- 1Department of Colorectal Surgery, The Second Affiliated Hospital of South China University of Technology, Guangzhou, China
- 2School of Medicine, South China University of Technology, Guangzhou, China
- 3Guangdong Provincial People’s Hospital, Guangdong Academy of Medical Sciences, Guangzhou, China
- 4Department of Thoracic Surgery, Guangdong Provincial People’s Hospital, Guangdong Academy of Medical Sciences, Guangzhou, China
Objective: The mesentery is a potential site of residual tumor in patients with colorectal cancer (CRC). However, the mesenteric immune microenvironment remains unclear. In this study, we investigated the immune landscape of the mesentery, particularly the role of lymphocytes and its association with the clinicopathological characteristics of CRC.
Methods: Flow cytometry was used to detect lymphocytes in the paired mesenteric tissue specimens adjacent to the colorectal tumors and normal mesenteric tissue specimens 10 cm away from the colorectal tumor edge and preoperative peripheral blood samples obtained from patients with CRC who underwent surgery. T-distributed stochastic neighbor embedding was utilized to analyze multiparameter flow cytometry data. Multiplex immunohistochemistry was performed to evaluate T cells subsets in the paired mesentery adjacent to the colorectal tumors and normal mesentery. The Fisher’s exact test and non-parametric Wilcoxon’s matched-pairs tests were used for statistical analysis. The non-parametric Mann-Whitney U test was used to determine associations between percentage data and clinical parameters of patients with CRC.
Results: We found that immune cells in the normal mesentery were mainly of lymphoid lineage. Compared with peripheral blood, the normal mesentery showed decreased NK cells and the CD4/CD8 ratio and increased CD3+ CD56+, memory CD4+ T, memory CD8+ T, CD4+ tissue-resident memory T (TRM), and CD8+ TRM cells. Compared with the normal mesentery, the mesentery adjacent to the colorectal tumor showed increased B and regulatory T cells and decreased NK, CD3+ CD56+, CD4+ TRM, and CD8+ TRM cells. Moreover, memory CD8+ T cells and plasmablasts are negatively correlated with the depth of invasion of CRC. Increased memory CD4+ T cells are associated with distant metastasis of CRC and high preoperative serum carcinoembryonic antigen levels.
Conclusion: The mesentery shows a specific immune microenvironment, which differs from that observed in peripheral blood. CRC can alter the mesenteric immune response to promote tumor progression.
Introduction
Colorectal cancer (CRC) is one of the most common malignant tumors, with an estimated 1.8 million new cases worldwide in 2018 and ranks third with regard to incidence and second with regard to mortality (1). Studies have shown that postoperative recurrence was associated with cancer-related death in most cases (2–4), and mortality rates were 3.47-fold higher in patients with CRC recurrence than in those who were recurrence-free (5). An intraoperative residual tumor is one of the main contributors to postoperative local CRC recurrence (6). The mesentery is a continuous membrane that attaches the intestines to the abdominal wall and is considered a potential site of residual tumor (7, 8). Metastatic cancer cells were detected in the colorectal mesentery in approximately 20% of patients with CRC, who underwent radical surgery (9, 10), and patients with mesenteric metastasis tend to show poor cumulative survival rates (11, 12).
Currently, the mesentery is classified as an organ that contains not only adipocytes but also blood vessels, lymphatics, nerve tissues, immune cells, and connective tissue matrix (13). Reportedly, mesenteric immune cells play a significant role in the pathogenesis and disease progression of intestinal diseases, particularly Crohn’s disease (14–17). Under steady-state conditions, the mesentery shows large numbers of anti-inflammatory cells, including M2 macrophages and regulatory T (Treg) cells (17). However, following intestinal inflammation, most immune cells detected in the mesenteric adipose tissue comprise pro-inflammatory cells, such as M1 macrophages (17). Moreover, compared with controls, CD68- and CD163-positive cells were shown to be significantly increased in the mesentery in patients with CRC (18). However, lymphocyte composition and phenotype in the mesenteric immune microenvironment remain unclear.
In this study, we investigated the lymphocyte landscape of the mesentery and the association between lymphocytic infiltration of the mesentery adjacent to the tumors and clinicopathological characteristics of patients with CRC. We observed that colorectal tumors can alter the lymphocyte phenotype of the mesentery. Memory CD8+ T cells and plasmablasts were negatively correlated with the depth of invasion of CRC, and memory CD4+ T cells were associated with distant metastasis of CRC, which highlight the role of the mesentery in CRC.
Material And Methods
Specimen Acquisition
We obtained 32 paired mesenteric tissue specimens adjacent to the colorectal tumors (MES T) and normal mesenteric tissue specimens (MES N) 10 cm away from the colorectal tumor edge in patients with CRC who underwent surgery between 2018 and 2020 at the Department of General Surgery, Guangzhou Digestive Disease Center, Guangzhou First People’s Hospital (Guangzhou, Guangdong, China). Peripheral blood (PB) samples were obtained from 13 of these patients preoperatively. Demographic and clinicopathological characteristics of 32 patients with CRC are shown in Table 1. Patients with a history of inflammatory bowel disease were excluded. The study protocol was approved by the Guangzhou First People’s Hospital Ethics Review Committee, and written informed consent was obtained from all patients. All specimens were anonymized.
Tissue Digestion and Cell Isolation
Mesenteric tissues were dissected, and lymph nodes and blood vessels were carefully removed. The remaining mesenteric tissues were washed with 1× phosphate-buffered saline containing 0.2% bovine serum albumin and cut into small fragments in a petri dish. Subsequently, tissues were treated with a mixture containing 10 mL of Dulbecco’s Modified Eagle Medium (DMEM)/F12 1:1 (1×) (HyClone, Logan, UT, USA) mixed with 1 mg/mL of collagenase II (Sigma, St. Louis, MO) for 30 min at 37°C in a shaking incubator at 200 rpm. Cell suspensions were passed through a nylon mesh (74 μm) and centrifuged at 450 ×g for 5 min at room temperature. Then, cells were mixed with 2 mL red blood cell lysis buffer (Beyotime, Shanghai, China) for 10 min at 4°C for depletion of red blood cells, and centrifuged at 450 ×g for 5 min at room temperature. PB samples (2 mL) were collected in a tube containing ethylenediaminetetraacetic acid, and plasma was removed after centrifugation at 450 ×g for 5 min. An equal volume of physiological saline was added to resuspend the cell pellet. Cell suspensions were transferred to a new tube containing 3 mL Ficoll (Lymphoprep, Alere Technologies AS, Oslo, Norway) for density centrifugation according to the manufacturer’s instructions. Mononuclear cells (MNCs) were collected at the interface after density centrifugation. Cells were counted after they were treated with trypan blue stain.
Flow Cytometry
Single-cell suspensions were initially incubated with the human Fc receptor blocking reagent and stained for 20 min at 4°C using a cocktail of fluorochrome-conjugated antibodies. Antibodies included anti-TCRVα7.2 PE/Cy7 (3C10), anti-CD161 PE/Dazzle 594 (HP-3G10), anti-CD25 PE/Cy5 (BC96), anti-CD69 PE (FN50), anti-CD56 BV605 (5.1H11), anti-CD45RO BV421 (UCHL1), anti-CD45RA BV510 (HI100), anti-CD19 BV785 (HIB19), anti-CD3 BV711 (OKT3), anti-CD103 APC (Ber-ACT8), anti-CD8α Alexa Fluor 700 (HIT8a), anti-CD45 APC/Cy7 (HI30), anti-CD127 BUV737 (HIL-7R-M21), anti-CD4 BUV563 (SK3), anti-CD38 FITC (HIT2), anti-immunoglobulin (Ig)A PE (polyclonal), anti-IgG BV421 (G18-145), anti-IgD APC (IA6-2), anti-IgM Alexa Fluor 700 (MHM-88), and anti-CD19 BUV563 (SJ25C1). All antibodies were purchased from BioLegend, except for anti-CD127 BUV737 (BD Biosciences), anti-CD4 BUV563 (BD Biosciences), anti-IgG BV421 (BD Biosciences), anti-CD19 BUV563 (BD Biosciences), and anti-IgA PE (SouthernBiotech). Following treatment with surface marker stains, cells were fixed and permeabilized using the Foxp3/Transcription Factor Staining Buffer Set (eBioscience, San Diego, CA, USA) to detect the transcription factor Foxp3 (anti-Foxp3 PE, 206D, BioLegend). Data were obtained using the BD LSRFortessa™ flow cytometer (BD Biosciences, San Jose, CA, USA) and analyzed using FlowJo software (BD Biosciences, San Jose, CA, USA).
