- 1Human Cancer Genomic Research, Research Center, King Faisal Specialist Hospital and Research Center, Riyadh, Saudi Arabia
- 2Department of Surgery, King Faisal Specialist Hospital and Research Center, Riyadh, Saudi Arabia
- 3Department of Pathology, King Faisal Specialist Hospital and Research Centre, Riyadh, Saudi Arabia
Mitogen-activated protein kinase kinase 1 (MAP2K1) is a dual specificity protein kinase that phosphorylates both threonine and tyrosine residues in ERK. MAP2K1 mutations have been identified in several cancers. However, their role in Middle Eastern papillary thyroid cancer (PTC) and colorectal cancer (CRC) is lacking. In this study, we evaluated the prevalence of MAP2K1 mutations in a large cohort of Middle Eastern PTC and CRC using whole-exome and Sanger sequencing technology. In the discovery cohort of 100 PTC and 100 CRC cases (comprising 50 MAPK mutant and 50 MAPK wildtype cases each), we found one MAP2K1 mutation each in PTC and CRC, both of which were MAPK wildtype. We further analyzed 286 PTC and 289 CRC MAPK wildtype cases and found three MAP2K1 mutant PTC cases and two MAP2K1 mutant CRC cases. Thus, the overall prevalence of MAP2K1 mutation in MAPK wildtype cases was 1.1% (4/336) in PTC and 0.9% (3/339) in CRC. Histopathologically, three of the four MAP2K1 mutant PTC cases were follicular variant and all four tumors were unifocal with absence of extra-thyroidal extension. All the three CRC cases harboring MAP2K1 mutation were of older age (> 50 years) and had moderately differentiated stage II/III tumors located in the left colon. In conclusion, this is the first comprehensive report of MAP2K1 somatic mutations prevalence in PTC and CRC from this ethnicity. The mutually exclusive nature of MAP2K1 and MAPK mutations suggests that each of these mutation may function as an initiating mutation driving tumorigenesis through MAPK signaling pathway.
Introduction
Mitogen-activated protein kinase (MAPK) pathway has been known to play an important role in the pathogenesis and survival of many tumors, especially thyroid and colorectal cancers (1–4). Genetic alterations that aberrantly activate this kinase pathway in cancers are typically the result of BRAF or KRAS mutations in the majority of cancers displaying MAPK activation (5–7). It is known that BRAF is not the only downstream kinase in the MAPK pathway subject to mutation and subsequent MAPK activation. Several reports have identified oncogenic mutations in MAPK kinase 1 (MAP2K1, also called MEK1) as alternative mechanism for MAPK pathway activation in BRAF wild-type tumor in various cancers (8–11). Mutations in MAP2K1, a primary downstream effector of RAF kinases, are uncommon and subsequently tumors harboring these mutations need to be explored, especially in patients who might be treated with MEK and RAF targeted therapy.
MAP2K1 encodes a dual specific serine/threonine and tyrosine kinase, activated in response to phosphorylation by RAF kinase (12). MAP2K1 mutations and deletions in the activation segment have been shown to constitutively activate the protein (13, 14). Mutations of MAP2K1 have been reported in several human cancers, especially melanoma, Langerhans histocytosis, hairy cell leukemia and lung adenocarcinoma (9–11, 15, 16). Most of the reported MAP2K1 mutations tend to be mutually exclusive with other MAPK driver mutations (8, 9, 15). Mutations affecting the N-terminal negative regulatory domain encoded by exon 2 and the catalytic core encoded by exon 3 accounted for majority of previously reported MAP2K1 mutations (9, 17–19).
Despite the major role of MAPK pathway in colorectal cancer (CRC) and papillary thyroid cancer (PTC), there are few reports about the prevalence of MAP2K1 mutations in these tumor sites (17, 20–22). More importantly, data on MAP2K1 mutations in PTC and CRC from Arab Middle Eastern ethnicity is lacking. Therefore, we sought to determine the prevalence of MAP2K1 mutations in a large cohort of Middle Eastern PTC and CRC. In the discovery cohort, we performed whole-exome sequencing on a discovery cohort of 100 PTC samples and 100 CRC samples. We then validated our findings in set of additional 286 PTC and 289 CRC using Sanger sequencing analysis.
