- State Key Laboratory of Oral Diseases, National Clinical Research Center for Oral Diseases, West China Hospital of Stomatology, Sichuan University, Chengdu, China
The protein kinase D (PKD) family is a family of serine-threonine kinases that are members of the calcium/calmodulin-dependent kinase (CaMK) superfamily. PKDs have been increasingly implicated in multiple pivotal cellular processes and pathological conditions. PKD dysregulation is associated with several diseases, including cancer, inflammation, and obesity. Over the past few years, small-molecule inhibitors have emerged as alternative targeted therapy with fewer adverse side effects than currently available chemotherapy, and these specifically targeted inhibitors limit non-specific toxicities. The successful development of PKD inhibitors would significantly suppress the growth and proliferation of various cancers and inhibit the progression of other diseases. Various PKD inhibitors have been studied in the preclinical setting. In this context, we summarize the PKD inhibitors under investigation and their application for different kinds of diseases.
Introduction
The protein kinase D (PKD) family is a serine-threonine kinase family that is a subfamily of the calcium/calmodulin-dependent kinase (CaMK) superfamily. There are three isoforms (PKD1, PKD2, and PKD3) that share high sequence identity in mammals. In 1994, PKD1 (first termed PKCμ) was characterized as a novel kinase activated by the secondary messenger diacylglycerol (DAG) (1, 2). PKD3 and PKD2 were subsequently discovered in 1999 (3) and 2001 (4), respectively. The PKDs are activated by many substances, including phorbol ester, platelet-derived growth factor, and G protein-coupled receptor ligands (5). In humans, the three PKD isoforms are expressed in most tissues, including the oral cavity, skin, liver, lung, pancreas, breast, and stomach (4, 6–10). Interestingly, the expression of different isoforms may be different depending on the physiological and pathological conditions. For example, PKD1 expression is high in normal breast tissue, while PKD2 and PKD3 expression levels are low. In contrast, PKD1 expression is absent and PKD2 and PKD3 expression levels are elevated in breast cancer (11). In animals, PKD1, PKD2, and PKD3 are widely expressed in the central nervous system, large intestine, adrenal gland, bladder, cerebellum, and colon, and the expression levels of the three isoforms are different (12–15). PKD activity is associated with many diseases and pathological conditions, such as cancer, metabolic diseases, and inflammation, where it regulates cell proliferation, differentiation, programmed cell death, migration, and invasion (16–19). Pharmacological targeting of PKDs to regulate their expression and kinase activity has important implication for treating disease (20).
Small-molecule inhibitors (SMIs) have been accepted as new therapeutic alternatives for treating disease because they can provide a targeted treatment approach, resulting in fewer undesirable side effects than currently available chemotherapy (21). SMIs are compounds of less than 500 Da, that can potentially bind to a wide range of extracellular and intracellular targets. PKDs have attracted the interest of many researchers because they can regulate cellular functions and play important roles in disease; however, there are no clinical inhibitors targeting PKDs (20). Recently, specific PKD inhibitors have been discovered, and confirmed the important role of the PKDs in the induction and progression of many diseases (22–24).
Over the past few decades, studies have shown that PKDs play an important role in the occurrence and development of various diseases (25). Many PKD SMIs have been investigated in preclinical studies as unique and effective potential drugs. In this review, we summarize the known PKD SMIs and their potential application in various diseases.
PKD Structure and Function
The PKD family members have a similar structure but different activation patterns and downstream functions (26). They contain a catalytic domain, a pleckstrin homology (PH) domain, and two N-terminal cysteine-rich zinc finger domains (C1a and C1b), which bind DAG and phorbol esters (27). The three isoforms have high homology, especially in the catalytic and C1 domains. Their differences are located in the N-terminal region and the regions flanked by the C1 and PH domains, possibly related to their functional differences (28). In the absence of the PH structure, enzyme activity increases, indicating that the PH domain inhibits enzyme activity (29) (Figure 1).
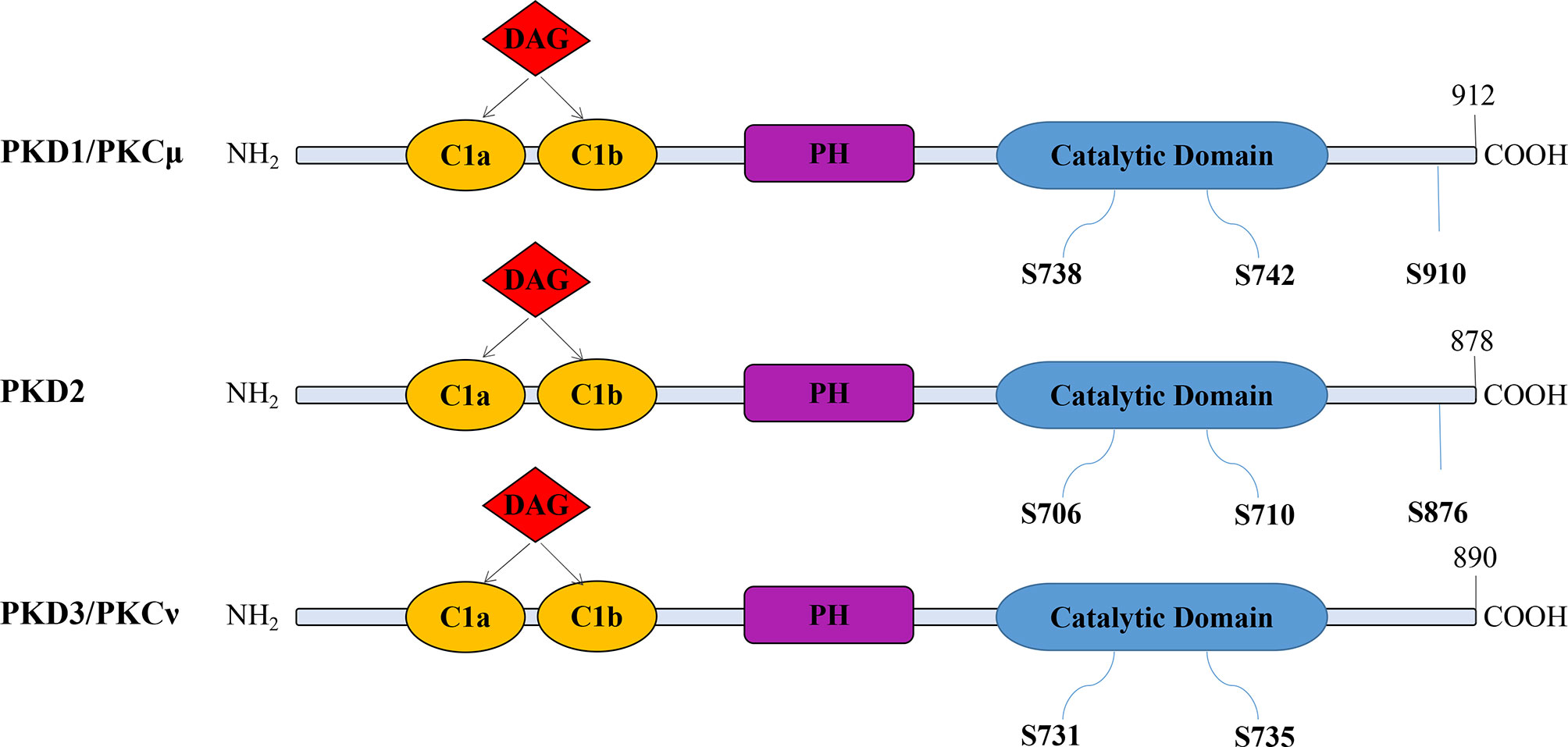
Figure 1 The modular structure of PKD isoforms. The PKD isoforms have highly homologous structures containing cysteine-rich Zn-finger-like motifs (CIa and CIb), a pleckstrin homology (PH) domain, and a C-terminal catalytic domain. PKDs are activated by the phosphorylation of serine residues. However, PKD3 lacks a C-terminal autophosphorylation site within the PDZ (PSD-95/Discs large/ZO-1) binding motif present in both PKD1 and PKD2 (S910 in PKD1; S876 in PKD2).