T-Distributed Stochastic Neighbor Embedding Analysis of Multiparameter Flow Cytometry Data
We gated the CD45+ MNCs in the FlowJo software and exported the expression matrices of three different samples from peripheral blood mononuclear cells (PBMCs), MES N, and the MES T to perform t-distributed stochastic neighbor embedding (t-SNE) analysis of multiparameter flow cytometry data. Thereafter, we randomly selected 8400 cells from each matrices and merged these into a single matrix in the R software (version 3.5.3). We normalized the matrix by channel using a centered log ratio transformation method embedded in the NormalizeData function of the Seurat package (version 3.1.5). The t-SNE algorithm was run with the normalized expression matrix using the RunTSNE function of the Seurat package. Dimensionality reduction of 4300 CD45+ CD3+ CD56- MNCs and 2250 CD45+ CD19+ MNCs from PBMCs, MES N, and MES T, respectively, was performed in a similar manner.
Multiplex Immunohistochemistry Staining
PANO 7-plex IHC kit (TSA-RM) (Panovue, 0004100100) was used for multiplex immunohistochemistry (mIHC) staining. 8 paired formalin-fixed paraffin-embedded (FFPE) MES N and MES T slides from patients with CRC were dewaxed and rehydrated. After antigen retrieval, slides were blocked with goat serum at room temperature (RT) for 20 min. The primary antibody, CD69 (1:500, ab233396, Abcam) was incubated overnight at 4°C. Slides were washed and incubated with horseradish peroxidase-conjugated secondary antibody (Panovue, 0004100100) at RT for 10 min. Finally, tyramide signal amplification (TSA) dye620 (1:100) was applied for 10 min after washed. The second antibody, CD4 (1:500, ab133616, Abcam) was incubated at RT for 2 h. And then, TSA dye690 (1:100) was applied. The third antibody, Foxp3 (1:100, 98377, CST) was incubated overnight at 4°C, and TSA dye570 (1:100) was applied. The last antibody, CD8α (1:400, 70306, CST) was incubated at RT for 2 h, and TSA dye520 (1:100) was applied. Nuclei were stained with DAPI (Beyotime, C1006) at RT for 5 min. Slides were imaged and scanned using a Vectra Polaris multispectral imaging system (Akoya Biosciences), and multispectral images were acquired using Phenochart software, version 1.0.12 (Akoya Biosciences) to unmix and remove autofluorescence. And slides were imported into HALO software version 3.2 (Indica Labs, Corrales, CA) for all subsequent steps, including annotation, training, and classification of multispectral slides.
Statistical Analysis
Data are presented as mean ± standard deviation. Statistical differences were determined using the Fisher’s exact test and non-parametric Wilcoxon’s matched-pairs tests. The non-parametric Mann-Whitney U test was used to determine the associations between percentage data and clinical parameters. All analyses were performed using the GraphPad PRISM software, version 8 (GraphPad Software, San Diego, CA), SPSS software, version 25.0 (SPSS Inc., Chicago, USA), and the R software, version 3.5.3. All p values were two-sided, and p<0.05 was considered statistically significant.
Results
Demographics and Clinicopathological Characteristics
The study included 32 patients (17 men and 15 women) with CRC (mean age 61.2 years) (Table 1). Notably, 23 patients were aged ≥55 years and 9 were aged <55 years. CRC lesions were located at the following sites: ascending colon (10 cases), transverse colon (5 cases), descending colon (2 cases), sigmoid colon (11 cases), and rectum (4 cases). The maximum tumor diameter was ≥4 cm in 19 and <4 cm in 13 patients, respectively. The 8th edition of the American Joint Committee on Cancer tumor, node, and metastasis (TNM) staging system was used in our study. The distribution of tumors based on the T stage was as follows: T2 stage (3 patients), T3 stage (14), and T4 stage (15). Distribution of tumors per the N stage was as follows: N0 stage (17 patients), N1 stage (9), and N2 stage (6). The distribution of tumors per the M stage was as follows: M0 stage (17 patients), M1 stage (5), and Mx stage (10). The preoperative serum carcinoembryonic antigen (CEA) levels were ≥5 ng/mL and <5 ng/mL in 11 and 21 patients, respectively. We observed no statistical differences in gender and age (≥55 years and <55 years) between patients with CRC showing different TNM stages (T2-3 stages vs.T4 stage, N0 stage vs. N1-2 stages, M0 stage vs. M1 stage) and preoperative serum CEA levels (≥5 ng/mL and <5 ng/mL) (Table S1).
A Specific Immune Microenvironment Exists in the Mesentery
We identified six immune cell clusters among the CD45+ MNCs, including mucosal associated invariant T (MAIT) cells, T cells, NK cells, CD3+ CD56+ cells, B cells, and other cells (monocytes/macrophages and dendritic cells) (Figure S1A). MAIT cells, T cells, NK cells, and B cells were identified as CD3+ TCRVα7.2+ CD161+, CD3+ CD56-, CD3- CD56+, and CD19+, respectively (Figure S1B). Based on the t-SNE plot of CD45+ MNCs, PBMCs and MES N differed significantly with regard to the compositions of T cells, NK cells, CD3+ CD56+ cells, and B cells (Figure 1A). Flow cytometry analysis showed that the percentage of NK cells was significantly lower in the MES N than in the PBMCs, and the percentage of CD3+ CD56+ cells was significantly higher than those of PBMCs (Figures 1B and S2).
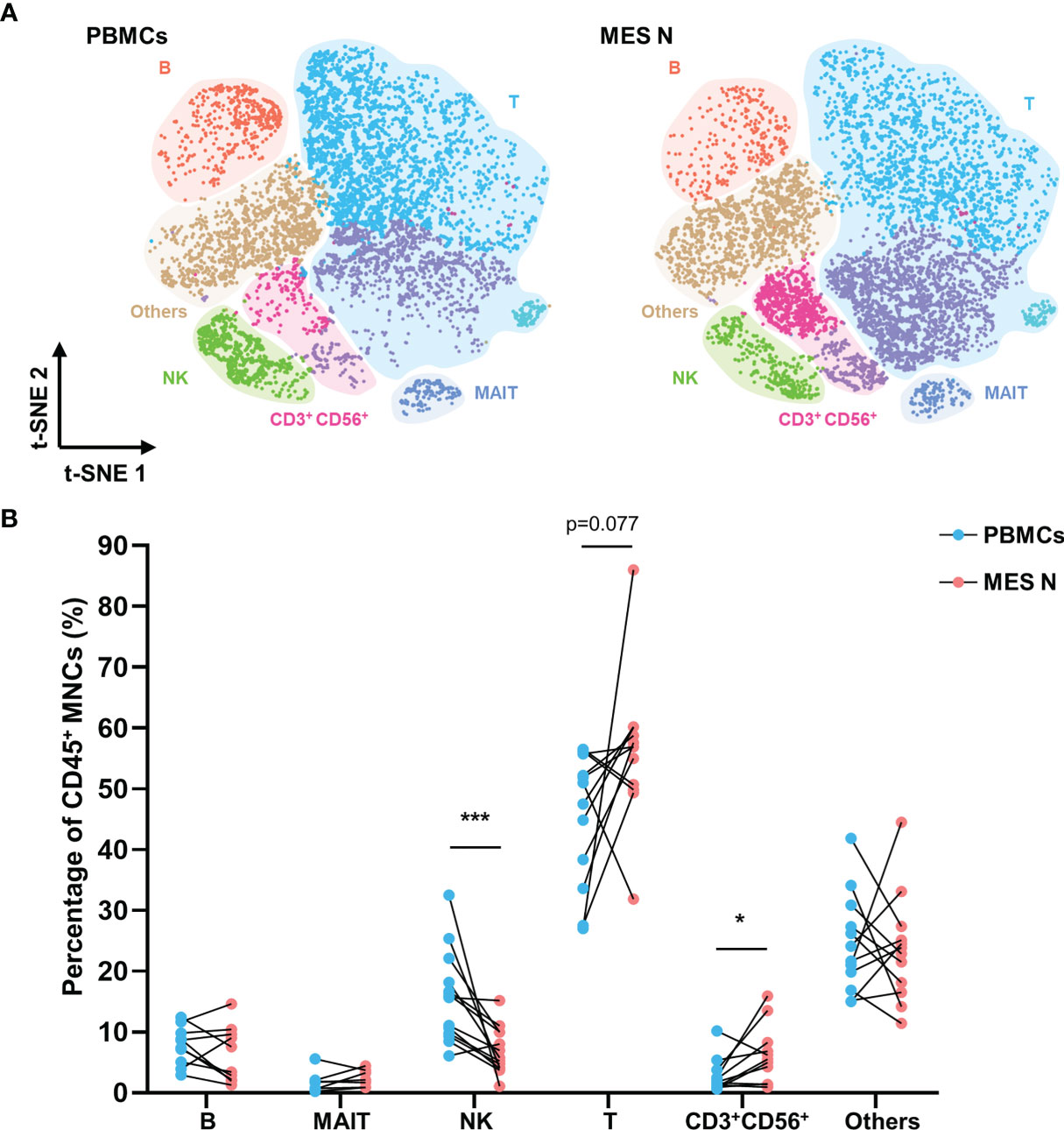
Figure 1 T-SNE plot and percentages of CD45+ MNCs in the PBMCs and MES N. (A) T-SNE plot of CD45+ MNCs in the PBMCs and MES N with main subclusters indicated. (B) Percentages of B cells, MAIT cells, NK cells, T cells, CD3+ CD56+ cells, and other cells (monocytes/macrophages and dendritic cells) in the PBMCs and MES N. (*p < 0.05; ***p < 0.001).