Materials and Methods
Patient Selection and Tumor Samples
The initial discovery cohort included 100 cases each of PTC and CRC (50 MAPK (BRAF/KRAS/NRAS/HRAS) mutant and 50 MAPK wildtype) diagnosed at King Faisal Specialist Hospital and Research Centre (KFSHRC). Subsequent validation cohort consisted of 286 PTC and 289 CRC samples, all of which lacked mutations in the MAPK genes. Clinico-pathological data were collected from case records, the details of which are summarized in Tables 1 and 2. Institutional Review Board of KFSHRC provided ethical approval for the current study. Research Advisory Council (RAC) granted waiver of informed consent for use of retrospective patient case data and archival tissue samples under project RAC# 2110 031 and 2190 016.
DNA Isolation
DNA samples were extracted from formalin-fixed and paraffin-embedded (FFPE) CRC tumor tissues utilizing Gentra DNA Isolation Kit (Gentra, Minneapolis, MN, USA) according to the manufacturer’s protocols as elaborated in the previous studies (23).
Whole-Exome Sequencing Analysis
DNA samples were analyzed by whole exome sequencing using Illumina Novaseq. Sequencing reads in fastq format were mapped to the human genome version 19 using Burrows-Wheeler Aligner (BWA) (24). PCR duplicate marking, local realignment and base-quality recalibration were performed with Picard tools (http://broadinstitute.github.io/picard/) and GATK (25).
Single nucleotide variants (SNVs) and indels were called with MuTect (26), and VarScan2 (http://varscan.sourceforge.net) respectively. Annotation of somatic variants was performed using ANNOVAR (27). The SNVs that passed the standard Mutect and VarScan2 filters were retained, and common SNPs with minor allele frequency (MAF) of > 0.001 in dbSNP, the NHLBI exome sequencing project, 1000 Genomes and our in-house exome database of around 800 normals were removed for further analysis. Somatic SNVs were manually checked using Integrated Genomics Viewer (IGV) to filter out the artifacts.
Sanger Sequencing Analysis
Sanger sequencing technology was utilized to sequence entire coding and splicing regions of exons 2 and 3 in MAP2K1 gene among 286 MAPK wildtype PTC and 289 MAPK wildtype CRC samples as validation cohort. In addition, the pathogenic variants detected by Exome sequencing analysis were further confirmed by Sanger sequencing analysis. Primer 3 online software was utilized to design the primers (available upon request). PCR and Sanger sequencing analysis were carried out as described previously (28). Reference sequences were downloaded from the NCBI GenBank and sequencing results were compared with the reference sequences by Mutation Surveyor V4.04 (Soft Genetics, LLC, State College, PA).
Pathogenicity Assessment of Mutations
Mutations were characterized as pathogenic according to The American College of Medical Genetics and Genomics (ACMG) guidelines or found functional in The Clinical Knowledgebase database (https://ckb.jax.org/). The remaining mutations were termed pathogenic if found deleterious by two of the three in silico algorithms (SIFT, PolyPhen, and MutationTaster).
Results
MAP2K1 Mutation in PTC and CRC
In the discovery cohort of PTC cases consisting of 50 MAPK wildtype and 50 MAPK mutant cases, one inframe deletion (p.I99_K104del) of MAP2K1 was identified in a MAPK wildtype case, accounting for 2% (1/50) of all MAPK wildtype PTC cases. No mutation was detected in MAPK mutant PTC cases. This mutation was reported as gain of function mutation by The Clinical Knowledgebase (CKB, https://ckb.jax.org). Furthermore, in the discovery cohort of CRC cases, one missense mutation, p.93V>A, was detected in one (2%) of 50 MAPK wildtype cases, while no mutation was found in 50 MAPK mutant cases. This mutation was predicted as pathogenic mutation by SIFT and MutationTaster (Table 3 and Figure 1).
