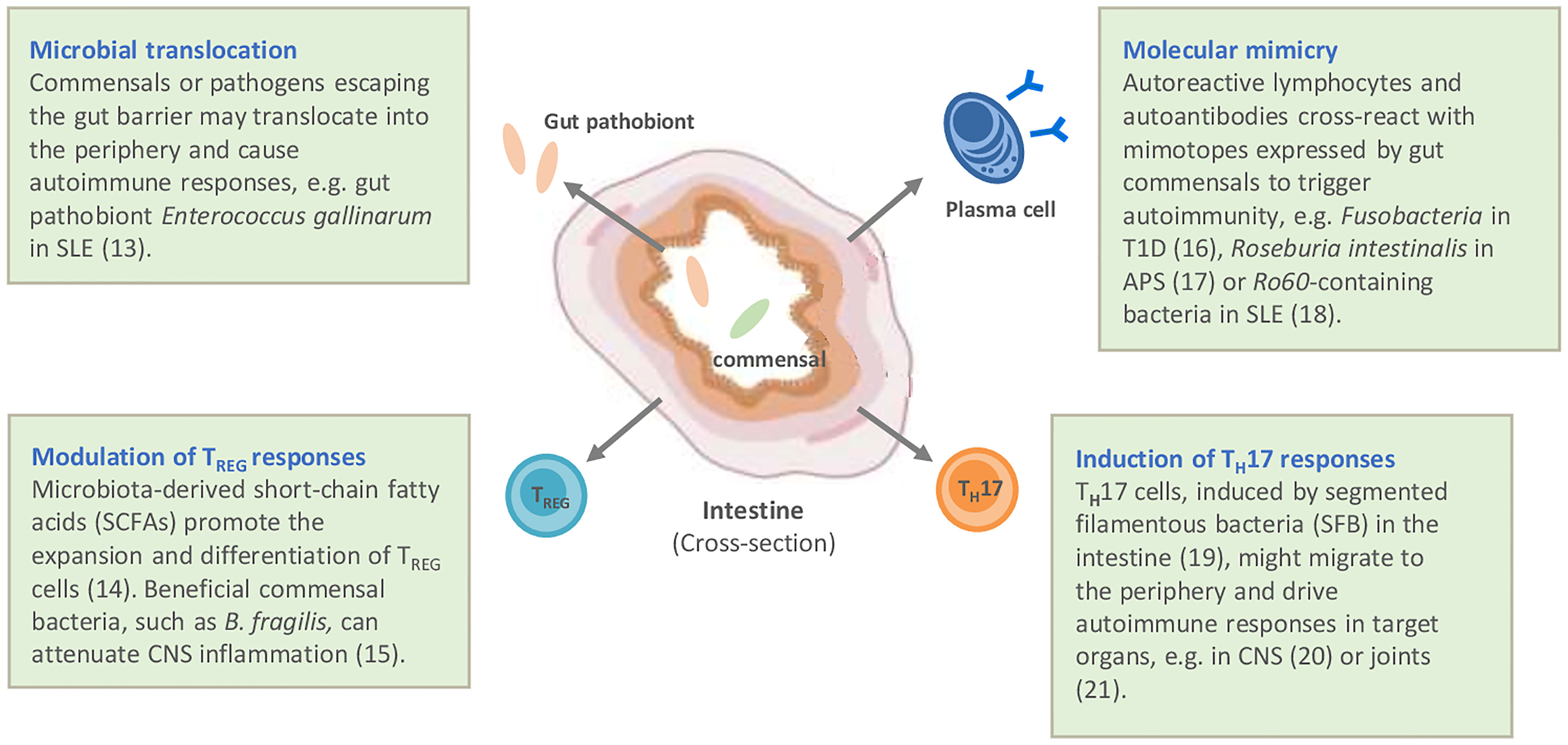
Figure 1 Potential mechanisms by which autoimmune diseases are linked to gut microbiota and intestinal immunity. Modified according to (12). APS, antiphospholipid syndrome; CNS, central nervous system; SLE, systemic lupus erythematosus; T1D, type I diabetes (13–21).
Microbial Profiling
The introduction of molecular biological methods for the characterization of the microbiota, in particular high-throughput sequencing, has greatly advanced our understanding of the diversity and function of the microbiota (22). Sequencing of the single or a combination of the 9 variable regions of the gene for the 16S ribosomal RNA of the small 30S ribosomal subunit is the mainstay to describe the composition of a microbial community. While 16S rRNA sequencing has become the method of choice due to its simplicity, it is often limited in the taxonomic resolution and is prone to bias e.g. PCR amplification and sampling depth (23). More extensive sequencing approaches include whole 16S rRNA gene sequencing allowing resolution of the microbiome to the species level, however often at the cost of sampling depth, and shot-gun metagenomics sequencing which will additionally yield information on the genetic repertoire, i.e. potential functional genes, of the bacterial community, the latter requiring extensive bioinformatics resources. Other “omics”, such as metaproteomics can also be used to define the composition as well as the function of the microbiota, while metabolomic profiling identifies the mediators with which the microbiota could interact within itself and with the host [reviewed in (24)]. Recently, the combination of absolute quantification of the microbiota by flow cytometry with 16S rRNA gene profiling was shown to better reflect clinically relevant changes of the microbiome in patients with inflammatory bowel diseases (IBD) (25). Flow cytometric analysis of the microbiota also has the potential to rapidly identify alterations in the microbiota on the single cell level for monitoring purposes (26) and when combined with cell sorting and 16S rRNA gene analysis could lead to the identification of relevant bacteria in a more targeted fashion.
Role of Intestinal Microbiota in Autoimmune Diseases
Inflammatory Bowel Diseases
The intestinal tract, home to the largest density and diversity of microorganisms in healthy humans, is the target organ of IBD comprising Crohn’s disease (CD) and Ulcerative Colitis (UC). The chronic intestinal inflammation in IBD is characterized by effector and tissue resident memory T cell responses to aspects of the intestinal microbiota (27). Despite large inter-individual variability, genetic analyses of microbial populations in stool and/or mucosal biopsies has revealed an overall decrease in diversity, a loss of symbionts and an increase in pathobionts (essentially Gram-negative proinflammatory microbes) in both UC and CD (27, 28). Whether these changes in microbial composition and IBD pathogenesis are a cause or consequence of intestinal inflammation remains a key area of study (29). Alterations in gut microbiota can disrupt epithelial and immune homeostasis, leading to increased permeability and eventual immune activation. Alternatively, the documented genetic and/or microbial-independent environmental factors associated with IBD may promote inflammation and oxidative stress, which subsequently results in a shift in microbial composition. A recent study has shown that increased fecal proteolytic activity and microbiota changes precede diagnoses of ulcerative colitis (30). In addition, the altered humoral and cellular acquired immune responses towards bacterial antigens that characterize IBD, particularly Crohn’s disease (31), may predate disease onset (32). This suggests that immune responses towards microbes, rather than microbial composition itself, drives epithelial barrier disruption and altered innate responses at disease onset. Thus, in marked contrast to the impressive efficacy seen in Clostridium difficile infection (33), FMT has shown some benefit in mild UC but no impact in CD (34–36). Nonetheless, regardless of whether dysbiosis is the initial event or the result of overt inflammation, shifts in microbial composition may help perpetuate disease, as well as impact response to therapy in IBD (37, 38), and thus represent a desirable target for future therapies.
