- 1Outpatient Department of Oral and Maxillofacial Surgery, Beijing Stomatological Hospital, School of Stomatology, Capital Medical University, Beijing, China
- 2Department of Periodontics, Beijing Stomatological Hospital, School of Stomatology, Capital Medical University, Beijing, China
- 3Multi-disciplinary Treatment Center, Beijing Stomatological Hospital, School of Stomatology, Capital Medical University, Beijing, China
- 4Department of Prosthodontics, Stomatological Hospital of Chongqing Medical University, Chongqing, China
- 5Chongqing Key Laboratory of Oral Diseases and Biomedical Sciences, Chongqing, China
- 6Chongqing Municipal Key Laboratory of Oral Biomedical Engineering of Higher Education, Chongqing, China
- 7Department of Dentistry, Chongqing Huamei Plastic Surgery Hospital, Chongqing, China
The anticancer drug 5-fluorouracil (5-FU) resistance is a major obstacle to reducing the effectiveness of cancer treatment, and its detailed mechanism has not been fully elucidated. Here, in 5-FU-resistant human oral squamous cell carcinoma (OSCC) HSC3 cells (HSC3/5-FU), the levels of 21 miRNA candidates were detected using RT-PCR and miR-155-5p level increased strikingly in HSC3/5-FU cells compared to HSC3 cells. Compared with HSC3 cells, the CCK-8 assay showed that the HSC3/5-FU cells transfected with miR-155-5p inhibitors decreased 5-FU IC50. Ectopic expression of miR-155-5p in HSC3 and HSC4 cells increased 5-FU IC50 (CCK-8 assay), migration (wound-healing and transwell assays) and invasion (transwell assay) abilities. Seven miR-155-5p target candidates were discovered by miRNA prediction algorithms (miRDB, Targetscan, and miRWalk), and the RT-PCR results showed that in HSC3/5-FU cells TP53INP1 was of the lowest mRNA expression level compared with HSC3 cells. The RT-PCR and Western blotting assays showed that ectopic expression of miR-155-5p in HSC3 and HSC4 cells decreased TP53INP1 expression level. Furthermore, the luciferase reporter and RNA pull-down assays determined the interference effect of miR-155-5p on TP53INP1 expression. The enhancement of cell viability (CCK-8 assay), migration (wound-healing and transwell assays) and invasion (transwell assay) by miR-155-5p after 5-FU treatment was reversed by TP53INP1 overexpression. After treatment with 5-FU, HSC3-miR-155-5p tumor-bearing nude mice presented growing tumors, while HSC3-TP53INP1 group possessed shrinking tumors. In conclusion, these results lead to the proposal that miR-155-5p enhances 5-FU resistance by decreasing TP53INP1 expression in OSCC.
Introduction
Globally, head and neck cancer is ranked as the sixth most common cancer and the majority cases are attributed to oral squamous cell carcinoma(OSCC) (1). Presently, a multidisciplinary approach, including surgery, radiotherapy, chemotherapy, or the combinations of these treatments has been applied for the treatment of OSCC patients (2). Although the treatment is effective for patients at the early stage, the 5-year survival rate of OSCC patients in advanced-stage remains <50% (3–5). Among the chemotherapy, 5-fluorouracil (5-FU) is commonly used and its active metabolites interferes with DNA and RNA synthesis, resulting to cell cycle arrest or cell death (6). However, the acquisition of resistance to 5-FU based chemotherapy leads to treatment failure in advanced and recurrent OSCC, resulting in a poor prognosis (7). Therefore, discovering the mechanism underlying the resistance to 5-FU of OSCC is of great importance for improving treatment regimen and bettering prognosis.
microRNAs (miRNAs/miRs) are critical gene expression regulators that bind to the 3’-untranslated region (3’-UTR) of target mRNAs, inducing mRNA degradation or translational suppression (8). Accumulating evidence has shown that aberrant expressions of miRNAs are involved in cancer progression by influencing various cell biological behaviors (9–11). miR-155 is one of the most important microRNAs, its upregulation increases cell growth, invasion, migration, stemness, and angiogenesis in many cancers (12). In OSCC, the overexpression of miR-155 facilitates proliferation and invasion of cancer cells and is associated with poor prognosis (13, 14). In addition, research documented that overexpressed miR-155 is found to be responsible for multidrug resistance in various tumor types (15–17). Notably, miRNA is associated with 5-FU resistance in colorectal cancer (17, 18). However, the role of miR-155 in 5-FU resistance of OSCC cells are still elusive.
Here, we displayed that miR-155 was increased in 5-FU resistant OSCC HSC3 cells. In silico analysis and functional studies further revealed that miR-155 contributes to 5-FU resistance in OSCC cells by targeting tumor protein p53 inducible nuclear protein 1 (TP53INP1). Our study suggests that miR-155 is a potential therapeutic target for overcoming 5-FU resistance during the treatment of OSCC.
Results
Establishment of miR-155-5p Associated with 5-FU Resistance in HSC3 Cells
To identify miRNAs contributing to 5-FU resistance of OSCC cells, we first incubated HSC3 cells with 5-FU (HSC3/5-FU) and increased the concentration of 5-FU every two weeks. After 5 months, the IC 50 of 5-Fu in HSC3/5-FU cells were approximately 6.945 μM, it was estimated to be approximately seventeen folders higher than that to HSC3 cells (Figure 1A). Then, we further investigated the expression profile of miRNA candidates [miR-155 (18, 19), miR-27a (19), miR-135b (20), miR-182 (20), miR-587 (21), miR-23a (22), miR-125b (23), miR-224 (24), miR-21 (25), miR-1290 (26), miR-425-5P (27), miR-10b (28), miR-196b-5p (29),miR-330 (30), miR-375-3p (31), miR-203 (32), miR-218 (33), miR-139-5p (34), miR-129 (35), miR-192 (36), miR-215 (36)] in HSC3/5-FU and HSC3 cells and analyzed the expression of miRNAs using RT-PCR. The results showed that the expression of miR-155-5p was strikingly upregulated in HSC3/5-FU cells compared to HSC3 cells (Figure 1B). These results indicate that the deregulation of miR-155 might be involved in the 5-FU-resistance of OSCC cells.
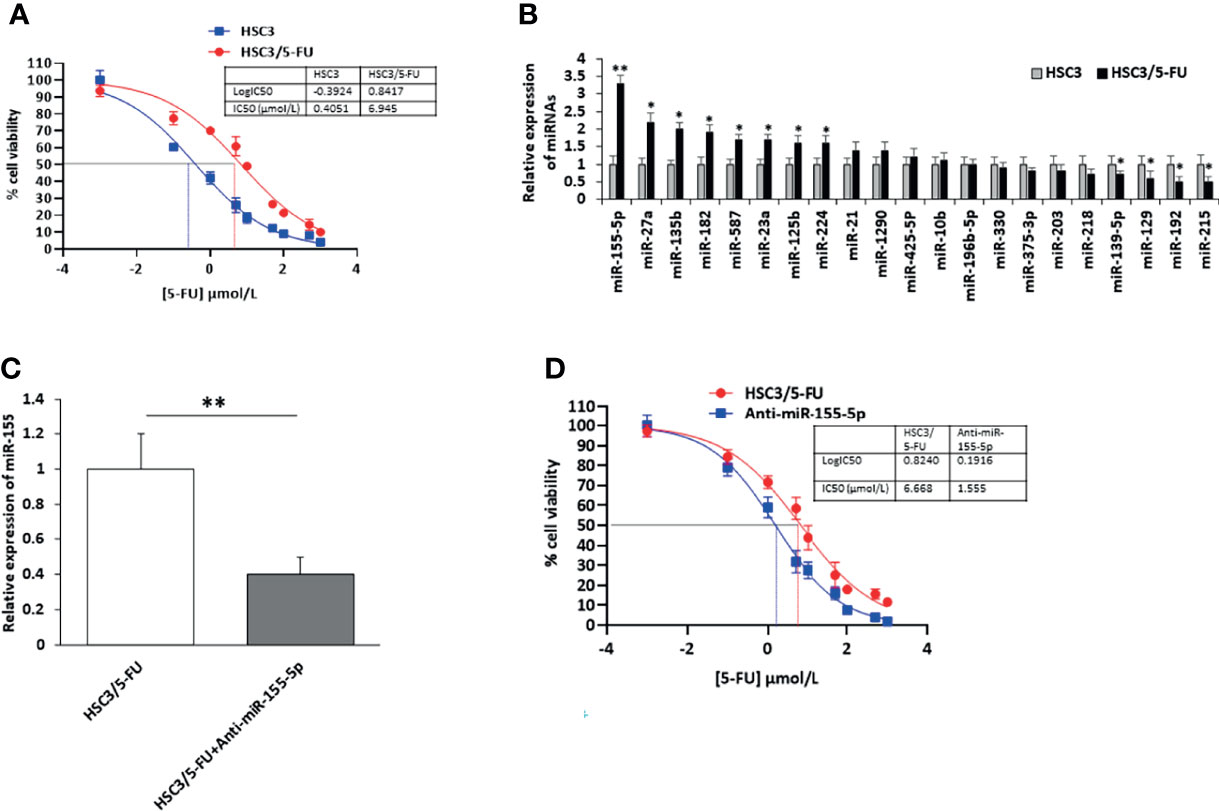
Figure 1 Upregulation of miR-155-5p in 5-FU resistant HSC3/5-FU cells. (A) The IC50 of 5-Fu in 5-FU resistant HSC3/5-FU cells. (B) The expression profile of miRNA candidates in HSC3/5-FU cells analyzed using RT-PCR. (C) The level of miR-155-5p in HSC3/5-FU cells with miR-155-5p inhibitors (Anti-miR-155-5p) was measured by RT-PCR. (D) The IC50 of 5-FU in HSC3/5-FU cells transfected with anti-miR-155-5p was measured using CCK-8 assay. IC50, half-maximal inhibitory concentration. *p < 0.05, **p < 0.01.
