- 1Department of Immunology and Pathogenic Biology, Yanbian University Medical College, Yanji, China
- 2Department of Dermatology, Graduate School of Medicine, Osaka University, Osaka, Japan
- 3Laboratory of Cutaneous Immunology, Osaka University Immunology Frontier Research Center, Osaka, Japan
Dihydroartemisinin (DHA) has been globally recognized for its efficacy and safety in the clinical treatment of malaria for decades. Recently, it has been found that DHA inhibits malignant tumor growth and regulates immune system function in addition to anti-malaria. In parasites and tumors, DHA causes severe oxidative stress by inducing excessive reactive oxygen species production. DHA also kills tumor cells by inducing programmed cell death, blocking cell cycle and enhancing anti-tumor immunity. In addition, DHA inhibits inflammation by reducing the inflammatory cells infiltration and suppressing the production of pro-inflammatory cytokines. Further, genomics, proteomics, metabolomics and network pharmacology of DHA therapy provide the basis for elucidating the pharmacological effects of DHA. This review provides a summary of the recent research progress of DHA in anti-tumor, inhibition of inflammatory diseases and the relevant pharmacological mechanisms. With further research of DHA, it is likely that DHA will become an alternative therapy in the clinical treatment of malignant tumors and inflammatory diseases.
1 Introduction
Dihydroartemisinin (DHA), a derivative of artemisinin, is the active metabolite of artemisinin-like compounds in vivo (1, 2). DHA is widely used in the clinical treatment of malaria and has saved countless lives, due to its 100% efficiency against malaria parasites and low toxicity. DHA kills plasmodium parasites by damaging their membranes, disrupting their mitochondrial function and causing oxidative stress through producing excessive reactive oxide species (ROS) (3, 4). In addition to directly killing malaria parasites, it has recently been found that DHA enhances the ability of the immune system to resist parasites, such as malaria parasites and toxoplasma gondii. Jagannathan et al. find that Ugandan children who receive DHA preventative administration from 6 to 24 months old have a lower incidence of malaria, more IL-2+CD4+ T cells and TNF-α+CD4+ T cells, and fewer IL-10+CD4+ T cells than their peers who do not receive DHA (5). Zhang and colleagues find that DHA increases the number of T-helper (Th) cells and CD8+ T cells and the levels of IL-5 and IL-22, decreases the number of B cells and the content of some inflammatory cytokines, including TNF-α, IFN-γ, IL-2, IL-4, IL-6, and IL-10 in the mice who are infected with Toxoplasma gondii or Plasmodium Berghei (6). The above studies show that DHA can not only directly kill parasites, but also prevent hosts from parasitic diseases by regulating T cell subsets and cytokines production.
With the development of DHA research, it has been found that DHA has the pharmacological effect of anti-tumor and inhibition inflammation (7–12). Our laboratory has investigated the anti-tumor and inhibition inflammation effects of DHA and conducts a preliminary analysis of its mechanisms. It has been found that DHA inhibits the growth and metastasis of melanoma in mice by several ways (13). In addition, DHA also significantly alleviates imiquimod-induced mice psoriatic lesions (14). The pharmacological effects of DHA on tumors, pathogen infections, etc. over the past decades have been described in the review of Ho et al. (15), Efferth (16) and Slezakova et al. (17). Therefore, this article will review the last advances in DHA anti-tumor (Table 1) and anti-inflammation (Table 2) studies in recent years.
2 The Anti-Tumor Activity of DHA
Malignant tumors threaten the life safety of people all over the world and have become the main cause of death together with cardiovascular and cerebrovascular diseases and respiratory diseases (115). It has been demonstrated that DHA exerts significant anti-tumor activity in a variety of malignant tumors, and it has no toxicity to normal cells at appropriate doses (18, 20, 85, 94, 116, 117). It has been found that DHA not only is used as an adjuvant drug in combination with other chemotherapy drugs to improve drug resistance and enhance tumor killing function, but also has a significant inhibitory effect on tumors (21, 29, 34, 39, 45, 46, 70). Gao et al. find that combination of DHA and resveratrol inhibits the proliferation, metastasis and invasion of Hep2 and MDA-MB-231 cancer cells (57). Chen et al. confirm that DHA alleviates the resistance of multiple myeloma to dexamethasone (73). Hu and colleagues demonstrate that DHA/miR-29b combination therapy significantly inhibits proliferation and promotes apoptosis of cholangiocarcinoma cells (31). Guo et al. find that DHA increases the sensitivity of drug-resistant breast cancer cells to chemodynamic therapy, and plays a role in promoting chemotherapy as an adjuvant therapy drug (25). Similar to the mechanism by which DHA kills plasmodium parasites, DHA causes oxidative stress in tumor cells by lysing the peroxide groups, then leading tumor to death (55). In addition, DHA also plays an anti-tumor role through inhibiting tumor metastasis, inducing programmed cell death, blocking cell cycle, and enhancing anti-tumor immunity.
2.1 Inhibition of Tumor Metastasis
Tumor metastasis is one of the main causes of tumor treatment failure. Epithelial-mesenchymal transformation (EMT), matrix metalloproteinases (MMPs) secretion and neovascularization are closely related to tumor metastasis. DHA has exerted anti-metastasis effect in a variety of tumors (26, 44, 47, 50, 68, 118). In our study, DHA inhibits the melanoma metastatic capacity by reducing the production of MMPs in vivo and in vitro (13). Furthermore, DHA significantly reduces the number of pulmonary melanoma nodules, inhibits EMT, and decreases the expression of MMPs in the mouse melanoma lung metastasis model (Data not shown). Li et al. find that DHA inhibits EMT of ovarian cancer, thereby reducing its metastasis to the lung, liver and intestine (82). TGF-β-Smad signaling pathway and Akt signaling pathway play important roles in DHA suppressing tumor metastasis. In breast cancer, DHA inhibits EMT by reducing TGF-β production and decreasing phosphorylation of Samd2 and Smad3 (27). DHA inhibits phosphorylation of Akt in glioblastoma cells, thereby inhibiting EMT and reducing MMPs secretion (51). Similarly, Ju et al. observe that DHA inhibits breast cancer metastasis by reducing the production of MMPs, vascular endothelial growth factor (VEGF), and TGF-β (23).
As metastatic tumors grow, they induce angiogenesis by upregulating the expression of HIF-1α and VEGF to combat the lack of oxygen and nutrients within the tumor. It has been shown that DHA induces autophagy in human umbilical vein endothelial cells by inhibiting Akt and mTOR signaling pathways, thereby inhibiting their ability to generate blood vessels in vitro (66). In addition, DHA also inhibits angiogenesis by increasing the expression of VEFGR1, a deceptive receptor of VEGF, to block the binding of VEGF and VEFGR2 (67). Li et al. find that DHA inhibits esophageal cancer growth and metastasis by inhibiting the phosphorylation of p65 and reducing the activity of HIF-1α and VEGF (40). These suggest that DHA has a strong inhibitory effect on angiogenesis.
2.2 Induction of Programmed Cell Death in Tumors
2.2.1 Apoptosis
Apoptosis, a kind of programmed cell death, is the main way that drugs cause tumor cell death and is regulated by many factors, such as Bcl-2 protein family (12, 35, 48). In non-small cell lung cancer (NSCLC), DHA inhibits the expression of anti-apoptotic Bcl-2, disrupts the balance of the Bcl-2 family, and induces apoptosis (76). Similarly, in cervical cancer, DHA regulates Bcl-2 family protein expression and induces HeLa cell apoptosis by inhibiting the phosphorylation of mTOR (30).
In addition to changing the balance of members of the Bcl-2 protein family, DHA causes the accumulation of ROS in tumor cells, leading to mitochondrial damage (61). With excessive damage of mitochondrial, caspase cascade is activated and eventually activates caspase 3, the executor of apoptosis, causing mitochondrial apoptosis (19). Im et al. find that DHA increases the expression of cleaved caspase 8, cleaved caspase 9 and cleaved caspase 3 by inhibiting MAKP signaling pathway in hepatocellular carcinoma, thus inducing caspase-dependent apoptosis (62). Li et al. use β-dihydroartemisinin-Emodin to treat human liver cancer HepG-2 cells and similar results are observed. β-dihydroartemisinin-Emodin activates caspase 8, caspase 9 and caspase 3 and induces apoptosis by regulating the balance of Bcl-2/Bax (60). In our study, DHA increases the ratio of Bax/Bcl-2, activates caspase cascade and induces mitochondrial apoptosis by regulating STAT3 and other signaling pathways, thereby inhibiting mouse melanoma (13). In addition, DHA also induces endoplasmic reticulum stress (119). In glioblastoma cells, DHA not only induces mitochondrial apoptosis mediated by cleaved caspase 9 and cleaved caspase 3, but also induces endoplasmic reticulum stress pathway of apoptosis by activating caspase 12 (52). Interestingly, Luo et al. demonstrate that DHA induces endoplasmic reticulum stress by activating PERK in porcine ovarian cancer (120). These results indicate that DHA has a wide range of anti-tumor effects, not only its pharmacological mechanisms are diverse, but also has obvious anti-tumor effects in many species.
2.2.2 Autophagy
In addition to inducing apoptosis, DHA also has the ability to induce autophagy in tumors (22, 41, 69, 77, 81). When DHA damages tumor cells, autophagy is activated to clear damaged organelles, but excessive autophagy often leads to cell death. During autophagy, a series of ATG proteins is activated, and microtubule-associated protein 1 light chain 3-I (LC3-I) is transformed into LC3-II to form the membrane structure of autophagosome (24). In cholangiocarcinoma and hepatocellular carcinoma, DHA induces autophagy by inhibiting the Akt-mTOR signaling pathway (32, 63). Further, Tang et al. find that DHA up-regulates LC3-II/LC3-I ratio and induces autophagy in endometrial cancer and cervical cancer cells (38).
2.2.3 Ferroptosis
Ferroptosis is a type of programmed cell death different from apoptosis and autophagy. In ferroptosis, excessive intracellular free iron reacts with lipid peroxides and produces a large amount of ROS, which causes cell damage and death after depletion of intracellular glutathione (121). In osteosarcoma, Shen et al. demonstrate that DHA induces autophagy and mitochondrial injury by increasing the content of ROS, and the above effects are associated with iron (80). Furthermore, it has been found that DHA induces ferroptosis by increasing ROS level in tumor cells (53). Yuan et al. confirm that DHA induces ferroptosis in NSCLC cells by regulating SLC7A11 expression, accompanied by a decrease in intracellular GSH content and an increase in ROS and MDA (78). Du et al. find that DHA induces ferroptosis in leukemia cells by autophagy mediated degradation of ferritin and subsequent increases of intracellular free iron (71). However, Chen et al. use DHA to treat lung cancer cells, colon cancer cells, breast cancer cells and other tumor cells and observe that DHA can also induce ferroptosis in the above cells, but the mechanism is not related to autophagy (91). Interestingly, Bai and colleagues find that the rearrangement of DHA to monoketo-aldehyde-peroxyhemiacetal under physiological significantly enhance its response to iron and this physiological process is pH-dependent (92). The above studies suggest that DHA induces ferroptosis in many tumor cells mainly through promoting ROS production. However, the underlying mechanisms, such as the role of autophagy in DHA-induced ferroptosis, remain controversial and require further research.