Systemic Sclerosis
In systemic sclerosis (SSc), a rare systemic autoimmune disease characterized by vasculopathy, immune activation and consequent progressive fibrosis, multiple genetic, epigenetic, and environmental factors are regarded as potential triggers for the onset and progression of the disease (39). Over the past decades, emerging evidence suggests that alterations of microbial populations colonizing epithelial surfaces (i.e., gastrointestinal tract, skin and lung), known as dysbiosis, may contribute to chronic inflammation and autoimmunity (4). Since the gastrointestinal tract is one of the organs highly affected in SSc, recent studies have aimed to investigate gastrointestinal microbiota alterations to elucidate the possible interaction with disease phenotype and clinical outcome of the disease (40). Initial studies found that specific bacteria, particularly beneficial commensal genera (Faecalibacterium, Clostridium and Rikenella) and, conversely, more potentially pathobiont genera (Bifidobacterium, Fusobacterium and Prevotella) were decreased in SSc patients compared to healthy controls (41–43). Notably, SSc patients with more severe gastrointestinal symptoms exhibited a prevalence of the pathobiont Fusobacterium compared to patients with mild or no gastrointestinal symptoms (41–43). Furthermore, overabundance of opportunistic pathogenic Clostridium and typically oral Streptococcus species was recently described in SSc, while Alistipes, Bacteroides, and butyrate-producing species were depleted, congruent with findings in patients with IgG4-related disease, suggesting a common signature in both fibrosis-prone autoimmune diseases (44). Altogether, these studies confirm the existence of a shift in gut microbiota population in SSc patients. Whether these changes are causative or rather reflect the gastrointestinal involvement by inflammatory and fibrotic processes remains to be demonstrated. The role of intestinal dysbiosis in the disease pathogenesis is further complicated by the possibility that, as showed in other diseases, the intestinal microbiota in SSc might modulate local immunological mechanisms possibly responsible of local and systemic alterations (45).
Multiple Sclerosis
Multiple sclerosis (MS) is a chronic immune-mediated disease of the central nervous system (CNS), which results from interactions of genetic and environmental factors (46). The underlying pathological process is complex but includes the abnormal activation of T and B cells targeting foreign and/or self-antigens, which could be primed within the CNS or in the periphery (47, 48). A potential source of such antigens is the gut microbiome, which exhibits a level of homology to human myelin proteins and may trigger cross-reactivity through the mechanism of ‘molecular mimicry’ (49, 50). Immune reconstitution studies have shown that gut Mucosal Associated Invariant T (MAIT) cells, which express chemokine receptor 6 (CCR6) to facilitate their transmigration into the CNS, are reduced following autologous HSCT, suggesting that they may play a role in crosslinking gut microbiome with the neuroaxis (51). In one experimental model, the presence of intestinal microbiota was necessary to induce CNS autoimmunity, suggesting that the gut has the ability to control systemic autoimmune responses (52, 53). Germ-free mice recipients receiving feces from patients with MS develop severe Experimental Allergic Encephalomyelitis (EAE) and the administration of Lactobacilli seems to suppress this process (54, 55). A number of case control studies have reported reduced gut microbiome diversity in patients with MS, particularly in those with active disease, although a consistent microbiome phenotype has not been identified (56, 57). Furthermore, some MS orally administered disease modifying therapies have been found to inhibit the growth of Clostridium in vitro, which may contribute to their anti-inflammatory mechanism of action (58). Given the increasing evidence that gut microbiome plays a role in the immune system homeostasis and in the pathogenesis of MS, changes in the microbiome in patients with MS undergoing HSCT warrant investigation.
Role of Intestinal Microbiota in Hematopoietic Stem Cell Transplantation
Correlation With HSCT Outcomes
The intestinal microbiome undergoes profound changes during the course of transplantation. Multiple transplant-related factors (i.e. conditioning regimen, broad-spectrum antibiotics, nutrition) drive microbial shifts. At the same time, the alteration in the composition of gut flora is associated with transplant outcomes, including overall survival (OS), progression-free survival (PFS), treatment-related mortality (TRM) and GvHD (Table 1). Bacterial diversity largely decreases after HSCT, and is correlated with increased risk of major transplant complications such as infections or GvHD, potentially affecting the outcome of the procedure (81, 82). A large multicenter observational study has confirmed lower mortality rates in patients showing higher diversity of intestinal microbiota at engraftment (3). Recently, microbiota injury has been observed also in recipients of autologous HSCT, who undergo similar antibiotic exposures and nutritional alterations after high-dose chemotherapy and transplant procedure (80). Reduced OS and PFS have been reported in patients with lower peri-engraftment microbiome diversity.
Impact of chemotherapy, Diet, and Antibiotics on the Intestinal Microbiome in Transplant Recipients
Microbiome and transplant correlations may be influenced by local practices, antibiotic choices, hospital flora, and diet. Gastrointestinal disturbances associated with chemotherapy and radiation (83) and subsequent mucositis can also impact the composition of intestinal microbiota. A reduction in α-diversity and significant differences in the composition of the intestinal microbiota have been observed in response to chemotherapy, such as increase in Bacteroides and Enterobacteriaceae paralleled by a decrease in Bifidobacterium, Faecalibacterium prausnitzii, and Clostridium cluster XIVa (84), and a drastic drop in Faecalibacterium accompanied by an increase of Escherichia (85). The impact of diet on gut flora is well-recognized (86). Depletion of the intestinal microbiota reduces visceral adipose tissue and caloric uptake from diet (87), and enteral feeding may exert a beneficial effect on intestinal flora by providing the required nutrients (88). Interestingly, a lactose-free diet can prevent microbial overdominance by detrimental commensal bacteria like Enterococcus (72). Broad-spectrum antibiotic prophylaxis/treatment, commonly used in HSCT recipients, in the early phase after HSCT can beneficially reduce the number of transmigrated bacteria. However, their long-term effects are detrimental, because they limit microbiota diversity, by killing beneficial commensal bacteria that inhibit pathogens and promote immune defenses (81). A drastic decrease in the diversity of enteric microbiome after administration of antibiotic therapy, and the loss of obligate anaerobic commensal bacteria such as Clostridia and Bacteroidetes after piperacillin-tazobactam and meropenem administration, are recurrent in literature (89). Metronidazole administration increases enterococcal domination, whereas fluoroquinolone administration reduces domination by Proteobacteria (59) and represents an important variable associated with overall survival (61). Broad spectrum antibiotics, by inducing loss of bacterial diversity, are also associated with increased GvHD-related mortality (65, 90).
Intestinal Microbiome, Immune Reconstitution, and Infection Prevention
Effective and appropriate immune reconstitution is central to successful HSCT. Microbiota populations may influence immune reconstitution and cell dynamics in humans (91). The depletion of the intestinal microbiota impairs post-transplant immune reconstitution (87). Analysis of daily changes in circulating immune cell counts and extended longitudinal microbiota analysis revealed consistent associations between gut bacteria and immune cell dynamics, paving the way for potential microbiota-targeted interventions to improve immunotherapy and treatments for immune-mediated diseases (91).
The gut microbiota play a critical role in maintaining colonization resistance against intestinal pathogens, thus preventing infections. Domination by Enterococcus and Proteobacteria are associated with the risk of bacteremia by Vancomycin-resistant Enterococcus and gram-negative rod respectively (59). A different baseline distribution of the gut microbiome (68) has been reported in patients at risk for microbiologically confirmed infection (high level of Enterobacteriaceae, low level of Lachnospiraceae), sepsis and septic shock (high level of Enterobacteriaceae). Moreover, a documented bloodstream infection may be anticipated by expansion and dominance of pathogenic strains in the gut flora (59, 68, 92, 93). Overall, a low diversity of the intestinal microbiota at engraftment has been shown to be an independent predictor of TRM from both infectious and non-infectious causes (61).