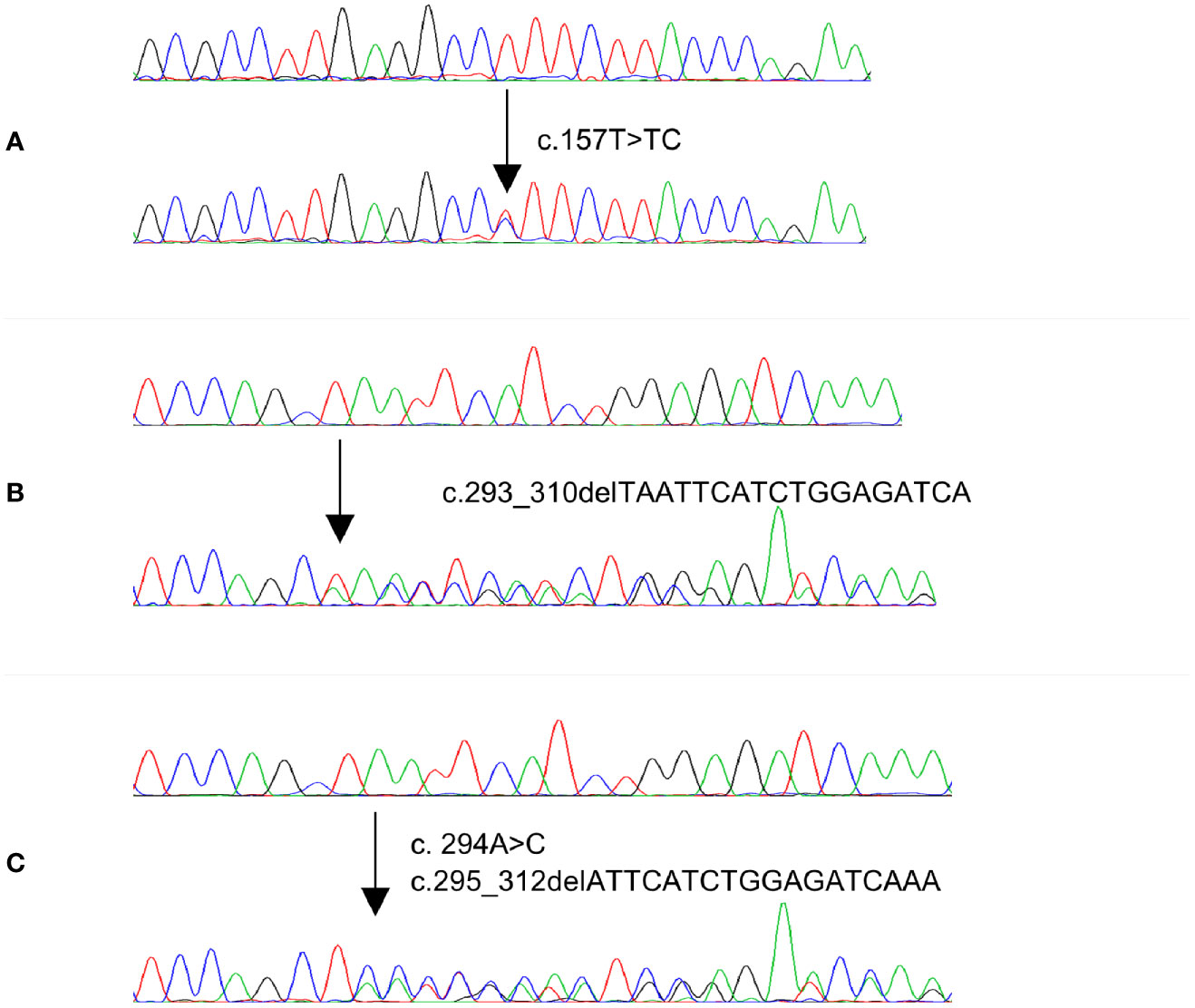
Figure 1 Electropherogram of three MAP2K1 representative mutations identified in PTC and CRC cases. Upper traces represent normal sequences while lower traces show mutated sequences.
Since MAP2K1 mutations were only identified in MAPK wildtype cases, we further analyzed 286 MAPK wildtype PTC and 289 MAPK wildtype CRC cases as validation cohort. Among 286 PTC cases, three cases (1.0%) carried gain of function mutations, while in 289 CRC cases, two (0.7%) cases harbored gain of function mutations of MAP2K1 gene. Altogether, four out of 336 (1.1%) MAPK wildtype PTC cases carried gain of function mutations while three out of 339 (0.9%) MAPK wildtype CRC cases carried mutations (Table 3 and Figure 1). Among five mutations identified in our study, one was novel mutation while four were reported as somatic mutations in COSMIC (Supplementary Table 1).