To further investigate the role of miR-155-5p in the resistance to 5-FU in OSCC cells, we transfected HSC3/5-FU cells with negative control (NC) and miR-155-5p inhibitors (anti-miR-155-5p) and determined the expression level of miR-155-5p by RT-PCR (Figure 1C). As a result, half-maximal inhibitory concentration (IC50) was determined to be 1.555 μmol/L for Anti-miR-155-5p group (Figure 1D).
MiR-155-5p Increased 5-FU Resistance in HSC3 and HSC4 Cells
To explore the influence of miR-155-5p on 5-FU resistance in OSCC cells, we transfected HSC3 and HSC4 cells with miR-155-5p mimic vector to overexpress miR-155-5p (Figure 2A), and the miR-155-5p increased cell viability (Figure 2B). IC50 was determined to be 6.086 μmol/L and 5.842 for the miR-155-5p overexpression HSC3 and HSC4 cells, while 0.277 μmol/L and 0.251 μmol/L for the HSC3 and HSC4 mock cells respectively (Figure 2C). The wound-healing assay (Figure 2D), transwell migration assay (Figure 2E) and transwell invasion assay (Figure 2F) showed that miR-155-5p increased sensitivity to 5-FU.
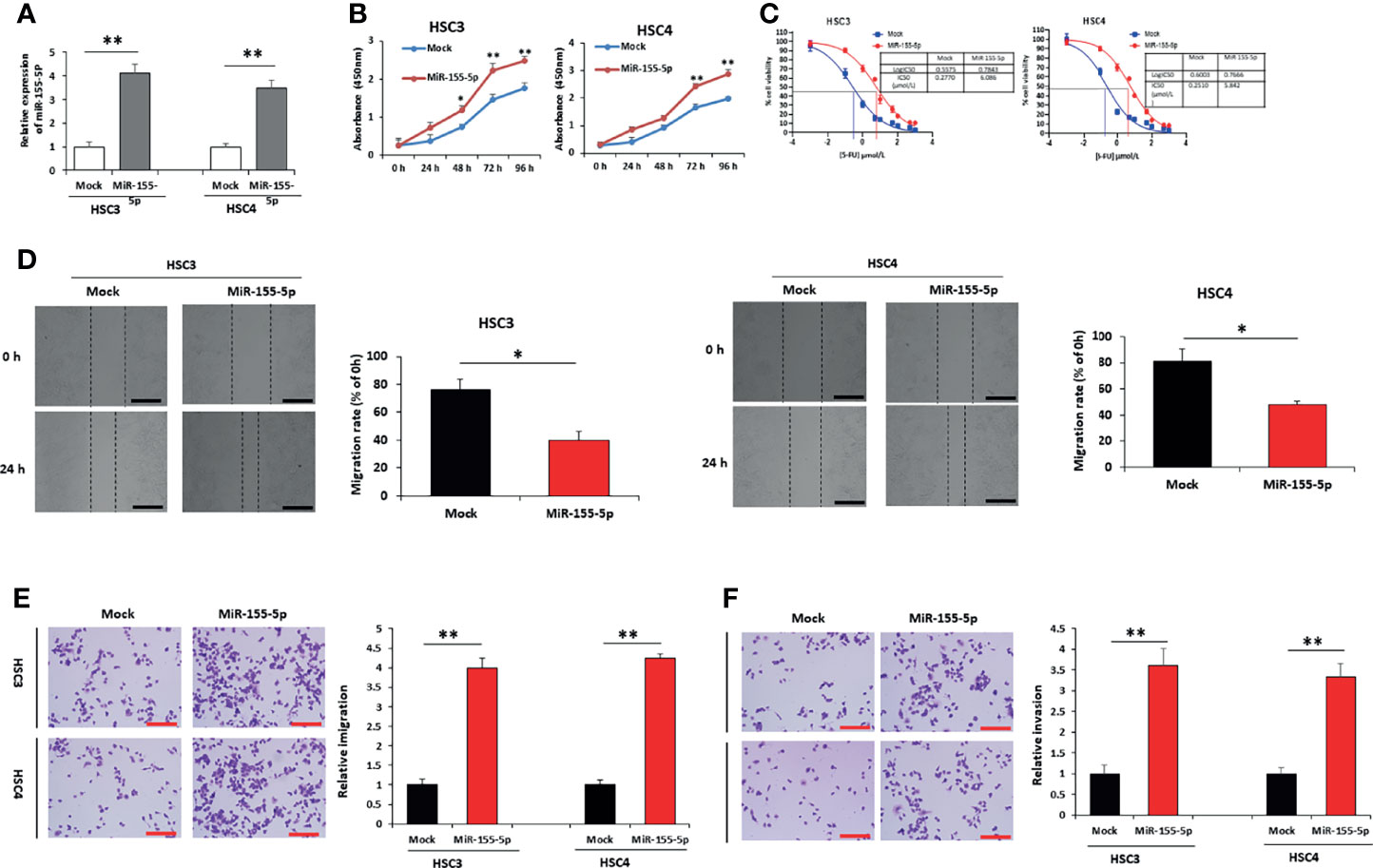
Figure 2 Over-expression of miR-155-5p induces resistance to 5-FU in HSC3 and HSC4 cells. (A) RT-PCR results showed the expression of miR-155-5p in HSC3 and HSC4 cells overexpressing miR-155-5p. (B) CCK-8 assay presented the proliferation of HSC3 and HSC4 cells overexpressing miR-155-5p. (C) The IC50 of 5-FU in HSC3 and HSC4 cells overexpressing miR-155-5p. Analysis of 5-FU-treated HSC3 and HSC4 cells transduction of miR-155-5p and mock vector using (D) the wound-healing assay, (E) the transwell migration assay, and (F) the transwell invasion assay. Scale bar, 100 μm. *p < 0.05, **p < 0.01.
