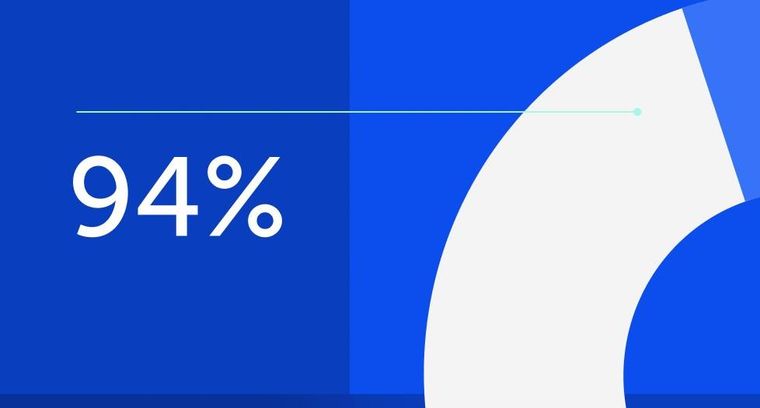
94% of researchers rate our articles as excellent or good
Learn more about the work of our research integrity team to safeguard the quality of each article we publish.
Find out more
ORIGINAL RESEARCH article
Front. Oncol., 19 October 2021
Sec. Molecular and Cellular Oncology
Volume 11 - 2021 | https://doi.org/10.3389/fonc.2021.670292
Hepatocellular carcinoma is one of the cancers with the highest mortality rate worldwide. HCC is often diagnosed when the disease is already in an advanced stage, making the discovery and implementation of biomarkers for the disease a critical aim in cancer research. In this study, we aim to quantify the transcript levels of key signaling molecules relevant to different pathways known to participate in tumorigenesis, with special emphasis on those related to cancer hallmarks and epithelial-mesenchymal transition, using as a model the murine transplantable hepatocarcinoma AS-30D. Using qPCR to quantify the mRNA levels of genes involved in tumorigenesis, we found elevated levels for Tgfb1 and Spp1, two master regulators of EMT. A mesenchymal signature profile for AS-30D cells is also supported by the overexpression of genes encoding for molecules known to be associated to aggressiveness and metastatic phenotypes such as Foxm1, C-met, and Inppl1. This study supports the use of the AS-30D cells as an efficient and cost-effective model to study gene expression changes in HCC, especially those associated with the EMT process.
Nowadays, hepatocellular carcinoma (HCC) is considered among the most frequent and aggressive cancer types, listed as the fourth cause of death worldwide when related to cancer (1). Considering the multifactorial character of cancer progression, its study becomes a challenging task that needs valuable models to study basic mechanisms related to proliferation, resistance to apoptosis, angiogenesis, and epithelial-mesenchymal transition (EMT), all hallmark features for the establishment and progression of cancer (2). In this study, employing the rat AS-30D hepatocarcinoma, we explore these hallmarks and phenotypical features of this model that are still unknown.
Established originally as a transplantable hepatoma model, the AS-30D cell, got its name because its ability to produce ascites fluid after 1 week of being inoculated, composed of cells of epithelial origin, morphologically similar to hepatocytes growing in clusters (3). Observations using electron microscopy show structures typical of epithelial cells, such as desmosomes and tight junctions, among others. Recent work shows that AS-30D cells injected directly into the liver of rats, develop solid tumors in defined sites of the liver parenchyma after 7 and up to 14 days of inoculation (4). Although neovascularization was confirmed after 7 days of inoculation, there is no evidence for the establishment of lung micrometastases even at day 14. As corresponding to highly malignant tumors, AS-30D cells show high glycolytic activity mediated by the type II hexokinase that becomes overexpressed after the promoter is demethylated (5, 6). Regarding the expression of cytokines regulating liver growth, AS-30D cells respond properly to TGF-β, and in comparison with normal hepatocytes, the Smad transcriptional corepressors Ski and SnoN, show larger half-lives (7). Another relevant signaling pathway present in the AS-30D model is PI3K-AKT-mTOR as mediator of the resistance of HCC to thermal ablative therapy (8), where the induction of EGFR signaling pathway has been demonstrated to be involved under heat stress conditions (9).
One of the main points of this work, is to explore the expression of osteopontin (OPN), also called secreted phosphoprotein 1 (SPP1, as the encoding gene), considered an extracellular matrix glycoprotein abundant in bones and teeth. In the last two decades, OPN function has been revisited, and nowadays, it is known to be a versatile molecule regulating several cellular processes such as migration, proliferation, apoptosis, and immune responses, among others (10, 11). According to this wide range of functions, OPN is expressed as several isoforms generated by alternative splicing. The protein also might go through several posttranslational modifications or can be processed by proteolytic cleavage into functional fragments.