Intestinal Microbiome, GvHD, and Immunosurveillance
In the allogeneic transplant setting, a regulatory effect of the gut microbiota in the maintenance of intestinal homeostasis has been reported (94). Loss of fecal diversity, as well as increased abundance of members from Enterococcus or Staphylococcus species have been associated with the incidence and severity of acute GvHD (79), while other organisms such as Blautia species have a protective role (64). Metabolites produced by intestinal bacteria may promote intestinal tissue homeostasis and immune tolerance in the context of acute GvHD (95). Moreover, commensal bacteria can also play a role in tumor immunosurveillance. Increased abundance of a cluster of related bacteria including Eubacterium limosum was associated with decreased risk of relapse or disease-progression (67).
Altogether, these results indicate that the intestinal microbiota represent a potentially important factor in the success or failure of HSCT. As such, the microbiome can be envisioned both as a biomarker for the identification of patients at higher risk for transplant-related complications, and also a target for intervention aiming to impact clinical outcomes through enhancing microbiota recovery (96).
Modulation of Gut Microbiota by Fecal Microbiota Transplantation
FMT is a recommended therapeutic strategy for treating recurrent Clostridioides difficile infection (97, 98). Additionally, FMT has been investigated for treatment of steroid-resistant acute GvHD and initial positive results (99) were confirmed by several case reports (100). A small cohort study recently reported a complete response in 10 out of 14 patients (71%) with steroid-refractory or steroid-dependent acute GvHD 28 days after FMT (101). This response was accompanied by an increase in microbial α-diversity, a partial engraftment of donor bacterial species, and increased abundance of butyrate-producing bacteria, including groups in the order Clostridiales, namely Blautia species. Malard et al. recently reported the use of a next-generation FMT product “MaaT013”, a standardized, pooled-donor, high-richness microbiota biotherapeutic, in the largest cohort of patients to date (n=29) with steroid-refractory or steroid-dependent intestinal acute GvHD (102). These patients had previously received and failed 1 to 5 lines of GvHD systemic treatments. The product was well tolerated and at day 28, overall response and complete remission rates were 59% and 31%, respectively. Furthermore, some studies have evaluated the role of FMT in treating dysbiosis after allogeneic HSCT. Taur et al. reported that autologous FMT after HSCT was safe and boosted microbial diversity, restoring bacterial populations lost during HSCT and reversing the disruptive effects of the broad-spectrum antibiotics (n=14) (81). Overall, FMT appears to be a promising strategy and several studies are ongoing to evaluate FMT for acute GvHD management (NCT03812705, NCGT03492502, NCT03359980, NCT03720392, NCT03678493). Regarding prevention of complications, additional studies are warranted to confirm that restoration of gut microbiota dysbiosis after FMT translates into clinical improvement after allogeneic HSCT, in particular a lower incidence of acute GvHD (96).
Discussion
It is increasingly accepted that understanding the complex interactions between the microbiome and immune system will be crucial to defining the pathogenesis of ADs, whilst optimizing therapeutic interventions and clinical outcomes. HSCT is increasingly used specifically to treat severe, resistant ADs, with now more than 3000 cases being reported to the registry of the European Society of Bone and Marrow Transplantation (EBMT) (103, 104). To date very limited data is available regarding microbiome biology in the setting of HSCT for ADs, where medium to long-term clinical outcomes are considered to be due to the induction of altered (or ‘re-booted’) immune reconstitution post-transplant. The ‘immune re-boot’ has been increasingly characterized in a range of ADs with a range of immunological markers, including evidence of generation of ‘re-educated’ and regulatory populations to support re-induction of self-tolerance lasting beyond the broad immunosuppressive effects of autologous HSCT (105, 106). Changes in immune reconstitution may affect not only on disease activity, but also adverse events, such as secondary ADs (107–110).
As for ADs outside the transplant setting, and for GvHD in allogeneic HSCT, the microbiome may significantly influence the baseline status of the underlying AD pre-transplant, the patients general condition peri-transplant (which will inevitably be influenced by the treatment and supportive care, especially antibiotics), and then the dynamics of the reconstituting immune system post-transplant. The microbiome may therefore influence short- and long-term immune recovery and clinical outcomes following autologous HSCT. Therefore, future investigations evaluating microbiome changes pre-, peri- and post-HSCT in ADs patients are warranted. Table 2 includes proposed recommendations for studies of the microbiome (111–115) that could be compared with clinical outcome and laboratory data related to immune reconstitution in patients undergoing HSCT for various ADs. Although bio-banking and testing cannot be regarded as routine care, they could be integrated into clinical trials or observational studies with appropriate institutional approvals. In future, a greater understanding may help design of prospective studies of interventions, including FMT, to test the proof of principle of modulation of the microbiome in this setting.
In conclusion, we have summarized the current evidence supporting the relationship between the microbiome, HSCT and ADs, and speculated on the potential impact of the microbiome on clinical outcomes and immune reconstitution following HSCT for severe, resistant ADs. The evidence in this specific field is currently very limited, warranting harmonization of the microbiome monitoring and prospective studies to evaluate properly any potential impact and/or clinical benefit.
Data Availability Statement
The original contributions presented in the study are included in the article/supplementary material. Further inquiries can be directed to the corresponding authors.
Author Contributions
TA, RG, and JS led on concept and design. TA and RG led on coordination and data analysis, provided expert and analytical feedback and were involved in reviewing, writing and editing the manuscript. All authors contributed to the analysis and interpretation of data, and writing sections of the manuscript. The experts on this panel are active members of the EBMT. All co-authors were involved in drafting the paper, revising it critically, and approval of the submitted and final versions.
Funding
The EBMT provided resources via the working party, data office and registry. H-DC is supported by the Dr. Rolf M. Schwiete Foundation and the German Research Foundation through CRC TRR241 B03.
Conflict of Interest
AS reports research grants from Roche-Genentech, Abbvie, GSK, Scipher Medicine, Alimentiv, Inc, Boehringer Ingelheim and Origo Biopharma; consulting fees from Genentech, GSK, Pfizer, HotSpot Therapeutics, Surrozen, Alimentiv, Origo Biopharma and Morphic Therapeutic. FM reports lecture honoraria from Therakos/Mallinckrodt, Janssen, Biocodex, Sanofi, JAZZ pharmaceuticals and Astellas, all outside the submitted work. MM reports grants and lecture honoraria from Janssen, Sanofi, and JAZZ pharmaceuticals, lecture honoraria from Celgene, Amgen, BMS, Takeda, and Pfizer, consultancy honoraria from MaaT Pharma and grants from Roche, all outside the submitted work. JS declares honoraria for speaking at educational events supported by Jazz, Mallinckrodt, Gilead, Janssen, and Actelion, advisory board participation for Medac, and IDMC membership for a Kiadis Pharma supported clinical trial, none of which are directly related to this review. PM discloses travel support and speaker honoraria from unrestricted educational activities organized by Novartis, Bayer HealthCare, Bayer Pharma, Biogen Idec, Merck-Serono and Sanofi Aventis; and consulting to Magenta Therapeutics and Jasper Therapeutics. RG discloses honoraria for speaking from educational events supported by Biotest, Pfizer, and Magenta. TA received financial support from Amgen, Janssen, Neovii and Mallinckrodt.
The remaining authors declare that the research was conducted in the absence of any commercial or financial relationships that could be construed as a potential conflict of interest.
Publisher’s Note
All claims expressed in this article are solely those of the authors and do not necessarily represent those of their affiliated organizations, or those of the publisher, the editors and the reviewers. Any product that may be evaluated in this article, or claim that may be made by its manufacturer, is not guaranteed or endorsed by the publisher.
Acknowledgments
The authors contribute this article on behalf of Autoimmune Diseases Working Party (ADWP) of the European Society for Blood and Marrow Transplantation (EBMT). We acknowledge the ADWP for support in working party activities and outputs; and all EBMT member centers and their clinicians, data managers, and patients for their essential contributions to the EBMT registry.