MiR-155-5p Reduced TP53INP1 Expression in OSCC Cells
To discover the target mRNAs of miR-155-5p, we employed three different miRNA prediction algorithms (miRDB, Targetscan, and miRWalk) (37–40). A survey revealed that seven mRNAs, JARID2, BACH1, KDM5B, TP53INP1, TSHZ3, VAV3 and RGP1 are possible targets of miR-155-5p (Figure 3A, Supplementary Table 2), and we measured their mRNA expression levels in HSC3, HSC3/5-FU, HSC3/5-FU+Anti-miR-155-5p cells. The results showed that among the seven genes, TP53INP1 presented the lowest expression level in HSC3/5-FU clone, compared to HSC3 cells (Figure 3B). To assess the roles of miR-155-5p in TP53INP1 regulation, we analyzed both mRNA and protein levels of TP53INP1 after transfection of mock, miR-155-5p mimic, miR-155-5p mimic+Anti-miR-155-5p (Rescue) vector into HSC3 and HSC4 cells. The results showed that both mRNA and protein levels of TP53INP1 were downregulated by miR-155-5p (Figures 3C, D). The 3′-UTR of TP53INP1 mRNA contains a complementary site for the seed region of miR-155-5p (Figure 3E). To further confirm TP53INP1 regulation by miR-155-5p, we generated luciferase reporter constructs containing the TP53INP1 mRNA 3′UTR (741-748 nt) (pluci-TP53INP1 3U) or mutant 3′UTR missing the seed region binding sites for miR-155-5p (pluci-TP53INP1 3UM), and 48 h post-transfection, analyzed luciferase reporter expression after miR-155-5p over-expression. As shown in Figure 3F, miR-155-5p downregulated pluci-TP53INP1 3U reporter expression but did not affect the luciferase control or pluci-TP53INP1 3UM. Moreover, the RNA pull-down assay showed that TP53INP1 was enriched by biotinylated miR-155-5p, proving their direct interaction (Supplementary Figure 2). These results indicate that miR-155-5p negatively regulated the expression of TP53INP1 by binding to its 3′-UTR.
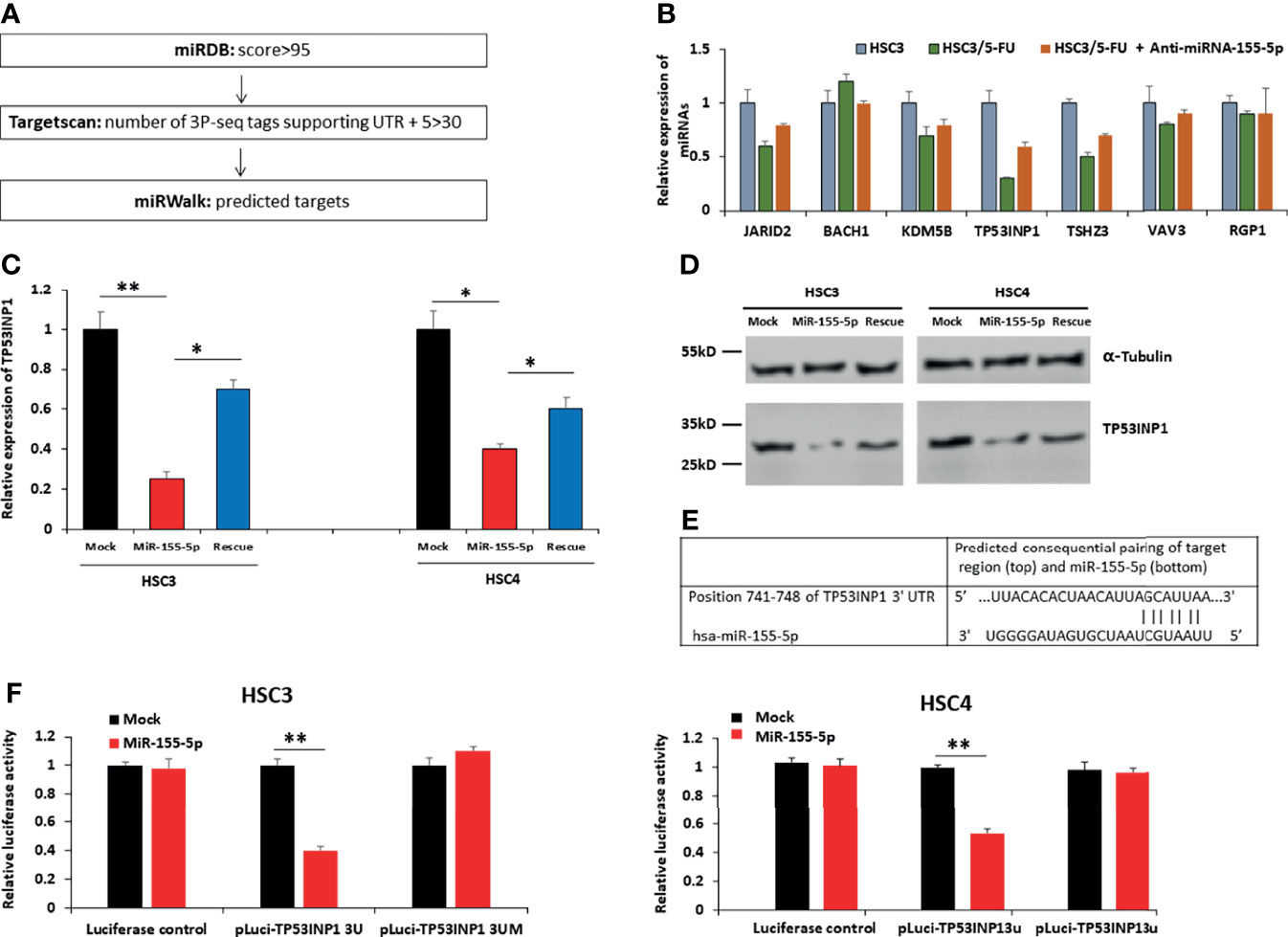
Figure 3 TP53INP1 is a direct target of miR-155-5p in OSCC cells. (A) Three different miRNA prediction algorithms (miRDB, Targetscan, and miRWalk) revealed miR-155-5p targets candidates. (B) The mRNA expression levels of miR-155-5p targets candidates in HSC3, HSC3/5-FU, HSC3/5-FU+Anti-miR-155-5p cells. (C) The mRNA and (D) protein levels of TP53INP1 in HSC3 and HSC4 cells infected with vectors expressing miR-155-5p mimic or miR-155-5p mimic +anti-miR-155-5p (rescue). (E) The prediction of the binding between miR-155-5p and TP53INP1 by Pictar. (F) Relative luciferase activity of the indicated TP53INP1 reporter constructs in HSC3 cells. *p < 0.05, **p < 0.01.
MiR-155-5p Stimulates 5-FU Resistance by Targeting TP53INP1 in OSCC
We noticed that miR-155-5p increased cell viability after 5-FU treatment (Figure 2B) and downregulated TP53INP1 expression (Figures 3C, D, F). To further determine whether the increase in 5-FU resistance by miR-155-5p is mediated by the downregulation of TP53INP1, we investigated the sensitivity of HSC3-miR-155-5p and HSC4-miR-155-5p cells to 5-FU after over-expression of TP53INP1 (Supplementary Figure 1). As a result, increased cell viability (Figure 4A), migration (Figures 4B, C) and invasion (Figure 4D) by miR-155-5p after 5-FU treatment was reversed by over-expression of TP53INP1. These results demonstrated that the miR-155-5p regulated the 5-FU resistance of OSCC cells via targeting TP53INP1.