2.3 Tumor Cell Cycle Arrest
Cell cycle progression requires interaction between cyclins and cyclin-dependent kinases (CDKs). In tumors, abnormal expression of cyclins and CDKs leads to dysfunction of cell cycle checkpoints and rapid tumor growth (36, 75). It has been shown that DHA blocks tumor cell cycle at G0/G1 or G2/M checkpoints by regulating the expression of cyclins and CDKs (42, 54, 74, 86). Zhao et al. find that combination treatment with DHA and curcumin have the effect of up-regulating the expression of miR-124 and blocking cell cycle at G0/G1 phase in ovarian carcinoma (56). Du et al. treat leukemia cells with DHA and observe that CDK2, CDK4 and Cyclin D expression are significantly inhibited and cell cycle is arrested at G0/G1 phase in the DHA treatment groups (71). Li et al. use ART or DHA treated ovarian carcinoma cells and find that DHA show a stronger anti-tumor effect and both ART and DHA block cell cycle at G2/M phase (83).
2.4 Enhance Anti-Tumor Immunity
In tumor microenvironment, CD8+ cytotoxic T lymphocytes (CTLs) play a major role in killing tumor cells. However, tumor cells and regulatory T cells (Tregs) suppress the killing effect of CTLs by secreting IL-10 and TGF-β, thus realizing tumor immune escape (122). It has been shown that DHA inhibits the production of TGF-β (23, 26–28) and TNF-α (49) in many tumors. Noori et al. observe that, in pancreatic cancer, DHA has the function of inhibiting Treg and increasing IFN-γ production in tumor microenvironment (87). In pancreatic cancer, Zhou et al. find that DHA enhances the activity of T cells and promotes the secretion of perforin, Granzyme B and IFN-γ (88). In our study, DHA exerts a significant modulation of anti-melanoma immunity. After DHA treatment, the levels of IL-6 and IL-10 in serum and melanoma of melanoma bearing mice are significantly decreased, while IFN-γ is significantly increased. Furthermore, DHA enhances the killing effect of CTLs to mouse melanoma by reducing IL-10 content and the number of Treg in tumor microenvironment (13). In melanoma lung metastasis models, DHA reduces the number of infiltrating Tregs in lung tissue and increases the infiltration and function of CTLs (Data not shown).
In addition to regulating the number and function of T cell subsets, DHA can also regulate other immune cells to promote anti-tumor immunity. Dendritic cells and macrophages are also involved in anti-tumor immunity. However, depending on their phenotypes, they can either inhibit or promote tumors (123, 124). Chen et al. confirm that DHA inhibits the differentiation of THP-1 cells to M2 macrophage and plays an anti-tumor role by inhibiting the phosphorylation of STAT3 (125). It has been demonstrated that DHA inhibits ovarian cancer metastasis by reducing the production of MMPs and the infiltration of macrophages in the metastatic sites of ovarian cancer (84). Furthermore, in colorectal tumor, DHA significantly strengthens anti-tumor immunity by enhancing the phagocytosis function of dendritic cells and macrophages and promoting the production of IFN-γ by T cells. The above effects are more obvious when DHA is combined with anti-PD-L1 mAb (33). Further, Zhang et al. confirm that in NSCLC, on the one hand, DHA reduces the expression of PD-L1 on tumor cells by inhibiting the expression of TGF-β and PI3K/Akt and STAT3 signaling pathways, thus impairs tumor immune escape. On the other hand, DHA increases the sensitivity of NSCLC to radiotherapy by regulating the expression of EMT-associated proteins (79). In summary, DHA promotes T-cell-centered anti-tumor immunity by reducing the secretion of immunosuppressive cytokines and suppressing the number and function of immunosuppressive cells.
2.5 Other Anti-Tumor Effects
The detection and analysis of abnormal expression of genes, proteins and metabolites in tumors are helpful to identify tumor pathogenesis and explore effective tumor treatment methods. Hepatocellular carcinoma MHCC97-L cells are treated with DHA for Global gene expression profiles analysis. The results show that DHA regulates gene expression which is associated with angiogenesis, apoptosis, cell cycle and various pathways (64). As a kind of non-coding RNA, Micro-RNA (miR) plays an important role in tumor by connecting to mRNA to regulate gene expression (72). Paccze et al. demonstrate that DHA inhibits tumor growth by up-regulation miR-7 and miR-34a in prostate cancer (90). In addition, Zhao et al. find that DHA binding curcumin up-regulate miR-124 and inhibit ovarian cancer (56). Hou et al. treat hepatocellular carcinoma cells MHCC97H and HCCLM3 with DHA and Sorafenib, and perform a proteomics analysis using Tandem Mass Tag peptide coupled with LC-MS/MS. Compared with the control group, there are 532, 426 and 628 differentially expressed proteins in the DHA group, Sorafenib group and DHA + Sorafenib group, respectively. And these differentially expressed proteins are mainly involved in cellular component organization, response to stress, and intracellular biochemical and metabolic reactions (58). Further, the metabonomic analysis of DHA in the treatment of esophageal cancer is performed. The results show that DHA down-regulates pyruvate kinase M2 and inhibits glycolysis, which is the main way for cancer cells getting energy (43). Zhu et al. demonstrate that DHA also inhibits glycolysis in prostate cancer (89). Liu et al. conduct a network pharmacologic analysis for the anti-hepatocellular carcinoma effect of DHA and find that DHA regulates the glycosylation and inhibits liver cancer by downregulating the expression of β1,6-branching of N-linked carbohydrate (65).
In summary, DHA has significant inhibitory effects on a variety of tumors in different species, and its pharmacological mechanisms are diverse, including inhibition of cell proliferation and metastasis, induction of autophagy and multiple programmed cell death, and arrest of cell cycle, etc. In addition, DHA exerts the strong effect in promoting anti-tumor immunity. DHA corrects the immunosuppression state of tumor-bearing mice by reducing the secretion of IL-10 and TGF-β and suppressing the number and function of Treg, and then activate the anti-tumor immune response mediated by T cells with CTL as the main body. In particular, DHA is not toxic to normal cells and is a potential tumor suppressive option (Figure 1).
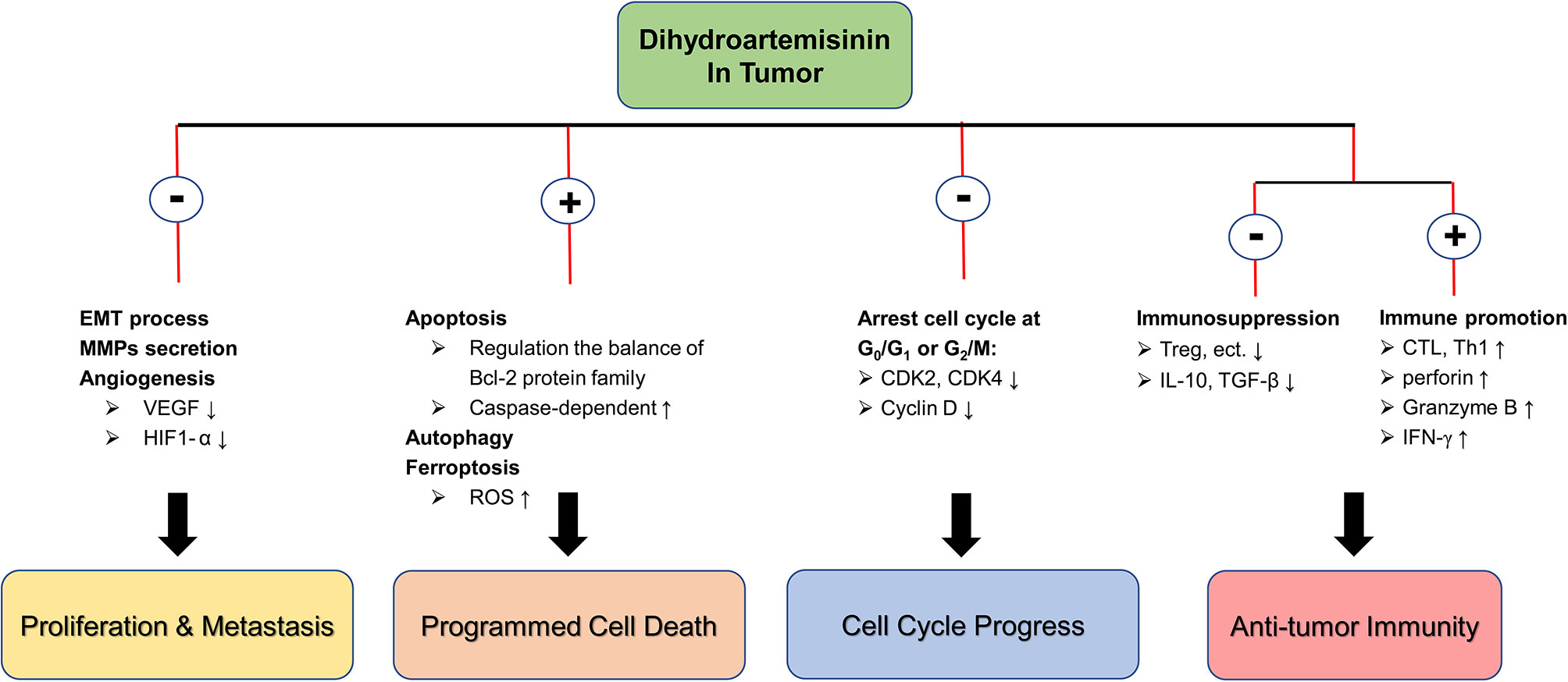
Figure 1 The molecular mechanism of DHA in anti-tumor. ⊕ means the promoting effect of DHA. ⊖ means the suppressive effect of DHA.
3 The Anti-Inflammation Activity of DHA
It has been shown that DHA regulates the immune function in the treatment of parasitic diseases and tumors (5, 6, 13, 33, 84, 87, 88, 125). In addition, it also has been reported that DHA significantly inhibits inflammatory diseases. As described by Xia et al. in IgA nephropathy mesangial cells (IgAN HMCs) induced by aggregated IgA1, DHA promotes autophagy of IgAN HMCs by down-regulating mTOR expression, and thus exerts an anti-proliferation effect (93). Further, as a potential anti-inflammatory drug, DHA plays a powerful role in inhibiting a variety of inflammation-related diseases. The potential pharmacological mechanisms of DHA anti-inflammation are described by following and list in Table 2.