Clinico-Pathological Characteristics of MAP2K1 Mutant PTC and CRC Cases
Among the four PTC cases harboring MAP2K1 mutation, the median age was 51 years (range: 30–70 years). The mutations were distributed equally among male and female PTC patients (two each). 75% (3/4) of PTCs with MAP2K1 mutation were of follicular variant and 25% (1/4) were classical variant. 75% (3/4) of the tumors harboring MAP2K1 mutation were encapsulated with only one case showing tumor capsule invasion. None of the cases showed vascular invasion or extra-thyroidal extension. All the tumors were unifocal. 75% (3/4) of patients had stage I PTC and one patient had stage IV with distant metastasis to the brain. On follow-up (median: 5.5 years, range: 1–9 years), the patient with distant metastasis died due to disease progression, whereas the other three patients had no evidence of disease (Table 4).
Among the three CRC cases harboring MAP2K1 mutation, the median age was 79 years (range: 60–81 years). All patients were male. All three patients had moderately differentiated left sided tumors. One patient presented with stage II disease and two patients presented with stage III disease. One of the tumors showed mismatch repair deficiency by immunohistochemistry (Table 5).
Discussion
Targeted therapies have emerged as a promising cancer therapeutic option due to their effectiveness in treating “oncogene-addicted” cancers (29, 30). Therefore, accurate prediction of anti-tumor effects of molecularly targeted agents before clinical trial design and implementation in cancer patients is important to achieve the goal of precision medicine. Many studies investigating the effect of inhibitors of the MAPK pathways in thyroid and colorectal cancers highlight the importance of identifying mutations in such signaling pathway and their impacts on the subsequent efficacy of targeted therapies, thus reinforcing the importance of better personalized therapeutic strategies (31–34). In this study, we identified the prevalence of MAP2K1 mutations in Middle Eastern PTC and CRC.
MAP2K1 mutations have been reported in several types of tumors, including CRC and PTC (9, 11, 15, 17, 20, 21, 35). The majority of previously reported MAP2K1 mutations or deletions targeted Exon 2 and 3 which encode the negative regulatory domain and the catalytic core (9, 17–19). Due to the recent easy access to next generation sequencing, we sought to analyze a small discovery cohort of 100 PTC and 100 CRC samples using exome sequencing to identify MAP2K1 mutations and their correlation to other MAPK pathway mutations, especially since recent reports have shown mutual exclusivity between MAP2K1 mutations and BRAFV600E mutations in these organ sites (17, 21). We have identified one case of inframe deletion in Exon 3 of PTC cohort and one CRC case carrying a missense mutation in Exon 2. Each of the above mentioned mutations occurred in a BRAF wild-type context, consistent with the notion that BRAF & MAP2K1 are acting in the same transformation pathway.
We then further expanded our study to include additional 286 PTC and 289 CRC samples as validation cohort. We found overall mutations in MAP2K1 occurring in 1.1% (4/336) of PTC and 0.9% (3/339) CRC MAPK wildtype cohorts. MAP2K1 mutations have been classified into 3 classes (36). Class 1 MAP2K1 mutations are RAF dependent and are least activating. Class 2 MAP2K1 mutations are activating in nature but can be upregulated further by upstream RAF. Class 3 MAP2K1 mutations lead to auto-phosphorylation of MEK which is independent of RAF and usually is mutually exclusive with other mutations that activate MAPK signaling and therefore considered driver mutations. Previous report has shown the ability of class 3 mutation in vivo to promote tumor growth more efficiently than class 1 and class 2 mutations (36).
In this study, we detected MAP2K1 mutation in 4/336 (1.1%) PTCs that otherwise had no known MAPK pathway genetic alterations. All mutations identified in our PTC cohort were in-frame deletions/class 3 mutations and located in the kinase domain encoded by Exon 3. These deletions cause gain of MAP2K1 function as demonstrated by activity, independent of Raf and increased phosphorylation of Mek and Erk relative to wild-type MAP2K1 (37) (CKB database, https://ckb.jax.org) and have been reported previously (8, 36, 38, 39). Interestingly, the missense mutation c.157T>TC;p.53F>F/L within the negative regulatory region was also reported as gain of MAP2K1 function due to increase of Erk and Mek phosphorylation (16). Although MAP2K1 mutated PTCs show no predilection to gender, stage and grade, majority (75%; 3/4) were encapsulated and showed follicular pattern. All of the MAP2K1 mutated cases were unifocal and showed absence of any extra-thyroidal extension. Upon follow-up, only one adverse event was registered.