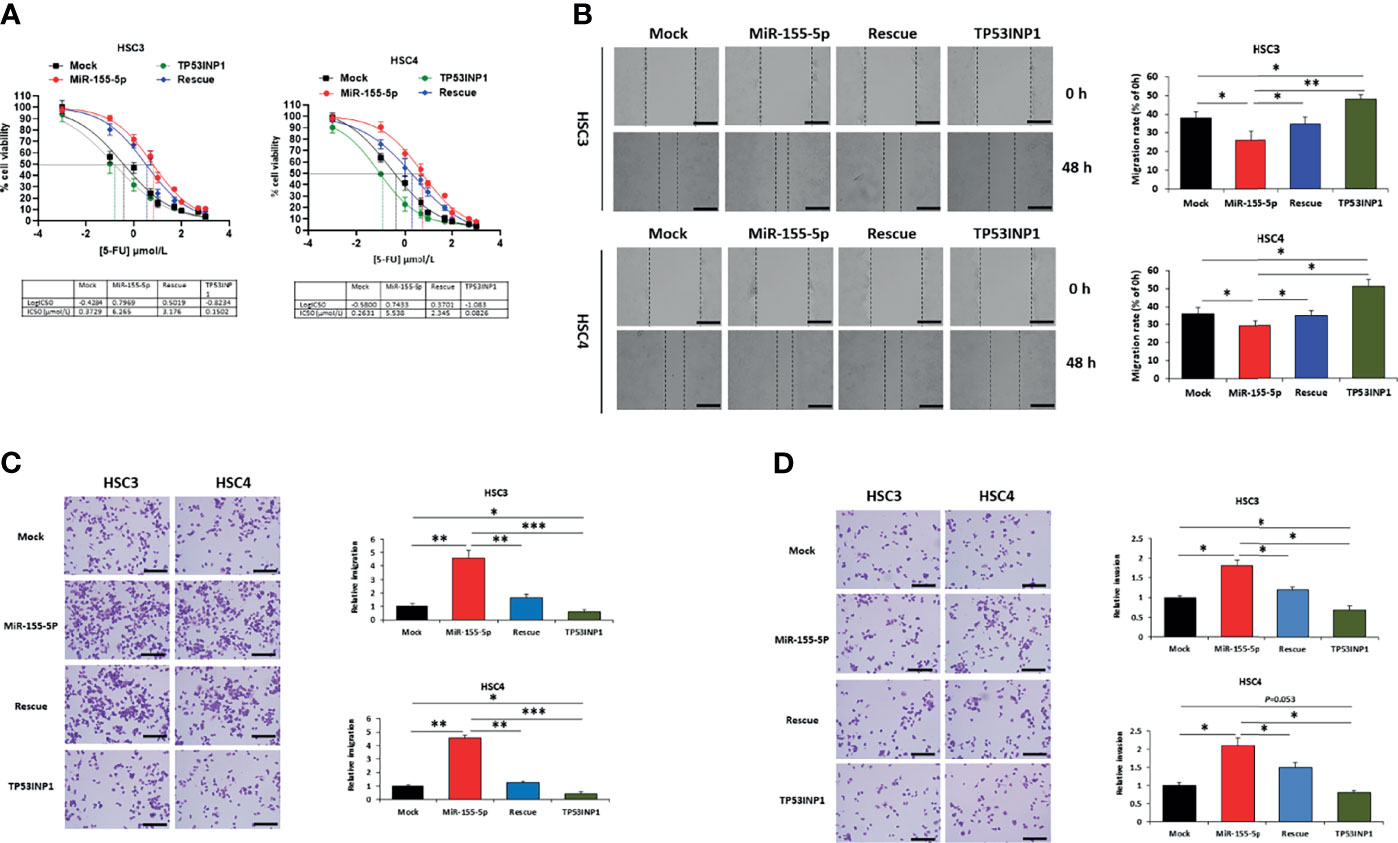
Figure 4 MiR-155-5p enhanced 5-FU resistance through targeting TP53INP1 in OSCC. After 5-FU treatment, (A) IC50 of 5-FU, (B) the wound-healing assay, (C) the transwell migration assay, and (D) the transwell invasion assay in HSC3 and HSC4 cells transfected with mock, miR-155-5p mimic, miR-155-5p+TP53INP1 (rescue) or TP53INP1 over-expression plasmid. Scale bar, 100 μm. *p < 0.05, **p < 0.01, *** p < 0.001.
MiR-155-5p Enhanced 5-FU Resistance of OSCC In Vivo by Targeting TP53INP1
To further explore the role of TP53INP1 in the treatment of OSCC using 5-FU, we constructed OSCC nude mice through subcutaneous injection of HSC3, HSC3/5-FU, HSC3-miR-155-5p, and TP53INP1 overexpression HSC3 (HSC3-TP53INP1) cells (Figure 5A). Then, we treated the OSCC nude mice with 5-FU and found that HSC3-TP53INP1 group possessed shrinking tumors compared with HSC3/5-FU and HSC3-miR-155-5p tumor-bearing mice (Figures 5B–D).
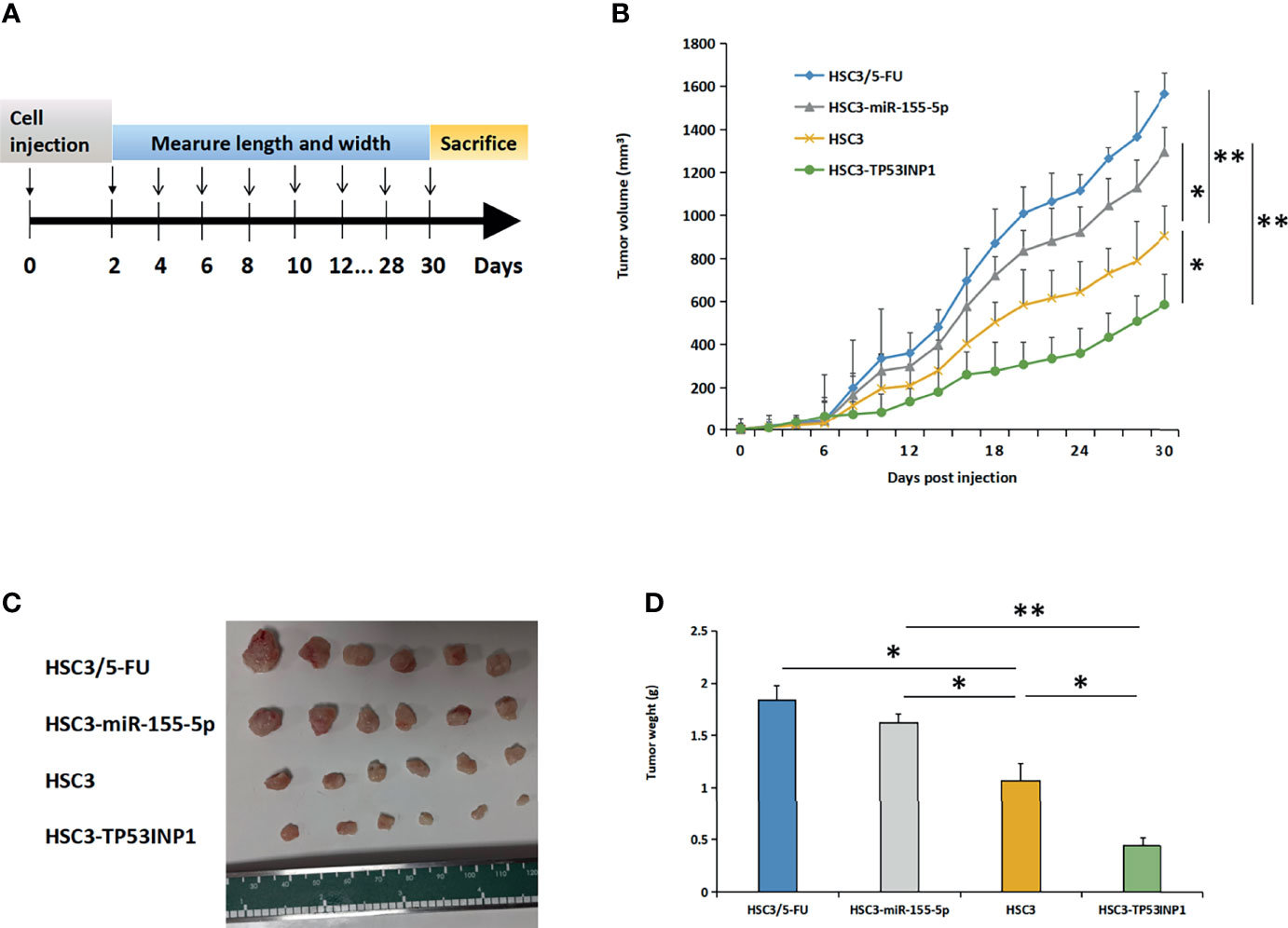
Figure 5 MiR-155-5p enhanced 5-FU resistance in OSCC mouse models by targeting TP53INP1. (A) HSC3, HSC3/5-FU, HSC3-miR-155-5p, or HSC3-TP53INP1 cells were subcutaneously injected into male athymic nude mice and were then treated with 5-FU. (B) The length and width of tumor bulks was measured every other day, tumor volume=length × width2 × π/6. Tumors were taken out, (C) photoed and (D) weighed. *p < 0.05, **p < 0.01.