3.1 Psoriasis
Psoriasis is a chronic inflammatory skin disease characterized by excessive proliferation of keratinocytes and excessive infiltration of white blood cells (e.g., neutrophils, T cells, etc.) into the dermis. As described by Wei et al., we find that DHA significantly alleviates imiquimod-induced psoriasis-like skin inflammation in mice and inhibits keratinocyte proliferation by regulating NF-κB and p38-MAPK signaling pathways (14). Furthermore, the content of IL-1β, IL-6 and IL-18 decrease significantly with the DHA treatment. In particular, the expression of CXCL-1 is markedly reduced, suggesting that DHA has a potential ability to inhibit neutrophil chemotaxis. In addition, our study also shows that DHA has a potent regulatory effect on a variety of immune cells, such as T cells, and pro-inflammatory cytokine production (94). The imbalanced proportion of T cell subsets leads to the skin immune system overactivation, which is considered to be closely related to psoriasis. Chen and colleagues demonstrate that DHA relieves mice psoriatic-like skin lesions and prevents psoriasis recurrence (95). Specifically, DHA treatment diminishes the number of pathogenic CD8+ central memory T cells and CD8+ resident T cells in the skin and reduces the content of pro-inflammatory cytokines such as IL-17. Further, the expression of eomesodermin and BCL-6 in CD8+ T cells is also down-regulated with DHA treatment.
3.2 Experimental Autoimmune Encephalomyelitis (EAE)
EAE is an autoimmune disease mediated by specific CD4+ T cells, characterized by mononuclear cell infiltration into the central nervous system and demyelination of white matter (126). Zhao et al. treat EAE mice with DHA and find that the symptoms are significantly improved and disease scores decrease markedly. The mechanism is that DHA increases the number of Treg in the spinal cord, spleen, inguinal lymph nodes and peripheral lymph nodes, promotes the differentiation of Treg and the production of TGF-β and reduces Th cell infiltration through inhibiting mTOR signaling pathway (96). In addition, DHA alleviates LPS-induced neuroinflammation by inhibiting PI3K/Akt signaling pathway and reducing the production of IL-1β, IL-6 and TNF-α (97).
3.3 Inflammatory Bowel Disease (IBD)
It has been reported that the pathogenesis of IBD is closely related to the over-activation of several signaling pathways (such as NF-κB signaling pathway and p38-MAPK signaling pathway), the production of pro-inflammatory cytokines and abnormal intestinal mucosal immune system (127). Li et al. find that DHA alleviates IBD in mice by inhibiting PI3K/Akt and NF-κB signaling pathways and reducing the production of TNF-α, IL-1β and IL-6 (98). It has been found that NLRP3 inflammasome, which acts as a bridge between some inflammation-related signaling pathways and pro-inflammatory cytokines, is often overexpressed in IBD. Liang et al. find that in IBD mice, DHA inhibits the phosphorylation of p65 and p38, down-regulates the expression of NLRP3 inflammasome, and thereby reduces the contents of IL-1β, IL-6 and TNF-α (99). Further to explore the regulatory effect of DHA on immune cells, Yan and colleagues demonstrate that DHA induces T cell apoptosis via HO-1, thus reducing the number of Th1, Th9 and Th17 cells, restoring the balance between Th cells and Treg, and improving IBD symptoms (100). In addition to reducing inflammatory cell infiltration and pro-inflammatory cytokine production in the gut, DHA also improves IBD by regulating intestinal flora. Lei et al. use 16S rDNA gene analysis and find that DHA restores the abundance of Bacteroidetes, Verrucomicrobia, Firmicutes and Proteobacteria, which are abnormal in mouse IBD (101). Interestingly, DHA exerts a significant inhibitory effect on IBD-induced bone loss by reducing osteoclast formation and increasing bone mineral density (102). The inhibitory effect of DHA on osteoclasts is not limited to IBD. In breast cancer (128) and other diseases (129, 130), DHA inhibits the formation of osteoclasts and relieves bone loss, suggesting that DHA seems to have a relieving effect on bone loss caused by a variety of diseases. DHA is expected to become an alternative therapeutic drug for the treatment of osteolytic bone disease.
3.4 Rheumatoid Arthritis (RA)
RA is an autoimmune disease characterized by extensive production of autoantibodies and severe damage of joints (131). Fan et al. use DC32, a derivative of DHA, to treat collagen-induced arthritis mouse, and find that after DHA treatment, the content of rheumatoid factor significantly decreases, cartilage damage is alleviated and arthritis is improved. Its mechanism is that on the one hand DC32 activates nuclear factor (erythroid-derived 2)-like 2 (Nrf-2)/HO-1 antioxidant pathway and on the other hand DC32 reduces IL-6 transcription and restores Treg/Th17 cells balance (103, 104). In addition, Li et al. demonstrate that DC32 reduces the expression of IL-6, IL-1β, TNF-α, CXCL12 and CX3CL1 by activating Nrf-2/HO-1 signaling pathway and inhibiting the phosphorylation of ERK and p65, thus improving Osteoarthritic Synovium (105).
3.5 Systemic Lupus Erythematosus (SLE)
SLE is a chronic autoimmune disease characterized by the production of autoantibodies and the involvement of multiple organs (132). Following the discovery of DHA in malaria, Tu Youyou’s team finds that DHA has a significant inhibitory effect on SLE. In BXSB mice, DHA significantly reduces the production of TNF-α by inhibiting the expression of p65 in peritoneal macrophages and renal tissue (106). In addition, Huang et al. confirm that DHA inhibits Toll-like receptor 4 (TLR4) and reduces IRF3 and type I interferon (IFN-α and IFN-β) production, thus alleviates SLE (107). Furthermore, Li et al. find that DHA delays the progression of SLE by inhibiting the senescence of myeloid-derived suppressor cells. In addition, DHA restores Treg/Th17 cells balance and reduces serum IL-1β, IL-6 and IL-8 levels in lupus mice (108).
Lupus nephritis is a complication of SLE and often leads patients to death. Diao et al. use LPS stimulation glomerular mesangial cell line MMC to build cell model of lupus nephritis and give DHA treatment. After DHA treatment, the expression of HMGB1, one of the genes closely associated with lupus nephritis, is significantly reduced. In addition, DHA attenuates the expression of TLR4 and p65 and reduces IL-1β, IL-8, IL-6 and TNF-α production in LPS-induced RAW264.7 cells (109). In LPS-induced acute renal injury, DHA therapy reduces the serum Scr and BUN levels and restores renal function. The mechanism is that DHA reduces the expression of IL-1β, IL-5, IL-6, IL-17A, TNF-α, IFN-γ, CXCL-1 and improves oxidative stress through inhibiting the phosphorylation of p65 (110).
3.6 Allergic Asthma
Allergic Asthma is a chronic airway allergic disease. It has been reported that Th2 cells, Th17 cells, mast cells and granulocytes are all involved in the pathogenesis of Asthma (133). Wei et al. find that DHA significantly reduces inflammatory cell infiltration and alleviates asthma-related airway hyper-responsiveness in ovalbumin (OVA)-induced mouse asthma. The mechanism is that DHA inhibits the activation of ERK, p38 and NF-κB and reduces the OVA-specific Ig E and Th2 cytokines secretion (111). In addition to the above effects, Zhu et al. demonstrate that DHA also relieves asthma by reducing the number of Th17 cells and the production of IL-17, IL-21, IL-22, IL-1β, TNF-α and GM-CSF (112). Furthermore, Ravindra et al. use Untargeted Proteomics to analyze the targets of DHA treatment human bronchial epithelial cells and find that DHA promotes the expression of forkhead box protein O1 (FOXO1), Nrf-2, serum response factor (SRF), STAT3 and Smad (113). For other lung injury diseases, DHA reduces the release of IL-1β, IL-6 and TNF-α by inhibiting the phosphorylation of p65 and activating Nrf-2 in LPS-induced acute lung injury. In addition, DHA also reduces the infiltration of macrophages and neutrophils into lung tissue to alleviate lung injury (114).
In inflammatory diseases, an unbalanced immune system forms a complex inflammatory immune network. The insufficiency of Treg cell function leads to the continuous development of inflammation with Th1/Th17 cells as the core. The release of pro-inflammatory cytokines producing by Th1/Th17 cells further inhibits the function of Treg. It forms a closed-loop structure of positive feedback, which leads to the continuous development of inflammatory disease and prolonged healing. DHA and its derivative DC32 have shown alleviative effects in multiple inflammatory diseases by regulating the signaling pathways (inhibiting pro-inflammatory signaling pathways and activating antioxidant signaling pathways), inhibiting pro-inflammatory cytokine production (such as TNF-α, IL-1β, etc.), and restoring immune cell balance. In addition to the aforementioned effect, in autoimmune diseases, DHA may also relieve symptoms and inhibit progression by reducing the production of autoantibodies. In laboratory studies, researchers have tentatively demonstrated the potential of DHA as an alternative treatment for inflammatory diseases, and further exploration of the underlying mechanisms of DHA inhibiting inflammation and the clinical use of DHA in treating inflammation needs to be performed (Figure 2).
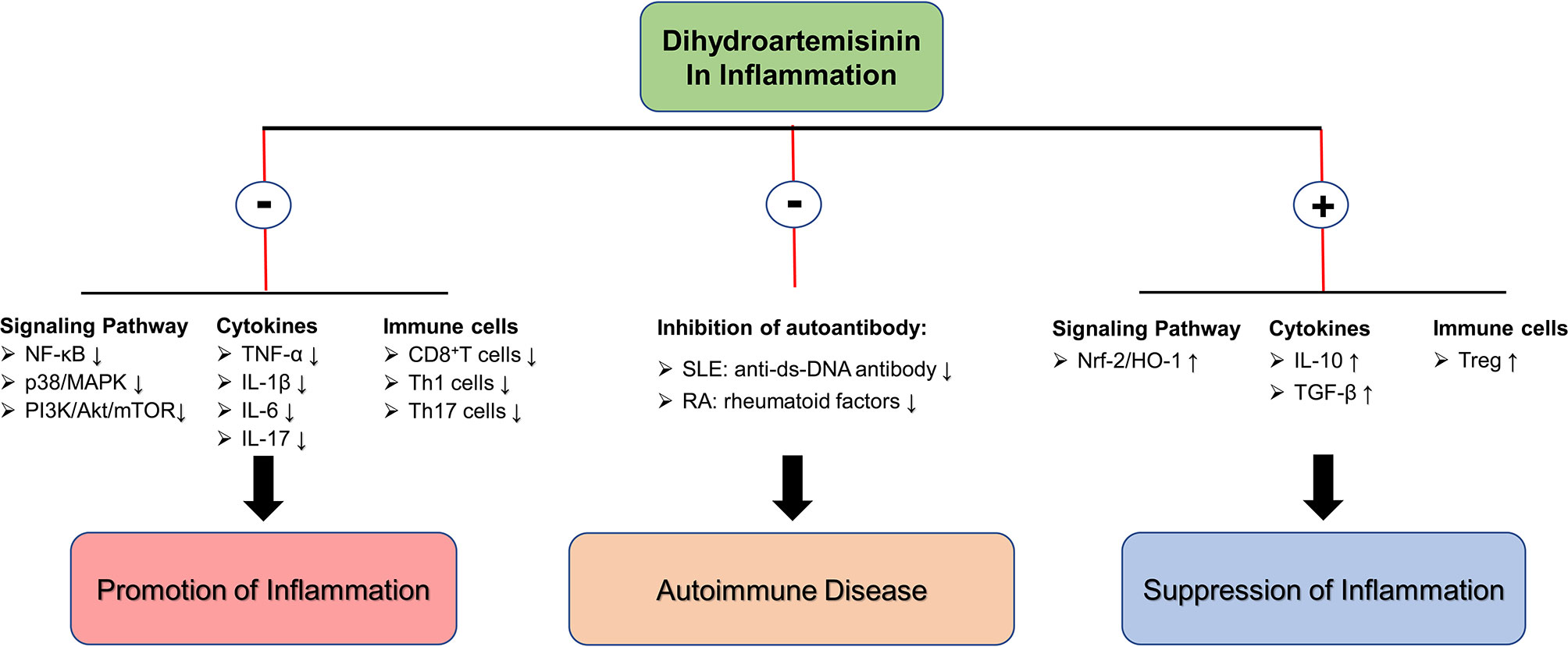
Figure 2 The molecular mechanism of DHA in inflammation inhibition. ⊕ means the promoting effect of DHA. ⊖ means the suppressive effect of DHA.