Till date, only two reports have been published about the incidence of MAP2K1 mutations in PTC. First published report was limited to MAP2K1 Exon 2 mutations which was not present in any of the PTC cases tested (20). Second, more recent report, examined Exon 2 and 3 and identified MAP2K1 mutation in 2% of their 101 PTC cohort which is higher than the frequency of mutations in our cohort (1.1%), but the difference was not statistically significant (17). Similar to our results, all PTC mutations identified were limited to Exon 3 (catalytic core) and all tumors showed similar histology where all the cases harboring MAP2K1 mutations were encapsulated, had predominantly follicular architecture and were intra-thyroidal with no lymphovascular invasion. In contrast to our study, TCGA reported MAP2K1 mutations in only 0.2% (1/482) PTC cases (40, 41).
Furthermore, our study has shown MAP2K1 mutation in 0.9% (3/339) of CRCs, all mutually exclusive with other MAPK driver mutations. One mutation was classified as Class 2 MAP2K1 mutation and was similar to previous studies (36), whereas one Class 3 mutation (p.E102_I103del) was identified in our cohort. The Cancer Genome Atlas (TCGA) has reported MAP2K1 mutations in a high percentage (2.4%; 13/549) of CRC cases (40, 42, 43), while other studies have shown incidence varying from 1% to 2% (20–22). All patients harboring MAP2K1 mutation in our cohort were older than 50 years with moderately differentiated tumors. Compared to previous studies (21, 41, 44), the clinico-pathological characteristics of MAP2K1 mutant CRC cases in our cohort differed with respect to gender distribution, tumor location and tumor stage.
The limitation of the present study is the low number of MAP2K1 mutant cases in both PTC (4/336, 1.2%) and CRC (3/339, 0.9%) and are not adequate for performing valid statistical associations. Subsequent multicenter study is needed to identify significant associations of these variants in Middle Eastern population.
In conclusion, this is the first comprehensive report of MAP2K1 somatic mutations prevalence in PTC and CRC from this ethnicity. The mutually exclusive nature of MAP2K1 and BRAF mutations suggests that each of these mutation may function as an initiating mutation driving tumorigenesis through MAPK signaling pathway. This might have important clinical implications for the use of BRAF and MEK inhibitor therapies in a subset of Middle Eastern PTC and CRC patients.
Data Availability Statement
The raw sequencing data has been deposited in Sequence Read Archive (SRA) database of National Center for Biotechnology Information (NCBI) under accession number PRJNA726736 (https://www.ncbi.nlm.nih.gov/bioproject/PRJNA726736).
Ethics Statement
The studies involving human participants were reviewed and approved by Research Advisory Council (RAC). The ethics committee waived the requirement of written informed consent for participation.
Author Contributions
RB, AS, and TM designed the study, performed targeted capture sequencing analysis, and helped write the manuscript. SP and KI analyzed the data. MA-R, WH, MD, IV, and SA were involved in performing the experiments. SA-S and FA-D provided the clinical resources and executed the study. KA-K designed the study, supervised the study, and drafted the manuscript. All authors contributed to the article and approved the submitted version.
Conflict of Interest
The authors declare that the research was conducted in the absence of any commercial or financial relationships that could be construed as a potential conflict of interest.
Supplementary Material