PKD dysfunction is associated with many pathological processes, including promoting cell proliferation, inhibiting apoptosis, promoting angiogenesis, and disrupting homeostasis (4, 20). Different isoforms can promote tumorigenesis and anti-tumor effects (25). In cancer, PKD1 plays different roles depending on tumor type, both pro-tumorigenesis and anti-tumor. PKD1 levels are significantly downregulated in breast cancer, acting as an antioncogene (11). In pancreatic cancer, PKD1 levels are upregulated. These increased levels promote mitosis and angiogenesis by mediating signal transduction and enhance chemical resistance and invasion of pancreatic cancer cells (30). Compared to PKD1, PKD2 and PKD3 are often associated with tumor progression. Hao et al. (31) have reported that PKD2 mediates endothelial cell proliferation, migration, and angiogenesis. In addition, PKD2 is involved in the development of various tumor types including pancreatic, colorectal, and breast cancer, glioma multiforme, and leukemia. It plays a versatile role in these cancers through uncontrolled growth, survival, neovascularization, metastasis, and invasion (32). The study showed that PKD3 enhances proliferation of cancer cells through the mTORC1-S6K1 signaling pathway in triple negative breast cancer (33). Silencing PKD3 also inhibited tumor immune escape by reducing PD-L1 expression in oral squamous cell carcinoma cells (OSCC) (7). Thus, PKDs play important roles in disease and are potential targets for treatment.
PKD Inhibitors
SMIs are typically chemically synthesized organic compounds with molecular weights of less than 500 Da. Over the past few decades, chemotherapy has been a key treatment for fighting cancer; however, it usually does not distinguish between normal and tumor cells and is limited by a narrow treatment index. In contrast, targeted therapies interfere with specific molecular targets, limiting non-specific toxicity. Compared with monoclonal antibody drugs that only act on cell surface receptors, small molecular inhibitors can enter the cell via a concentration gradient so that they have a broader range of targets (21). Small-molecule inhibitors have been investigated in cancer and cardiovascular, endocrine, infectious, immune, metabolic, and nervous system diseases (25). More and more PKD inhibitors have been identified, and pan-PKD inhibitors have demonstrated in vitro and in vivo antitumoral activity in various disease models, despite the opposing roles of the PKD isoforms in some disease (34, 35).
CRT0066101
In 2010, Harikumar et al. (23) discovered CRT0066101 (2-[4-((2R)-2-aminobutyl-amino)-pyrimidin-2-yl]-4-(1-methyl-1H-pyrazol-4-yl)-phenol dihydrochloride) (Figure 2A), which is the most effective, selective, orally bioavailable pan-PKD inhibitor (IC50 = 1, 2.5, and 2 nM for PKD1, 2, and 3, respectively). CRT0066101 can inhibit tumor growth in animal models of breast, colorectal, and pancreatic cancer (23, 36, 37). In addition, CRT0066101 exerts synergistic effects against colorectal cancer with regorafenib (38), an FDA-approved multi-kinase inhibitor for the treatment of metastatic colorectal cancer. The anti-tumor effects of CRT0066101 are mediated its inhibition of cell growth, and angiogenesis and its promotion of apoptosis. Another study showed that CRT0066101 could inhibit virus replication (39). These studies showed that the compound is well tolerated in mice with no significant side effects, making CRT0066101 an ideal candidate for clinical development.
CID755673
CID755673 (2,3,4,5-Tetrahydro-7-hydroxy-1H-benzofuro[2,3-c] azepin-1-one) was, identified by high-throughput screening, and is a powerful, selective, cell-active PKD inhibitor (Figure 2B). Interestingly, CID755673 inhibits PKD1 with an IC50 of 182 nM and demonstrated selective PKD1 inhibition compared to three different PKC isoforms, AKT, polo-like kinase 1 (PLK1), CDK-activating kinase (CAK), and CAMKII. This compound does not compete with ATP for enzyme inhibition. CID755673 can inhibit cell proliferation and metastasis, phorbol ester-induced nuclear exclusion of class IIa histone deacetylase 5 (HDAC5), and transport of protein from the Golgi to the plasma membrane (22). Furthermore, CID755673 inhibits the pro-angiogenic effect of astragaloside IV by blocking the PKD1-HDAC5-VEGF signaling pathway, to some extent (40). CID755673 can maintain the undifferentiated state of mouse embryonic stem cells (ESCs) when combined with a mitogen-activated protein kinase kinase (MEK) inhibitor by increasing AKT phosphorylation and activating PI3K/AKT signaling (12).
kb-NB142-70 and kb-NB165-09
kb-NB142-70 (Figure 2C) and its methoxy-analog kb-NB165-09 (Figure 2D) are structural analogs of CID755673 with two- to six-fold improved selectivity over CID755637. In particular, modification of the aromatic core significantly increased potency while maintaining high PKD specificity. Both kb-NB142-70 and kb-NB165-09 inhibit the proliferation of prostate cancer cells by inducing G2/M cell cycle arrest. Importantly, these analogs dramatically arrest cell proliferation, increase cytotoxicity, and inhibit cell migration and invasion (41). Although toxicity to tumor cells and inhibition of cell proliferation by these analogs are significantly increased, they are rapidly degraded in vivo, reducing their anti-tumor effects (41). Therefore, further optimization of these analogs required to increase their metabolic stability.