We categorized T cells into subclusters and identified each subcluster using several T cell-specific surface markers including CD4, CD8, CD45RO, CD45RA, CD69, CD103, CD25, and CD127 (Figures S1C, D). Memory CD4+ T cells, naïve CD4+ T cells, memory CD8+ T cells, naïve CD8+ T cells, and double-negative (DN) cells were identified as CD4+ CD45RO+, CD4+ CD45RA+, CD8+ CD45RO+, CD8+ CD45RA+, and CD4- CD8-, respectively, based on expression levels of these specific markers. Treg cells were defined as those showing CD4+ CD25+ CD127- expression (Figure S1D). Studies have reported that CD69 expression can indicate early activation of T cells, as well as their retention in tissues (19, 20) and that tissue-resident memory T (TRM) cells manifest upregulated CD69 expression, maintaining tissue resident and cytotoxic features (21–24). CD8+ CD69+ T cells have been shown to be more cytotoxic (25). In our study, CD4+ TRM and CD8+ TRM cells were phenotypically defined as those showing CD4+ CD45RO+ CD69+ and CD8+ CD45RO+ CD69+ expression, respectively (Figure S1D). As shown in Figure 2A, CD69 was expressed in T cells from the MES N but rarely in those from PBMCs. The CD4/CD8 ratio was significantly lower in the MES N than in the PBMCs; however, the percentages of memory CD8+ T cells, CD8+ TRM cells, memory CD4+ T cells, and CD4+ TRM cells were significantly higher in the MES N (Figures 2B and S2).
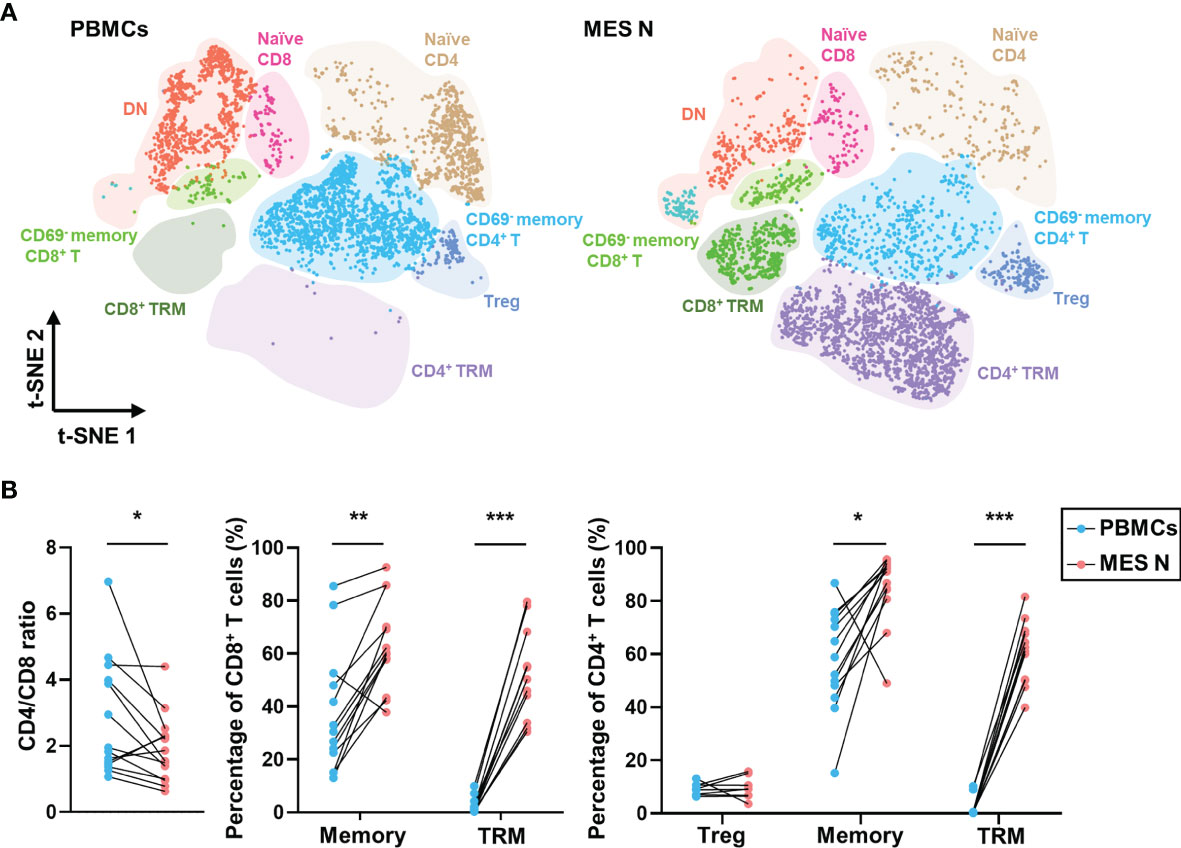
Figure 2 T-SNE plot and percentages of T cells in the PBMCs and MES N. (A) T-SNE plot of T cells in the PBMCs and MES N with main subclusters indicated. (B) CD4/CD8 ratio, percentages of memory CD8+ T cells, CD8+ TRM cells, Treg cells, memory CD4+ T cells, and CD4+ TRM cells in the PBMCs and MES N. (*p < 0.05; **p < 0.01; ***p < 0.001).
Based on CD38 and IgD expression levels (26), B cells can be clustered into plasmablasts (CD38+ IgD-), unswitched B cells (CD38- IgD+), and memory B cells (CD38- IgD-) (Figures S1E, F and S4A). B cells can undergo class-switch recombination from initial expression of membrane-bound IgM and IgD to membrane-bound IgG, IgA, or IgE in response to antigen stimulation (27, 28). As shown in the t-SNE plot of B cells, no significant differences were observed between PBMCs and MES N with regard to the compositions of plasmablasts, unswitched B cells, and memory B cells (Figure S5A). However, differences can be observed in the expression levels of IgM, IgA, and IgG in B cells between PBMCs and MES N. Flow cytometry analysis showed no statistically significant differences in B cell subclusters between PBMCs and the MES N; however, the percentages of unswitched B cells and IgM+ unswitched B cells in the MES N showed a downward trend, and the percentage of IgA+ memory B cells in the MES N showed an upward trend (Figures S4A and S5B). Therefore, our data confirm the existence of a specific mesenteric immune microenvironment, which differs from that observed in the PB.
Decreased Tissue-Resident Memory T Cells and Increased Regulatory T Cells in the Mesentery Adjacent to the Colorectal Tumor
In view of its function as a conduit of intestinal blood and lymph, the mesentery is known to be involved in a variety of intestinal diseases. However, the effects of colorectal tumors on the mesenteric immune phenotype remain unclear. We investigated the immune landscape of the MES T. The t-SNE plot showed that the MES N and MES T differed with regard to the compositions of T cells, NK cells, CD3+ CD56+ cells, and B cells (Figure 3A). Flow cytometry data analysis revealed that the percentage of B cells was significantly higher and the percentages of NK cells, CD3+ CD56+ cells, and other cells (monocytes/macrophages and dendritic cells) were significantly lower in the MES T than in the MES N (Figure 3B).