Discussion
There are more than 650,000 oral cancer cases annually and OSCC accounts for >90% (41). Despite the current treatment is effective for patients at early stage, OSCC still has a high recurrence rate and causes 330,000 deaths every year (41, 42). As adjuvant chemotherapy, 5-FU is frequently used alongside surgery in patients with advanced OSCC. However, drug resistance makes tumor regression extremely difficult. And the mechanisms of the acquisition of drug resistance to 5-FU in OSCC cells are not fully understood. In this study, we constructed the 5-FU resistant cell HSC3/5-FU and explored the mechanism of miR-155-5p in the acquisition of 5-FU resistance in OSCC.
After inhibiting miR-155-5p in HSC3/5-FU cells, we found that the cell viability of 5-FU treated cells was reduced compared to the parental cells. We overexpressed miR-155-5p in HSC3 cell and processed it with 5-FU, miR-155-5p decreased sensitivity to 5-FU. In this study, we only selected the 5-FU resistant cell HSC3/5-FU, the selection results from this single cell line may not be common in OSCC. To explore whether this mechanism is universal in OSCC resistance to 5-FU, we also conducted the above-mentioned experiments in the OSCC cell line HSC4 and found consistent results. In addition to inhibiting tumor cell proliferation, it is also documented that 5-FU could suppress inhibit the migration and invasion of a variety of tumor cells (43–45). Consistent with these published researches, we found that 5-FU could also inhibit the migration and invasion of OSCC, and further experiments proved that miR-155-5p could attenuate these effects of 5-FU.
Because miRNAs function through interference with gene expression, we sought the target gene candidates of miR-155-5p using in silico analysis. TP53INP1 presented the lowest mRNA expression among the candidates in HSC3/5-FU cells, and this low expression could be improved by inhibiting miR-155-5p. MiR-155-5p overexpression suppressed the expression of TP53INP1 in both HSC3 while the rescue experiments could weaken this inhibition. Through luciferase assay and RNA pull-down, we determined the direct interference effect of miR-155-5p on TP53INP1.
Therefore, we focused on TP53INP1 for further exploration. This study showed that the TP53INP1 expression in OSCC is consistent with former research that it is often lost during cancer development from different organs (46–48). It has been documented that TP53INP1 exerts tumor suppressor function through involvement in cell death, cell-cycle arrest and cellular migration (47–49). It was found that miR-155-5p could promote epithelial-to-mesenchymal transition (EMT) through regulating TP53INP1 in paclitaxel-resistant gastric cancer cells (50). And exosomal miR-155-5p-5p could also enhance proliferation and migration capabilities of cancer cells by inhibiting TP53INP1 expression in gastric cancer (51). In addition, miR-155-5p could also promote liver cancer stem cell acquisition and self-renewal by targeting the gene TP53INP1 (52).
Up to now, there are few studies on the influence of miRNA on chemotherapy resistance through TP53INP1. It was reported that miR-182 increases cisplatin resistance in hepatocellular carcinoma cells by targeting TP53INP1 (53). Tie et al. claimed that let-7f-5p promotes 5-FU resistance and it could directly repress several pro-apoptotic proteins including TP53INP1 in colorectal cancer, indicating that TP53INP1 might negatively regulate 5-FU resistance (54). However, the regulation of TP53INP1 on 5-FU resistance still lacks direct evidence. In this study we demonstrated that miR-155-5p contributes to 5-FU through directly targeting the 3’ UTR of TP53INP1 in OSCC.
Because 5-FU could inhibit cell proliferation, migration, and invasion (55, 56), we evaluated the influence of miR-155-5p/TP53INP1 pathway on these capacities of HSC3/5-FU cells using the corresponding experiments. This study found that miR-155-5p suppressed the OSCC cell proliferation, migration and invasion through interfering with TP53INP1. Our findings confirmed that in vivo, resistance of OSCC to 5-FU was associated with the overexpression of miR-155-5p. In mice OSCC model accepting 5-FU treatment, HSC3-miR-155-5p cells-based tumor progressed rapidly, compared to that of the OSCC model based on HSC3 parent cells. Meanwhile, we demonstrated the role of TP53INP1 in improving the sensitivity of OSCC cells to 5-FU treatment. HSC3-TP53INP1 cells-based tumors were sensitive to 5-FU treatment, the tumor grew slowly, and has a good prognosis.
Our research provides novel insights into the role of miR-155-5p in the resistance of OSCC to 5-FU, which is conducive to the development of the use of miR-155-5p as a prognostic marker, or the use of anti-miR-155-5p as a treatment strategy to improve the efficacy of chemotherapy, especially 5-FU.
Materials and Methods
Cell Lines and Cell Culture
HSC3 and HSC4 cells were maintained in Dulbecco’s modified Eagle’s medium (DMEM, Invitrogen, Carlsbad, CA, USA) containing 10% fetal bovine serum (FBS) and 1% penicillin/streptomycin. The cells were cultured at 37°C in humidified air with 5% CO2.
Establishment of 5-FU-Resistant Clones
To identify 5-FU resistant cell lines, human OSCC HSC3 cells were seeded in 10 cm dishes and first incubated with medium containing 0.2 μM 5-FU (Catalog No.: 51-21-8, Sigma-Aldrich, Shanghai, China). The 5-FU concentration increased 0.5 μM every two weeks. The selection continued for 5 months, and the final IC 50 of 5-Fu in HSC3/5-FU cells was 6.945 μM.
In Vitro Cytotoxicity Tests
HCS3 or HSC4 cells were plated in triplicate at 1 × 104 cells per well in 96-well plates. Four hours later, 5-FU in different concentrations was added and incubated for 72 h.
Cell Viability Assay
Cell viability was assessed using CCK-8 assay (Catalog No.: 96992, Sigma-Aldrich), and it was conducted following manufacture’ instruction (https://www.sigmaaldrich.cn/CN/en/product/sigma/96992?context=product). In brief, cells in 24-well plates were transfected with miR-155-5p mimic, inhibitor or control miRNA and incubated with 5-FU for 72 h and then further incubated with CCK-8 for 4 h. CCK-8 assay was performed at 0, 24, 48, 72, 96 h after seeding cells. All experiments were performed at least three times.
Cell Wound-Healing Assay
Cell migration was assessed using wound-healing assay. HSC3 or HSC4 cells transfected with miR-155 grew to confluence in 24-well plates, cells were scratched using a sterile 200 μL pipette tip and maintained in serum-free medium. Images were taken at 0, 24, 48h. Wound closure was photographed along the scrape line using phase contrast microscopy (Nikon Eclipse TS100 inverted microscope). The wound distance was calculated based on the distance migrated compared to the original scratch width.
Transwell Assay
The chambers were washed thoroughly with 10 mM PBS, fixed in 4% paraformaldehyde for 30 min, and stained with 0.2% crystal violet for 10 min. Non-invading cells, from the membrane upper surface, were removed using a cotton swab. The membranes containing the invaded cells (under the surface of membrane), were photographed. Images of three random microscope fields were captured in duplicate, using an inverted optical microscope (Floid Cell Imaging Station, Life Technologies, Carlsbad, CA, USA). The areas of cell invasion were determined by Image J software.
Cell migration and invasion were analyzed using transwell culture system. To detect cell migration, 2×105 cells in 200μl serum-free DMEM were seeded into the upper chambers, and DMEM containing 20% FBS was added to the lower chamber as a chemoattractant. Following incubation at 37°C for 24 h, supernatant was discarded and cells in the membrane upper surface were removed using a cotton swab. Invasive cells located on the lower surface were fixed with paraformaldehyde (Thermo Fisher Scientific) and stained with 0.1% crystal violet (Catalog No.: V5265, Sigma-Aldrich) for 10 min at room temperature. To detect cell invasion, the inner sides of the Transwell chambers were pre-coated with Matrigel (BD Biosciences, San Jose, CA, USA) at room temperature, after which, the procedure was performed to assess cell invasion. An inverted optical microscope (Floid Cell Imaging Station, Life Technologies, Carlsbad, CA, USA) was used to count and photograph the migrated and invaded cells. The areas of cell migration and invasion were determined by Image J software (NIH, Bethesda, MD, USA).