4 Summary and Future Outlook
With the development of anti-tumor and inhibition of inflammation effects of DHA, in recent years, more and more studies have focused on how to improve the efficacy of DHA. The main strategies to enhance the efficacy of DHA include modifying the chemical structure of DHA (55), developing new DHA derivatives (134), building multimeric DHA conjugates (135), and coupling other drugs with DHA to achieve targeted tumor therapy (37, 59, 136–138). Liu et al. construct the R8 modified epirubicin-dihydroartemisinin liposomes for the treatment of NSCLC, and find R8 modified epirubicin-dihydroartemisinin liposomes have a stronger killing effect on A549 cells. R8 modified epirubicin-dihydroartemisinin liposomes attenuate the metastasis of A549 cells by inhibiting the production of TGF-β, MMP2 and HIF-1α. In vivo, R8 modified epirubicin-dihydroartemisinin liposomes accumulate in tumor and show the targeted functions, indicating that it is an effective treatment method for NSCLC (139).
Moreover, DHA and artemisinin-based drugs are also increasingly reported in clinical oncology trials. For clinical treatment, DHA is widely used in the treatment of malaria which provides a solid and effective basis for its safety. In laboratory studies, appropriate concentration of DHA has low toxicity to normal cells, but some studies have reported that even though DHA is a high safety drug, excessively high concentration of DHA (≥100μmol/L) can still cause damage to normal cells (140, 141), which also suggests that the clinical concentration of DHA should not be too high. And, when using large doses of DHA, clinician should be aware of its toxic effects. Jansen and colleagues treat advanced cervical cancer patients with Artenimol-R, hemi-succinate ester of DHA, for 28 days and find that Artenimol-R significantly alleviates cervical cancer and has no toxic effects. The mechanism of Artenimol-R improves cervical cancer is that Artenimol-R treatment results in significant reductions of p53, epidermal growth factor receptor (EGFR), Ki-67 (a marker of tumor proliferation) and angiogenesis (142). Deeken et al. recruit 19 patients with refractory solid tumors for a phase I clinical trial of ART and find that the maximum tolerated dose (MTD) of intravenous ART administration is 18mg/kg with a disease control rate of 27% (143). The above clinical studies provide the basis for the efficacy and safety of DHA in the treatment of malignant tumors.
In conclusion, DHA has demonstrated significant anti-tumor and inhibition of inflammation effects and is a promising drug with a wide range of targets. In recent years, studies on the genomics (64), proteomics (72, 113), metabolomics (58, 90) and network pharmacology (43) of DHA therapy have provided a basis for elucidating the specific pharmacological mechanism and targets of DHA, but there are few clinical studies on DHA. Further phase II and III clinical trials are required for DHA. To address the limitations and challenges of DHA in experimental research and clinical application, we consider that the mechanisms of DHA anti-tumor and inflammation inhibition need to be deeply elucidated, for example to explore the genetic or protein targets of DHA. Further, clinical trials of DHA as a therapeutic agent for tumor and inflammatory diseases need to be expanded. Although there have been few reports of serious adverse reactions to DHA, the safety of DHA in clinical use still needs to be monitored by clinicians. With the development of research on the pharmacological effects of DHA, it is certain that DHA will one day become an alternative treatment for tumors and inflammatory diseases.
Author Contributions
RY: conceptualization, writing—original draft preparation and project administration. GJ: supervision, funding acquisition and writing—review and editing. MF: supervision and writing—review and editing. All authors contributed to the article and approved the submitted version.
Funding
This study was sponsored by National Natural Science Foundation of China (No. 81960305, 61671098).
Conflict of Interest
The authors declare that the research was conducted in the absence of any commercial or financial relationships that could be construed as a potential conflict of interest.
Publisher’s Note
All claims expressed in this article are solely those of the authors and do not necessarily represent those of their affiliated organizations, or those of the publisher, the editors and the reviewers. Any product that may be evaluated in this article, or claim that may be made by its manufacturer, is not guaranteed or endorsed by the publisher.
References
1. Wang D, Zhong B, Li Y, Liu X. Dihydroartemisinin Increases Apoptosis of Colon Cancer Cells Through Targeting Janus Kinase 2/Signal Transducer and Activator of Transcription 3 Signaling. Oncol Leff (2018) 15(2):1949–54. doi: 10.3892/ol.2017.7502
2. Liu Y, Gao S, Zhu J, Zheng Y, Zhang H, Sun H. Dihydroartemisinin Induces Apoptosis and Inhibits Proliferation, Migration, and Invasion in Epithelial Ovarian Cancer via Inhibition of the Hedgehog Signaling Pathway. Cancer Med (2018) 7(11):5704–15. doi: 10.1002/cam4.1827
3. Meshnick SR, Thomas A, Ranz A, Xu CM, Pan HZ. Artemisinin (Qinghaosu): The Role of Intracellular Hemin in Its Mechanism of Antimalarial Action. Mol Biochem Parasitol (1991) 49(2):181–9. doi: 10.1016/0166-6851(91)90062-b
4. Zhan S, Chen H, Gerhard GS. Heme Synthesis Increases Artemisinin-Induced Radical Formation and Cytotoxicity That Can Be Suppressed by Superoxide Scavengers. Chem Biol Interact (2010) 186(1):30–5. doi: 10.1016/j.cbi.2010.03.021
5. Jagannathan P, Bowen K, Nankya F, McIntyre TI, Auma A, Wamala S, et al. Effective Antimalarial Chemoprevention in Childhood Enhances the Quality of CD4+ T Cells and Limits Their Production of Immunoregulatory Interleukin 10. J Infect Dis (2016) 214(2):329–38. doi: 10.1093/infdis/jiw147
6. Zhang T, Zhang Y, Jiang N, Zhao X, Sang X, Yang N, et al. Dihydroartemisinin Regulates the Immune System by Promotion of CD8+ T Lymphocytes and Suppression of B Cell Responses. Sci China Life Sci (2020) 63(5):737–49. doi: 10.1007/s11427-019-9550-4
7. Zhang T, Hu Y, Wang T, Cai P. Dihydroartemisinin Inhibits the Viability of Cervical Cancer Cells by Upregulating Caveolin 1 and Mitochondrial Carrier Homolog 2: Involvement of P53 Activation and NAD(P)H: Quinone Oxidoreductase 1 Downregulation. Int J Mol Med (2017) 40(1):21–30. doi: 10.3892/ijmm.2017.2980
8. Jiang C, Li S, Li Y, Bai Y. Anticancer Effects of Dihydroartemisinin on Human Esophageal Cancer Cells In Vivo. Anal Cell Pathol (Amst) (2018) 2018:8759745. doi: 10.1155/2018/8759745
9. Yang S, Zhang D, Shen N, Wang G, Tang Z, Chen X. Dihydroartemisinin Increases Gemcitabine Therapeutic Efficacy in Ovarian Cancer by Inducing Reactive Oxygen Species. J Cell Biochem (2019) 120(1):634–44. doi: 10.1002/jcb.27421
10. He J, Neumann D, Kakazu A, Pham TL, Musarrat F, Cortina MS, et al. PEDF Plus DHA Modulate Inflammation and Stimulate Nerve Regeneration After HSV-1 Infection. Exp Eye Res (2017) 161(1):153–62. doi: 10.1016/j.exer.2017.06.015
11. Zhou C, Tang X, Xu J, Wang J, Yang Y, Chen Y, et al. Opening of the CLC-3 Chloride Channel Induced by Dihydroartemisinin Contributed to Early Apoptotic Events in Human Poorly Differentiated Nasopharyngeal Carcinoma Cells. J Cell Biochem (2018) 119(11):9560–72. doi: 10.1002/jcb.27274
12. Chaib I, Cai X, Llige D, Santarpia M, Jantus-Lewintre E, Filipska M, et al. Osimertinib and Dihydroartemisinin: A Novel Drug Combination Targeting Head and Neck Squamous Cell Carcinoma. Ann Transl Med (2019) 7(22):651. doi: 10.21037/atm.2019.10.80
13. Yu R, Jin L, Li F, Fujimoto M, Wei Q, Lin Z, et al. Dihydroartemisinin Inhibits Melanoma by Regulating CTL/Treg Anti-Tumor Immunity and STAT3-Mediated Apoptosis via IL-10 Dependent Manner. J Dermatol Sci (2020) 99(3):193–202. doi: 10.1016/j.jdermsci.2020.08.001
14. Wei Q, Jin Q, Jin L, Yu R, Li F, Li H, et al. Dihydroartemisinin Alleviates Psoriasis-Like Skin Inflammation in Mice by Inhibiting Proliferation of Keratinocytes and Expression of Pro-Inflammatory Cytokines. Chin J Immunol (2020) 36(5):543–8. doi: 10.3969/j.issn.1000-484X.2020.05.006