The EBMT Autoimmune Diseases Working Party (ADWP) included: Raffaella Greco (ADWP Chair), Tobias Alexander (ADWP Secretary), John Snowden (ADWP Chair April 2016-May 2020, EBMT Secretary), Manuela Badoglio (ADWP Study Coordinator), Myriam Labopin (ADWP Statistician) and actively participating clinicians; Mario Abinun, Shashikant Apte, Renate Arnold, Claudia Boglione (ADWP EBMT Nurses Group representative), Charlotte Brierley, Joachim Burman, Cristina Castilla-Llorente, Nichola Cooper, Giulia Daghia, Thomas Daikeler, Nicoletta del Papa, Jeska de Vries-Bouwstra, Dominique Farge, Jurgen Finke, Hans Hagglund, Chris Hawkey, Jörg Henes, Falk Hiepe, Helen Jessop (EBMT Nurses Group representative), David Kiely, Majid Kazmi, Kirill Kirgizov, Ellen Kramer, Gianluigi Mancardi, Zora Marjanovic, Roland Martin, Thierry Martin, David Ma, John Moore, Paul Miller, Paolo Muraro, Maria-Carolina Oliveira, Alexey Polushin, Francesco Onida, Belinda Simoes, Mathieu Puyade, Igor Resnick, Elena Ricart, Montserrat Rovira, Riccardo Saccardi, Muhammad Saif, Ioanna Sakellari, Basil Sharrack, Emilian Snarski, Hans-Ulrich Scherer, Claudia Sossa, Barbara Withers, Nico Wulffraat, Eleanora Zaccara.
References
1. Belkaid Y, Hand TW. Role of the Microbiota in Immunity and Inflammation. Cell (2014) 157(1):121–41. doi: 10.1016/j.cell.2014.03.011
2. Taur Y. Intestinal Microbiome Changes and Stem Cell Transplantation: Lessons Learned. Virulence (2016) 7(8):930–8. doi: 10.1080/21505594.2016.1250982
3. Peled JU, Gomes ALC, Devlin SM, Littmann ER, Taur Y, Sung AD, et al. Microbiota as Predictor of Mortality in Allogeneic Hematopoietic-Cell Transplantation. N Engl J Med (2020) 382(9):822–34. doi: 10.1056/NEJMoa1900623
4. Wu WH, Zegarra-Ruiz DF, Diehl GE. Intestinal Microbes in Autoimmune and Inflammatory Disease. Front Immunol (2020) 11:597966. doi: 10.3389/fimmu.2020.597966
5. Round JL, Mazmanian SK. The Gut Microbiota Shapes Intestinal Immune Responses During Health and Disease. Nat Rev Immunol (2009) 9(5):313–23. doi: 10.1038/nri2515
6. Ganal SC, Sanos SL, Kallfass C, Oberle K, Johner C, Kirschning C, et al. Priming of Natural Killer Cells by Nonmucosal Mononuclear Phagocytes Requires Instructive Signals From Commensal Microbiota. Immunity (2012) 37(1):171–86. doi: 10.1016/j.immuni.2012.05.020
7. Arpaia N, Campbell C, Fan X, Dikiy S, van der Veeken J, deRoos P, et al. Metabolites Produced by Commensal Bacteria Promote Peripheral Regulatory T-Cell Generation. Nature (2013) 504(7480):451–5. doi: 10.1038/nature12726
8. Smith PM, Howitt MR, Panikov N, Michaud M, Gallini CA, Bohlooly YM, et al. The Microbial Metabolites, Short-Chain Fatty Acids, Regulate Colonic Treg Cell Homeostasis. Science (2013) 341(6145):569–73. doi: 10.1126/science.1241165
9. Macpherson AJ, Yilmaz B, Limenitakis JP, Ganal-Vonarburg SC. Iga Function in Relation to the Intestinal Microbiota. Annu Rev Immunol (2018) 36:359–81. doi: 10.1146/annurev-immunol-042617-053238
10. Petersen C, Round JL. Defining Dysbiosis and Its Influence on Host Immunity and Disease. Cell Microbiol (2014) 16(7):1024–33. doi: 10.1111/cmi.12308
11. Kamada N, Seo SU, Chen GY, Nunez G. Role of the Gut Microbiota in Immunity and Inflammatory Disease. Nat Rev Immunol (2013) 13(5):321–35. doi: 10.1038/nri3430
12. Salvador R, Zhang A, Horai R, Caspi RR. Microbiota as Drivers and as Therapeutic Targets in Ocular and Tissue Specific Autoimmunity. Front Cell Dev Biol (2020) 8:606751. doi: 10.3389/fcell.2020.606751
13. Manfredo Vieira S, Hiltensperger M, Kumar V, Zegarra-Ruiz D, Dehner C, Khan N, et al. Translocation of a Gut Pathobiont Drives Autoimmunity in Mice and Humans. Science (2018) 359(6380):1156–61. doi: 10.1126/science.aar7201
14. Geuking MB, Cahenzli J, Lawson MA, Ng DC, Slack E, Hapfelmeier S, et al. Intestinal Bacterial Colonization Induces Mutualistic Regulatory T Cell Responses. Immunity (2011) 34(5):794–806. doi: 10.1016/j.immuni.2011.03.021
15. Ochoa-Reparaz J, Mielcarz DW, Wang Y, Begum-Haque S, Dasgupta S, Kasper DL, et al. A Polysaccharide From the Human Commensal Bacteroides Fragilis Protects Against CNS Demyelinating Disease. Mucosal Immunol (2010) 3(5):487–95. doi: 10.1038/mi.2010.29
16. Tai N, Peng J, Liu F, Gulden E, Hu Y, Zhang X, et al. Microbial Antigen Mimics Activate Diabetogenic CD8 T Cells in NOD Mice. J Exp Med (2016) 213(10):2129–46. doi: 10.1084/jem.20160526
17. Ruff WE, Dehner C, Kim WJ, Pagovich O, Aguiar CL, Yu AT, et al. Pathogenic Autoreactive T and B Cells Cross-React With Mimotopes Expressed by a Common Human Gut Commensal to Trigger Autoimmunity. Cell Host Microbe (2019) 26(1):100–113 e8. doi: 10.1016/j.chom.2019.05.003
18. Greiling TM, Dehner C, Chen X, Hughes K, Iniguez AJ, Boccitto M, et al. Commensal Orthologs of the Human Autoantigen Ro60 as Triggers of Autoimmunity in Lupus. Sci Transl Med (2018) 10(434):eaan2306. doi: 10.1126/scitranslmed.aan2306
19. Ivanov II, Atarashi K, Manel N, Brodie EL, Shima T, Karaoz U, et al. Induction of Intestinal Th17 Cells by Segmented Filamentous Bacteria. Cell (2009) 139(3):485–98. doi: 10.1016/j.cell.2009.09.033
20. Lee YK, Menezes JS, Umesaki Y, Mazmanian SK. Proinflammatory T-Cell Responses to Gut Microbiota Promote Experimental Autoimmune Encephalomyelitis. Proc Natl Acad Sci USA (2011) 108 Suppl 1:4615–22. doi: 10.1073/pnas.1000082107
21. Wu HJ, Ivanov II, Darce J, Hattori K, Shima T, Umesaki Y, et al. Mathis: Gut-Residing Segmented Filamentous Bacteria Drive Autoimmune Arthritis via T Helper 17 Cells. Immunity (2010) 32(6):815–27. doi: 10.1016/j.immuni.2010.06.001
22. Human Microbiome Project C. Structure, Function and Diversity of the Healthy Human Microbiome. Nature (2012) 486(7402):207–14. doi: 10.1038/nature11234
23. Kennedy K, Hall MW, Lynch MD, Moreno-Hagelsieb G, Neufeld JD. Evaluating Bias of Illumina-Based Bacterial 16S Rrna Gene Profiles. Appl Environ Microbiol (2014) 80(18):5717–22. doi: 10.1128/AEM.01451-14