OPN is overexpressed in several pathological processes affecting the liver, such as fibrosis, nonalcoholic or alcoholic fatty liver disease, cirrhosis, and cancer (12–14). It has been shown that liver samples from patients suffering these diseases, Spp1 transcripts are enriched (15–18). The neutralization of OPN through antibodies or aptamers as a resource to successfully treat liver fibrosis has been shown (15). Importantly, OPN has been proposed as a prognosis molecule and biomarker when associated to HCC in both, the mouse and the human (19–22). Moreover, specific splice variants can differentially influence the progression and prognosis of the disease (23). During the early stages of cancer establishment where local inflammatory processes occur, OPN interacts with receptors such as CD44 and integrins, stimulating cell adhesion and migration, and therefore contributing to metastasis.
During our study, in an attempt to better understand the development of HCC, we used the hepatoma model AS-30D to analyze the expression pattern of Spp1 transcripts and other genes critical in cancer progression and metastasis. These genes cover fundamental hallmarks in cancer as EMT, angiogenesis, cell proliferation, and extracellular protein matrix processing. While EMT involves the major cell trans-differentiation critical for cancer cell migration and metastasis (23), under normal conditions, EMT is essential during embryogenesis. Nevertheless, during metastatic process EMT is reactivated allowing cells to inhibit the normal cell-cell adhesion, and therefore becoming mobile and invasive cells (24, 25). Moreover, since it is well known that calcium homeostasis is altered in cancer cells (26), and a series of molecules involved with EMT such as cadherins and OPN, present a calcium-dependent activation, we also investigated if changes in genes codifying for the plasma membrane calcium ATPases (PMCA) named Atp2b1-Atp2b4, that in normal conditions maintain intracellular Ca2+ at the nanomolar range, could present a correlation with possible changes on Spp1 expression. Supported on a series of studies carried out by our research group over the years (26–29), we hold the possibility that this correlation might be of key importance in the establishment of HCC and further progression of the disease.
The Oncomine database was used to explore the expression of osteopontin (SPP1) in human samples derived from normal liver and HCC (https://www.oncomine.org) (30). For the analysis, we used two datasets: Mas et al. (31), which includes 19 normal and 38 HCC samples, and Chen et al. (32), which includes 75 normal and 105 HCC samples. We also used Oncomine to compare the expression levels in normal and HCC samples of the genes included in this work: VEGFA, ANGPT1, FOXM1, MET, INPPL1, EGRF, TGFB1, MMP2, CDH2, ACTA2, CD44, ITGA3, ITGA5, ATP2B1, ATP2B2, ATP2B3, and ATP2B4. We used the dataset from Chen et al. (32), except for ITGA5, which is not included in this microarray; then, for ITGA5, we presented the data from Mas et al. (31).
All salts and buffers employed were from Sigma-Aldrich (St. Louis, MO, USA). The Trizol reagent was from Thermo Fisher Scientific (Waltham, MA, USA). The iScript cDNA synthesis kit was purchased from Bio-Rad (Hercules, CA, USA) and PowerUp Sybr Green Master Mix 2X from Applied Biosystems (Foster City, CA, USA). Several primers were used as reported previously (33–35) or designed using the platform of the Universal Probe Library from Roche (Pleasanton, CA, USA) (Table 1).
Experimental animals were handled according to the Mexican Official Norm for Laboratory Animals (NOM-062-ZOO-1999) and the procedures approved by the Animal Care and Use Committee of our Institute (Protocol JMO119(89)-17). To expand the transplantable hepatocarcinoma, a vial containing ~3 × 106 AS-30D cells/ml preserved in Dulbecco’s modified medium plus 10% dimethyl sulfoxide was inoculated intraperitoneally to male Wistar rats (n = 4) weighing 200 g. After 1 week, rats developed ascites that was collected as described before (27). In order to eliminate erythrocytes, the ascitic fluid was washed several times with a buffer containing 150 mM NaCl, 5 mM KCl, and 20 mM Tris-HCl (pH 7.4) by centrifugation at 1,000×g for 10 min at 4°C. Clean pellets were resuspended in PBS or in Trizol, then frozen in liquid nitrogen and stored at −70°C until use. Fresh hepatocytes were isolated from male Wistar rats (n = 3) weighing 250 g as previously described (28). Briefly, rats were anesthetized intramuscularly with ketamine and xylazine (40–80 and 5–10 mg/kg, respectively). After perfusion of the liver with Krebs-Ringer solution containing 0.05% collagenase (Worthington, Lakewood, NJ, USA), the obtained cell suspension was filtered and centrifuged at 500 rpm during 2 min. Hepatocytes were recovered from the pellet, washed with Krebs-Ringer solution and snap frozen in liquid nitrogen and stored at −70°C.