SD-208
The pteridine analog SD-208 is an ATP-competitive pan-PKD SMI (Figure 2E), with IC50s of 107, 94, and 105 nM for PKD1, PKD2, and PKD3, respectively. The first PKD-related SAR (structure-activity relationship) of this structure was established by a series of SD-208 analogs and demonstrated that an electron-deficient aromatic ring is preferred in zone 1 and 4-aminopyridine in zone 2 is essential for activity. SD-208 exhibited a narrow SAR profile with low nanomolar potency. SD-208 is bioactive in vitro and in vivo and can effectively inhibit cells proliferation, and reduce cell invasion and survival of cancer cells (24). However, SD-208 has also been reported as a transforming growth factor βreceptor I (TGF-Bri) inhibitor that blocks tumor invasion and metastasis (42, 43). Therefore, its anti-tumor effects may be mediated in part by its inhibition of (TGF-βRI) (42).
BPKDi
Bipyridyl PKD inhibitor (BPKDi) is a selective PKDi identified through high throughput screening and pharmaceutical chemistry (Figure 2F) (44, 45). BPKDi inhibits all three isoforms of the PKD family, with IC50s of 1, 9, and 1 nM, respectively. PKD promotes the phosphorylation of class IIA HDACs, which induces pathological cardiac hypertrophy (44, 46). BPKDi can abolish the phosphorylation and nuclear export of the class IIa HDACs in cardiomyocytes, concomitantly suppressing hypertrophy of these cells (44).
Other Inhibitors
PKD inhibitors of other chemotypes have been profiled in disease areas beyond cancer. Examples include the 3,5-diarylisoxazoles and 2,6-naphthyridines that potently inhibit PKD and are being investigated as potential treatments for cardiac hypertrophy (47, 48). The 3, 5-diarylisoxazoles are not only active in cell assays of phosphorylation-dependent HDAC5 nuclear export but are, also orally bioavailable, and highly selective compared to a panel of additional putative histone deacetylase (HDAC) kinases (47). The 2,6-naphthyridines have similar effects to the 3,5-diarylisoxazoles, but the PKD selectivity is not very high. Indeed, it also can inhibit PKC to some degree (48). Both 1-NA-PP1 and IKK-16 were identified as pan-PKD inhibitors through a small-scale targeted kinase inhibitor library screen. They are ATP-competitive inhibitors that have high potency and selectivity for PKD. SAR analysis suggests that 1-NA-PP1 is considerably more potent than IKK-16, with distinct substituent effects at the pyrazolopyrimidine core (49). Another compound (3-IN-PP1) is 10-fold more potent than 1-NM-PP1 (the lead compound of 3-IN-PP1), with strong anti-proliferation activity against PANC-1 cells (50, 51).
PKD Inhibitors as Effective Therapeutic Strategies Against Disease
Cancer
Pancreatic ductal adenocarcinoma (PDAC) accounts for 90% of all pancreatic cancers and is considered one of the deadliest cancers with a 5-year survival rate of about 3–5% (52). Many studies have shown that PKD is involved in various pathological processes in pancreatic cancer, including cell proliferation, invasion, energy metabolism, and drug resistance (30, 35, 53). CRT0066101 can inhibit cell proliferation, induce apoptosis, reduce PKD1/2 activity induced by neurotensin, reduce neurotensin-induced PKD-mediated Hsp27 phosphorylation, inhibit NF- κB activation, and block NF-κB-dependent proliferation and survival in pancreatic cancer. CRT0066101 also inhibits the proliferation and growth of human PANC-1 PDAC xenografts, indicating that PKD is a potential therapeutic target for this cancer (23).
Moreover, early pancreatic lesions characterized by ADM, the transdifferentiation of pancreatic acinar cells to a ductal phenotype, are important inducers of pancreatic cancer. AMD may be transformed into pancreatic intraepithelial lesions (PanINs) in the progress of activating Kras mutations or persistent epidermal growth factor receptor (EGF-R) signaling, eventually leading to the occurrence of pancreatic cancer. Liou et al. (54) identified PKD1 as an essential signaling protein downstream of Kras and EGF-R that can drive the formation of such duct-like cells. Interestingly, this process can be reversed by PKD silencing or with PKD inhibitors kb-NB-142-70 and CRT0066101. These studies demonstrated that PKD is an effective drug target, and PKD-specific inhibitors may have important role in the treatment and prognosis of pancreatic cancer patients.
Several studies have shown that various PKD SMIs can inhibit pathological processes in prostate cancer cells. In particular, the PKD inhibitor (CID755673) and its analogs inhibit the proliferation, migration, and invasion of prostate cancer cells by blocking cell cycle progression at G2/M (24, 41). In addition, Chen et al. (55) have suggested that CID755673 and KB-NB142-70 can enhance PMA-induced PARP cleavage in prostate cancer cells by inhibiting PKD activity, thus synergizing with PMA to promote apoptosis.
Unlike PKD1 and PKD2, PKD3 expression is highly upregulated in ER negative breast cancer. Normally, the estrogen receptor (ER) directly binds to the PRKD3 gene promoter to inhibit PKD3 expression. Loss of the ER leads to PKD3 upregulation, resulting in all the hallmarks of aggressive invasive ductal carcinomas (IDC), including tumor progression (36). Targeting PKD in ER-negative breast cancer with the selective PKD inhibitor CRT0066101 or PKD3 knockdown causes decreased growth and metastasis of primary tumors in vivo. These data support the development of PKD inhibitors for clinical use against ER-negative breast cancer (36). Furthermore, PRKD2 and PRKD3 are preferentially expressed in triple-negative breast cancer (TNBC) (56). CRT0066101 inhibits the proliferation of TNBC cells and increases apoptosis and the G1-phase population in vitro. Similarly, this inhibitor reduces breast tumor volume in vivo. These anti-TNBC effects by CRT0066101 are mediated by inhibiting the phosphorylation of MYC, MAPK1/3, AKT, and YAP (56).
PKD-specific inhibitors are also being investigated in various other cancers. For instance, CRTO066101 can cause a dose-dependent suppression of PKD2 activation in colorectal cancer (CRC) cells, resulting in G2/M arrest and apoptosis. The researchers further found a series of signaling events affected by CRT0066101, including PARP cleavage, caspase-3 activation, AKT and ERK signaling suppression, and increased NF-κB activity. Moreover, daily administration of CRT0066101 significantly inhibits the growth of HCT116 xenograft in nude mice. Taken together, these data suggest that PKD plays an important role in regulating growth signals in CRC, and its inhibition by small molecular inhibitors may represent a new treatment for this cancer (37). Additionally, CRT0066101 can suppress bladder cancer growth by inhibiting PKD2 and blocking cell cycle progression at G2/M (57).
All in all, PKD is a likely target in multiple cancers, and PKD SMIs are showing promising results as cancer chemotherapy.
Inflammation
The inflammatory response plays a pivotal role in acute pancreatitis, and studies have shown that PKD is involved in regulating inflammation. Inhibition of PKD by the specific PKD inhibitor CRT0066101 alleviates the symptoms of pancreatitis (58, 59). Specifically, the inhibitors attenuate the inflammatory response and reduce the severity of the disease by inhibiting NF-κB activation, which decreases inflammatory cell infiltration, pancreatic interleukin-6 (IL-6), and monocyte chemoattractant protein-1 (MCP-1) (58). In addition, inflammatory lysophosphatidic acid (LPA) levels increase microglia migration and promote the pro-inflammatory phenotype through the LPAR5/PKD axis (60). When this axis is inhibited by PKD inhibitor CRT0066101, glial cell migration decreases, cytotoxicity is detected, and the expression and secretion of pro-inflammatory mediators are abrogated (60).