15. Ho WE, Peh HY, Chan TK, Wong WSF. Artemisinins: Pharmacological Actions Beyond Anti-Malarial. Pharmacol Ther (2014) 142(1):126–39. doi: 10.1016/j.pharmthera.2013.12.001
16. Efferth T. From Ancient Herb to Modern Drug: Artemisia Annua and Artemisinin for Cancer Therapy. Semin Cancer Biol (2017) 46(1):65–83. doi: 10.1016/j.semcancer.2017.02.009
17. Slezakova S, Ruda-Kucerova J. Anticancer Activity of Artemisinin and Its Derivatives. Anticancer Res (2017) 37(11):5995–6003. doi: 10.21873/anticanres.12046
18. D’Amico S, Krasnowska EK, Manni I, Toietta G, Baldari S, Piaggio G, et al. DHA Affects Microtubule Dynamics Through Reduction of Phospho-TCTP Levels and Enhances the Antiproliferative Effect of T-DM1 in Trastuzumab-Resistant HER2-Positive Breast Cancer Cell Lines. Cells (2020) 9(5):1260. doi: 10.3390/cells9051260
19. Poupel F, Aghaei M, Movahedian A, Jafari SM, Keyvanloo Shahrestanaki M. Dihydroartemisinin Induces Apoptosis in Human Bladder Cancer Cell Lines Through Reactive Oxygen Species, Mitochondrial Membrane Potential, and Cytochrome C Pathway. Int J Prev Med (2017) 8:78. doi: 10.4103/ijpvm.IJPVM_258_17
20. Zhou X, Zijlstra SN, Soto-Gamez A, Setroikromo R, Quax WJ. Artemisinin Derivatives Stimulate DR5-Specific TRAIL-Induced Apoptosis by Regulating Wildtype P53. Cancers (Basel) (2020) 12(9):2514. doi: 10.3390/cancers12092514
21. Jin T, Diao L, Chen F, Shen A, Wang S, Jin H, et al. pH-Sensitive Nanoparticles Codelivering Docetaxel and Dihydroartemisinin Effectively Treat Breast Cancer by Enhancing Reactive Oxidative Species-Mediated Mitochondrial Apoptosis. Mol Pharm (2021) 18(1):74–86. doi: 10.1021/acs.molpharmaceut.0c00432
22. Hu Y-J, Zhang J-Y, Luo Q, Xu J-R, Yan Y, Mu L-M, et al. Nanostructured Dihydroartemisinin Plus Epirubicin Liposomes Enhance Treatment Efficacy of Breast Cancer by Inducing Autophagy and Apoptosis. Nanomater (Basel) (2018) 8(10):804. doi: 10.3390/nano8100804
23. Ju R-J, Cheng L, Peng X-M, Wang T, Li C-Q, Song X-L, et al. Octreotide-Modified Liposomes Containing Daunorubicin and Dihydroartemisinin for Treatment of Invasive Breast Cancer. Artif Cells Nanomed Biotechnol (2018) 46(sup1):616–28. doi: 10.1080/21691401.2018.1433187
24. Liu Q, Zhou X, Li C, Zhang X, Li CL. Rapamycin Promotes the Anticancer Action of Dihydroartemisinin in Breast Cancer MDA-MB-231 Cells by Regulating Expression of Atg7 and DAPK. Oncol Lett (2018) 15(4):5781–6. doi: 10.3892/ol.2018.8013
25. Guo S, Yao X, Jiang Q, Wang K, Zhang Y, Peng H, et al. Dihydroartemisinin-Loaded Magnetic Nanoparticles for Enhanced Chemodynamic Therapy. Front Pharmacol (2020) 11:226. doi: 10.3389/fphar.2020.00226
26. Yao Y, Guo Q, Cao Y, Qiu Y, Tan R, Yu Z, et al. Artemisinin Derivatives Inactivate Cancer-Associated Fibroblasts Through Suppressing TGF-β Signaling in Breast Cancer. J Exp Clin Cancer Res (2018) 37(1):282. doi: 10.1186/s13046-018-0960-7
27. Li Y, Zhou X, Liu J, Gao N, Yang R, Wang Q, et al. Dihydroartemisinin Inhibits the Tumorigenesis and Metastasis of Breast Cancer via Downregulating CIZ1 Expression Associated With TGF-β1 Signaling. Life Sci (2020) 248:117454. doi: 10.1016/j.lfs.2020.117454
28. Dong J, Yang W, Han J, Cheng R, Guo X, Li L. Effect of Dihydroartemisinin on Epithelial-To-Mesenchymal Transition in Canine Mammary Tumour Cells. Res Vet Sci (2019) 124(1):240–7. doi: 10.1016/j.rvsc.2019.03.020
29. Li Z, Teng M, Wang Y, Feng Y, Xiao Z, Hu H, et al. Dihydroartemisinin Administration Improves the Effectiveness of 5-Aminolevulinic Acid-Mediated Photodynamic Therapy for the Treatment of High-Risk Human Papillomavirus Infection. Photodiag Photodyn Ther (2021) 33:102078. doi: 10.1016/j.pdpdt.2020.102078
30. Wang >L, Li J, Shi X, Li S, Tang PM-K, Li Z, et al. Antimalarial Dihydroartemisinin Triggers Autophagy Within HeLa Cells of Human Cervical Cancer Through Bcl-2 Phosphorylation at Ser70. Phytomedicine (2019) 52(1):147–56. doi: 10.1016/j.phymed.2018.09.221
31. Hu H, Wang Z, Tan C, Liu X, Zhang H, Li K. Dihydroartemisinin/miR-29b Combination Therapy Increases the Pro-Apoptotic Effect of Dihydroartemisinin on Cholangiocarcinoma Cell Lines by Regulating Mcl-1 Expression. Adv Clin Exp Med (2020) 29(8):911–9. doi: 10.17219/acem/121919
32. Thongchot S, Vidoni C, Ferraresi A, Loilome W, Yongvanit P, Namwat N, et al. Dihydroartemisinin Induces Apoptosis and Autophagy-Dependent Cell Death in Cholangiocarcinoma Through a DAPK1-BECLIN1 Pathway. Mol Carcinog (2018) 57(12):1735–50. doi: 10.1002/mc.22893
33. Duan X, Chan C, Han W, Guo N, Weichselbaum RR, Lin W. Immunostimulatory Nanomedicines Synergize With Checkpoint Blockade Immunotherapy to Eradicate Colorectal Tumors. Nat Commun (2019) 10(1):1899. doi: 10.1038/s41467-019-09221-x
34. Kang X-J, Wang H-Y, Peng H-G, Chen B-F, Zhang W-Y, Wu A-H, et al. Codelivery of Dihydroartemisinin and Doxorubicin in Mannosylated Liposomes for Drug-Resistant Colon Cancer Therapy. Acta Pharmacol Sin (2017) 38(6):885–96. doi: 10.1038/aps.2017.10
35. Yao Z, Bhandari A, Wang Y, Pan Y, Yang F, Chen R, et al. Dihydroartemisinin Potentiates Antitumor Activity of 5-Fluorouracil Against a Resistant Colorectal Cancer Cell Line. Biochem Biophys Res Commun (2018) 501(3):636–42. doi: 10.1016/j.bbrc.2018.05.026
36. Fan H-N, Zhu M-Y, Peng S-Q, Zhu J-S, Zhang J, Qu G-Q. Dihydroartemisinin Inhibits the Growth and Invasion of Gastric Cancer Cells by Regulating Cyclin D1-CDK4-Rb Signaling. Pathol Res Pract (2020) 216(2):152795. doi: 10.1016/j.prp.2019.152795
37. Yu H, Hou Z, Tian Y, Mou Y, Guo C. Design, Synthesis, Cytotoxicity and Mechanism of Novel Dihydroartemisinin-Coumarin Hybrids as Potential Anti-Cancer Agents. Eur J Med Chem (2018) 151(1):434–49. doi: 10.1016/j.ejmech.2018.04.005
38. Tang T, Xia Q, Xi M. Dihydroartemisinin and Its Anticancer Activity Against Endometrial Carcinoma and Cervical Cancer: Involvement of Apoptosis, Autophagy and Transferrin Receptor. Singapore Med J (2021) 62(2):96–103. doi: 10.11622/smedj.2019138
39. Cui W, Fang T, Duan Z, Xiang D, Wang Y, Zhang M, et al. Dihydroartemisinin Sensitizes Esophageal Squamous Cell Carcinoma to Cisplatin by Inhibiting Sonic Hedgehog Signaling. Front Cell Dev Biol (2020) 8:596788. doi: 10.3389/fcell.2020.596788
40. Li Y, Sui H, Jiang C, Li S, Han Y, Huang P, et al. Dihydroartemisinin Increases the Sensitivity of Photodynamic Therapy Via NF-κb/HIF-1α/VEGF Pathway in Esophageal Cancer Cell n Vitro and in Vivo. Cell Physiol Biochem (2018) 48(5):2035–45. doi: 10.1159/000492541
41. Chen X, He L-Y, Lai S, He Y. Dihydroartemisinin Inhibits the Migration of Esophageal Cancer Cells by Inducing Autophagy. Oncol Lett (2020) 20(4):94. doi: 10.3892/ol.2020.11955
42. Ma Q, Liao H, Xu L, Li Q, Zou J, Sun R, et al. Autophagy-Dependent Cell Cycle Arrest in Esophageal Cancer Cells Exposed to Dihydroartemisinin. Chin Med (2020) 15:37. doi: 10.1186/s13020-020-00318-w
43. Li S, Huang P, Gan J, Ling X, Du X, Liao Y, et al. Dihydroartemisinin Represses Esophageal Cancer Glycolysis by Down-Regulating Pyruvate Kinase M2. Eur J Pharmacol (2019) 854(1):232–9. doi: 10.1016/j.ejphar.2019.04.018
44. Zhang F, Ma Q, Xu Z, Liang H, Li H, Ye Y, et al. Dihydroartemisinin Inhibits TCTP-Dependent Metastasis in Gallbladder Cancer. J Exp Clin Cancer Res (2017) 36(1):68. doi: 10.1186/s13046-017-0531-3
45. Luo Q, Zhang S, Zhang D, Feng R, Li N, Chen W, et al. Effects and Mechanisms of Anlotinib and Dihydroartemisinin Combination Therapy in Ameliorating Malignant Biological Behavior of Gastric Cancer Cells. Curr Pharm Biotechnol (2021) 22(4):523–33. doi: 10.2174/1389201021666200623132803
46. Li N, Zhang S, Luo Q, Yuan F, Feng R, Chen X, et al. The Effect of Dihydroartemisinin on the Malignancy and Epithelial-Mesenchymal Transition of Gastric Cancer Cells. Curr Pharm Biotechnol (2019) 20(9):719–26. doi: 10.2174/1389201020666190611124644
47. Liang R, Chen W, Chen X-Y, Fan H-N, Zhang J, Zhu J-S. Dihydroartemisinin Inhibits the Tumorigenesis and Invasion of Gastric Cancer by Regulating STAT1/KDR/MMP9 and P53/BCL2L1/CASP3/7 Pathways. Pathol Res Pract (2020) 218:153318. doi: 10.1016/j.prp.2020.153318