Total RNA was obtained with Trizol, following manufacturer’s instructions, and quality of RNA samples was verified in 1.5% agarose gels. In order to synthesize cDNA, 1 μg of RNA was used for reverse transcription and reactions set using iScript RT Supermix as recommended by the provider. cDNA obtained from different tissues or cells was used for qPCR together with the PowerUp Sybr Green Master Mix 2X, according to manufacturer’s instructions. qPCR reactions and dissociation analysis were performed in an ABI PRISM 7000 Sequence Detection equipment from Applied Biosystems according to the standard cycling program suggested by the manufacturer, comprising a step for UDG inactivation for 2 min at 50°C, a dual-lock DNA polymerase step for 2 min at 95°C, and 40 cycles including two steps for 15 s at 95°C and for 1 min at 60°C. A preliminary validation was performed, including hepatic C9 cells and fresh hepatocytes; hepatocytes were determined appropriate as reference of normal expression of the analyzed transcripts. The housekeeping gene Rpl13 (60S ribosomal protein L13) was used as reference and qPCR data were analyzed with the 2−ΔΔCt method.
All samples were measured in triplicates; mean values, standard deviations (SD), and t-test comparison were calculated using the Graphpad Prism 8 software (San Diego, CA, USA). Statistically significant differences were those with *p-values ≤0.05; **p ≤ 0.01; ***p ≤ 0.001; and ****p ≤ 0.0001.
Fresh hepatocytes and AS-30D cells were lysed with RIPA buffer plus a cocktail of protease inhibitors (cOmplet protease Inhibitors, Roche, Basel, CHE) and phosphatase inhibitors (1 mM NaF, 1 mM NaVO3); 100 μg of total protein extracts were run in SDS-PAGE as described before (36). Antibodies used were the following ones: vimentin 5741, pSmad2 3108 (Cell Signaling Technology, Danvers, MA, USA), osteopontin AF808 (R&D Systems, Minneapolis, MN, USA), PMCA 1-4 sc-20028 (Santa Cruz Biotechnology, Dallas, TX, USA), and β-actin A2228 (Sigma-Aldrich, St. Louis MO, USA). Recombinant Human TGF-β was obtained from PeproTech (Rocky Hill, NJ, USA). AS-30D cells were cultured in DMEM with 10% FCS; cells were incubated with 2 ng/ml of TGF-β for 24 or 48 h.
First, to obtain information related to the latest findings on the relevance of SPP1 in HCC, we compared the expression of this gene in normal liver and HCC samples using the database Oncomine (30). The level of SPP1 expression in HCC samples was consistently higher in HCC compared with normal liver; specifically, 7.5 times higher in the dataset from Mas et al. (31) and 4.0 times higher in the dataset from Chen et al. (32) (Figure 1). To facilitate a comparison between human HCC and the hepatoma AS-30D, we obtained gene expression profile of all the genes analyzed in this study, using Oncomine with the dataset of Chen et al. (32) (Supplementary Figure S1).
Figure 1 Comparative expression of SPP1 in human HCC samples compared with normal liver. SPP1 expression is 7.5-fold higher in HCC than in normal liver. Box plot obtained from Oncomine database (30).
Since the characterization of Spp1 is still not available in HCC developed in the rat, we proceeded to quantify Spp1, and other genes related to HCC progression using the AS-30D cells as a model of HCC. cDNA from AS-30D hepatoma cells obtained from different specimens was prepared and amplified by qPCR in parallel with samples obtained from fresh rat hepatocytes, used as a basal expression control. For clarity purposes, the analyzed genes were grouped according to the specific physiological processes where they participate.
As part of this group of genes, Vegfα and Angpt1 transcripts were studied finding a contrasting pattern between them (Figure 2). Vegfα mRNA showed a consistent higher expression in AS-30D samples in comparison with normal fresh hepatocytes, while Angpt1 mRNA was highly expressed in normal hepatocytes, and lightly expressed in hepatoma cells.