Group B streptococcus (GBS) can infect fetal membranes and cause chorioamnionitis during pregnancy, resulting in adverse pregnancy outcomes. This infection is characterized by the release of inflammatory cytokines, the assembly of NLRP3 inflammatory bodies, and the activation of NF-κB. Interestingly, CRT0066101 can inhibit these responses, suggesting that PKD is involved in mediating the immune response of human placental macrophages to GBS (61). Thus, CRT0066101 may represent an important treatment for chorioamnionitis.
Obesity
Obesity is one of the strongest predictors of peripheral insulin resistance (62). AMPK (AMP-activated protein kinase, AMPK) is an energy-sensing enzyme that is inhibited by insulin resistance. PKD is emerging as an important regulator of cellular adaptation to an obese environment (63–65). The specific PKD inhibitor CRT0066101 or PKD1 knockdown can eliminate the inhibitory effect on AMPK, illustrating that PKD1 inhibitor restores AMPK signaling to abolish insulin resistance in muscle cells (66). PKD inhibitor CID755673 can also enhance cardiac function in obese and diabetic patients independent of glucose homeostasis, insulin action, and changes in body composition by inhibiting PKD phosphorylation and expression (67). Taken together, these data suggest that PKD inhibitors can abrogate various complications caused by obesity.
Pathological Hypertrophy
PKD can promote the phosphorylation-dependent nuclear export of HDACs, leading to pathological hypertrophy (46). Therefore, the inhibition of PKD may represent a potential treatment for this disease. Indeed, various specific PKD inhibitors (e.g., BPKDi,3,5-diarylazoles and 2,6-naphthyridine) have been shown to have good efficacy against pathological cardiac hypertrophy, with good bioavailability. PKD inhibition mediated these therapeutic effects by blocking the phosphorylation and nuclear export of HDACs (44, 47, 48).
Metabolic Bone Diseases
Bones serve as scaffolds for the human body. In bone metabolism, osteoclasts and osteoblasts complement each other. When this balance is broken, or bone cell disorders arise, bone tissue destruction occurs, clinically manifesting as bone tuberculosis and malignant bone tumors. Several studies have shown that the PKD family is important for bone metabolism and pathology. Balanced osteoclast and osteoblast activity is necessary for bone health. PKD plays an important role in osteoclast formation. Treatment of bone marrow macrophages with CRT0066101 or CID755673 prevents them from becoming osteoclasts, indicating the importance of PKD in bone health (68). In another study, CRT6600101 carried by nanomaterials reduced IL-1β-induced inflammatory stress in chondrocytes via the NF-κB pathway (69). Overall, PKD inhibitors are a potential treatment for diseases of bone metabolism.
Conclusion and Perspectives
There is increasing evidence that PKD is a vital signaling molecule, and its dysregulation causes various pathological conditions, including cancer, inflammation, and obesity (Table 1). However, the same PKD isoform can have similar or opposite effects in different cancers, and different PKD isoforms can behave differently in the same cancer. In general, PKD can have both anti-cancer and pro-cancer effects in different cells. New evidence indicates that PKD1 acts as a tumor suppressor in breast cancer but is a tumor driver in other cancers (e.g., pancreatic and prostate cancer). Through different mechanisms, PKD1 positively regulates the ERK/MAPK pathway, increases DNA replication, and inhibits apoptosis (70). PKD2 is a pro-tumor protein in various cancers. It activates NF-κB signaling, induces angiogenesis, inhibits apoptosis, and promotes the development of prostate, breast, pancreas, and stomach cancer (32). PRKD3 promotes the proliferation, growth, migration, and invasion of cancer cells in various tumor types (e.g., colorectal, gastric, liver, prostate, and breast cancer), and accumulating evidence suggests that PRKD3 is a promising therapeutic target for cancer treatment (10).