48. Zhang S, Shi L, Ma H, Li H, Li Y, Lu Y, et al. Dihydroartemisinin Induces Apoptosis in Human Gastric Cancer Cell Line BGC-823 Through Activation of JNK1/2 and P38 MAPK Signaling Pathways. J Recept Signal Transduct Res (2017) 37(2):174–80. doi: 10.1080/10799893.2016.1203942
49. Su T, Li F, Guan J, Liu L, Huang P, Wang Y, et al. Artemisinin and Its Derivatives Prevent Helicobacter Pylori-Induced Gastric Carcinogenesis via Inhibition of NF-κb Signaling. Phytomedicine (2019) 63:152968. doi: 10.1016/j.phymed.2019.152968
50. Que Z, Wang P, Hu Y, Xue Y, Liu X, Qu C, et al. Dihydroartemisin Inhibits Glioma Invasiveness via a ROS to P53 to β-Catenin Signaling. Pharmacol Res (2017) 119(1):72–88. doi: 10.1016/j.phrs.2017.01.014
51. Shao Y-Y, Zhang T-L, Wu L-X, Zou H-C, Li S, Huang J, et al. AKT Axis, miR-21, and RECK Play Pivotal Roles in Dihydroartemisinin Killing Malignant Glioma Cells. Int J Mol Sci (2017) 18(2):350. doi: 10.3390/ijms18020350
52. Qu C, Ma J, Liu X, Xue Y, Zheng J, Liu L, et al. Dihydroartemisinin Exerts Anti-Tumor Activity by Inducing Mitochondrion and Endoplasmic Reticulum Apoptosis and Autophagic Cell Death in Human Glioblastoma Cells. Front Cell Neurosci (2017) 11:310. doi: 10.3389/fncel.2017.00310
53. Chen Y, Mi Y, Zhang X, Ma Q, Song Y, Zhang L, et al. Dihydroartemisinin-Induced Unfolded Protein Response Feedback Attenuates Ferroptosis via PERK/ATF4/HSPA5 Pathway in Glioma Cells. J Exp Clin Cancer Res (2019) 38(1):402. doi: 10.1186/s13046-019-1413-7
54. Xu C-H, Liu Y, Xiao L-M, Guo C-G, Zheng S-Y, Zeng E-M, et al. Dihydroartemisinin Treatment Exhibits Antitumor Effects in Glioma Cells Through Induction of Apoptosis. Mol Med Rep (2017) 16(6):9528–32. doi: 10.3892/mmr.2017.7832
55. Li H, Li X, Shi X, Li Z, Sun Y. Effects of Magnetic Dihydroartemisinin Nano-Liposome in Inhibiting the Proliferation of Head and Neck Squamous Cell Carcinomas. Phytomedcine (2019) 56(1):215–28. doi: 10.1016/j.phymed.2018.11.007
56. Zhao J, Pan Y, Li X, Zhang X, Xue Y, Wang T, et al. Dihydroartemisinin and Curcumin Synergistically Induce Apoptosis in SKOV3 Cells Via Upregulation of MiR-124 Targeting Midkine. Cell Physiol Biochem (2017) 43(2):589–601. doi: 10.1159/000480531
57. Gao J, Ma F, Wang X, Li G. Combination of Dihydroartemisinin and Resveratrol Effectively Inhibits Cancer Cell Migration via Regulation of the DLC1/TCTP/Cdc42 Pathway. Food Funct (2020) 11(11):9573–84. doi: 10.1039/d0fo00996b
58. Hou C, Guo D, Yu X, Wang S, Liu T. TMT-Based Proteomics Analysis of the Anti-Hepatocellular Carcinoma Effect of Combined Dihydroartemisinin and Sorafenib. BioMed Pharmacother (2020) 126:109862. doi: 10.1016/j.biopha.2020.109862
59. Kumar R, Singh M, Meena J, Singhvi P, Thiyagarajan D, Saneja A, et al. Hyaluronic Acid - Dihydroartemisinin Conjugate: Synthesis, Characterization and in Vitro Evaluation in Lung Cancer Cells. Int J Biol Macromol (2019) 133(1):495–502. doi: 10.1016/j.ijbiomac.2019.04.124
60. Li C, Gao S, Yang W-S, Jin G-Z, Sun S. β-Dihydroartemisinin-Emodin Promotes Apoptosis by Activating Extrinsic and Intrinsic Pathways in Human Liver Cancer Cells. Ann Clin Lab Sci (2019) 49(3):281–90.
61. Shi X, Wang L, Ren L, Li J, Li S, Cui Q, et al. Dihydroartemisinin, an Antimalarial Drug, Induces Absent in Melanoma 2 Inflammasome Activation and Autophagy in Human Hepatocellular Carcinoma HepG2215 Cells. Phytother Res (2019) 33(5):1413–25. doi: 10.1002/ptr.6332
62. Im E, Yeo C, Lee H-J, Lee E-O. Dihydroartemisinin Induced Caspase-Dependent Apoptosis Through Inhibiting the Specificity Protein 1 Pathway in Hepatocellular Carcinoma SK-Hep-1 Cells. Life Sci (2018) 192(1):286–92. doi: 10.1016/j.lfs.2017.11.008
63. Zou J, Ma Q, Sun R, Cai J, Liao H, Xu L, et al. Dihydroartemisinin Inhibits HepG2.2.15 Proliferation by Inducing Cellular Senescence and Autophagy. BMB Rep (2019) 52(8):520–4. doi: 10.5483/BMBRep.2019.52.8.058
64. Wu L, Cheng Y, Deng J, Tao W, Ye J. Dihydroartemisinin Inhibits Proliferation and Induces Apoptosis of Human Hepatocellular Carcinoma Cell by Upregulating Tumor Necrosis Factor via JNK/NF- κ B Pathways. Evid Based Complement Alternat Med (2019) 2019:9581327. doi: 10.1155/2019/9581327
65. Liu T, Guo J, Wang T, Zhang S, Yu X, Hou C, et al. Network Pharmacology-Based Analysis of Mechanisms of the Anti-Hepatocellular Carcinoma Effect by Dihydroartemisinin. Discov Med (2019) 28(153):139–47.
66. Liu J, Ren Y, Hou Y, Zhang C, Wang B, Li X, et al. Dihydroartemisinin Induces Endothelial Cell Autophagy Through Suppression of the Akt/mTOR Pathway. J Cancer (2019) 10(24):6057–64. doi: 10.7150/jca.33704
67. Niu N, Yu C, Li L, Liu Q, Zhang W, Liang K, et al. Dihydroartemisinin Enhances VEGFR1 Expression Through Up-Regulation of ETS-1 Transcription Factor. J Cancer (2018) 9(18):3366–72. doi: 10.7150/jca.25082
68. Wang W, Sun Y, Li X, Shi X, Li Z, Lu X. Dihydroartemisinin Prevents Distant Metastasis of Laryngeal Carcinoma by Inactivating STAT3 in Cancer Stem Cells. Med Sci Monit (2020) 26:e922348. doi: 10.12659/MSM.922348
69. Shi X, Li S, Wang L, Li H, Li Z, Wang W, et al. RalB Degradation by Dihydroartemisinin Induces Autophagy and IFI16/caspase-1 Inflammasome Depression in the Human Laryngeal Squamous Cell Carcinoma. Chin Med (2020) 15:64. doi: 10.1186/s13020-020-00340-y
70. Budhraja A, Turnis ME, Churchman ML, Kothari A, Yang X, Xu H, et al. Modulation of Navitoclax Sensitivity by Dihydroartemisinin-Mediated MCL-1 Repression in BCR-ABL+ B-Lineage Acute Lymphoblastic Leukemia. Clin Cancer Res (2017) 23(24):7558–68. doi: 10.1158/1078-0432.CCR-17-1231
71. Du J, Wang T, Li Y, Zhou Y, Wang X, Yu X, et al. DHA Inhibits Proliferation and Induces Ferroptosis of Leukemia Cells Through Autophagy Dependent Degradation of Ferritin. Free Radic Biol Med (2019) 131(1):356–69. doi: 10.1016/j.freeradbiomed.2018.12.011
72. Mastroianni J, Stickel N, Andrlova H, Hanke K, Melchinger W, Duquesne S, et al. miR-146a Controls Immune Response in the Melanoma Microenvironment. Cancer Res (2019) 79(1):183–95. doi: 10.1158/0008-5472.CAN-18-1397
73. Chen Y, Li R, Zhu Y, Zhong S, Qian J, Yang D, et al. Dihydroartemisinin Induces Growth Arrest and Overcomes Dexamethasone Resistance in Multiple Myeloma. Front Oncol (2020) 10:767. doi: 10.3389/fonc.2020.00767
74. Jin H, Jiang A-Y, Wang H, Cao Y, Wu Y, Jiang X-F. Dihydroartemisinin and Gefitinib Synergistically Inhibit NSCLC Cell Growth and Promote Apoptosis via the Akt/mTOR/STAT3 Pathway. Mol Med Rep (2017) 16(3):3475–81. doi: 10.3892/mmr.2017.6989
75. Chen H, Gu S, Dai H, Li X, Zhang Z. Dihydroartemisinin Sensitizes Human Lung Adenocarcinoma A549 Cells to Arsenic Trioxide via Apoptosis. Biol Trace Elem Res (2017) 179(2):203–12. doi: 10.1007/s12011-017-0975-5
76. Yan X, Li P, Zhan Y, Qi M, Liu J, An Z, et al. Dihydroartemisinin Suppresses STAT3 Signaling and Mcl-1 and Survivin Expression to Potentiate ABT-263-Induced Apoptosis in Non-Small Cell Lung Cancer Cells Harboring EGFR or RAS Mutation. Biochem Pharmacol (2018) 150(1):72–85. doi: 10.1016/j.bcp.2018.01.031
77. Liu X, Wu J, Fan M, Shen C, Dai W, Bao Y, et al. Novel Dihydroartemisinin Derivative DHA-37 Induces Autophagic Cell Death Through Upregulation of HMGB1 in A549 Cells. Cell Death Dis (2018) 9(11):1048. doi: 10.1038/s41419-018-1006-y
78. Yuan B, Liao F, Shi Z-Z, Ren Y, Deng X-L, Yang T-T, et al. Dihydroartemisinin Inhibits the Proliferation, Colony Formation and Induces Ferroptosis of Lung Cancer Cells by Inhibiting PRIM2/SLC7A11 Axis. Onco Targets Ther (2020) 13:10829–40. doi: 10.2147/OTT.S248492
79. Zhang H, Zhou F, Wang Y, Xie H, Luo S, Meng L, et al. Eliminating Radiation Resistance of Non-Small Cell Lung Cancer by Dihydroartemisinin Through Abrogating Immunity Escaping and Promoting Radiation Sensitivity by Inhibiting PD-L1 Expression. Front Oncol (2020) 10:595466. doi: 10.3389/fonc.2020.595466
80. Shen Y, Zhang B, Su Y, Atik Badshah S, Wang X, Li X, et al. Iron Promotes Dihydroartemisinin Cytotoxicity via ROS Production and Blockade of Autophagic Flux via Lysosomal Damage in Osteosarcoma. Front Pharmacol (2020) 11:444. doi: 10.3389/fphar.2020.00444