Figure 2 Expression of genes involved in angiogenesis in rat hepatocytes and AS-30D cells. Rat hepatocytes were freshly isolated, and AS-30D samples were obtained from cells from the ascitic fluid of experimental rats. Data represented as mean ± SD. ***p ≤ 0.001; and ****p ≤ 0.0001.
In this group of genes, we found a higher expression of FoxM1, C-met, and Inppl1 (Figure 3). FoxM1 gene encodes a protein corresponding to an important transcription factor for cell cycle progression and therefore, cancer (37). C-met that is overexpressed in cancer, encodes for the hepatocyte-growth factor receptor, a major regulator during embryogenesis and wound healing. Inppl1 encodes for a phosphatase negatively regulating P13K pathways through the hydrolysis of the 5-phosphate of phosphatidylinositol-3,4,5-trisphosphate to convert it in phosphatidylinositol-4,5-diphosphate. This pathway is indicative of a high metabolic activity compatible with an aggressive type of cancer (38, 39). On the other hand, Egfr, that encodes for the epidermal growth factor receptor, is clearly underexpressed in hepatoma cells with respect to normal hepatocytes.
Figure 3 Expression of several genes related to metabolic processes or regulation of cell cycle in rat hepatocytes and AS-30D cells. Rat hepatocytes were freshly isolated, and AS-30D samples were obtained from cells isolated the ascitic fluid of experimental rats. Data represented as mean ± SD. *p-values ≤0.05; **p ≤0.01; ***p ≤ 0.001.
Based on the fact that TGF-β is recognized as a major inductor of EMT (40), we explored the expression of this cytokine in our model. Interestingly, mainly Tgfb1 and Spp1 mRNA are highly overexpressed in the hepatoma cells with respect to the levels observed in normal fresh hepatocytes (Figure 4). In an opposite manner, mRNA levels of some EMT markers such as Mmp2, Cdh2, and Acta2 are virtually absent in the ascites tumor cells.
Figure 4 Expression of genes encoding EMT markers in rat hepatocytes and AS-30D cells. Rat hepatocytes were freshly isolated, and AS-30D samples were obtained from cells from the ascitic fluid of experimental rats. Data represented as mean ± SD. *p-values ≤0.05; **p ≤0.01; ***p ≤ 0.001; and ****p ≤ 0.0001.
We analyzed Cd44, and several other members of the family of integrins, recognized to be the canonical receptors for OPN (10). Although Cd44 showed the tendency to increase in AS-30D cells, the difference was not statistically significant. Regarding integrins, we analyzed those especially relevant in the context of cancer. Itgb3 or integrin β3 mRNA, shows similar levels of expression between fresh hepatocytes and AS-30D cells, while Itga5 or integrin αV mRNA, shows a lower expression in AS-30D cells (Figure 5).
Figure 5 Gene expression of canonical receptors recognizing OPN in rat hepatocytes and AS-30D cells. Rat hepatocytes were freshly isolated, and AS-30D samples were obtained from cells from the ascitic fluid of experimental rats. Data represented as mean ± SD.
Taking into consideration that the control of cell calcium homeostasis has been known to be critical factor for the survival of most eukaryotic cells, we analyzed the expression of several PMCAs that contribute to maintain Ca2+ concentration at the nanomolar range. From these PMCA isoforms, a number of them have been recognized to play an important role not only in normal but also in neoplastic cells.
We observed that the housekeeping isoform Atp2b1 (PMCA1) represents the isoform showing the highest level of expression with an important difference between AS-30D cells and normal hepatocytes (Figure 6). Although the expression of Atp2b4, also considered to be a housekeeping isoform, shows a level several orders of magnitude smaller, an increased level of this isoform is again found in tumor cells. Regarding the less abundant isoforms, Atp2b2 showed a significant decrease in tumor cells, while Atp2b3 showed an increasing but not significant trend in hepatoma cells.
Figure 6 Expression of genes encoding for the plasma membrane Ca2+-ATPases (Atp2b1-Atp2b4) in rat hepatocytes and AS-30D cells. Rat hepatocytes were freshly isolated and AS-30D samples were obtained from cells from the ascitic fluid of experimental rats. Data represented as mean ± SD. *p-values ≤0.05; **p ≤0.01.