24. Zhang X, Li L, Butcher J, Stintzi A, Figeys D. Advancing Functional and Translational Microbiome Research Using Meta-Omics Approaches. Microbiome (2019) 7(1):154. doi: 10.1186/s40168-019-0767-6
25. Vandeputte D, Kathagen G, D’Hoe K, Vieira-Silva S, Valles-Colomer M, Sabino J, et al. Quantitative Microbiome Profiling Links Gut Community Variation to Microbial Load. Nature (2017) 551(7681):507–11. doi: 10.1038/nature24460
26. Zimmermann J, Hubschmann T, Schattenberg F, Schumann J, Durek P, Riedel R, et al. High-Resolution Microbiota Flow Cytometry Reveals Dynamic Colitis-Associated Changes in Fecal Bacterial Composition. Eur J Immunol (2016) 46(5):1300–3. doi: 10.1002/eji.201646297
27. Paap EM, Muller TM, Sommer K, Neurath MF, Zundler S. Total Recall: Intestinal TRM Cells in Health and Disease. Front Immunol (2020) 11:623072. doi: 10.3389/fimmu.2020.623072
28. Vich Vila A, Imhann F, Collij V, Jankipersadsing SA, Gurry T, Mujagic Z, et al. Gut Microbiota Composition and Functional Changes in Inflammatory Bowel Disease and Irritable Bowel Syndrome. Sci Transl Med (2018) 10(472):eaap8914. doi: 10.1126/scitranslmed.aap8914
29. Ni J, Wu GD, Albenberg L, Tomov VT. Gut Microbiota and IBD: Causation or Correlation? Nat Rev Gastroenterol Hepatol (2017) 14(10):573–84. doi: 10.1038/nrgastro.2017.88
30. Galipeau HJ, Caminero A, Turpin W, Bermudez-Brito M, Santiago A, Libertucci J, et al. Novel Fecal Biomarkers That Precede Clinical Diagnosis of Ulcerative Colitis. Gastroenterology (2021) 160(5):1532–45. doi: 10.1053/j.gastro.2020.12.004
31. Calderon-Gomez E, Bassolas-Molina H, Mora-Buch R, Dotti I, Planell N, Esteller M, et al. Commensal-Specific Cd4(+) Cells From Patients With Crohn’s Disease Have a T-Helper 17 Inflammatory Profile. Gastroenterology (2016) 151(3):489–500.e3. doi: 10.1053/j.gastro.2016.05.050
32. Choung RS, Princen F, Stockfisch TP, Torres J, Maue AC, Porter CK, et al. Serologic Microbial Associated Markers can Predict Crohn’s Disease Behaviour Years Before Disease Diagnosis. Aliment Pharmacol Ther (2016) 43(12):1300–10. doi: 10.1111/apt.13641
33. van Nood E, Vrieze A, Nieuwdorp M, Fuentes S, Zoetendal EG, de Vos WM, et al. Duodenal Infusion of Donor Feces for Recurrent Clostridium Difficile. N Engl J Med (2013) 368(5):407–15. doi: 10.1056/NEJMoa1205037
34. Costello SP, Soo W, Bryant RV, Jairath V, Hart AL, Andrews JM. Systematic Review With Meta-Analysis: Faecal Microbiota Transplantation for the Induction of Remission for Active Ulcerative Colitis. Aliment Pharmacol Ther (2017) 46(3):213–24. doi: 10.1111/apt.14173
35. Imdad A, Nicholson MR, Tanner-Smith EE, Zackular JP, Gomez-Duarte OG, Beaulieu DB, et al. Fecal Transplantation for Treatment of Inflammatory Bowel Disease. Cochrane Database Syst Rev (2018) 11:CD012774. doi: 10.1002/14651858.CD012774.pub2
36. Paramsothy S, Paramsothy R, Rubin DT, Kamm MA, Kaakoush NO, Mitchell HM, et al. Faecal Microbiota Transplantation for Inflammatory Bowel Disease: A Systematic Review and Meta-Analysis. J Crohns Colitis (2017) 11(10):1180–99. doi: 10.1093/ecco-jcc/jjx063
37. Aden K, Rehman A, Waschina S, Pan WH, Walker A, Lucio M, et al. Metabolic Functions of Gut Microbes Associate With Efficacy of Tumor Necrosis Factor Antagonists in Patients With Inflammatory Bowel Diseases. Gastroenterology (2019) 157(5):1279–92.e11. doi: 10.1053/j.gastro.2019.07.025
38. Ananthakrishnan AN, Luo C, Yajnik V, Khalili H, Garber JJ, Stevens BW, et al. Gut Microbiome Function Predicts Response to Anti-Integrin Biologic Therapy in Inflammatory Bowel Diseases. Cell Host Microbe (2017) 21(5):603–610 e3. doi: 10.1016/j.chom.2017.04.010
39. Gabrielli A, Avvedimento EV, Krieg T. Scleroderma. N Engl J Med (2009) 360(19):1989–2003. doi: 10.1056/NEJMra0806188
40. Bellocchi C, Fernandez-Ochoa A, Montanelli G, Vigone B, Santaniello A, Milani C, et al. Microbial and Metabolic Multi-Omic Correlations in Systemic Sclerosis Patients. Ann N Y Acad Sci (2018) 1421(1):97–109. doi: 10.1111/nyas.13736
41. Andreasson K, Alrawi Z, Persson A, Jonsson G, Marsal J. Intestinal Dysbiosis is Common in Systemic Sclerosis and Associated With Gastrointestinal and Extraintestinal Features of Disease. Arthritis Res Ther (2016) 18(1):278. doi: 10.1186/s13075-016-1182-z
42. Volkmann ER, Hoffmann-Vold AM, Chang YL, Jacobs JP, Tillisch K, Mayer EA, et al. Systemic Sclerosis is Associated With Specific Alterations in Gastrointestinal Microbiota in Two Independent Cohorts. BMJ Open Gastroenterol (2017) 4(1):e000134. doi: 10.1136/bmjgast-2017-000134
43. Patrone V, Puglisi E, Cardinali M, Schnitzler TS, Svegliati S, Festa A, et al. Gut Microbiota Profile in Systemic Sclerosis Patients With and Without Clinical Evidence of Gastrointestinal Involvement. Sci Rep (2017) 7(1):14874. doi: 10.1038/s41598-017-14889-6
44. Plichta DR, Somani J, Pichaud M, Wallace ZS, Fernandes AD, Perugino CA, et al. Congruent Microbiome Signatures in Fibrosis-Prone Autoimmune Diseases: Igg4-Related Disease and Systemic Sclerosis. Genome Med (2021) 13(1):35. doi: 10.1186/s13073-021-00853-7
45. Durack J, Lynch SV. The Gut Microbiome: Relationships With Disease and Opportunities for Therapy. J Exp Med (2019) 216(1):20–40. doi: 10.1084/jem.20180448
46. Compston A, Coles A. Multiple Sclerosis. Lancet (2008) 372(9648):1502–17. doi: 10.1016/S0140-6736(08)61620-7
47. Lindert RB, Haase CG, Brehm U, Linington C, Wekerle H, Hohlfeld R. Multiple Sclerosis: B- and T-Cell Responses to the Extracellular Domain of the Myelin Oligodendrocyte Glycoprotein. Brain (1999) 122(Pt 11):2089–100. doi: 10.1093/brain/122.11.2089
48. Correale J, de los Milagros Bassani Molinas M. Time Course of T-Cell Responses to MOG and MBP in Patients With Clinically Isolated Syndromes. J Neuroimmunol (2003) 136(1-2):162–71. doi: 10.1016/s0165-5728(03)00035-3
49. Bhargava P, Mowry EM. Gut Microbiome and Multiple Sclerosis. Curr Neurol Neurosci Rep (2014) 14(10):492. doi: 10.1007/s11910-014-0492-2