81. Yao G-D, Ge M-Y, Li D-Q, Chen L, Hayashi T, Tashiro S-I, et al. L-A03, a Dihydroartemisinin Derivative, Promotes Apoptotic Cell Death of Human Breast Cancer MCF-7 Cells by Targeting C-Jun N-Terminal Kinase. BioMed Pharmacother (2018) 105(1):320–5. doi: 10.1016/j.biopha.2018.05.093
82. Li X, Ba Q, Liu Y, Yue Q, Chen P, Li J, et al. Dihydroartemisinin Selectively Inhibits Pdgfrα-Positive Ovarian Cancer Growth and Metastasis Through Inducing Degradation of Pdgfrα Protein. Cell Discov (2017) 3:17042. doi: 10.1038/celldisc.2017.42
83. Li B, Bu S, Sun J, Guo Y, Lai D. Artemisinin Derivatives Inhibit Epithelial Ovarian Cancer Cells via Autophagy-Mediated Cell Cycle Arrest. Acta Biochim Biophys Sin (Shanghai) (2018) 50(12):1227–35. doi: 10.1093/abbs/gmy125
84. Wu B, Hu K, Li S, Zhu J, Gu L, Shen H, et al. Dihydroartiminisin Inhibits the Growth and Metastasis of Epithelial Ovarian Cancer. Oncol Rep (2012) 27(1):101–8. doi: 10.3892/or.2011.1505
85. Wang T, Luo R, Li W, Yan H, Xie S, Xiao W, et al. Dihydroartemisinin Suppresses Bladder Cancer Cell Invasion and Migration by Regulating KDM3A and P21. J Cancer (2020) 11(5):1115–24. doi: 10.7150/jca.36174
86. Du S, Xu G, Zou W, Xiang T, Luo Z. Effect of Dihydroartemisinin on UHRF1 Gene Expression in Human Prostate Cancer PC-3 Cells. Anticancer Drugs (2017) 28(4):384–91. doi: 10.1097/CAD.0000000000000469
87. Noori S, Hassan ZM. Dihydroartemisinin Shift the Immune Response Towards Th1, Inhibit the Tumor Growth in Vitro and in Vivo. Cell Immunol (2011) 271(1):67–72. doi: 10.1016/j.cellimm.2011.06.008
88. Zhou Z-H, Chen F-X, Xu W-R, Qian H, Sun L-Q, Lü X-T, et al. Enhancement Effect of Dihydroartemisinin on Human γδ T Cell Proliferation and Killing Pancreatic Cancer Cells. Int Immunopharmacol (2013) 17(3):850–7. doi: 10.1016/j.intimp.2013.09.015
89. Zhu W, Li Y, Zhao D, Li H, Zhang W, Xu J, et al. Dihydroartemisinin Suppresses Glycolysis of LNCaP Cells by Inhibiting PI3K/AKT Pathway and Downregulating HIF-1α Expression. Life Sci (2019) 233:116730. doi: 10.1016/j.lfs.2019.116730
90. Paccez JD, Duncan K, Sekar D, Correa RG, Wang Y, Gu X, et al. Dihydroartemisinin Inhibits Prostate Cancer via JARID2/miR-7/miR-34a-Dependent Downregulation of Axl. Oncogenesis (2019) 8(3):14. doi: 10.1038/s41389-019-0122-6
91. Chen G-Q, Benthani FA, Wu J, Liang D, Bian Z-X, Jiang X. Artemisinin Compounds Sensitize Cancer Cells to Ferroptosis by Regulating Iron Homeostasis. Cell Death Differ (2020) 27(1):242–54. doi: 10.1038/s41418-019-0352-3
92. Bai G, Gao Y, Liu S, Shui S, Liu G. pH-Dependent Rearrangement Determines the Iron-Activation and Antitumor Activity of Artemisinins. Free Radic Biol Med (2021) 163:234–42. doi: 10.1016/j.freeradbiomed.2020.12.024
93. Xia M, Liu D, Tang X, Liu Y, Liu H, Liu Y, et al. Dihydroartemisinin Inhibits the Proliferation of IgAN Mesangial Cells Through the mTOR Signaling Pathway. Int Immunopharmacol (2020) 80:106125. doi: 10.1016/j.intimp.2019.106125
94. Liu J, Jin Q, Fujimoto M, Li F, Jin L, Yu R, et al. Dihydroartemisinin Alleviates Imiquimod-Induced Psoriasis-Like Skin Lesion in Mice. Front Pharmacol (2021) 12:704481. doi: 10.3389/fphar.2021.704481
95. Chen Y, Yan Y, Liu H, Qiu F, Liang C-L, Zhang Q, et al. Dihydroartemisinin Ameliorates Psoriatic Skin Inflammation and Its Relapse by Diminishing CD8+ T-Cell Memory in Wild-Type and Humanized Mice. Theranostics (2020) 10(23):10466–82. doi: 10.7150/thno.45211
96. Zhao YG, Wang Y, Guo Z, Gu A-d, Dan HC, Baldwin AS, et al. Dihydroartemisinin Ameliorates Inflammatory Disease by Its Reciprocal Effects on Th and Regulatory T Cell Function via Modulating the Mammalian Target of Rapamycin Pathway. J Immunol (2012) 189(9):4417–25. doi: 10.4049/jimmunol.1200919
97. Gao Y, Cui M, Zhong S, Feng C, Kenechukwu Nwobodo A, Chen B, et al. Dihydroartemisinin Ameliorates LPS-Induced Neuroinflammation by Inhibiting the PI3K/AKT Pathway. Metab Brain Dis (2020) 35(4):661–72. doi: 10.1007/s11011-020-00533-2
98. Li N, Sun W, Zhou X, Gong H, Chen Y, Chen D, et al. Dihydroartemisinin Protects Against Dextran Sulfate Sodium-Induced Colitis in Mice Through Inhibiting the PI3K/AKT and NF- κb Signaling Pathways. BioMed Res Int (2019) 2019:1415809. doi: 10.1155/2019/1415809
99. Liang R, Chen W, Fan H, Chen X, Zhang J, Zhu J-S. Dihydroartemisinin Prevents Dextran Sodium Sulphate-Induced Colitisthrough Inhibition of the Activation of NLRP3 Inflammasome and P38 MAPK Signaling. Int Immunopharmacol (2020) 88:106949. doi: 10.1016/j.intimp.2020.106949
100. Yan SC, Wang YJ, Li YJ, Cai WY, Weng XG, Li Q, et al. Dihydroartemisinin Regulates the Th/Treg Balance by Inducing Activated CD4+ T Cell Apoptosis via Heme Oxygenase-1 Induction in Mouse Models of Inflammatory Bowel Disease. Molecules (2019) 24(13):2475. doi: 10.3390/molecules24132475
101. Lei Z, Yang Y, Liu S, Lei Y, Yang L, Zhang X, et al. Dihydroartemisinin Ameliorates Dextran Sulfate Sodium Induced Inflammatory Bowel Diseases in Mice. Bioorg Chem (2020) 100:103915. doi: 10.1016/j.bioorg.2020.103915
102. Ge X, Chen Z, Xu Z, Lv F, Zhang K, Yang Y. The Effects of Dihydroartemisinin on Inflammatory Bowel Disease-Related Bone Loss in a Rat Model. Exp Biol Med (Maywood) (2018) 243(8):715–24. doi: 10.1177/1535370218769420
103. Fan M, Li Y, Yao C, Liu X, Liu X, Liu J. Dihydroartemisinin Derivative DC32 Attenuates Collagen-Induced Arthritis in Mice by Restoring the Treg/Th17 Balance and Inhibiting Synovitis Through Down-Regulation of IL-6. Int Immunopharmacol (2018) 65:233–43. doi: 10.1016/j.intimp.2018.10.015
104. Fan M, Li Y, Yao C, Liu X, Liu J, Boyang Y. DC32, a Dihydroartemisinin Derivative, Ameliorates Collagen-Induced Arthritis Through an Nrf2-P62-Keap1 Feedback Loop. Front Immunol (2018) 9:2762. doi: 10.3389/fimmu.2018.02762
105. Li Y-N, Fan M-L, Liu H-Q, Ma B, Dai W-L, Yu B-Y, et al. Dihydroartemisinin Derivative DC32 Inhibits Inflammatory Response in Osteoarthritic Synovium Through Regulating Nrf2/NF-κb Pathway. Int Immunopharmacol (2019) 74:105701. doi: 10.1016/j.intimp.2019.105701
106. Li W-d, Dong Y-j, Tu Y-y, Lin Z-b. Dihydroarteannuin Ameliorates Lupus Symptom of BXSB Mice by Inhibiting Production of TNF-Alpha and Blocking the Signaling Pathway NF-Kappa B Translocation. Int Immunopharmacol (2006) 6(8):1243–50. doi: 10.1016/j.intimp.2006.03.004
107. Huang X, Xie Z, Liu F, Han C, Zhang D, Wang D, et al. Dihydroartemisinin Inhibits Activation of the Toll-Like Receptor 4 Signaling Pathway and Production of Type I Interferon in Spleen Cells From Lupus-Prone MRL/lpr Mice. Int Immunopharmacol (2014) 22(1):266–72. doi: 10.1016/j.intimp.2014.07.001
108. Li D, Qi J, Wang J, Pan Y, Li J, Xia X, et al. Protective Effect of Dihydroartemisinin in Inhibiting Senescence of Myeloid-Derived Suppressor Cells From Lupus Mice via Nrf2/HO-1 Pathway. Free Radic Biol Med (2019) 143(1):260–74. doi: 10.1016/j.freeradbiomed.2019.08.013
109. Diao L, Tao J, Wang Y, Hu Y, He W. Co-Delivery Of Dihydroartemisinin And HMGB1 siRNA By TAT-Modified Cationic Liposomes Through The TLR4 Signaling Pathway For Treatment Of Lupus Nephritis. Int J Nanomed (2019) 14:8627–45. doi: 10.2147/IJN.S220754
110. Liu X, Lu J, Liao Y, Liu S, Chen Y, He R, et al. Dihydroartemisinin Attenuates Lipopolysaccharide-Induced Acute Kidney Injury by Inhibiting Inflammation and Oxidative Stress. BioMed Pharmacother (2019) 117:109070. doi: 10.1016/j.biopha.2019.109070
111. Wei M, Xie X, Chu X, Yang X, Guan M, Wang D. Dihydroartemisinin Suppresses Ovalbumin-Induced Airway Inflammation in a Mouse Allergic Asthma Model. Immunopharmacol Immunotoxicol (2013) 35(3):382–89. doi: 10.3109/08923973.2013.785559
112. Zhu H, Ji W. Dihydroartemisinin Ameliorated Ovalbumin-Induced Asthma in Mice via Regulation of MiR-183c. Med Sci Monit (2019) 25:3804–14. doi: 10.12659/MSM.915399
113. Ravindra KC, Ho WE, Cheng C, Godoy LC, Wishnok JS, Ong CN, et al. Untargeted Proteomics and Systems-Based Mechanistic Investigation of Artesunate in Human Bronchial Epithelial Cells. Chem Res Toxicol (2015) 28(10):1903–13. doi: 10.1021/acs.chemrestox.5b00105