Next, we proceeded to corroborate that the differences in mRNA concentration for some of the genes analyzed in normal hepatocytes and AS-30D cells were also reflected at the protein level. We measured by Western blotting the protein levels for PMCA1-4 and OPN. Our results show an elevated expression of PMCA and OPN in AS-30D cells compared with normal hepatocytes (Figure 7A). We expect this band corresponds to PMCA1, the isoform most abundant in liver. Similar results were obtained for OPN, showing higher protein levels detected in AS-30D cells compared with normal hepatocytes. These results enable us to corroborate that in the hepatoma AS-30D, there is a correlation between the high mRNA and protein levels detected for PMCA and OPN.
Figure 7 AS-30D cells express high protein levels of PMCA and OPN and increase the expression of mesenchymal markers after TGF-β stimulation. (A) Protein levels for PMCA and OPN in hepatocytes and AS-30D cells were detected by Western blot. One hundred micrograms of protein (first lane) and 200 μg (second lane) were run for each cell type. (B) AS-30D cells were treated with 2 ng/ml of TGF-β for 24 and 48 h OPN, vimentin, and phospho-Smad2 protein levels were detected by Western blot. One hundred micrograms of protein were run in each lane. β-Actin was used as a loading control.
Finally, with the purpose to study if the AS-30D cells could be considered a suitable model in vitro to study EMT, we analyzed the effect of TGF-β, a potent inducer of EMT, upon the expression OPN and vimentin. AS-30D cells treated with TGF-β increased the expression of the mesenchymal markers OPN and vimentin, with the highest OPN expression at 24 h and vimentin at 48 h after TGF-β stimulation (Figure 7B). The activation of the TGF-β pathway was corroborated by the detection of phospho-Smad2 24 h after treatment.
Our results for the first time present a quantitative analysis of several genes encoding for cancer hallmarks employing the hepatocarcinoma transplantable model AS-30D. The use of this model and the analysis performed studying genes related to signaling molecules that mediate EMT, allowed us to propose that changes in the overall expression of these genes might be critical during cancer progression and important in the establishment of metastasis. AS-30D cells show high glycolytic activity and vascularization properties (3, 4), two essential hallmarks for HCC. Also, AS-30D cells are able to produce ascites, reported in HCC as a feature associated with bad prognosis, in rats 7 days after being inoculated. As in humans, the intraperitoneal accumulation of ascites in rats is related to the development of different etiologies where also kidney malfunction and sodium retention are involved (41). In a similar fashion, the presence of ascites in patients with HCC has been related to tumoral and cirrhosis factors, and a low survival rate (42).
Currently, there is not an animal model in use in the laboratory that exactly reproduces all the characteristics of HCC in humans. This is not surprising as it is cancer with a great degree of heterogeneity, in part due to the several etiologies that can cause it: chronic inflammation, viral infection, aflatoxin toxicity, alcohol, obesity, among others (43). However, our model of hepatoma AS-30D has similarities in the expression patterns for normal and cancer tissues for genes as FoxM1, Met, and especially Spp1 (Supplementary Figure S1). The gene profiles presented in this panel correspond to the dataset from Chen et al. (32), and other similarities can be found when using different datasets.
There are some advantages for using AS-30D cells over other models employed in the study of HCC. For example, the AS-30D model requires just a single inoculation to develop tumoral cells in the ascites after 1 week, making the procedure simple, cost-effective, and less time-consuming. These are all ideal characteristics for a model to be used in animals in high-throughput screenings for drug discovery.
Angiogenesis, the production of new blood vessels, allows the tumor to spread and to become metastatic. For this process to occur, permeability changes in the vascular endothelium mediated by proinflammatory cytokines, and growth factors such as VEGFα have to develop (2). In agreement with these observations, we found that cells collected from the ascites fluid overexpressed Vegfα when compared with hepatocytes, whereas Angpt1 shows the opposite pattern. In lung tumors, it has been shown that angiopoietin-like factors also present this kind of behavior (44). Interestingly, an elevated expression of Angpt1 in the normal hepatocyte, resembles the pattern observed during proliferation in livers undergoing the process of regeneration (45).