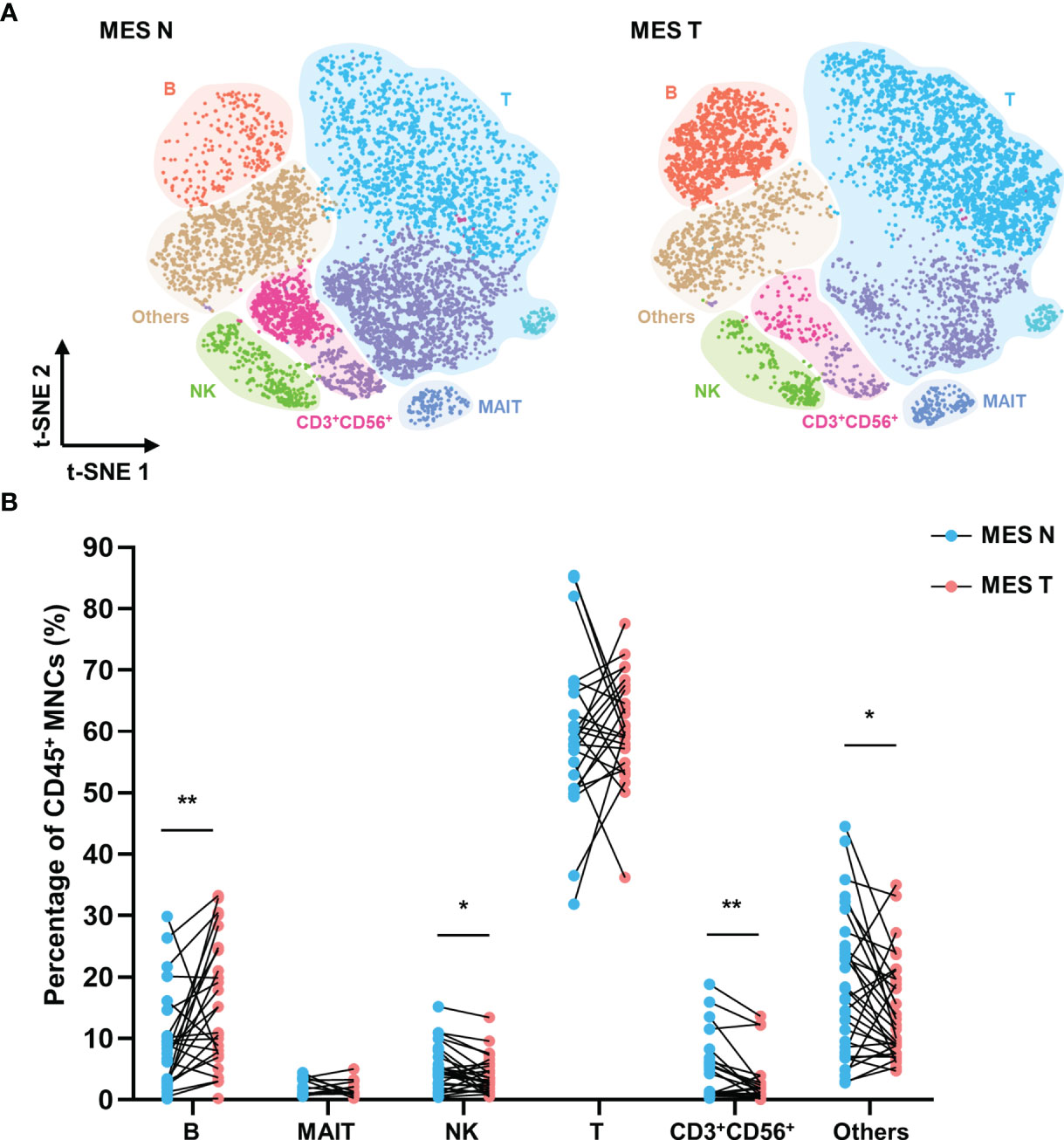
Figure 3 T-SNE plot and percentages of CD45+ MNCs in the MES N and MES T (A) T-SNE plot of CD45+ MNCs in the MES N and MES T with main subclusters indicated. (B) Percentages of B cells, MAIT cells, NK cells, T cells, CD3+ CD56+ cells, and other cells (monocytes/macrophages and dendritic cells) in the MES N and MES T. (*p < 0.05; **p < 0.01).
As shown in Figure 4A, we identified different T cell clusters in the MES N and MES T and observed that CD45RO and CD69 expression levels were significantly low in T cells in the MES T. Compared with the MES N, the percentage of memory CD4+ T cells showed a downward trend in the MES T, and the percentage of CD4+ TRM cells, particularly the CD4+ CD103- TRM cells was significantly decreased in the MES T (Figures 4B, C). Moreover, the percentage of Treg cells was significantly higher in the MES T than in the MES N (Figure 4D). Reportedly, Foxp3+ Treg cells can be categorized into three subclusters (29), including naïve/resting Treg cells (Foxp3lo CD45RA+), effector/activated Treg cells (Foxp3hi CD45RA-), and non Treg cells (Foxp3lo CD45RA-) (Figure S4B). Treg cells, particularly effector Treg cells induce marked immunosuppression, including suppression of T cell proliferation, cytokine production, and anti-tumor activity (29, 30). We observed that effector Treg cells were enriched in the MES T (Figure 4E). Additionally, CD8+ TRM cells, specifically CD8+ CD103- TRM cells were significantly decreased in the MES T (Figure 4F). Furthermore, the percentage of Treg (Foxp3+ CD4+) cells was upregulated and the percentage of CD69+ CD4+ cells was downregulated in the MES T by mIHC (Figures S3A–C). However, the percentage of CD69+ CD8+ cells did not change in the MES T (Figures S3A–C). Moreover, although the t-SNE plot of B cells in the MES N and MES T did not significantly differ between B cell subclusters (Figure S6A), the percentages of unswitched B cells and IgM+ unswitched B cells in the MES T showed an upward trend (Figure S6B).