Here, we summarized various PKD SMIs and their applications in various diseases (Figure 3). Although different PKD isoforms may play opposing roles in different pathological processes or even in the same disease, pan-PKD inhibitors have shown antagonistic effects in vivo and in vitro in various disease models. Various PKD inhibitors have progressed the study of various diseases, including cancer, inflammation, and obesity. These PKD specific inhibitors have shown therapeutic effects in preclinical studies and are promising targeted drug candidates.
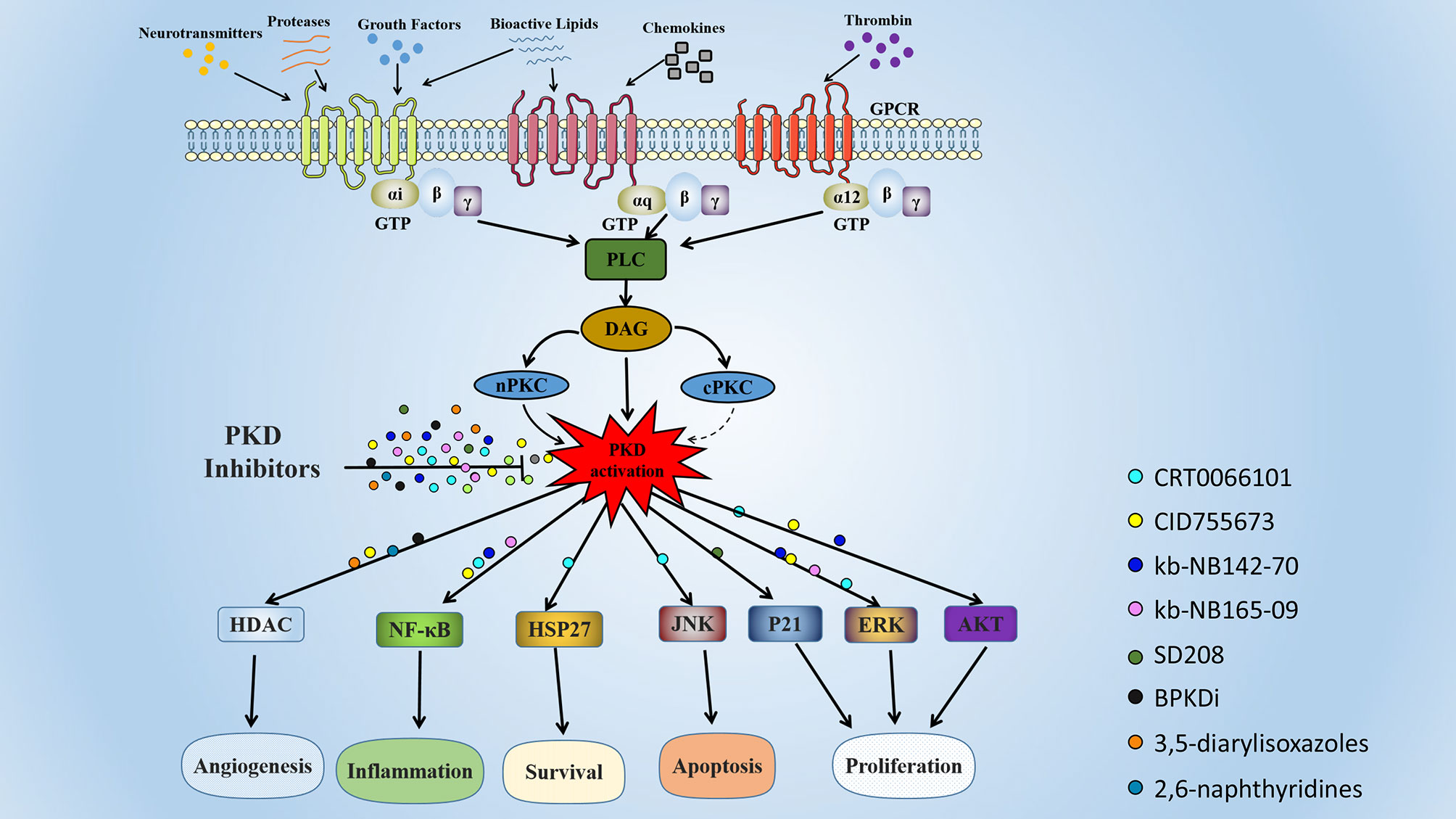
Figure 3 Signaling pathways and biological functions of PKD. The PKDs are activated by many substances, including phorbol ester, platelet-derived growth factor, and G protein-coupled receptor ligands. These extracellular stimuli activate intracellular phospholipase C (PLC) by binding to cell surface receptors. Activated PLC catalyzes the production of intracellular second messenger diacylglycerol (DAG), which recruits PKD and PKC to the plasma membrane. Activated PKC phosphorylates PKD at two serine residues (Ser 738 and 742 in human PKD1), resulting in PKD activation. Activated PKD regulates multiple biological functions, including angiogenesis, inflammation, cell proliferation, apoptosis, and survival. The colored globules represent the different PKD small molecule inhibitors, and the globules on the arrow represent the action of PKD inhibitors in different cellular processes.
Although PKD SMIs have shown therapeutic potential in various disease models, there are no PKD-related inhibitors currently entering clinical trials. Their use in the clinic is limited by two major factors. First, the three PKD isoforms may play different roles in diseases. There is no known comprehensive PKD crystal structure, and all PKD inhibitors to date have been pan-PKD inhibitors (19). Additional research is needed to develop PKD isoform-specific drugs that will make disease treatments more precise. Second, PKD inhibitors have off-target effects to some extent. Like most kinase inhibitors, the PKD inhibitor CRT0066101 has demonstrated activity against many other protein kinases at concentrations of 1 µM and ATP concentrations equivalent to the Km of each kinase (39). Therefore, future research must explore the crystal structure of the catalytic region of the three PKD isoforms to design SMIs with higher selectivity and fewer side effects.
Author Contributions
Visualization, DL, YF, HC, and YK. Writing—original draft, DL, JC, and BC. Writing—review and editing, DL, PZ, ML, and JC. All authors contributed to the article and approved the submitted version.
Funding
This work was supported by the National Natural Science Foundation of China (Grant number 81802717).
Conflict of Interest
The authors declare that the research was conducted in the absence of any commercial or financial relationships that could be construed as a potential conflict of interest.
Abbreviations
PKD, Protein kinase D; CaMK, Calcium/calmodulin-dependent kinase; DAG, Diacylglycerol; SMIs, Small molecule inhibitors; mTORC1-S6K1, Mammalian target of rapamycin complex 1 - S6 kinase 1; OSCC, Oral squamous cell carcinoma cells; HDAC, Histone deacetylase; HSP27, Heat shock protein 27; NF-κB, Nuclear factor kappa-light-chain-enhancer of activated B cells; VEGF, Vascular endothelial growth factor; ESCs, Embryonic stem cells; MEK, Mitogen-activated protein kinase kinase; CDKI, Cyclin-dependent kinase inhibitor; MEF2, Myocyte enhancer factor 2; ANF, Atrial natriuretic factor; b-MyHC, b-myosin heavy chain; a-Sk-actin, a-skeletal actin;PKC, Protein kinase C; SAR, Structure activity relationship; PDAC, Pancreatic ductal adenocarcinoma; PanINs, Pancreatic intraepithelial lesions; EGF-R, Epidermal growth factor receptor; ER, Estrogen receptor; IDC, Invasive ductal carcinomas; TNBC, Triple-negative breast cancer; CRC, Colorectal cancer; ERK, Extracellular regulated protein kinases; IL-6, Interleukin-6; MCP-1, Monocyte chemoattractant protein-1; LPA, Lysophosphatidic acid; LPAR5, LPA-receptor 5; GBS, Group B streptococcus; T2DM, Type 2 diabetes mellitus; AMPK, AMP-activated protein kinase; PLC, Phospholipase C; MMP, matrix metalloproteinase.