114. Huang X-T, Liu W, Zhou Y, Hao C-X, Zhou Y, Zhang C-Y, et al. Dihydroartemisinin Attenuates Lipopolysaccharide−induced Acute Lung Injury in Mice by Suppressing Nf−κb Signaling in an Nrf2−dependent Manner. Int J Mol Med (2019) 4(6):2213–22. doi: 10.3892/ijmm.2019.4387
115. Miller KD, Siegel RL, Lin CC, Mariotto AB, Kramer JL, Rowland JH, et al. Cancer Treatment and Survivorship Statistics, 2016. CA Cancer J Clin (2016) 66(4):271–89. doi: 10.3322/caac.21349
116. Liao Y-H, Li C-I, Lin C-C, Lin J-G, Chiang J-H, Li T-C. Traditional Chinese Medicine as Adjunctive Therapy Improves the Long-Term Survival of Lung Cancer Patients. J Cancer Res Clin Oncol (2017) 143(12):2425–35. doi: 10.1111/and.13280
117. Kong J, Li S-S, Ma Q, Liu L, Zheng L-J. Effects of Dihydroartemisinin on HSP70 Expression in Human Prostate Cancer PC-3 Cells. Andrologia (2019) 51(6):e13280. doi: 10.1111/and.13280
118. Lu Z-H, Peng J-H, Zhang R-X, Wang F, Sun H-P, Fang Y-J, et al. Dihydroartemisinin Inhibits Colon Cancer Cell Viability by Inducing Apoptosis Through Up-Regulation of Pparγ Expression. Saudi J Biol Sci (2018) 25(2):372–6. doi: 10.1016/j.sjbs.2017.02.002
119. Elhassanny AEM, Soliman E, Marie M, McGuire P, Gul W, ElSohly M, et al. Heme-Dependent ER Stress Apoptosis: A Mechanism for the Selective Toxicity of the Dihydroartemisinin, NSC735847, in Colorectal Cancer Cells. Front Oncol (2020) 10:965. doi: 10.3389/fonc.2020.00965
120. Luo Y, Guo Q, Zhang L, Zhuan Q, Meng L, Fu X, et al. Dihydroartemisinin Exposure Impairs Porcine Ovarian Granulosa Cells by Activating PERK-Eif2α-ATF4 Through Endoplasmic Reticulum Stress. Toxicol Appl Pharmacol (2020) 403:115159. doi: 10.1016/j.taap.2020.115159
121. Xie Y, Hou W, Song X, Yu Y, Huang J, Sun X, et al. Ferroptosis: Process and Function. Cell Death Differ (2016) 23(3):369–79. doi: 10.1038/cdd.2015.158
122. Ohue Y, Nishikawa H. Regulatory T (Treg) Cells in Cancer: Can Treg Cells Be a New Therapeutic Target? Cancer Sci (2019) 110(7):2080–9. doi: 10.1111/cas.14069
123. Gardner A, Ruffell B. Dendritic Cells and Cancer Immunity. Trends Immunol (2016) 37(12):855–65. doi: 10.1016/j.it.2016.09.006
124. Ruffell B, Coussens LM. Macrophages and Therapeutic Resistance in Cancer. Cancer Cell (2015) 27(4):462–72. doi: 10.1016/j.ccell.2015.02.015
125. Chen R, Lu X, Li Z, Sun Y, He Z, Li X. Dihydroartemisinin Prevents Progression and Metastasis of Head and Neck Squamous Cell Carcinoma by Inhibiting Polarization of Macrophages in Tumor Microenvironment. Onco Targets Ther (2020) 13(1):3375–87. doi: 10.2147/OTT.S249046
126. Jin G, Hamaguchi Y, Matsushita T, Hasegawa M, Le Huu D, Ishiura N, et al. B-Cell Linker Protein Expression Contributes to Controlling Allergic and Autoimmune Diseases by Mediating IL-10 Production in Regulatory B Cells. J Allergy Clin Immunol (2013) 131(6):1674–82. doi: 10.1016/j.jaci.2013.01.044
127. Khor B, Gardet A, Xavier RJ. Genetics and Pathogenesis of Inflammatory Bowel Disease. Nature (2011) 474(7351):307–17. doi: 10.1038/nature10209
128. Feng M, Hong J, Wang Q, Fan Y, Yuan C, Lei X, et al. Dihydroartemisinin Prevents Breast Cancer-Induced Osteolysis via Inhibiting Both Breast Cancer Cells and Osteoclasts. Sci Rep (2016) 6:19074. doi: 10.1038/srep19074
129. Zhou L, Liu Q, Yang M, Wang T, Yao J, Cheng J, et al. Dihydroartemisinin, an Anti-Malaria Drug, Suppresses Estrogen Deficiency-Induced Osteoporosis, Osteoclast Formation, and RANKL-Induced Signaling Pathways. J Bone Miner Res (2016) 31(5):964–74. doi: 10.1002/jbmr.2771
130. Dou C, Ding N, Xing J, Zhao C, Kang F, Hou T, et al. Dihydroartemisinin Attenuates Lipopolysaccharide-Induced Osteoclastogenesis and Bone Loss via the Mitochondria-Dependent Apoptosis Pathway. Cell Death Dis (2016) 7(3):e2162. doi: 10.1038/cddis.2016.69
131. Yang J, Ren J, Yang Y, Sun J, Zhou X, Zheng S, et al. BANK1 Alters B Cell Responses and Influences the Interactions Between B Cells and Induced T Regulatory Cells in Mice With Collagen-Induced Arthritis. Arthritis Res Ther (2018) 20(1):9. doi: 10.1186/s13075-017-1503-x
132. Carter EE, Barr SG, Clarke AE. The Global Burden of SLE: Prevalence, Health Disparities and Socioeconomic Impact. Nat Rev Rheumatol (2016) 12(10):605–20. doi: 10.1038/nrrheum.2016.137
133. Medoff BD, Thomas SY, Luster AD. T Cell Trafficking in Allergic Asthma: The Ins and Outs. Annu Rev Immunol (2008) 26(1):205–32. doi: 10.1146/annurev.immunol.26.021607.090312
134. Gour R, Ahmad F, Prajapati SK, Giri SK, Lal Karna SK, Ravindranathan Kartha KP, et al. Synthesis of Novel S-Linked Dihydroartemisinin Derivatives and Evaluation of Their Anticancer Activity. Eur J Med Chem (2019) 178(1):552–70. doi: 10.1016/j.ejmech.2019.06.018
135. Zhu Y, Klausen C, Zhou J, Guo X, Zhang Y, Zhu H, et al. Novel Dihydroartemisinin Dimer Containing Nitrogen Atoms Inhibits Growth of Endometrial Cancer Cells and May Correlate With Increasing Intracellular Peroxynitrite. Sci Rep (2019) 9(1):15528. doi: 10.1038/s41598-019-52108-6
136. Wang Z, Duan X, Lv Y, Zhao Y. Low Density Lipoprotein Receptor (LDLR)-Targeted Lipid Nanoparticles for the Delivery of Sorafenib and Dihydroartemisinin in Liver Cancers. Life Sci (2019) 239:117013. doi: 10.1016/j.lfs.2019.117013
137. Phung CD, Le TG, Nguyen VH, Vu TT, Nguyen HQ, Kim JO, et al. PEGylated-Paclitaxel and Dihydroartemisinin Nanoparticles for Simultaneously Delivering Paclitaxel and Dihydroartemisinin to Colorectal Cancer. Pharm Res (2020) 37(7):129. doi: 10.1007/s11095-020-02819-7
138. Huang T-E, Deng Y-N, Hsu J-L, Leu W-J, Marchesi E, Capobianco ML, et al. Evaluation of the Anticancer Activity of a Bile Acid-Dihydroartemisinin Hybrid Ursodeoxycholic-Dihydroartemisinin in Hepatocellular Carcinoma Cells. Front Pharmacol (2020) 11:599067. doi: 10.3389/fphar.2020.599067
139. Liu J-J, Tang W, Fu M, Gong X-Q, Kong L, Yao X-M, et al. Development of R8 Modified Epirubicin-Dihydroartemisinin Liposomes for Treatment of Non-Small-Cell Lung Cancer. Artif Cells Nanomed Biotechnol (2019) 47(1):1947–60. doi: 10.1080/21691401.2019.1615932
140. Li L, Wu J, Weng S, Yang L, Wang H, Yizhuang X, et al. Fourier Transform Infrared Spectroscopy Monitoring of Dihydroartemisinin-Induced Growth Inhibition in Ovarian Cancer Cells and Normal Ovarian Surface Epithelial Cells. Cancer Manag Res (2020) 12:653–61. doi: 10.2147/CMAR.S240285
141. Ou L, Qin K, Yang Z, Bie M. The Effects and Mechanisms of Dihydroartemisinin on Influenza A Virus H1N1 Induces TNF-α and IL-6 Expression in Bronchial Epithelial Cells. J Sichuan Univ (Med Sci Edi) (2020) 51(2):171–7. doi: 10.12182/20200360604
142. Jansen FH, Adoubi I, Kouassi Comoe JC, Cnodder TDE, Jansen N, Tschulakow A, et al. First Study of Oral Artenimol-R in Advanced Cervical Cancer: Clinical Benefit, Tolerability and Tumor Markers. Anticancer Res (2011) 31(12):4417–22.
Keywords: dihydroartemisinin (DHA), anti-tumor activity, programmed cell death, anti-tumor immunity, inflammatory diseases, pharmacological mechanism
Citation: Yu R, Jin G and Fujimoto M (2021) Dihydroartemisinin: A Potential Drug for the Treatment of Malignancies and Inflammatory Diseases. Front. Oncol. 11:722331. doi: 10.3389/fonc.2021.722331
Received: 08 June 2021; Accepted: 10 September 2021;
Published: 07 October 2021.
Edited by:
Olivier Cuvillier, UPR8241 Laboratoire de Chimie de Coordination (LCC), FranceReviewed by:
Zhaofeng Liang, Jiangsu University, ChinaHoi Huen Chan, Hong Kong Polytechnic University, Hong Kong, SAR China
Copyright © 2021 Yu, Jin and Fujimoto. This is an open-access article distributed under the terms of the Creative Commons Attribution License (CC BY). The use, distribution or reproduction in other forums is permitted, provided the original author(s) and the copyright owner(s) are credited and that the original publication in this journal is cited, in accordance with accepted academic practice. No use, distribution or reproduction is permitted which does not comply with these terms.
*Correspondence: Guihua Jin, Z2hqaW5AeWJ1LmVkdS5jbg==; Manabu Fujimoto, ZnVqaW1vdG9AZGVybWEubWVkLm9zYWthLXUuYWMuanA=