Luciferase Reporter Assay
To confirm the binding of miR-155a-5p to TP53INP1 mRNA, a Dual-Luciferase Reporter Assay System (cat. no. E1910; Promega Corporation) was utilized according to the manufacturer’s instructions. Briefly, miR-155a-5p mimics (5’-UUACACACUAACAUUAGCAUUAA-3’) and a miR-155a-5p mutant (5’-UUACACACUAACAUUGAAUCGUA-3’) were synthesized by BioSunne Inc. (Shanghai, China). The Luciferase control, pLuci-TP53INP1 3U or pLuci-TP53INP1 3UM luciferase reporter plasmid (8 µg) and NC (GFP plasmid) or miR-155a-5p mimic plasmid (100 ppm) were transfected into HSC3 cells using Lipofectamine 3000 Transfection Reagent (Catalog No.: L3000015, Thermo Fisher Scientific.). At 48 h following transfection, the cells were lysed, and the luciferase activity was measured using the Reporter Assay System (Promega Corporation). Cotransfection experiments were carried out in triplicate.
RNA Pull-Down
The biotin-labeled miR-155-5p mimic, biotin-labeled mutated miR-155-5p and negative control were transfected into HSC3 and HSC4 cells. After 48 h, the cells were harvested and lysed, and the lysate was added to the Dynabeads™ M-280 streptavidin magnetic beads (Invitrogen, Carlsbad, CA, USA). The mixture was incubated at room temperature for 15-30 min. The enrichment of the co-deposited TP53INP1 RNA was determined using RT-PCR.
Real-Time Reverse Transcription PCR
The expression levels of miRNA and miR-155a-5p target candidate mRNA were analyzed via the real-time reverse transcription PCR (RT-PCR). Total RNAs were isolated for mRNA analysis before cDNAs were prepared via reverse transcription, The abundance of transcripts was assessed by RT-PCR analysis using LightCycler® RNA Master SYBR Green I (Roche, Basel, Switzerland) and gene-specific primer sets on a StepOne Plus instrument (Applied Biosystems, Foster City, CA, United States). The relative level of miRNA or mRNA was normalized to the U6 RNA or GAPDH mRNA using the comparative delta CT (2-ΔΔCT) method. Primer sequences are listed in Supplementary Tables 1 and 3.
Western Blotting
Whole cell lysates were prepared using RIPA buffer (Catalog No.: 89900, Thermo Fisher Scientific, Waltham, MA, USA), and the protein concentration was measured using Pierce™ BCA Protein Assay Kit (Catalog No.: 23227, Thermo Fisher Scientific). Protein extracts were separated by electrophoresis in SDS-containing polyacrylamide gels, and then transferred onto Immun-Blot® PVDF Membrane (Catalog No.: 162017, BIO-RAD, Hercules, CA, USA). The membranes were incubated with the primary rabbit monoclonal antibody against human TP53INP1 (Catalog No.: MA5-34751, Thermo Fisher Scientific) or α-tublin (Catalog No.: A11126, Thermo Fisher Scientific) and then incubated with HRP-conjugated goat anti-Rabbit IgG secondary antibody conjugated to horseradish peroxidase (Catalog No.: 31466, Thermo Fisher Scientific). GAPDH was used as an internal control.
Animal Experiments
To evaluate the roles of miR-155-5p and TP53INP1 in 5-FU resistance in OSCC, we injected HSC3, HSC3/5-FU, HSC3-miR-155-5p, or HSC3-TP53INP1 cells (1×107 cells/100 μl DMEM/mouse) subcutaneously into male athymic nude mice (6-week-old). All mice were treated with 5-FU in the concentration of 6.945 μmol/L. The volume of tumor bulks was measured per 2 days using digital calipers and were calculated using the formula: volume= length × width2 × π/6. 1 month after cell inoculation, all mice were sacrificed, and tumors were taken out and weighed. The animal studies were approved by Animal Welfare and Research Ethics Committee of Capital Medical University, and the animal experiments were conducted in accordance with the institutional and national regulations.
Statistics
All data are expressed as mean ± standard deviation. Statistical analysis was performed using GraphPad Prism 8.0 (GraphPad Software, CA, USA). Student’s t-test (two-tailed) was used to compare two groups, and analysis of variance was used to compare multiple groups. The differences were considered statistically significant when p < 0.05, and indicated as * (P < 0.05), ** (0.05< P < 0.001), and *** (P < 0.001). All experiments were performed in triplicate.
Data Availability Statement
The original contributions presented in the study are included in the article/Supplementary Material. Further inquiries can be directed to the corresponding author.
Ethics Statement
The animal study was reviewed and approved by Animal Welfare and Research Ethics Committee of Capital Medical University.
Author Contributions
BL designed the research, conducted experiments, analyzed the data, wrote the manuscript, and replied to the reviewers. JH and HZ performed in vitro experiments and analyzed data. LZ designed the research design and revised the manuscript. SP replied to the reviewers, designed research, conducted experiments, analyzed data, and revised the final manuscript. All authors contributed to the article and approved the submitted version.
Funding
This work was supported by Discipline Construction Fund from the Beijing Stomatological Hospital, School of Stomatology, Capital Medical University (17-09-09 to BL).
Conflict of Interest
The authors declare that the research was conducted in the absence of any commercial or financial relationships that could be construed as a potential conflict of interest.
Publisher’s Note
All claims expressed in this article are solely those of the authors and do not necessarily represent those of their affiliated organizations, or those of the publisher, the editors and the reviewers. Any product that may be evaluated in this article, or claim that may be made by its manufacturer, is not guaranteed or endorsed by the publisher.