50. Probstel AK, Baranzini SE. The Role of the Gut Microbiome in Multiple Sclerosis Risk and Progression: Towards Characterization of the “Ms Microbiome”. Neurotherapeutics (2018) 15(1):126–34. doi: 10.1007/s13311-017-0587-y
51. Abrahamsson SV, Angelini DF, Dubinsky AN, Morel E, Oh U, Jones JL, et al. Non-Myeloablative Autologous Haematopoietic Stem Cell Transplantation Expands Regulatory Cells and Depletes IL-17 Producing Mucosal-Associated Invariant T Cells in Multiple Sclerosis. Brain (2013) 136(Pt 9):2888–903. doi: 10.1093/brain/awt182
52. Berer K, Mues M, Koutrolos M, Rasbi ZA, Boziki M, Johner C, et al. Commensal Microbiota and Myelin Autoantigen Cooperate to Trigger Autoimmune Demyelination. Nature (2011) 479(7374):538–41. doi: 10.1038/nature10554
53. Yokote H, Miyake S, Croxford JL, Oki S, Mizusawa H, Yamamura T. NKT Cell-Dependent Amelioration of a Mouse Model of Multiple Sclerosis by Altering Gut Flora. Am J Pathol (2008) 173(6):1714–23. doi: 10.2353/ajpath.2008.080622
54. Cekanaviciute E, Yoo BB, Runia TF, Debelius JW, Singh S, Nelson CA, et al. Gut Bacteria From Multiple Sclerosis Patients Modulate Human T Cells and Exacerbate Symptoms in Mouse Models. Proc Natl Acad Sci USA (2017) 114(40):10713–8. doi: 10.1073/pnas.1711235114
55. Takata K, Kinoshita M, Okuno T, Moriya M, Kohda T, Honorat JA, et al. The Lactic Acid Bacterium Pediococcus Acidilactici Suppresses Autoimmune Encephalomyelitis by Inducing IL-10-Producing Regulatory T Cells. PloS One (2011) 6(11):e27644. doi: 10.1371/journal.pone.0027644
56. Jangi S, Gandhi R, Cox LM, Li N, von Glehn F, Yan R, et al. Alterations of the Human Gut Microbiome in Multiple Sclerosis. Nat Commun (2016) 7:12015. doi: 10.1038/ncomms12015
57. Mentis AA, Dardiotis E, Grigoriadis N, Petinaki E, Hadjigeorgiou GM. Viruses and Endogenous Retroviruses in Multiple Sclerosis: From Correlation to Causation. Acta Neurol Scand (2017) 136(6):606–16. doi: 10.1111/ane.12775
58. Katz Sand I, Zhu Y, Ntranos A, Clemente JC, Cekanaviciute E, Brandstadter R, et al. Disease-Modifying Therapies Alter Gut Microbial Composition in MS. Neurol Neuroimmunol Neuroinflamm (2019) 6(1):e517. doi: 10.1212/NXI.0000000000000517
59. Taur Y, Xavier JB, Lipuma L, Ubeda C, Goldberg J, Gobourne A, et al. Intestinal Domination and the Risk of Bacteremia in Patients Undergoing Allogeneic Hematopoietic Stem Cell Transplantation. Clin Infect Dis (2012) 55(7):905–14. doi: 10.1093/cid/cis580
60. Ubeda C, Bucci V, Caballero S, Djukovic A, Toussaint NC, Equinda M, et al. Intestinal Microbiota Containing Barnesiella Species Cures Vancomycin-Resistant Enterococcus Faecium Colonization. Infect Immun (2013) 81(3):965–73. doi: 10.1128/IAI.01197-12
61. Taur Y, Jenq RR, Perales MA, Littmann ER, Morjaria S, Ling L, et al. The Effects of Intestinal Tract Bacterial Diversity on Mortality Following Allogeneic Hematopoietic Stem Cell Transplantation. Blood (2014) 124(7):1174–82. doi: 10.1182/blood-2014-02-554725
62. Holler E, Butzhammer P, Schmid K, Hundsrucker C, Koestler J, Peter K, et al. Metagenomic Analysis of the Stool Microbiome in Patients Receiving Allogeneic Stem Cell Transplantation: Loss of Diversity Is Associated With Use of Systemic Antibiotics and More Pronounced in Gastrointestinal Graft-Versus-Host Disease. Biol Blood Marrow Transplant (2014) 20(5):640–5. doi: 10.1016/j.bbmt.2014.01.030
63. Weber D, Oefner PJ, Hiergeist A, Koestler J, Gessner A, Weber M, et al. Low Urinary Indoxyl Sulfate Levels Early After Transplantation Reflect a Disrupted Microbiome and Are Associated With Poor Outcome. Blood (2015) 126(14):1723–8. doi: 10.1182/blood-2015-04-638858
64. Jenq RR, Taur Y, Devlin SM, Ponce DM, Goldberg JD, Ahr KF, et al. Intestinal Blautia Is Associated With Reduced Death From Graft-Versus-Host Disease. Biol Blood Marrow Transplant (2015) 21(8):1373–83. doi: 10.1016/j.bbmt.2015.04.016
65. Shono Y, Docampo MD, Peled JU, Perobelli SM, Velardi E, Tsai JJ, et al. Increased GVHD-Related Mortality With Broad-Spectrum Antibiotic Use After Allogeneic Hematopoietic Stem Cell Transplantation in Human Patients and Mice. Sci Transl Med (2016) 8(339):339ra71. doi: 10.1126/scitranslmed.aaf2311
66. Harris B, Morjaria SM, Littmann ER, Geyer AI, Stover DE, Barker JN, et al. Gut Microbiota Predict Pulmonary Infiltrates After Allogeneic Hematopoietic Cell Transplantation. Am J Respir Crit Care Med (2016) 194(4):450–63. doi: 10.1164/rccm.201507-1491OC
67. Peled JU, Devlin SM, Staffas A, Lumish M, Khanin R, Littmann ER, et al. Intestinal Microbiota and Relapse After Hematopoietic-Cell Transplantation. J Clin Oncol (2017) 35(15):1650–9. doi: 10.1200/JCO.2016.70.3348
68. Mancini N, Greco R, Pasciuta R, Barbanti MC, Pini G, Morrow OB, et al. Enteric Microbiome Markers as Early Predictors of Clinical Outcome in Allogeneic Hematopoietic Stem Cell Transplant: Results of a Prospective Study in Adult Patients. Open Forum Infect Dis (2017) 4(4):ofx215. doi: 10.1093/ofid/ofx215
69. Doki N, Suyama M, Sasajima S, Ota J, Igarashi A, Mimura I, et al. Clinical Impact of Pre-Transplant Gut Microbial Diversity on Outcomes of Allogeneic Hematopoietic Stem Cell Transplantation. Ann Hematol (2017) 96(9):1517–23. doi: 10.1007/s00277-017-3069-8
70. Lee YJ, Arguello ES, Jenq RR, Littmann E, Kim GJ, Miller LC, et al. Protective Factors in the Intestinal Microbiome Against Clostridium Difficile Infection in Recipients of Allogeneic Hematopoietic Stem Cell Transplantation. J Infect Dis (2017) 215(7):1117–23. doi: 10.1093/infdis/jix011
71. Golob JL, Pergam SA, Srinivasan S, Fiedler TL, Liu C, Garcia K, et al. Stool Microbiota at Neutrophil Recovery Is Predictive for Severe Acute Graft vs Host Disease After Hematopoietic Cell Transplantation. Clin Infect Dis (2017) 65(12):1984–91. doi: 10.1093/cid/cix699
72. Stein-Thoeringer CK, Nichols KB, Lazrak A, Docampo MD, Slingerland AE, Slingerland JB, et al. Lactose Drives Enterococcus Expansion to Promote Graft-Versus-Host Disease. Science (2019) 366(6469):1143–9. doi: 10.1126/science.aax3760