References
1. Johannes F, Prestle J, Eis S, Oberhagemann P, Pfizenmaier K. Pkcu is a Novel, Atypical Member of the Protein Kinase C Family. J Biol Chem (1994) 269(8):6140–8. doi: 10.1016/S0021-9258(17)37580-4
2. Valverde A, Sinnett-Smith J, Van Lint J, Rozengurt E. Molecular Cloning and Characterization of Protein Kinase D: A Target for Diacylglycerol and Phorbol Esters With a Distinctive Catalytic Domain. Proc Natl Acad Sci USA (1994) 91(18):8572–6. doi: 10.1073/pnas.91.18.8572
3. Hayashi A, Seki N, Hattori A, Kozuma S, Saito T. Pkcnu, a New Member of the Protein Kinase C Family, Composes a Fourth Subfamily With Pkcmu. Biochim Biophys Acta (1999) 1450(1):99–106. doi: 10.1016/s0167-4889(99)00040-3
4. Sturany S, Van Lint J, Muller F, Wilda M, Hameister H, Hocker M, et al. Molecular Cloning and Characterization of the Human Protein Kinase D2. A Novel Member of the Protein Kinase D Family of Serine Threonine Kinases. J Biol Chem (2001) 276(5):3310–8. doi: 10.1074/jbc.M008719200
5. Steinberg S. Regulation of Protein Kinase D1 Activity. Mol Pharmacol (2012) 81(3):284–91. doi: 10.1124/mol.111.075986
6. Chen J, Cui B, Fan Y, Li X, Li Q, Du Y, et al. Protein Kinase D1 Regulates Hypoxic Metabolism Through HIF-1α and Glycolytic Enzymes Incancer Cells. Oncol Rep (2018) 40(2):1073–82. doi: 10.3892/or.2018.6479
7. Cui B, Chen J, Luo M, Wang L, Chen H, Kang Y, et al. Protein Kinase D3 Regulates the Expression of the Immunosuppressive Protein, PD−L1, Through STAT1/STAT3 Signaling. Int J Oncol (2020) 56(4):909–20. doi: 10.3892/ijo.2020.4974
8. Rashel M, Alston N, Ghazizadeh S. Protein Kinase D1 has a Key Role in Wound Healing and Skin Carcinogenesis. J Invest Dermatol (2014) 134(4):902–9. doi: 10.1038/jid.2013.474
9. Gan H, Mckenzie R, Hao Q, Idell S, Tang H. Protein Kinase D is Increased and Activated in Lung Epithelial Cells and Macrophages in Idiopathic Pulmonary Fibrosis. PloS One (2014) 9(7):e101983. doi: 10.1371/journal.pone.0101983
10. Liu Y, Song H, Zhou Y, Ma X, Xu J, Yu Z, et al. The Oncogenic Role of Protein Kinase D3 in Cancer. J Cancer (2021) 12(3):735–9. doi: 10.7150/jca.50899
11. Durand N, Borges S, Storz P. Functional and Therapeutic Significance of Protein Kinase D Enzymes in Invasive Breast Cancer. Cell Mol Life Sci (2015) 72(22):4369–82. doi: 10.1007/s00018-015-2011-2
12. Zhu Z, Zhang Y, Wang X, Wang X, Ye SD. Inhibition of Protein Kinase D by CID755673 Promotes Maintenance of the Pluripotency of Embryonic Stem Cells. Development (2020) 147(16):dev18526. doi: 10.1242/dev.185264
13. Georgess D, Padmanaban V, Sirka OK, Coutinho K, Choi A, Frid G, et al. Twist1-Induced Epithelial Dissemination Requires Prkd1 Signaling. Cancer Res (2020) 80(2):204–18. doi: 10.1158/0008-5472.Can-18-3241
14. Avriyanti E, Atik N, Kunii M, Furumoto N, Iwano T, Yoshimura S, et al. Functional Redundancy of Protein Kinase D1 and Protein Kinase D2 in Neuronal Polarity. Neurosci Res (2015) 95:12–20. doi: 10.1016/j.neures.2015.01.007
15. Ellwanger K, Pfizenmaier K, Lutz S, Hausser A. Expression Patterns of Protein Kinase D 3 During Mouse Development. BMC Dev Biol (2008) 8:47. doi: 10.1186/1471-213x-8-47
16. Kolczynska K, Loza-Valdes A, Hawro I, Sumara G. Diacylglycerol-Evoked Activation of PKC and PKD Isoforms in Regulation of Glucose and Lipid Metabolism: A Review. Lipids Health Dis (2020) 19(1):113. doi: 10.1186/s12944-020-01286-8
17. Yuan J, Chheda C, Piplani H, Geng M, Tan G, Thakur R, et al. Pancreas-Specific Deletion of Protein Kinase D Attenuates Inflammation, Necrosis, and Severity of Acute Pancreatitis. Biochim Biophys Acta Mol Basis Dis (2020) 1867(1):165987. doi: 10.1016/j.bbadis.2020.165987
18. Cobbaut M, Van Lint J. Function and Regulation of Protein Kinase D in Oxidative Stress: A Tale of Isoforms. Oxid Med Cell Longev (2018) 2018:2138502. doi: 10.1155/2018/2138502
19. Roy A, Ye J, Deng F, Wang QJ. Protein Kinase D Signaling in Cancer: A Friend or Foe? Biochim Biophys Acta Rev Cancer (2017) 1868(1):283–94. doi: 10.1016/j.bbcan.2017.05.008
20. Lavalle C, George K, Sharlow E, Lazo J, Wipf P, Wang Q. Protein Kinase D as a Potential New Target for Cancer Therapy. Biochim Biophys Acta (2010) 1806(2):183–92. doi: 10.1016/j.bbcan.2010.05.003
21. Bedard PL, Hyman DM, Davids MS, Siu LL. Small Molecules, Big Impact: 20 Years of Targeted Therapy in Oncology. Lancet (2020) 395(10229):1078–88. doi: 10.1016/s0140-6736(20)30164-1
22. Sharlow E, Giridhar K, Lavalle C, Chen J, Leimgruber S, Barrett R, et al. Potent and Selective Disruption of Protein Kinase D Functionality by a Benzoxoloazepinolone. J Biol Chem (2008) 283(48):33516–26. doi: 10.1074/jbc.M805358200
23. Harikumar K, Kunnumakkara A, Ochi N, Tong Z, Deorukhkar A, Sung B, et al. A Novel Small-Molecule Inhibitor of Protein Kinase D Blocks Pancreatic Cancer Growth In Vitro and In Vivo. Mol Cancer Ther (2010) 9(5):1136–46. doi: 10.1158/1535-7163.Mct-09-1145
24. Tandon M, Salamoun J, Carder E, Farber E, Xu S, Deng F, et al. Sd-208, a Novel Protein Kinase D Inhibitor, Blocks Prostate Cancer Cell Proliferation and Tumor Growth In Vivo by Inducing G2/M Cell Cycle Arrest. PloS One (2015) 10(3):e0119346. doi: 10.1371/journal.pone.0119346
25. Roy A, Ye J, Deng F, Wang Q. Protein Kinase D Signaling in Cancer: A Friend or Foe? Biochim Biophys Acta Rev Cancer (2017) 1868(1):283–94. doi: 10.1016/j.bbcan.2017.05.008
26. Wood B, Bossuyt J. Emergency Spatiotemporal Shift: The Response of Protein Kinase D to Stress Signals in the Cardiovascular System. Front Pharmacol (2017) 8:9. doi: 10.3389/fphar.2017.00009
27. Rozengurt E, Rey O, Waldron R. Protein Kinase D Signaling. J Biol Chem (2005) 280(14):13205–8. doi: 10.1074/jbc.R500002200