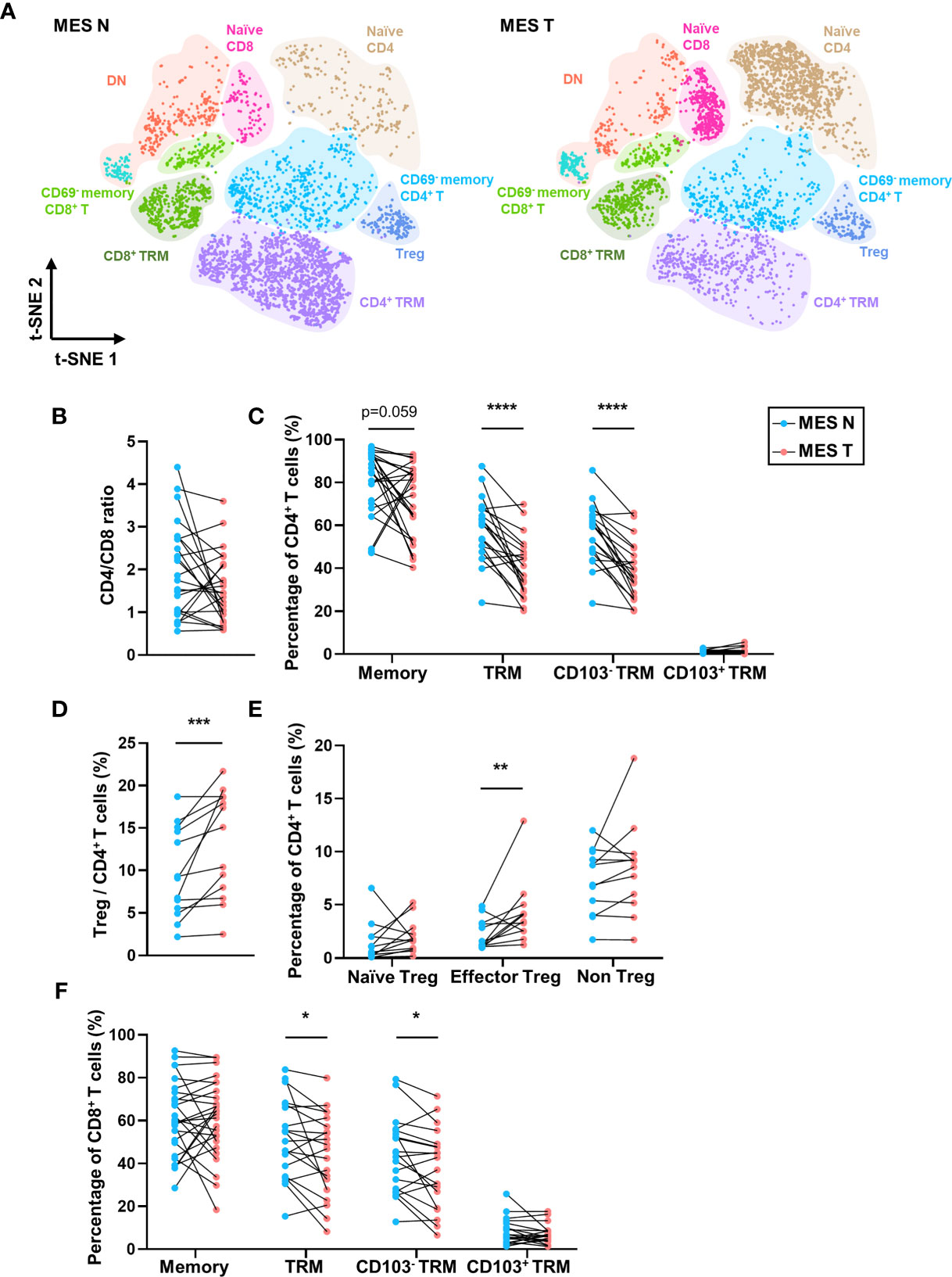
Figure 4 T-SNE plot and percentages of T cells in the MES N and MES T. (A) T-SNE plot of T cells in the MES N and MES T with main subclusters indicated. (B) CD4/CD8 ratio in the MES N and MES T. (C) Percentages of memory CD4+ T cells, CD4+ TRM cells, CD4+ CD103- TRM cells, and CD4+ CD103+ TRM cells in the MES N and MES T. (D) Percentage of Treg cells in the MES N and MES T. (E) Percentages of naïve Treg cells, effector Treg cells, and non Treg cells in the MES N and MES T. (F) Percentages of memory CD8+ T cells, CD8+ TRM cells, CD8+ CD103- TRM cells, and CD8+ CD103+ TRM cells in the MES N and MES T. (*p < 0.05; **p < 0.01; ***p < 0.001; ****p < 0.0001).
The reduced percentage of TRM cells and increased percentage of Treg cells in the MES T indicate poor anti-tumor immunity in the MES T. Therefore, it can be concluded that in addition to functioning as connective tissue that supports the intestine, the mesentery is an important component of the tumor microenvironment.
Memory CD8+ T Cells and Plasmablasts Are Negatively Correlated With the Depth of Invasion of Colorectal Cancer, and Increased Memory CD4+ T Cells Are Associated With Distant Metastasis of Colorectal Cancer
We investigated the association between lymphocytes in the MES T and the tumor stage in 32 patients with CRC. We observed that the percentages of memory CD8+ T cells and CD8+ TRM cells, particularly CD8+ CD103- TRM cells, as well as plasmablasts in the MES T were significantly lower in patients with CRC showing T4 stage disease than those with T2-3 stage disease (Figures 5A, B). Moreover, our results showed that the percentage of memory CD4+ T cells in the MES T was higher in patients with distant metastasis (M1 stage CRC) than in those without distant metastasis (M0 stage CRC) (Figure 5C). Other lymphocytes were irrelevant to the tumor stage (data not shown). Therefore, our results indicate that decreased percentages of memory CD8+ T cells and plasmablasts in the MES T are associated with an increased depth of invasion of CRC, and increased percentage of memory CD4+ T cells is associated with distant metastasis of CRC.
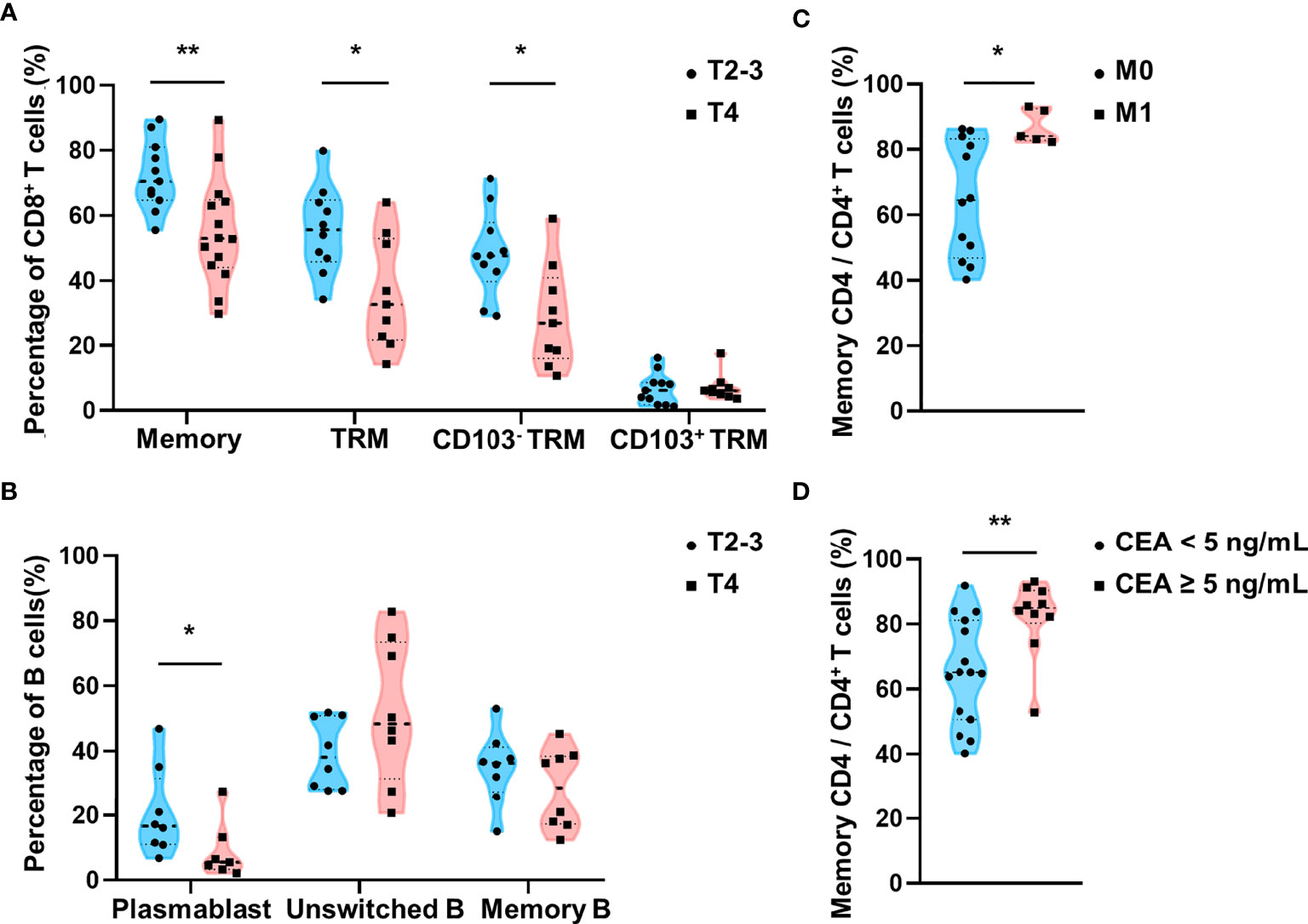
Figure 5 Relationship between lymphocytes in the MES T and TNM stages and preoperative serum CEA levels. (A) Comparison of the percentages of memory CD8+ T cells, CD8+ TRM cells, CD8+ CD103- TRM cells, and CD8+ CD103+ TRM cells in the MES T between patients with T2-3 stage CRC and T4 stage CRC. (B) Comparison of the percentages of plasmablasts, unswitched B cells, and memory B cells in the MES T between patients with T2-3 stage CRC and T4 stage CRC. (C) Comparison of the percentage of memory CD4+ T cells in the MES T between patients with M0 stage CRC and M1 stage CRC. (D) Comparison of the percentage of memory CD4+ T cells in the MES T between patients with CRC showing different preoperative serum CEA levels (<5ng/mL and ≥5ng/mL). (mean ± SD; *p < 0.05; **p < 0.01).