73. Galloway-Pena JR, Peterson CB, Malik F, Sahasrabhojane PV, Shah DP, Brumlow CE, et al. Fecal Microbiome, Metabolites, and Stem Cell Transplant Outcomes: A Single-Center Pilot Study. Open Forum Infect Dis (2019) 6(5):ofz173. doi: 10.1093/ofid/ofz173
74. Biagi E, Zama D, Rampelli S, Turroni S, Brigidi P, Consolandi C, et al. Early Gut Microbiota Signature of Agvhd in Children Given Allogeneic Hematopoietic Cell Transplantation for Hematological Disorders. BMC Med Genomics (2019) 12(1):49. doi: 10.1186/s12920-019-0494-7
75. Han L, Zhang H, Chen S, Zhou L, Li Y, Zhao K, et al. Intestinal Microbiota can Predict Acute Graft-Versus-Host Disease Following Allogeneic Hematopoietic Stem Cell Transplantation. Biol Blood Marrow Transplant (2019) 25(10):1944–55. doi: 10.1016/j.bbmt.2019.07.006
76. Lee SE, Lim JY, Ryu DB, Kim TW, Park SS, Jeon YW, et al. Alteration of the Intestinal Microbiota by Broad-Spectrum Antibiotic Use Correlates With the Occurrence of Intestinal Graft-Versus-Host Disease. Biol Blood Marrow Transplant (2019) 25(10):1933–43. doi: 10.1016/j.bbmt.2019.06.001
77. Payen M, Nicolis I, Robin M, Michonneau D, Delannoye J, Mayeur C, et al. Functional and Phylogenetic Alterations in Gut Microbiome Are Linked to Graft-Versus-Host Disease Severity. Blood Adv (2020) 4(9):1824–32. doi: 10.1182/bloodadvances.2020001531
78. Han L, Zhao K, Li Y, Han H, Zhou L, Ma P, et al. A Gut Microbiota Score Predicting Acute Graft-Versus-Host Disease Following Myeloablative Allogeneic Hematopoietic Stem Cell Transplantation. Am J Transplant (2020) 20(4):1014–27. doi: 10.1111/ajt.15654
79. Greco R, Nitti R, Mancini N, Pasciuta R, Lorentino F, Lupo-Stanghellini MT, et al. Microbiome Markers Are Early Predictors of Acute GVHD in Allogeneic Hematopoietic Stem Cell Transplant Recipients. Blood (2021) 137(11):1556–9. doi: 10.1182/blood.2020007158
80. Khan N, Lindner S, Gomes ALC, Devlin SM, Shah GL, Sung AD, et al. Fecal Microbiota Diversity Disruption and Clinical Outcomes After Auto-HCT: A Multicenter Observational Study. Blood (2021) 137(11):1527–37. doi: 10.1182/blood.2020006923
81. Taur Y, Coyte K, Schluter J, Robilotti E, Figueroa C, Gjonbalaj M, et al. Reconstitution of the Gut Microbiota of Antibiotic-Treated Patients by Autologous Fecal Microbiota Transplant. Sci Transl Med (2018) 10(460):eaap9489. doi: 10.1126/scitranslmed.aap9489
82. Peled JU, Jenq RR, Holler E, van den Brink MR. Role of Gut Flora After Bone Marrow Transplantation. Nat Microbiol (2016) 1:16036. doi: 10.1038/nmicrobiol.2016.36
83. Liu J, Liu C, Yue J. Radiotherapy and the Gut Microbiome: Facts and Fiction. Radiat Oncol (2021) 16(1):9. doi: 10.1186/s13014-020-01735-9
84. Touchefeu Y, Montassier E, Nieman K, Gastinne T, Potel G, Bruley des Varannes S, et al. Systematic Review: The Role of the Gut Microbiota in Chemotherapy- or Radiation-Induced Gastrointestinal Mucositis - Current Evidence and Potential Clinical Applications. Aliment Pharmacol Ther (2014) 40(5):409–21. doi: 10.1111/apt.12878
85. Montassier E, Batard E, Massart S, Gastinne T, Carton T, Caillon J, et al. 16S Rrna Gene Pyrosequencing Reveals Shift in Patient Faecal Microbiota During High-Dose Chemotherapy as Conditioning Regimen for Bone Marrow Transplantation. Microb Ecol (2014) 67(3):690–9. doi: 10.1007/s00248-013-0355-4
86. David LA, Maurice CF, Carmody RN, Gootenberg DB, Button JE, Wolfe BE, et al. Diet Rapidly and Reproducibly Alters the Human Gut Microbiome. Nature (2014) 505(7484):559–63. doi: 10.1038/nature12820
87. Staffas A, Burgos da Silva M, Slingerland AE, Lazrak A, Bare CJ, Holman CD, et al. Nutritional Support From the Intestinal Microbiota Improves Hematopoietic Reconstitution After Bone Marrow Transplantation in Mice. Cell Host Microbe (2018) 23(4):447–57.e4. doi: 10.1016/j.chom.2018.03.002
88. Staffas A, Burgos da Silva M, van den Brink MR. The Intestinal Microbiota in Allogeneic Hematopoietic Cell Transplant and Graft-Versus-Host Disease. Blood (2017) 129(8):927–33. doi: 10.1182/blood-2016-09-691394
89. Morjaria S, Schluter J, Taylor BP, Littmann ER, Carter RA, Fontana E, et al. Antibiotic-Induced Shifts in Fecal Microbiota Density and Composition During Hematopoietic Stem Cell Transplantation. Infect Immun (2019) 87(9):e00206–19. doi: 10.1128/IAI.00206-19
90. Weber D, Jenq RR, Peled JU, Taur Y, Hiergeist A, Koestler J, et al. Microbiota Disruption Induced by Early Use of Broad-Spectrum Antibiotics Is an Independent Risk Factor of Outcome After Allogeneic Stem Cell Transplantation. Biol Blood Marrow Transplant (2017) 23(5):845–52. doi: 10.1016/j.bbmt.2017.02.006
91. Schluter J, Peled JU, Taylor BP, Markey KA, Smith M, Taur Y, et al. The Gut Microbiota is Associated With Immune Cell Dynamics in Humans. Nature (2020) 588(7837):303–7. doi: 10.1038/s41586-020-2971-8
92. Taur Y, Jenq RR, Ubeda C, van den Brink M, Pamer EG. Role of Intestinal Microbiota in Transplantation Outcomes. Best Pract Res Clin Haematol (2015) 28(2-3):155–61. doi: 10.1016/j.beha.2015.10.013
93. Ubeda C, Taur Y, Jenq RR, Equinda MJ, Son T, Samstein M, et al. Vancomycin-Resistant Enterococcus Domination of Intestinal Microbiota is Enabled by Antibiotic Treatment in Mice and Precedes Bloodstream Invasion in Humans. J Clin Invest (2010) 120(12):4332–41. doi: 10.1172/JCI43918
94. Atarashi K, Tanoue T, Oshima K, Suda W, Nagano Y, Nishikawa H, et al. Treg Induction by a Rationally Selected Mixture of Clostridia Strains From the Human Microbiota. Nature (2013) 500(7461):232–6. doi: 10.1038/nature12331
95. Kohler N, Zeiser R. Intestinal Microbiota Influence Immune Tolerance Post Allogeneic Hematopoietic Cell Transplantation and Intestinal GVHD. Front Immunol (2018) 9:3179. doi: 10.3389/fimmu.2018.03179
96. Malard F, Mohty M. Let’s Reconstitute Microbiota Diversity. Blood (2021) 137(11):1442–4. doi: 10.1182/blood.2020009963