The Supplementary Material for this article can be found online at: https://www.frontiersin.org/articles/10.3389/fonc.2021.670423/full#supplementary-material
References
1. Braicu C, Buse M, Busuioc C, Drula R, Gulei D, Raduly L, et al. A Comprehensive Review on MAPK: A Promising Therapeutic Target in Cancer. Cancers (Basel) (2019) 11:1618. doi: 10.3390/cancers11101618
2. Yuan J, Dong X, Yap J, Hu J. The MAPK and AMPK Signalings: Interplay and Implication in Targeted Cancer Therapy. J Hematol Oncol (2020) 13:113. doi: 10.1186/s13045-020-00949-4
3. Zaballos MA, Santisteban P. Key Signaling Pathways in Thyroid Cancer. J Endocrinol (2017) 235:R43–61. doi: 10.1530/JOE-17-0266
4. Koveitypour Z, Panahi F, Vakilian M, Peymani M, Seyed Forootan F, Nasr Esfahani MH, et al. Signaling Pathways Involved in Colorectal Cancer Progression. Cell Biosci (2019) 9:97. doi: 10.1186/s13578-019-0361-4
5. Drosten M, Barbacid M. Targeting the MAPK Pathway in KRAS-Driven Tumors. Cancer Cell (2020) 37:543–50. doi: 10.1016/j.ccell.2020.03.013
6. Morkel M, Riemer P, Blaker H, Sers C. Similar But Different: Distinct Roles for KRAS and BRAF Oncogenes in Colorectal Cancer Development and Therapy Resistance. Oncotarget (2015) 6:20785–800. doi: 10.18632/oncotarget.4750
7. Turski ML, Vidwans SJ, Janku F, Garrido-Laguna I, Munoz J, Schwab R, et al. Genomically Driven Tumors and Actionability Across Histologies: BRAF-Mutant Cancers as a Paradigm. Mol Cancer Ther (2016) 15:533–47. doi: 10.1158/1535-7163.MCT-15-0643
8. Chakraborty R, Hampton OA, Shen X, Simko SJ, Shih A, Abhyankar H, et al. Mutually Exclusive Recurrent Somatic Mutations in MAP2K1 and BRAF Support a Central Role for ERK Activation in LCH Pathogenesis. Blood (2014) 124:3007–15. doi: 10.1182/blood-2014-05-577825
9. Arcila ME, Drilon A, Sylvester BE, Lovly CM, Borsu L, Reva B, et al. MAP2K1 (MEK1) Mutations Define a Distinct Subset of Lung Adenocarcinoma Associated With Smoking. Clin Cancer Res (2015) 21:1935–43. doi: 10.1158/1078-0432.CCR-14-2124
10. Lian T, Li C, Wang H. Trametinib in the Treatment of Multiple Malignancies Harboring MEK1 Mutations. Cancer Treat Rev (2019) 81:101907. doi: 10.1016/j.ctrv.2019.101907
11. Williams EA, Montesion M, Shah N, Sharaf R, Pavlick DC, Sokol ES, et al. Melanoma With in-Frame Deletion of MAP2K1: A Distinct Molecular Subtype of Cutaneous Melanoma Mutually Exclusive From BRAF, NRAS, and NF1 Mutations. Mod Pathol (2020) 33:2397–406. doi: 10.1038/s41379-020-0581-5
12. Ohren JF, Chen H, Pavlovsky A, Whitehead C, Zhang E, Kuffa P, et al. Structures of Human MAP Kinase Kinase 1 (MEK1) and MEK2 Describe Novel Noncompetitive Kinase Inhibition. Nat Struct Mol Biol (2004) 11:1192–7. doi: 10.1038/nsmb859
13. Cowley S, Paterson H, Kemp P, Marshall CJ. Activation of MAP Kinase Kinase is Necessary and Sufficient for PC12 Differentiation and for Transformation of NIH 3T3 Cells. Cell (1994) 77:841–52. doi: 10.1016/0092-8674(94)90133-3
14. Mansour SJ, Candia JM, Gloor KK, Ahn N. Constitutively Active Mitogen-Activated Protein Kinase Kinase 1 (MAPKK) and MAPKK2 Mediate Similar Transcriptional and Morphological Responses. Cell Growth Differ (1996) 7:243–50.
15. Brown NA, Furtado LV, Betz BL, Kiel MJ, Weigelin HC, Lim MS, et al. High Prevalence of Somatic MAP2K1 Mutations in BRAF V600E–Negative Langerhans Cell Histiocytosis. Blood (2014) 124:1655–8. doi: 10.1182/blood-2014-05-577361
16. Waterfall JJ, Arons E, Walker RL, Pineda M, Roth L, Killian JK, et al. High Prevalence of MAP2K1 Mutations in Variant and IGHV4-34–Expressing Hairy-Cell Leukemias. Nat Genet (2014) 46:8–10. doi: 10.1038/ng.2828