Increased Memory CD4+ T Cells Are Associated With High Preoperative Serum Carcinoembryonic Antigen Levels
Serum CEA is hyperexpressed in patients with CRC and is widely used as a biomarker for CRC (31, 32). A preoperative serum CEA level ≥5 ng/mL is considered an independent prognostic factor for poor overall survival (33). Moreover, preoperative serum CEA elevation (≥5 ng/mL) is associated with a high recurrence rate (34). In our study, we observed a significantly high percentage of memory CD4+ T cells in the MES T of patients with CRC, who showed preoperative serum CEA levels ≥5 ng/mL (Figure 5D). Other lymphocytes were irrelevant to the preoperative serum CEA levels (data not shown). Overall, our data indicate that a high percentage of memory CD4+ T cells in the MES T is a predictor of distant metastasis of CRC and CRC recurrence. The schema of the immune landscapes of the PB, the MES N, and MES T in patients with CRC is displayed in Figure 6.
Discussion
Following latest research in this field, the mesentery is currently classified as a distinct organ (13) that is histologically characterized by surface mesothelium, the connective tissue lattice, and adipocytes dispersed within the interstices of the lattice (13). However, the immune landscape of the mesentery remains unclear. Based on the findings of this study, we could successfully establish that the MES N shows a specific immune microenvironment that differs from that observed in the PB. We also observed that the immune cells detected in the MES N are primarily of lymphoid lineage. CRC can alter the mesenteric immune response to one which favors tumor promotion. Our study identified more specific and comprehensive lymphocyte populations in the mesentery, which provides a deeper understanding of the mesenteric immune microenvironment and its association with clinicopathological characteristics of patients with CRC.
Intestinal tumors can invade the contiguous mesentery. Studies have proved that the mesentery could serve as a potential site of residual tumors, leading to postoperative local CRC recurrence (6–8). Previous reports have characterized the mesenteric immune microenvironment with regard to macrophage infiltration (18); however, the lymphocyte subpopulations of the MES T remain unclear. Our study is the first to highlight that anti-tumor lymphocytes (NK and CD8+ TRM cells, specifically CD8+ CD103- TRM cells) were decreased and immunosuppressive lymphocytes (Treg cells, specifically effector Treg cells) were increased in the MES T. These results indicate poor anti-tumor immunity in the MES T, which favors CRC development. Therefore, it can be inferred that the MES T may represent a component of the tumor microenvironment, and it is important to consider the potential role of the mesentery as a contributor to the development and progression of intestinal diseases.
Furthermore, our data indicate that memory CD8+ T cells, particularly CD8+ TRM cells and plasmablasts in the MES T are negatively correlated with the depth of invasion of CRC. Based on expression of high levels of TNF-α, IFN-γ, and granzyme B, CD8+ TRM cell infiltration in various human cancers is correlated with favorable clinical outcomes, and CD8+ TRM cells have emerged as a predictive marker of survival in several human epithelial cancers (35–40). These results suggest that mesenteric CD8+ TRM cells may play a protective role against CRC. Additionally, a high percentage of memory CD4+ T cells is a hallmark of adaptive immune memory (41). Our results indicate that enhanced expression of memory CD4+ T cells in the MES T is associated with distant metastasis of CRC, which could be attributable to the fact that CD4+ T cells are activated and differentiated into memory CD4+ T cells in the MES T in patients with distant metastasis. Moreover, our data show a significantly high percentage of memory CD4+ T cells in the MES T in patients with preoperative serum CEA levels ≥5 ng/mL. Serum CEA, which is a well-known marker of CRC, is hyperexpressed in patients with CRC, particularly in those showing CRC recurrence (31). High preoperative serum CEA levels suggest advanced disease with local or distant metastasis (42, 43). A cut-off level of 5 ng/mL is used to define CEA elevation; cancer-specific mortality rates are higher and prognosis is poorer in patients with CRC showing preoperative serum CEA levels ≥5 ng/mL (44). Taken together, our results indicate that memory CD4+ T cells may emerge as a useful predictive marker of distant metastasis of CRC and CRC recurrence, and this subpopulation of lymphocytes may serve as a potential target for the treatment of patients with CRC.
In addition to CRC, many intra-abdominal tumors, such as ovarian (45, 46), endometrial (47), gastric (48), and pancreatic cancer (49) show a predilection for peritoneal metastasis with poor prognosis. Moreover, recent studies have provided a wide range of evidence that describes visceral obesity as an important etiological contributor to cancer (50, 51). Therefore, further research on the mesenteric immune microenvironment is warranted considering its overall significance, not limited to CRC. Furthermore, regulation of the mesenteric immune microenvironment may be a useful therapeutic strategy for metastatic tumors. It is noteworthy that the clinical relevance of the mesentery is not confined to abdominal disease (52–54); the mesentery is the single greatest contributor to visceral adiposity (55), which plays an important role in the pathobiology of obesity, diabetes, and metabolic syndrome (56). It is equally important to focus on the involvement of the mesenteric immune microenvironment in these disorders.
In summary, we investigated the lymphocyte landscape of the mesentery and observed that it was correlated with CRC progression. However, the limitations of our study cannot be ignored; the mechanisms of how CRC affects the composition and phenotype of mesenteric lymphocytes and the long-term effects of mesenteric lymphocytes on the prognosis of patients with CRC remain unclear. Further investigations and large-scale studies that include long-term survival data are warranted to confirm the association between CRC and the mesenteric immune microenvironment.
Data Availability Statement
The original contributions presented in the study are included in the article/Supplementary Material. Further inquiries can be directed to the corresponding authors.
Ethics Statement
The studies involving human participants were reviewed and approved by Guangzhou First People’s Hospital Ethics Review Committee. The patients/participants provided their written informed consent to participate in this study. Written informed consent was obtained from the individual(s) for the publication of any potentially identifiable images or data included in this article.
Author Contributions
Z-XL, JC, Z-BZ, and W-LL conceived and participated in the design of the study and revised the manuscript. The manuscript was written and revised by Z-XW and FW. Z-XW, FW, and Q-QL performed the experimental work. Z-XW and Q-QL collected the clinical data. LL, YY, and JL participated in data analysis. All authors contributed to the article and approved the submitted version.
Funding
This work was supported by Program for Guangdong Introducing Innovative and Entrepreneurial Teams (2017ZT07S054), Guangdong Basic and Applied Basic Research Foundation (2020A1515010897), Special Clinical Technology Program of Guangzhou (2019TS52), Guangzhou Science Technology and Innovation Commission (201804010073), National Natural Science Foundation of China (81871943), Guangzhou Science Technology and Innovation Commission (201805010003), Outstanding Scholar Program of Bioland Laboratory (Guangzhou Regenerative Medicine and Health Guangdong Laboratory) (2018GZR110102001) and Guangdong Basic and Applied Basic Research Foundation (2021B1515420004).
Conflict of Interest
The authors declare that the research was conducted in the absence of any commercial or financial relationships that could be construed as a potential conflict of interest.
Publisher’s Note
All claims expressed in this article are solely those of the authors and do not necessarily represent those of their affiliated organizations, or those of the publisher, the editors and the reviewers. Any product that may be evaluated in this article, or claim that may be made by its manufacturer, is not guaranteed or endorsed by the publisher.