97. Debast SB, Bauer MP, Kuijper EJ. European Society of Clinical Microbiology and Infectious Diseases: Update of the Treatment Guidance Document for Clostridium Difficile Infection. Clin Microbiol Infect (2014) 20 (Suppl 2):1–26. doi: 10.1111/1469-0691.12418
98. McDonald LC, Gerding DN, Johnson S, Bakken JS, Carroll KC, Coffin SE, et al. Clinical Practice Guidelines for Clostridium Difficile Infection in Adults and Children: 2017 Update by the Infectious Diseases Society of America (IDSA) and Society for Healthcare Epidemiology of America (SHEA). Clin Infect Dis (2018) 66(7):987–94. doi: 10.1093/cid/ciy149
99. Kakihana K, Fujioka Y, Suda W, Najima Y, Kuwata G, Sasajima S, et al. Fecal Microbiota Transplantation for Patients With Steroid-Resistant Acute Graft-Versus-Host Disease of the Gut. Blood (2016) 128(16):2083–8. doi: 10.1182/blood-2016-05-717652
100. Shouval R, Geva M, Nagler A, Youngster I. Fecal Microbiota Transplantation for Treatment of Acute Graft-Versus-Host Disease. Clin Hematol Int (2019) 1(1):28–35. doi: 10.2991/chi.d.190316.002
101. van Lier YF, Davids M, Haverkate NJE, de Groot PF, Donker ML, Meijer E, et al. Donor Fecal Microbiota Transplantation Ameliorates Intestinal Graft-Versus-Host Disease in Allogeneic Hematopoietic Cell Transplant Recipients. Sci Transl Med (2020) 12(556):eaaz8926. doi: 10.1126/scitranslmed.aaz8926
102. Malard F, Loschi M, Legrand F, Devillier R, Guenounou S, Borel C, et al. Successful and Safe Treatment of Intestinal Graft-Versus-Host Disease (Gvhd) With Pooled-Donor Full Ecosystem Microbiota Biotherapeutic: Results From a 29 Patient-Cohort of a Compassionate Use/Expanded Access Treatment Program. Blood (2020) 136(Suppl 1):15–5. doi: 10.1182/blood-2020-136604
103. Snowden JA, Badoglio M, Labopin M, Giebel S, McGrath E, Marjanovic Z, et al. Evolution, Trends, Outcomes, and Economics of Hematopoietic Stem Cell Transplantation in Severe Autoimmune Diseases. Blood Adv (2017) 1(27):2742–55. doi: 10.1182/bloodadvances.2017010041
104. Alexander T, Greco R, Snowden JA. Hematopoietic Stem Cell Transplantation for Autoimmune Disease. Annu Rev Med (2021) 72:215–28. doi: 10.1146/annurev-med-070119-115617
105. Alexander T, Farge D, Badoglio M, Lindsay JO, Muraro PA, Snowden JA. Hematopoietic Stem Cell Therapy for Autoimmune Diseases - Clinical Experience and Mechanisms. J Autoimmun (2018) 92:35–46. doi: 10.1016/j.jaut.2018.06.002
106. Swart JF, Delemarre EM, van Wijk F, Boelens JJ, Kuball J, van Laar JM, et al. Haematopoietic Stem Cell Transplantation for Autoimmune Diseases. Nat Rev Rheumatol (2017) 13(4):244–56. doi: 10.1038/nrrheum.2017.7
107. Bosch M, Khan FM, Storek J. Immune Reconstitution After Hematopoietic Cell Transplantation. Curr Opin Hematol (2012) 19(4):324–35. doi: 10.1097/MOH.0b013e328353bc7d
108. Burt RK, Muraro PA, Farge D, Oliveira MC, Snowden JA, Saccardi R, et al. New Autoimmune Diseases After Autologous Hematopoietic Stem Cell Transplantation for Multiple Sclerosis. Bone Marrow Transplant (2021) 56(7):1509–17. doi: 10.1038/s41409-021-01277-y
109. Farge D, Arruda LC, Brigant F, Clave E, Douay C, Marjanovic Z, et al. Long-Term Immune Reconstitution and T Cell Repertoire Analysis After Autologous Hematopoietic Stem Cell Transplantation in Systemic Sclerosis Patients. J Hematol Oncol (2017) 10(1):21. doi: 10.1186/s13045-016-0388-5
110. Muraro PA, Martin R, Mancardi GL, Nicholas R, Sormani MP, Saccardi R. Autologous Haematopoietic Stem Cell Transplantation for Treatment of Multiple Sclerosis. Nat Rev Neurol (2017) 13(7):391–405. doi: 10.1038/nrneurol.2017.81
111. Wang Z, Zolnik CP, Qiu Y, Usyk M, Wang T, Strickler HD, et al. Comparison of Fecal Collection Methods for Microbiome and Metabolomics Studies. Front Cell Infect Microbiol (2018) 8:301. doi: 10.3389/fcimb.2018.00301
112. Vogtmann E, Chen J, Kibriya MG, Chen Y, Islam T, Eunes M, et al. Comparison of Fecal Collection Methods for Microbiota Studies in Bangladesh. Appl Environ Microbiol (2017) 83(10):e00361–17. doi: 10.1128/AEM.00361-17
113. Tang Q, Jin G, Wang G, Liu T, Liu X, Wang B, et al. Current Sampling Methods for Gut Microbiota: A Call for More Precise Devices. Front Cell Infect Microbiol (2020) 10:151. doi: 10.3389/fcimb.2020.00151
114. Gorzelak MA, Gill SK, Tasnim N, Ahmadi-Vand Z, Jay M, Gibson DL. Methods for Improving Human Gut Microbiome Data by Reducing Variability Through Sample Processing and Storage of Stool. PloS One (2015) 10(8):e0134802. doi: 10.1371/journal.pone.0134802
Keywords: autoimmune diseases, autoimmunity, fecal transplantation, intestinal, microbiome, stem cell transplantation, HSCT = hematopoietic stem cell transplant
Citation: Alexander T, Snowden JA, Burman J, Chang H-D, Del Papa N, Farge D, Lindsay JO, Malard F, Muraro PA, Nitti R, Salas A, Sharrack B, Mohty M and Greco R (2021) Intestinal Microbiome in Hematopoietic Stem Cell Transplantation For Autoimmune Diseases: Considerations and Perspectives on Behalf of Autoimmune Diseases Working Party (ADWP) of the EBMT. Front. Oncol. 11:722436. doi: 10.3389/fonc.2021.722436
Received: 08 June 2021; Accepted: 07 October 2021;
Published: 22 October 2021.
Edited by:
Weili Zhao, Shanghai Jiao Tong University, ChinaReviewed by:
Sarah Wall, The Ohio State University, United StatesRehan Khan, Case Western Reserve University, United States
Copyright © 2021 Alexander, Snowden, Burman, Chang, Del Papa, Farge, Lindsay, Malard, Muraro, Nitti, Salas, Sharrack, Mohty and Greco. This is an open-access article distributed under the terms of the Creative Commons Attribution License (CC BY). The use, distribution or reproduction in other forums is permitted, provided the original author(s) and the copyright owner(s) are credited and that the original publication in this journal is cited, in accordance with accepted academic practice. No use, distribution or reproduction is permitted which does not comply with these terms.
*Correspondence: Tobias Alexander, tobias.alexander@charite.de; Raffaella Greco, greco.raffaella@hsr.it