17. Borrelli N, Panebianco F, Condello V, Barletta JA, Kaya C, Yip L, et al. Characterization of Activating Mutations of the MEK1 Gene in Papillary Thyroid Carcinomas. Thyroid (2019) 29:1279–85. doi: 10.1089/thy.2019.0065
18. Zhou J, Yang H, Deng Q, Gu X, He P, Lin Y, et al. Oncogenic Driver Mutations in Patients With Non-Small-Cell Lung Cancer At Various Clinical Stages. Ann Oncol (2013) 24:1319–25. doi: 10.1093/annonc/mds626
19. Kris MG, Johnson BE, Berry LD, Kwiatkowski DJ, Iafrate AJ, Wistuba II, et al. Using Multiplexed Assays of Oncogenic Drivers in Lung Cancers to Select Targeted Drugs. JAMA (2014) 311:1998–2006. doi: 10.1001/jama.2014.3741
20. Murugan AK, Dong J, Xie J, Xing M. MEK1 Mutations, But Not ERK2 Mutations, Occur in Melanomas and Colon Carcinomas, But None in Thyroid Carcinomas. Cell Cycle (2009) 8:2122–4. doi: 10.4161/cc.8.13.8710
21. Chuang J, Wang C, Guo Y, Valenzuela V, Wu J, Fakih M. MAP2K1 Mutations in Advanced Colorectal Cancer Predicts a Poor Response to Anti-EGFR Therapy and to Vertical Targeting of the MAPK Pathway. Clin Colorectal Cancer (2020) 20:72–8. doi: 10.1016/j.clcc.2020.12.003
22. Jing C, Li H, Du Y, Cao H, Liu S, Wang Z, et al. MEK Inhibitor Enhanced the Antitumor Effect of Oxaliplatin and 5−Fluorouracil in MEK1 Q56P−Mutant Colorectal Cancer Cells. Mol Med Rep (2019) 19:1092–100. doi: 10.3892/mmr.2018.9730
23. Abubaker J, Jehan Z, Bavi P, Sultana M, Al-Harbi S, Ibrahim M, et al. Clinicopathological Analysis of Papillary Thyroid Cancer With PIK3CA Alterations in a Middle Eastern Population. J Clin Endocrinol Metab (2008) 93:611–8. doi: 10.1210/jc.2007-1717
24. Li H, Durbin R. Fast and Accurate Long-Read Alignment With Burrows–Wheeler Transform. Bioinformatics (2010) 26:589–95. doi: 10.1093/bioinformatics/btp698
25. McKenna A, Hanna M, Banks E, Sivachenko A, Cibulskis K, Kernytsky A, et al. The Genome Analysis Toolkit: A Mapreduce Framework for Analyzing Next-Generation DNA Sequencing Data. Genome Res (2010) 20:1297–303. doi: 10.1101/gr.107524.110
26. Cibulskis K, Lawrence MS, Carter SL, Sivachenko A, Jaffe D, Sougnez C, et al. Sensitive Detection of Somatic Point Mutations in Impure and Heterogeneous Cancer Samples. Nat Biotechnol (2013) 31:213–9. doi: 10.1038/nbt.2514
27. Wang K, Li M, Hakonarson H. ANNOVAR: Functional Annotation of Genetic Variants From High-Throughput Sequencing Data. Nucleic Acids Res (2010) 38:e164. doi: 10.1093/nar/gkq603
28. Bu R, Siraj AK, Al-Obaisi KA, Beg S, Al Hazmi M, Ajarim D, et al. Identification of Novel BRCA Founder Mutations in Middle Eastern Breast Cancer Patients Using Capture and Sanger Sequencing Analysis. Int J Cancer (2016) 139:1091–7. doi: 10.1002/ijc.30143
29. Torti D, Trusolino L. Oncogene Addiction as a Foundational Rationale for Targeted Anti-Cancer Therapy: Promises and Perils. EMBO Mol Med (2011) 3:623–36. doi: 10.1002/emmm.201100176
30. Sharma SV, Settleman J. Oncogene Addiction: Setting the Stage for Molecularly Targeted Cancer Therapy. Genes Dev (2007) 21:3214–31. doi: 10.1101/gad.1609907
31. Dienstmann R, Tabernero J. Spectrum of Gene Mutations in Colorectal Cancer: Implications for Treatment. Cancer J (2016) 22:149–55. doi: 10.1097/PPO.0000000000000191
32. Ryan MB, Corcoran RB. Therapeutic Strategies to Target RAS-Mutant Cancers. Nat Rev Clin Oncol (2018) 15:709–20. doi: 10.1038/s41571-018-0105-0
33. Crispo F, Notarangelo T, Pietrafesa M, Lettini G, Storto G, Sgambato A, et al. BRAF Inhibitors in Thyroid Cancer: Clinical Impact, Mechanisms of Resistance and Future Perspectives. Cancers (Basel) (2019) 11:1388. doi: 10.3390/cancers11091388