Supplementary Material
The Supplementary Material for this article can be found online at: https://www.frontiersin.org/articles/10.3389/fonc.2021.685577/full#supplementary-material
References
1. Bray F, Ferlay J, Soerjomataram I, Siegel RL, Torre LA, Jemal A. Global Cancer Statistics 2018: GLOBOCAN Estimates of Incidence and Mortality Worldwide for 36 Cancers in 185 Countries. CA Cancer J Clin (2018) 68:394–424. doi: 10.3322/caac.21492
2. Sargent DJ, Wieand HS, Haller DG, Gray R, Benedetti JK, Buyse M, et al. Disease-Free Survival Versus Overall Survival as a Primary End Point for Adjuvant Colon Cancer Studies: Individual Patient Data From 20,898 Patients on 18 Randomized Trials. J Clin Oncol (2005) 23:8664–70. doi: 10.1200/JCO.2005.01.6071
3. Kievit J. Follow-Up of Patients With Colorectal Cancer: Numbers Needed to Test and Treat. Eur J Cancer (2002) 38:986–99. doi: 10.1016/S0959-8049(02)00061-8
4. Pfannschmidt J, Dienemann H, Hoffmann H. Surgical Resection of Pulmonary Metastases From Colorectal Cancer: A Systematic Review of Published Series. Ann Thorac Surg (2007) 84:324–38. doi: 10.1016/j.athoracsur.2007.02.093
5. Deshpande AD, Schootman M, Mayer A. Development of a Claims-Based Algorithm to Identify Colorectal Cancer Recurrence. Ann Epidemiol (2015) 25:297–300. doi: 10.1016/j.annepidem.2015.01.005
6. Abulafi AM, Williams NS. Local Recurrence of Colorectal Cancer: The Problem, Mechanisms, Management and Adjuvant Therapy. Br J Surg (1994) 81:7–19. doi: 10.1002/bjs.1800810106
7. Smith FM, Ahad A, Perez RO, Marks J, Bujko K, Heald RJ. Local Excision Techniques for Rectal Cancer After Neoadjuvant Chemoradiotherapy: What Are We Doing? Dis Colon Rectum (2017) 60:228–39. doi: 10.1097/DCR.0000000000000749
8. Bowne WB, Lee B, Wong WD, Ben-Porat L, Shia L, Cohen AM, et al. Operative Salvage for Locoregional Recurrent Colon Cancer After Curative Resection: An Analysis of 100 Cases. Dis Colon Rectum (2005) 48:897–909. doi: 10.1007/s10350-004-0881-8
9. Ma CS, Tong YX, Gong JP. Distribution of Metastatic Cancer Cells in Colorectal Mesentery. World J Surg (2020) 44:967–72. doi: 10.1007/s00268-019-05284-5
10. Luo XL, Xie DX, Wu JX, Wu AD, Ge ZQ, Li HJ, et al. Detection of Metastatic Cancer Cells in Mesentery of Colorectal Cancer Patients. World J Gastroenterol (2017) 23:6315–20. doi: 10.3748/wjg.v23.i34.6315
11. Veronese N, Nottegar A, Pea A, Solmi M, Stubbs B, Capelli P, et al. Prognostic Impact and Implications of Extracapsular Lymph Node Involvement in Colorectal Cancer: A Systematic Review With Meta-Analysis. Ann Oncol (2016) 27:42–8. doi: 10.1093/annonc/mdv494
12. Goldstein NS, Turner JR. Pericolonic Tumor Deposits in Patients With T3N+M0 Colon Adenocarcinomas - Markers of Reduced Disease Free Survival and Intra-Abdominal Metastases and Their Implications for TNM Classification. Cancer (2000) 88:2228–38. doi: 10.1002/(SICI)1097-0142(20000515)88:10<2228::AID-CNCR5>3.0.CO;2-1
13. Coffey JC, O'Leary DP. The Mesentery: Structure, Function, and Role in Disease. Lancet Gastroenterol Hepatol (2016) 1:238–47. doi: 10.1016/S2468-1253(16)30026-7
14. Li Y, Zhu W, Zuo L, Shen B. The Role of the Mesentery in Crohn's Disease: The Contributions of Nerves, Vessels, Lymphatics, and Fat to the Pathogenesis and Disease Course. Inflammation Bowel Dis (2016) 22:1483–95. doi: 10.1097/MIB.0000000000000791
15. Peyrin-Biroulet L, Chamaillard M, Gonzalez F, Beclin E, Decourcelle C, Antunes L, et al. Mesenteric Fat in Crohn's Disease: A Pathogenetic Hallmark or an Innocent Bystander? Gut (2007) 56:577–83. doi: 10.1136/gut.2005.082925
16. Yamamoto K, Kiyohara T, Murayama Y, Kihara S, Okamoto Y, Funahashi T, et al. Production of Adiponectin, an Anti-Inflammatory Protein, in Mesenteric Adipose Tissue in Crohn's Disease. Gut (2005) 54:789–96. doi: 10.1136/gut.2004.046516
17. Goncalves P, Magro F, Martel F. Metabolic Inflammation in Inflammatory Bowel Disease: Crosstalk Between Adipose Tissue and Bowel. Inflamm Bowel Dis (2015) 21:453–67. doi: 10.1097/MIB.0000000000000209
18. Kredel LI, Batra A, Stroh T, Kühl AA, Zeitz M, Erben U, et al. Adipokines From Local Fat Cells Shape the Macrophage Compartment of the Creeping Fat in Crohn's Disease. Gut (2013) 62:852–62. doi: 10.1136/gutjnl-2011-301424
19. Cibrian D, Sanchez-Madrid F. CD69: From Activation Marker to Metabolic Gatekeeper. Eur J Immunol (2017) 47:946–53. doi: 10.1002/eji.201646837
20. Radulovic K, Niess JH. CD69 Is the Crucial Regulator of Intestinal Inflammation: A New Target Molecule for IBD Treatment? J Immunol Res (2015) 2015:497056. doi: 10.1155/2015/497056
21. Cyster JG, Schwab SR. Sphingosine-1-Phosphate and Lymphocyte Egress From Lymphoid Organs. Annu Rev Immunol (2012) 30:69–94. doi: 10.1146/annurev-immunol-020711-075011
22. Matloubian M, Lo CG, Cinamon G, Lesneski MJ, Xu Y, Brinkmann V, et al. Lymphocyte Egress From Thymus and Peripheral Lymphoid Organs Is Dependent on S1P Receptor 1. Nature (2004) 427:355–60. doi: 10.1038/nature02284
23. Skon CN, Lee JY, Anderson KG, Masopust D, Hogquist KA, Jameson SC. Transcriptional Downregulation of S1pr1 Is Required for the Establishment of Resident Memory CD8(+) T Cells. Nat Immunol (2013) 14:1285–93. doi: 10.1038/ni.2745
24. Chen L, Shen Z. Tissue-Resident Memory T Cells and Their Biological Characteristics in the Recurrence of Inflammatory Skin Disorders. Cell Mol Immunol (2020) 17:64–75. doi: 10.1038/s41423-019-0291-4
25. Lan B, Zhang J, Lu D, Li W. Generation of Cancer-Specific CD8(+) CD69(+) Cells Inhibits Colon Cancer Growth. Immunobiology (2016) 221:1–5. doi: 10.1016/j.imbio.2015.08.010
26. Liechti T, Gunthard HF, Trkola A. OMIP-047: High-Dimensional Phenotypic Characterization of B Cells. Cytometry A (2018) 93:592–6. doi: 10.1002/cyto.a.23488
27. Shi Z, Zhang Q, Yan H, Yang Y, Wang P, Zhang Y, et al. More Than One Antibody of Individual B Cells Revealed by Single-Cell Immune Profiling. Cell Discov (2019) 5:64. doi: 10.1038/s41421-019-0137-3
28. Zhang YY, Zhang ZM. The History and Advances in Cancer Immunotherapy: Understanding the Characteristics of Tumor-Infiltrating Immune Cells and Their Therapeutic Implications. Cell Mol Immunol (2020) 17:807–21. doi: 10.1038/s41423-020-0488-6
29. Miyara M, Gorochov G, Ehrenstein M, Musset L, Sakaguchi S, Amoura Z. Human FoxP3+ Regulatory T Cells in Systemic Autoimmune Diseases. Autoimmun Rev (2011) 10:744–55. doi: 10.1016/j.autrev.2011.05.004
30. Yang DD, Zhao XQ, Lin X. Bcl10 Is Required for the Development and Suppressive Function of Foxp3(+) Regulatory T Cells. Cell Mol Immunol (2021) 18:206–18. doi: 10.1038/s41423-019-0297-y
31. Ma J, Liu X, Chen H, Abbas MK, Yang L, Sun H, et al. C-KIT-ERK1/2 Signaling Activated ELK1 and Upregulated Carcinoembryonic Antigen Expression to Promote Colorectal Cancer Progression. Cancer Sci (2021) 112:655–67. doi: 10.1111/cas.14750