Supplementary Material
The Supplementary Material for this article can be found online at: https://www.frontiersin.org/articles/10.3389/fonc.2021.706095/full#supplementary-material
References
1. Chi AC, Day TA, Neville BW. Oral Cavity and Oropharyngeal Squamous Cell Carcinoma–an Update. CA Cancer J Clin (2015) 65:401–21. doi: 10.3322/caac.21293
2. Huang SH, O’Sullivan B. Oral Cancer: Current Role of Radiotherapy and Chemotherapy. Med Oral Patol Oral Cir Bucal (2013) 18:e233–40. doi: 10.4317/medoral.18772
3. Sequeira I, Neves JF, Carrero D, Peng Q, Palasz N, Liakath-Ali K, et al. Immunomodulatory Role of Keratin 76 in Oral and Gastric Cancer. Nat Commun (2018) 9:3437. doi: 10.1038/s41467-018-05872-4
4. Li YY, Tao YW, Gao S, Li P, Zheng JM, Zhang SE, et al. Cancer-Associated Fibroblasts Contribute to Oral Cancer Cells Proliferation and Metastasis via Exosome-Mediated Paracrine MiR-34a-5p. EBioMedicine (2018) 36:209–20. doi: 10.1016/j.ebiom.2018.09.006
5. Rivera C, Oliveira AK, Costa RAP, De Rossi T, Paes Leme AF. Prognostic Biomarkers in Oral Squamous Cell Carcinoma: A Systematic Review. Oral Oncol (2017) 72:38–47. doi: 10.1016/j.oraloncology.2017.07.003
6. Longley DB, Harkin DP, Johnston PG. 5-Fluorouracil: Mechanisms of Action and Clinical Strategies. Nat Rev Cancer (2003) 3:330–8. doi: 10.1038/nrc1074
7. Leemans CR, Braakhuis BJ, Brakenhoff RH. The Molecular Biology of Head and Neck Cancer. Nat Rev Cancer (2011) 11:9–22. doi: 10.1038/nrc2982
8. Ali Syeda Z, Langden SSS, Munkhzul C, Lee M, Song SJ. Regulatory Mechanism of Microrna Expression in Cancer. Int J Mol Sci (2020) 21(5):1723. doi: 10.3390/ijms21051723
9. Lai X, Eberhardt M, Schmitz U, Vera J. Systems Biology-Based Investigation of Cooperating MicroRNAs as Monotherapy or Adjuvant Therapy in Cancer. Nucleic Acids Res (2019) 47:7753–66. doi: 10.1093/nar/gkz638
10. Harrandah AM, Mora RA, Chan EKL. Emerging MicroRNAs in Cancer Diagnosis, Progression, and Immune Surveillance. Cancer Lett (2018) 438:126–32. doi: 10.1016/j.canlet.2018.09.019
11. Rather MI, Nagashri MN, Swamy SS, Gopinath KS, Kumar A. Oncogenic MicroRNA-155 Down-Regulates Tumor Suppressor CDC73 and Promotes Oral Squamous Cell Carcinoma Cell Proliferation: Implications for Cancer Therapeutics. J Biol Chem (2013) 288:608–18. doi: 10.1074/jbc.M112.425736
12. Catela Ivkovic T, Voss G, Cornella H, Ceder Y. MicroRNAs as Cancer Therapeutics: A Step Closer to Clinical Application. Cancer Lett (2017) 407:113–22. doi: 10.1016/j.canlet.2017.04.007
13. Zeng Q, Tao X, Huang F, Wu T, Wang J, Jiang X, et al. Overexpression of MiR-155 Promotes the Proliferation and Invasion of Oral Squamous Carcinoma Cells by Regulating BCL6/Cyclin D2. Int J Mol Med (2016) 37:1274–80. doi: 10.3892/ijmm.2016.2529
14. Baba O, Hasegawa S, Nagai H, Uchida F, Yamatoji M, Kanno NI, et al. MicroRNA-155-5p is Associated With Oral Squamous Cell Carcinoma Metastasis and Poor Prognosis. J Oral Pathol Med (2016) 45:248–55. doi: 10.1111/jop.12351
15. Yang LW, Wu XJ, Liang Y, Ye GQ, Che YC, Wu XZ, et al. MiR-155 Increases Stemness and Decitabine Resistance in Triple-Negative Breast Cancer Cells by Inhibiting TSPAN5. Mol Carcinog (2020) 59:447–61. doi: 10.1002/mc.23167
16. Gao Y, Liu Z, Ding Z, Hou S, Li J, Jiang K. MicroRNA-155 Increases Colon Cancer Chemoresistance to Cisplatin by Targeting Forkhead Box O3. Oncol Lett (2018) 15:4781–8. doi: 10.3892/ol.2018.7976
17. Bayraktar R, Van Roosbroeck K. MiR-155 in Cancer Drug Resistance and as Target for MiRNA-Based Therapeutics. Cancer Metastasis Rev (2018) 37:33–44. doi: 10.1007/s10555-017-9724-7
18. La X, Zhang L, Li Z, Li H, Yang Y. (-)-Epigallocatechin Gallate (EGCG) Enhances the Sensitivity of Colorectal Cancer Cells to 5-FU by Inhibiting GRP78/NF-Kappab/MiR-155-5p/MDR1 Pathway. J Agric Food Chem (2019) 67:2510–8. doi: 10.1021/acs.jafc.8b06665
19. Li Y, Tian Z, Tan Y, Lian G, Chen S, Chen S, et al. Bmi-1-Induced MiR-27a and MiR-155 Promote Tumor Metastasis and Chemoresistance by Targeting RKIP in Gastric Cancer. Mol Cancer (2020) 19:109. doi: 10.1186/s12943-020-01229-y
20. Liu B, Liu Y, Zhao L, Pan Y, Shan Y, Li Y, et al. Upregulation of MicroRNA-135b and MicroRNA-182 Promotes Chemoresistance of Colorectal Cancer by Targeting ST6GALNAC2 via PI3K/AKT Pathway. Mol Carcinog (2017) 56:2669–80. doi: 10.1002/mc.22710
21. Zhang Y, Talmon G, Wang J. MicroRNA-587 Antagonizes 5-FU-Induced Apoptosis and Confers Drug Resistance by Regulating PPP2R1B Expression in Colorectal Cancer. Cell Death Dis (2015) 6:e1845. doi: 10.1038/cddis.2015.200
22. Li X, Li X, Liao D, Wang X, Wu Z, Nie J, et al. Elevated MicroRNA-23a Expression Enhances the Chemoresistance of Colorectal Cancer Cells With Microsatellite Instability to 5-Fluorouracil by Directly Targeting ABCF1. Curr Protein Pept Sci (2015) 16:301–9. doi: 10.2174/138920371604150429153309
23. Yu X, Shi W, Zhang Y, Wang X, Sun S, Song Z, et al. CXCL12/CXCR4 Axis Induced MiR-125b Promotes Invasion and Confers 5-Fluorouracil Resistance Through Enhancing Autophagy in Colorectal Cancer. Sci Rep (2017) 7:42226. doi: 10.1038/srep42226
24. Amankwatia EB, Chakravarty P, Carey FA, Weidlich S, Steele RJ, Munro AJ, et al. MicroRNA-224 Is Associated With Colorectal Cancer Progression and Response to 5-Fluorouracil-Based Chemotherapy by KRAS-Dependent and -Independent Mechanisms. Br J Cancer (2015) 112:1480–90. doi: 10.1038/bjc.2015.125
25. Liang G, Zhu Y, Ali DJ, Tian T, Xu H, Si K, et al. Engineered Exosomes for Targeted Co-Delivery of MiR-21 Inhibitor and Chemotherapeutics to Reverse Drug Resistance in Colon Cancer. J Nanobiotech (2020) 18:10. doi: 10.1186/s12951-019-0563-2
26. Ye L, Jiang T, Shao H, Zhong L, Wang Z, Liu Y, et al. MiR-1290 is a Biomarker in DNA-Mismatch-Repair-Deficient Colon Cancer and Promotes Resistance to 5-Fluorouracil by Directly Targeting Hmsh2. Mol Ther Nucleic Acids (2017) 7:453–64. doi: 10.1016/j.omtn.2017.05.006
27. Zhang Y, Hu X, Miao X, Zhu K, Cui S, Meng Q, et al. MicroRNA-425-5p Regulates Chemoresistance in Colorectal Cancer Cells via Regulation of Programmed Cell Death 10. J Cell Mol Med (2016) 20:360–9. doi: 10.1111/jcmm.12742
28. Nishida N, Yamashita S, Mimori K, Sudo T, Tanaka F, Shibata K, et al. MicroRNA-10b is a Prognostic Indicator in Colorectal Cancer and Confers Resistance to the Chemotherapeutic Agent 5-Fluorouracil in Colorectal Cancer Cells. Ann Surg Oncol (2012) 19:3065–71. doi: 10.1245/s10434-012-2246-1
29. Ren D, Lin B, Zhang X, Peng Y, Ye Z, Ma Y, et al. Maintenance of Cancer Stemness by MiR-196b-5p Contributes to Chemoresistance of Colorectal Cancer Cells via Activating STAT3 Signaling Pathway. Oncotarget (2017) 8:49807–23. doi: 10.18632/oncotarget.17971