28. Wang Q. PKD at the Crossroads of DAG and PKC Signaling. Trends Pharmacol Sci (2006) 27(6):317–23. doi: 10.1016/j.tips.2006.04.003
29. Storz P, Döppler H, Johannes F, Toker A. Tyrosine Phosphorylation of Protein Kinase D in the Pleckstrin Homology Domain Leads to Activation. J Biol Chem (2003) 278(20):17969–76. doi: 10.1074/jbc.M213224200
30. Kumari S, Khan S, Sekhri R, Mandil H, Behrman S, Yallapu M, et al. Protein Kinase D1 Regulates Metabolic Switch in Pancreatic Cancer Via Modulation of Mtorc1. Br J Cancer (2020) 122(1):121–31. doi: 10.1038/s41416-019-0629-9
31. Hao Q, Wang L, Zhao Z, Tang H. Identification of Protein Kinase D2 as a Pivotal Regulator of Endothelial Cell Proliferation, Migration, and Angiogenesis. J Biol Chem (2009) 284(2):799–806. doi: 10.1074/jbc.M807546200
32. Azoitei N, Cobbaut M, Becher A, Van Lint J, Seufferlein T. Protein Kinase D2: A Versatile Player in Cancer Biology. Oncogene (2018) 37(10):1263–78. doi: 10.1038/s41388-017-0052-8
33. Huck B, Duss S, Hausser A, Olayioye MA. Elevated Protein Kinase D3 (PKD3) Expression Supports Proliferation of Triple-Negative Breast Cancer Cells and Contributes to mTORC1-S6K1 Pathway Activation. J Biol Chem (2014) 289(6):3138–47. doi: 10.1074/jbc.M113.502633
34. Borges S, Döppler H, Perez E, Andorfer C, Sun Z, Anastasiadis P, et al. Pharmacologic Reversion of Epigenetic Silencing of the PRKD1 Promoter Blocks Breast Tumor Cell Invasion and Metastasis. Breast Cancer Res BCR (2013) 15(2):R66. doi: 10.1186/bcr3460
35. Guha S, Tanasanvimon S, Sinnett-Smith J, Rozengurt E. Role of Protein Kinase D Signaling in Pancreatic Cancer. Biochem Pharmacol (2010) 80(12):1946–54. doi: 10.1016/j.bcp.2010.07.002
36. Borges S, Perez E, Thompson E, Radisky D, Geiger X, Storz P. Effective Targeting of Estrogen Receptor-Negative Breast Cancers With the Protein Kinase D Inhibitor Crt0066101. Mol Cancer Ther (2015) 14(6):1306–16. doi: 10.1158/1535-7163.Mct-14-0945
37. Wei N, Chu E, Wipf P, Schmitz J. Protein Kinase D as a Potential Chemotherapeutic Target for Colorectal Cancer. Mol Cancer Ther (2014) 13(5):1130–41. doi: 10.1158/1535-7163.Mct-13-0880
38. Wei N, Chu E, Wu SY, Wipf P, Schmitz JC. The Cytotoxic Effects of Regorafenib in Combination With Protein Kinase D Inhibition in Human Colorectal Cancer Cells. Oncotarget (2015) 6(7):4745–56. doi: 10.18632/oncotarget.2938
39. Guedán A, Swieboda D, Charles M, Toussaint M, Johnston SL, Asfor A, et al. Investigation of the Role of Protein Kinase D in Human Rhinovirus Replication. J Virol (2017) 91(9):dev185264. doi: 10.1128/jvi.00217-17
40. Yang L, Liu N, Zhao W, Li X, Han L, Zhang Z, et al. Angiogenic Function of Astragaloside IV in Rats With Myocardial Infarction Occurs Via the PKD1-HDAC5-VEGF Pathway. Exp Ther Med (2019) 17(4):2511–8. doi: 10.3892/etm.2019.7273
41. Guo J, Clausen D, Beumer J, Parise R, Egorin M, Bravo-Altamirano K, et al. In Vitro Cytotoxicity, Pharmacokinetics, Tissue Distribution, and Metabolism of Small-Molecule Protein Kinase D Inhibitors, kb-NB142-70 and kb-NB165-09, in Mice Bearing Human Cancer Xenografts. Cancer Chemother Pharmacol (2013) 71(2):331–44. doi: 10.1007/s00280-012-2010-z
42. Uhl M, Aulwurm S, Wischhusen J, Weiler M, Ma JY, Almirez R, et al. Sd-208, a Novel Transforming Growth Factor Beta Receptor I Kinase Inhibitor, Inhibits Growth and Invasiveness and Enhances Immunogenicity of Murine and Human Glioma Cells In Vitro and In Vivo. Cancer Res (2004) 64(21):7954–61. doi: 10.1158/0008-5472.Can-04-1013
43. Mohammad KS, Javelaud D, Fournier PG, Niewolna M, Mckenna CR, Peng XH, et al. TGF-beta-RI Kinase Inhibitor SD-208 Reduces the Development and Progression of Melanoma Bone Metastases. Cancer Res (2011) 71(1):175–84. doi: 10.1158/0008-5472.Can-10-2651
44. Monovich L, Vega RB, Meredith E, Miranda K, Rao C, Capparelli M, et al. A Novel Kinase Inhibitor Establishes a Predominant Role for Protein Kinase D as a Cardiac Class IIa Histone Deacetylase Kinase. FEBS Lett (2010) 584(3):631–7. doi: 10.1016/j.febslet.2009.12.014
45. Meredith EL, Beattie K, Burgis R, Capparelli M, Chapo J, Dipietro L, et al. Identification of Potent and Selective Amidobipyridyl Inhibitors of Protein Kinase D. J Med Chem (2010) 53(15):5422–38. doi: 10.1021/jm100076w
46. Avkiran M, Rowland A, Cuello F, Haworth R. Protein Kinase D in the Cardiovascular System: Emerging Roles in Health and Disease. Circ Res (2008) 102(2):157–63. doi: 10.1161/circresaha.107.168211
47. Gamber G, Meredith E, Zhu Q, Yan W, Rao C, Capparelli M, et al. 3,5-Diarylazoles as Novel and Selective Inhibitors of Protein Kinase D. Bioorg Med Chem Lett (2011) 21(5):1447–51. doi: 10.1016/j.bmcl.2011.01.014
48. Meredith E, Ardayfio O, Beattie K, Dobler M, Enyedy I, Gaul C, et al. Identification of Orally Available Naphthyridine Protein Kinase D Inhibitors. J Med Chem (2010) 53(15):5400–21. doi: 10.1021/jm100075z
49. Tandon M, Johnson J, Li Z, Xu S, Wipf P, Wang Q. New Pyrazolopyrimidine Inhibitors of Protein Kinase D as Potent Anticancer Agents for Prostate Cancer Cells. PloS One (2013) 8(9):e75601. doi: 10.1371/journal.pone.0075601
50. Verschueren K, Cobbaut M, Demaerel J, Saadah L, Voet A, Van Lint J, et al. Discovery of a Potent Protein Kinase D Inhibitor: Insights in the Binding Mode of Pyrazolo[3,4-]Pyrimidine Analogues. Medchemcomm (2017) 8(3):640–6. doi: 10.1039/c6md00675b
51. Gilles P, Kashyap R, Freitas M, Ceusters S, Van Asch K, Janssens A, et al. Design, Synthesis and Biological Evaluation of Pyrazolo[3,4-D]Pyrimidine-Based Protein Kinase D Inhibitors. Eur J Med Chem (2020) 205:112638. doi: 10.1016/j.ejmech.2020.112638