32. Hammarstrom S. The Carcinoembryonic Antigen (CEA) Family: Structures, Suggested Functions and Expression in Normal and Malignant Tissues. Semin Cancer Biol (1999) 9:67–81. doi: 10.1006/scbi.1998.0119
33. Yeh CY, Hsieh PS, Chiang JM, Lai CC, Chen JS, Wang JY, et al. Preoperative Carcinoembryonic Antigen Elevation in Colorectal Cancer. Hepatogastroenterology (2011) 58:1171–6. doi: 10.5754/hge10564
34. Tokodai K, Narimatsu H, Nishida A, Takaya K, Hara Y, Kawagishi N, et al. Risk Factors for Recurrence in Stage II/III Colorectal Cancer Patients Treated With Curative Surgery: The Impact of Postoperative Tumor Markers and an Infiltrative Growth Pattern. J Surg Oncol (2016) 114:368–74. doi: 10.1002/jso.24320
35. Hombrink P, Helbig C, Backer RA, Piet B, Oja AE, Stark R, et al. Programs for the Persistence, Vigilance and Control of Human CD8(+) Lung-Resident Memory T Cells. Nat Immunol (2016) 17:1467–78. doi: 10.1038/ni.3589
36. Fraczek A, Owczarczyk-Saczonek A, Placek W. The Role of T-RM Cells in the Pathogenesis of Vitiligo-A Review of the Current State-of-the-Art. Int J Mol Sci (2020) 21:3552. doi: 10.3390/ijms21103552
37. Ganesan AP, Clarke J, Wood O, Garrido-Martin EM, Chee SJ, Mellows T, et al. Tissue-Resident Memory Features Are Linked to the Magnitude of Cytotoxic T Cell Responses in Human Lung Cancer. Nat Immunol (2017) 18:940–+. doi: 10.1038/ni.3775
38. Webb JR, Milne K, Watson P, Deleeuw RJ, Nelson BH. Tumor-Infiltrating Lymphocytes Expressing the Tissue Resident Memory Marker CD103 Are Associated With Increased Survival in High-Grade Serous Ovarian Cancer. Clin Cancer Res (2014) 20:434–44. doi: 10.1158/1078-0432.CCR-13-1877
39. Djenidi F, Adam J, Goubar A, Durgeau A, Meurice G, de Montpréville V, et al. CD8(+) CD103(+) Tumor-Infiltrating Lymphocytes Are Tumor-Specific Tissue-Resident Memory T Cells and a Prognostic Factor for Survival in Lung Cancer Patients. J Immunol (2015) 194:3475–86. doi: 10.4049/jimmunol.1402711
40. Sun HY, Sun C, Xiao WH, Sun R. Tissue-Resident Lymphocytes: From Adaptive to Innate Immunity. Cell Mol Immunol (2019) 16:205–15. doi: 10.1038/s41423-018-0192-y
41. MacLeod MK, McKee A, Crawford F, White J, Kappler J, Marrack P. CD4 Memory T Cells Divide Poorly in Response to Antigen Because of Their Cytokine Profile. Proc Natl Acad Sci USA (2008) 105:14521–6. doi: 10.1073/pnas.0807449105
42. Ishizuka D, Shirai Y, Sakai Y, Hatakeyama K. Colorectal Carcinoma Liver Metastases: Clinical Significance of Preoperative Measurement of Serum Carcinoembryonic Antigen and Carbohydrate Antigen 19-9 Levels. Int J Colorectal Dis (2001) 16:32–7. doi: 10.1007/s003840000268
43. Holubec L Jr, Topolcan O, Pikner R, Pecen L, Holubec Sen L, Finek J, et al. Criteria for the Selection of Referential Groups in Tumor Marker Statistical Evaluation on the Basis of a Retrospective Study. Anticancer Res (2003) 23:865–70.
44. Thirunavukarasu P, Talati C, Munjal S, Attwood K, Edge SB, Francescutti V. Effect of Incorporation of Pretreatment Serum Carcinoembryonic Antigen Levels Into AJCC Staging for Colon Cancer on 5-Year Survival. JAMA Surg (2015) 150:747–55. doi: 10.1001/jamasurg.2015.0871
45. Landen CN Jr, Birrer MJ, Sood AK. Early Events in the Pathogenesis of Epithelial Ovarian Cancer. J Clin Oncol (2008) 26:995–1005. doi: 10.1200/JCO.2006.07.9970
46. Cho KR, Shih Ie M. Ovarian Cancer. Annu Rev Pathol (2009) 4:287–313. doi: 10.1146/annurev.pathol.4.110807.092246
47. Joo WD, Schwartz PE, Rutherford TJ, Seong SJ, Ku J, Park H, et al. Microscopic Omental Metastasis in Clinical Stage I Endometrial Cancer: A Meta-Analysis. Ann Surg Oncol (2015) 22:3695–700. doi: 10.1245/s10434-015-4443-1
48. Coccolini F, Gheza F, Lotti M, Virzì S, Iusco D, Ghermandi C, et al. Peritoneal Carcinomatosis. World J Gastroenterol (2013) 19:6979–94. doi: 10.3748/wjg.v19.i41.6979
49. Peixoto RD, Speers C, McGahan CE, Renouf DJ, Schaeffer DF, Kennecke HF. Prognostic Factors and Sites of Metastasis in Unresectable Locally Advanced Pancreatic Cancer. Cancer Med (2015) 4:1171–7. doi: 10.1002/cam4.459
50. Divella R, De Luca R, Abbate I, Naglieri E, Daniele A. Obesity and Cancer: The Role of Adipose Tissue and Adipo-Cytokines-Induced Chronic Inflammation. J Cancer (2016) 7:2346–59. doi: 10.7150/jca.16884
51. Nieman KM, Kenny HA, Penicka CV, Ladanyi A, Buell-Gutbrod R, Zillhardt MR, et al. Adipocytes Promote Ovarian Cancer Metastasis and Provide Energy for Rapid Tumor Growth. Nat Med (2011) 17:1498–503. doi: 10.1038/nm.2492
52. Kredel LI, Siegmund B. Adipose-Tissue and Intestinal Inflammation - Visceral Obesity and Creeping Fat. Front Immunol (2014) 5:462. doi: 10.3389/fimmu.2014.00462
53. Schaffler A, Scholmerich J, Buchler C. Mechanisms of Disease: Adipocytokines and Visceral Adipose Tissue–Emerging Role in Intestinal and Mesenteric Diseases. Nat Clin Pract Gastroenterol Hepatol (2005) 2:103–11. doi: 10.1038/ncpgasthep0090
54. Rethorst CD, Bernstein I, Trivedi MH. Inflammation, Obesity, and Metabolic Syndrome in Depression: Analysis of the 2009-2010 National Health and Nutrition Examination Survey (NHANES). J Clin Psychiatry (2014) 75:e1428–32. doi: 10.4088/JCP.14m09009
55. Yang YK, Chen M, Clements RH, Abrams GA, Aprahamian CJ, Harmon CM. Human Mesenteric Adipose Tissue Plays Unique Role Versus Subcutaneous and Omental Fat in Obesity Related Diabetes. Cell Physiol Biochem (2008) 22:531–8. doi: 10.1159/000185527
Keywords: colorectal cancer, clinical significance, immune microenvironment, lymphocyte, mesentery
Citation: Wu Z-X, Wang F, Li L, Yao Y, Long J, Luo Q-Q, Zhao Z-B, Li W-L, Cao J and Lian Z-X (2021) The Clinical Significance of Mesenteric Lymphocytes in Human Colorectal Cancer. Front. Oncol. 11:685577. doi: 10.3389/fonc.2021.685577
Received: 25 March 2021; Accepted: 27 August 2021;
Published: 16 September 2021.
Edited by:
Pranav Shivakumar, Cincinnati Children’s Hospital Medical Center, United StatesCopyright © 2021 Wu, Wang, Li, Yao, Long, Luo, Zhao, Li, Cao and Lian. This is an open-access article distributed under the terms of the Creative Commons Attribution License (CC BY). The use, distribution or reproduction in other forums is permitted, provided the original author(s) and the copyright owner(s) are credited and that the original publication in this journal is cited, in accordance with accepted academic practice. No use, distribution or reproduction is permitted which does not comply with these terms.
*Correspondence: Wang-Lin Li, ZXlsd2xAc2N1dC5lZHUuY24=; Zhi-Bin Zhao, enpiaW5AbWFpbC51c3RjLmVkdS5jbg==
†These authors have contributed equally to this work and share first authorship