30. Xu W, Jiang H, Zhang F, Gao J, Hou J. MicroRNA-330 Inhibited Cell Proliferation and Enhanced Chemosensitivity to 5-Fluorouracil in Colorectal Cancer by Directly Targeting Thymidylate Synthase. Oncol Lett (2017) 13:3387–94. doi: 10.3892/ol.2017.5895
31. Xu F, Ye ML, Zhang YP, Li WJ, Li MT, Wang HZ, et al. MicroRNA-375-3p Enhances Chemosensitivity to 5-Fluorouracil by Targeting Thymidylate Synthase in Colorectal Cancer. Cancer Sci (2020) 111:1528–41. doi: 10.1111/cas.14356
32. Li T, Gao F, Zhang XP. MiR-203 Enhances Chemosensitivity to 5-Fluorouracil by Targeting Thymidylate Synthase in Colorectal Cancer. Oncol Rep (2015) 33:607–14. doi: 10.3892/or.2014.3646
33. Li PL, Zhang X, Wang LL, Du LT, Yang YM, Li J, et al. MicroRNA-218 is a Prognostic Indicator in Colorectal Cancer and Enhances 5-Fluorouracil-Induced Apoptosis by Targeting BIRC5. Carcinogenesis (2015) 36:1484–93. doi: 10.1093/carcin/bgv145
34. Liu H, Yin Y, Hu Y, Feng Y, Bian Z, Yao S, et al. MiR-139-5p Sensitizes Colorectal Cancer Cells to 5-Fluorouracil by Targeting NOTCH-1. Pathol Res Pract (2016) 212:643–9. doi: 10.1016/j.prp.2016.04.011
35. Karaayvaz M, Zhai H, Ju J. MiR-129 Promotes Apoptosis and Enhances Chemosensitivity to 5-Fluorouracil in Colorectal Cancer. Cell Death Dis (2013) 4:e659. doi: 10.1038/cddis.2013.193
36. Boni V, Bitarte N, Cristobal I, Zarate R, Rodriguez J, Maiello E, et al. MiR-192/MiR-215 Influence 5-Fluorouracil Resistance Through Cell Cycle-Mediated Mechanisms Complementary to its Post-Transcriptional Thymidilate Synthase Regulation. Mol Cancer Ther (2010) 9:2265–75. doi: 10.1158/1535-7163.MCT-10-0061
37. Chen Y, Wang X. MiRdb: An Online Database for Prediction of Functional MicroRNA Targets. Nucleic Acids Res (2020) 48:D127–31. doi: 10.1093/nar/gkz757
38. Liu W, Wang X. Prediction of Functional MicroRNA Targets by Integrative Modeling of MicroRNA Binding and Target Expression Data. Genome Biol (2019) 20:18. doi: 10.1186/s13059-019-1629-z
39. Agarwal V, Bell GW, Nam JW, Bartel DP. Predicting Effective MicroRNA Target Sites in Mammalian MRNAs. Elife (2015) 4:e05005. doi: 10.7554/eLife.05005
40. Sticht C, de la Torre C, Parveen A, Gretz N. Mirwalk: An Online Resource for Prediction of MicroRNA Binding Sites. PloS One (2018) 13:e0206239. doi: 10.1371/journal.pone.0206239
41. Siegel RL, Miller KD, Jemal A. Cancer Statistics, 2018. CA Cancer J Clin (2018) 68:7–30. doi: 10.3322/caac.21442
42. Okura M, Aikawa T, Sawai NY, Iida S, Kogo M. Decision Analysis and Treatment Threshold in a Management for the N0 Neck of the Oral Cavity Carcinoma. Oral Oncol (2009) 45:908–11. doi: 10.1016/j.oraloncology.2009.03.013
43. Ding XQ, Zhao S, Wang JY, Zheng HC, Ma CM. Inhibitory Effects and Molecular Mechanisms of Pentagalloyl Glucose in Combination With 5-FU on Aggressive Phenotypes of Hepg2 Cells. Nat Prod Res (2021) 35:815–8. doi: 10.1080/14786419.2019.1598991
44. Korourian A, Madjd Z, Roudi R, Shariftabrizi A, Soleimani M. Induction of MiR-31 Causes Increased Sensitivity to 5-FU and Decreased Migration and Cell Invasion in Gastric Adenocarcinoma. Bratisl Lek Listy (2019) 120:35–9. doi: 10.4149/BLL_2019_005
45. Russo A, Saide A, Cagliani R, Cantile M, Botti G, Russo G. Rpl3 Promotes the Apoptosis of P53 Mutated Lung Cancer Cells by Down-Regulating CBS and Nfkappab Upon 5-FU Treatment. Sci Rep (2016) 6:38369. doi: 10.1038/srep38369
46. Zidi B, Vincent-Fabert C, Pouyet L, Seillier M, Vandevelde A, N’Guessan P, et al. TP53INP1 Deficiency Maintains Murine B Lymphopoiesis in Aged Bone Marrow Through Redox-Controlled IL-7R/STAT5 Signaling. Proc Natl Acad Sci USA (2019) 116:211–6. doi: 10.1073/pnas.1809980116
47. Li N, Cui T, Guo W, Wang D, Mao L. MiR-155-5p Accelerates the Metastasis of Cervical Cancer Cell via Targeting TP53INP1. Onco Targets Ther (2019) 12:3181–96. doi: 10.2147/OTT.S193097
48. Ye W, Liang F, Ying C, Zhang M, Feng D, Jiang X. Downregulation of MicroRNA-3934-5p Induces Apoptosis and Inhibits the Proliferation of Neuroblastoma Cells by Targeting TP53INP1. Exp Ther Med (2019) 18:3729–36. doi: 10.3892/etm.2019.8007
49. Yu SJ, Yang L, Hong Q, Kuang XY, Di GH, Shao ZM. MicroRNA-200a Confers Chemoresistance by Antagonizing TP53INP1 and YAP1 in Human Breast Cancer. BMC Cancer (2018) 18:74. doi: 10.1186/s12885-017-3930-0
50. Wang M, Qiu R, Yu S, Xu X, Li G, Gu R, et al. Paclitaxelresistant Gastric Cancer MGC803 Cells Promote Epithelialtomesenchymal Transition and Chemoresistance in Paclitaxelsensitive Cells via Exosomal Delivery of MiR1555p. Int J Oncol (2019) 54:326–38. doi: 10.3892/ijo.2018.4601
51. Shi SS, Zhang HP, Yang CQ, Li LN, Shen Y, Zhang YQ. Exosomal MiR-155-5p Promotes Proliferation and Migration of Gastric Cancer Cells by Inhibiting TP53INP1 Expression. Pathol Res Pract (2020) 216:152986. doi: 10.1016/j.prp.2020.152986
52. Liu F, Kong X, Lv L, Gao J. MiR-155 Targets TP53INP1 to Regulate Liver Cancer Stem Cell Acquisition and Self-Renewal. FEBS Lett (2015) 589:500–6. doi: 10.1016/j.febslet.2015.01.009
53. Qin J, Luo M, Qian H, Chen W. Upregulated MiR-182 Increases Drug Resistance in Cisplatin-Treated HCC Cell by Regulating TP53INP1. Gene (2014) 538:342–7. doi: 10.1016/j.gene.2013.12.043
54. Tie Y, Chen C, Yang Y, Qian Z, Yuan H, Wang H, et al. Upregulation of Let-7f-5p Promotes Chemotherapeutic Resistance in Colorectal Cancer by Directly Repressing Several Pro-Apoptotic Proteins. Oncol Lett (2018) 15:8695–702. doi: 10.3892/ol.2018.8410
55. Francipane MG, Bulanin D, Lagasse E. Establishment and Characterization of 5-Fluorouracil-Resistant Human Colorectal Cancer Stem-Like Cells: Tumor Dynamics Under Selection Pressure. Int J Mol Sci (2019) 20(8):1817. doi: 10.3390/ijms20081817
Keywords: microRNA-155-5p, 5-fluorouracil, resistance, TP53INP1, oral squamous cell carcinoma
Citation: Liu B, Hu J, Zhao H, Zhao L and Pan S (2022) MicroRNA-155-5p Contributes to 5-Fluorouracil Resistance Through Down-Regulating TP53INP1 in Oral Squamous Cell Carcinoma. Front. Oncol. 11:706095. doi: 10.3389/fonc.2021.706095
Received: 06 May 2021; Accepted: 15 December 2021;
Published: 06 January 2022.
Edited by:
Jan Baptist Vermorken, University of Antwerp, BelgiumCopyright © 2022 Liu, Hu, Zhao, Zhao and Pan. This is an open-access article distributed under the terms of the Creative Commons Attribution License (CC BY). The use, distribution or reproduction in other forums is permitted, provided the original author(s) and the copyright owner(s) are credited and that the original publication in this journal is cited, in accordance with accepted academic practice. No use, distribution or reproduction is permitted which does not comply with these terms.
*Correspondence: Shiyuan Pan, yifan516_2006@163.com