52. Adamska A, Domenichini A, Falasca M. Pancreatic Ductal Adenocarcinoma: Current and Evolving Therapies. Int J Mol Sci (2017) 18(7):1338. doi: 10.3390/ijms18071338
53. Ferdaoussi M, Bergeron V, Zarrouki B, Kolic J, Cantley J, Fielitz J, et al. G Protein-Coupled Receptor (GPR)40-Dependent Potentiation of Insulin Secretion in Mouse Islets Is Mediated by Protein Kinase D1. Diabetologia (2012) 55(10):2682–92. doi: 10.1007/s00125-012-2650-x
54. Liou G, Döppler H, Braun U, Panayiotou R, Scotti Buzhardt M, Radisky D, et al. Protein Kinase D1 Drives Pancreatic Acinar Cell Reprogramming and Progression to Intraepithelial Neoplasia. Nat Commun (2015) 6:6200. doi: 10.1038/ncomms7200
55. Chen J, Giridhar KV, Zhang L, Xu S, Wang QJ. A Protein Kinase C/protein Kinase D Pathway Protects LNCaP Prostate Cancer Cells From Phorbol Ester-Induced Apoptosis by Promoting ERK1/2 and NF-κB Activities. Carcinogenesis (2011) 32(8):1198–206. doi: 10.1093/carcin/bgr113
56. Liu Y, Wang Y, Yu S, Zhou Y, Ma X, Su Q, et al. The Role and Mechanism of CRT0066101 as an Effective Drug for Treatment of Triple-Negative Breast Cancer. Cell Physiol Biochem (2019) 52(3):382–96. doi: 10.33594/000000027
57. Li Q, Hsu I, Sanford T, Railkar R, Balaji N, Sourbier C, et al. Protein Kinase D Inhibitor CRT0066101 Suppresses Bladder Cancer Growth In Vitro and Xenografts Via Blockade of the Cell Cycle at G2/M. Cell Mol Life Sci (2018) 75(5):939–63. doi: 10.1007/s00018-017-2681-z
58. Thrower E, Yuan J, Usmani A, Liu Y, Jones C, Minervini S, et al. A Novel Protein Kinase D Inhibitor Attenuates Early Events of Experimental Pancreatitis in Isolated Rat Acini. Am J Physiol Gastrointest Liver Physiol (2011) 300(1):G120–129. doi: 10.1152/ajpgi.00300.2010
59. Yuan J, Tan T, Geng M, Tan G, Chheda C, Pandol S. Novel Small Molecule Inhibitors of Protein Kinase D Suppress NF-kappaB Activation and Attenuate the Severity of Rat Cerulein Pancreatitis. Front Physiol (2017) 8:1014. doi: 10.3389/fphys.2017.01014
60. Plastira I, Bernhart E, Goeritzer M, Devaney T, Reicher H, Hammer A, et al. Lysophosphatidic Acid Via LPA-Receptor 5/Protein Kinase D-dependent Pathways Induces a Motile and Pro-Inflammatory Microglial Phenotype. J Neuroinflamm (2017) 14(1):253. doi: 10.1186/s12974-017-1024-1
61. Sutton J, Rogers L, Dixon B, Kirk L, Doster R, Algood H, et al. Protein Kinase D Mediates Inflammatory Responses of Human Placental Macrophages to Group B Streptococcus. Am J Reprod Immunol (2019) 81(3):e13075. doi: 10.1111/aji.13075
62. Lee D, Keum N, Hu F, Orav E, Rimm E, Willett W, et al. Comparison of the Association of Predicted Fat Mass, Body Mass Index, and Other Obesity Indicators With Type 2 Diabetes Risk: Two Large Prospective Studies in US Men and Women. Eur J Epidemiol (2018) 33(11):1113–23. doi: 10.1007/s10654-018-0433-5
63. Bergeron V, Ghislain J, Vivot K, Tamarina N, Philipson L, Fielitz J, et al. Deletion of Protein Kinase D1 in Pancreatic β-Cells Impairs Insulin Secretion in High-Fat Diet-Fed Mice. Diabetes (2018) 67(1):71–7. doi: 10.2337/db17-0982
64. Löffler M, Mayer A, Trujillo Viera J, Loza Valdes A, El-Merahbi R, Ade C, et al. Protein Kinase D1 Deletion in Adipocytes Enhances Energy Dissipation and Protects Against Adiposity. EMBO J (2018) 37(22):e99182. doi: 10.15252/embj.201899182
65. Mayer A, Löffler M, Loza Valdés A, Schmitz W, El-Merahbi R, Viera J, et al. The Kinase PKD3 Provides Negative Feedback on Cholesterol and Triglyceride Synthesis by Suppressing Insulin Signaling. Sci Signal (2019) 12(593):eaav9150. doi: 10.1126/scisignal.aav9150
66. Coughlan K, Valentine R, Sudit B, Allen K, Dagon Y, Kahn B, et al. Pkd1 Inhibits Ampkα2 Through Phosphorylation of Serine 491 and Impairs Insulin Signaling in Skeletal Muscle Cells. J Biol Chem (2016) 291(11):5664–75. doi: 10.1074/jbc.M115.696849
67. Venardos K, De Jong K, Elkamie M, Connor T, Mcgee S. The PKD Inhibitor CID755673 Enhances Cardiac Function in Diabetic Db/Db Mice. PloS One (2015) 10(3):e0120934. doi: 10.1371/journal.pone.0120934
68. Leightner A, Mello Guimaraes Meyers C, Evans M, Mansky K, Gopalakrishnan R, Jensen E. Regulation of Osteoclast Differentiation at Multiple Stages by Protein Kinase D Family Kinases. Int J Mol Sci (2020) 21(3):1056. doi: 10.3390/ijms21031056
69. Cho H, Bhatti F, Hasty K, Yi A. Nanosome-Mediated Delivery of Protein Kinase D Inhibitor Protects Chondrocytes From Interleukin-1β-Induced Stress and Apoptotic Death. Int J Nanomed (2019) 14:8835–46. doi: 10.2147/ijn.S218901
Keywords: protein kinase D, small-molecule inhibitor, function, therapeutics, diseases
Citation: Lv D, Chen H, Feng Y, Cui B, Kang Y, Zhang P, Luo M and Chen J (2021) Small-Molecule Inhibitor Targeting Protein Kinase D: A Potential Therapeutic Strategy. Front. Oncol. 11:680221. doi: 10.3389/fonc.2021.680221
Received: 13 March 2021; Accepted: 07 June 2021;
Published: 24 June 2021.
Edited by:
Qingxin Mu, University of Washington, United StatesReviewed by:
Pirjo Spuul, Tallinn University of Technology, EstoniaStephen J. Pandol, Cedars Sinai Medical Center, United States
Copyright © 2021 Lv, Chen, Feng, Cui, Kang, Zhang, Luo and Chen. This is an open-access article distributed under the terms of the Creative Commons Attribution License (CC BY). The use, distribution or reproduction in other forums is permitted, provided the original author(s) and the copyright owner(s) are credited and that the original publication in this journal is cited, in accordance with accepted academic practice. No use, distribution or reproduction is permitted which does not comply with these terms.
*Correspondence: Jiao Chen, Y2owMTIzQDE2My5jb20=