34. Falchook GS, Millward M, Hong D, Naing A, Piha-Paul S, Waguespack SG, et al. BRAF Inhibitor Dabrafenib in Patients With Metastatic BRAF-Mutant Thyroid Cancer. Thyroid (2015) 25:71–7. doi: 10.1089/thy.2014.0123
35. Schmidt J, Ramis-Zaldivar JE, Nadeu F, Gonzalez-Farre B, Navarro A, Egan C, et al. Mutations of MAP2K1 are Frequent in Pediatric-Type Follicular Lymphoma and Result in ERK Pathway Activation. Blood (2017) 130:323–7. doi: 10.1182/blood-2017-03-776278
36. Gao Y, Chang MT, McKay D, Na N, Zhou B, Yaeger R, et al. Allele-Specific Mechanisms of Activation of MEK1 Mutants Determine Their Properties. Cancer Discov (2018) 8:648–61. doi: 10.1158/2159-8290.CD-17-1452
37. Kohsaka S, Hayashi T, Nagano M, Ueno T, Kojima S, Kawazu M, et al. Identification of Novel CD74-NRG2α Fusion From Comprehensive Profiling of Lung Adenocarcinoma in Japanese Never or Light Smokers. J Thorac Oncol (2020) 15:948–61. doi: 10.1016/j.jtho.2020.01.021
38. Wang C, Sandhu J, Fakih M. A Case of Class 3 MEK1 Mutated Metastatic Colorectal Cancer With a Non-Durable Tumor Marker Response to MEK and ERK Inhibitors. J Gastrointest Oncol (2019) 10:1140–3. doi: 10.21037/jgo.2019.08.02
39. Yuan J, Ng WH, Tian Z, Yap J, Baccarini M, Chen Z, et al. Activating Mutations in MEK1 Enhance Homodimerization and Promote Tumorigenesis. Sci Signal (2018) 11:eaar6795. doi: 10.1126/scisignal.aar6795
40. Hoadley KA, Yau C, Hinoue T, Wolf DM, Lazar AJ, Drill E, et al. Cell-of-Origin Patterns Dominate the Molecular Classification of 10,000 Tumors From 33 Types of Cancer. Cell (2018) 173:291–304.e6. doi: 10.1016/j.cell.2018.03.022
41. Cerami E, Gao J, Dogrusoz U, Gross BE, Sumer SO, Aksoy BA, et al. The Cbio Cancer Genomics Portal: An Open Platform for Exploring Multidimensional Cancer Genomics Data. Cancer Discovery (2012) 2:401–4. doi: 10.1158/2159-8290.CD-12-0095
42. Weinstein JN, Collisson EA, Mills GB, Shaw KR, Ozenberger BA, Ellrott K, et al. The Cancer Genome Atlas Pan-Cancer Analysis Project. Nat Genet (2013) 45:1113–20. doi: 10.1038/ng.2764
43. Ciriello G, Miller ML, Aksoy BA, Senbabaoglu Y, Schultz N, Sander C. Emerging Landscape of Oncogenic Signatures Across Human Cancers. Nat Genet (2013) 45:1127–33. doi: 10.1038/ng.2762
Keywords: mutation, MAP2K1, papillary thyroid cancer, colorectal cancer, somatic
Citation: Bu R, Siraj AK, Masoodi T, Parvathareddy SK, Iqbal K, Al-Rasheed M, Haqawi W, Diaz M, Victoria IG, Aldughaither SM, Al-Sobhi SS, Al-Dayel F and Al-Kuraya KS (2021) Recurrent Somatic MAP2K1 Mutations in Papillary Thyroid Cancer and Colorectal Cancer. Front. Oncol. 11:670423. doi: 10.3389/fonc.2021.670423
Received: 21 February 2021; Accepted: 12 April 2021;
Published: 11 May 2021.
Edited by:
Obul Reddy Bandapalli, Hopp Children’s Cancer Center Heidelberg (KiTZ), GermanyReviewed by:
Shaolei Teng, Howard University, United StatesBeifang Niu, Chinese Academy of Sciences (CAS), China
Copyright © 2021 Bu, Siraj, Masoodi, Parvathareddy, Iqbal, Al-Rasheed, Haqawi, Diaz, Victoria, Aldughaither, Al-Sobhi, Al-Dayel and Al-Kuraya. This is an open-access article distributed under the terms of the Creative Commons Attribution License (CC BY). The use, distribution or reproduction in other forums is permitted, provided the original author(s) and the copyright owner(s) are credited and that the original publication in this journal is cited, in accordance with accepted academic practice. No use, distribution or reproduction is permitted which does not comply with these terms.
*Correspondence: Khawla S. Al-Kuraya, a2t1cmF5YUBrZnNocmMuZWR1LnNh
†These authors have contributed equally to this work