Linked to a proliferative, invasive, and metastatic phenotype in HCC (37, 45, 46), we further detected high levels of expression of factors essential for tumor progression such as FoxM1, C-met, and Inppl1 in AS-30D cells compared with normal hepatocytes. While FoxM1 and C-met are related to the active proliferative ability of cells in different types of cancer (46, 47), Inppl1 is associated to a bad prognosis in HCC patients (38, 39). These data are consistent with the aggressiveness observed for the AS-30D model. On the other hand, in the context of HCC development, the epidermal growth factor receptor (EGFR) modulates relevant aspects of tumor progression such as proliferation, apoptosis, and metastasis (48). It has been shown to be overexpressed in HCC, and it is associated with a poor prognosis (49). In our model, we observed Egfr to present a low level of expression in comparison with normal hepatocytes. It has been reported that EGRF expression levels are heterogeneous in HCC, especially in tumor samples with metastatic features (50). In our case, this downregulation seems to allow HCC cells to undergo an EMT process and acquire a motile and migratory phenotype. Nevertheless, another possibility might be that we had detected a specific isoform of the molecule or even an EGFR mutant, both recognized as main contributors for the specific signaling pathway (51). Thus, it will be necessary in the near future to further investigate if the receptor also shows a low expression, and to explore in a closer detail, the activation state and modulatory mechanisms of this pathway with special emphasis on the crosstalk between EGFR and TGF-β. Also, since there are in the literature examples where transcripts levels do not correlate well with protein expression (9), we shall have to also study this scenario.
Our results suggest that TGF-β could play a central role during EMT in AS-30D cells via OPN. The expression of the mesenchymal markers OPN and vimentin induced by TGF-β, is a clear sign that AS-30D cells can undergo an EMT. The increase in OPN, will could lead to synergistic effect with the TGF-β in EMT, to potentiate the expression of mesenchymal markers. Accordingly, it has been reported that in epithelial cells, EMT is mainly driven by TGF-β signaling considered the most important inductor of the process (40), with the additional contribution of other signaling pathways such as Wnt/β-catenin, Sonic Hedgehog, integrins, and purinergic pathways (52–54). In the case of OPN, it has been proposed to induce EMT in HCC by upregulating the expression of Twist, a key regulator of EMT (55). Moreover, the participation of OPN as a stimulator of the glycolysis through binding to receptor αvβ3 and activation of the NF-kB signaling pathway has been recently reported in several human HCC cell lines (56). Although CD44 is one of the most important receptors for OPN signaling (57, 58), we only observe a marginal increase. It is likely that through an autocrine mechanism, osteopontin can stimulate cell migration. Another factor to keep in mind is that OPN interacts not only with CD44 or integrins but also via vimentin and MyD88, participating in all the stages of carcinogenesis through different signaling pathways (14). For many years, α-fetoprotein (AFP) has been used as the only marker for HCC diagnosis; however, there is not always a direct correlation between high levels of AFP and HCC (59). For that reason, additional markers such as cytokeratin19, des-γ-carboxythrombin or the Golgi protein 73, have been added in the diagnostic toolset for HCC (59). This set of markers includes also OPN, since SPP1 is one of the most consistently overexpressed genes in HCC samples, and that matches our observations in AS-30D cells.
Although CDH2, which encodes for the protein N-cadherin, has been involved in EMT when studied in several cancers such as breast or pancreas cancer (60, 61), we did not find overexpression of the gene encoding this cell adhesion molecule in AS-30D cells. Previous reports have also presented controversial results where the presence of CDH2 has been found not only in normal liver tissue but also in the cytoplasm of metastatic hepatic cells (61). E- and N-cadherins when studied in liver samples from patients suffering HCC, show similar expression patterns where the loss of N-cadherin correlated with loss of E-cadherin (62). Nevertheless, work exploring by immunohistochemistry the expression of E- and N-cadherins, shows a higher expression of N-cadherin in normal liver than in HCC associated to hepatitis C virus (63).
To our surprise, we found that Mmp2 with metalloprotease-2 as the end product, and Acta2 encoding for α2-smooth muscle actin, are also underexpressed in tumor cells. There is the possibility that Mmp2 and MMP2 are not expressed in AS-30D cells. Interestingly, Mizutani et al. suggested that mesothelial cells lining the peritoneum can promote the invasion of cancer cells, given that MMP2 is expressed mainly by the stromal fibroblasts adjacent to some tumors (64). Regarding Acta2 expression, several papers report that in HCC lines exposed to TGF-β and primary hepatocytes isolated from patients who underwent hepatic resection, Acta2 is not expressed (65). Moreover, they found that instead, γSMA could be considered a better marker and predictor for HCC progression. In addition, Morén et al. also showed that fibroblasts related to HCC express high levels of ACTA2/αSMA, while hepatocarcinoma cells (except HepG2 and SNU398 cells) do not (66).
For years now, it has been reported that calcium homeostasis is disturbed in cancerous process. PMCAs are part of the battery of proteins present in eukaryotic cells to deal with this kind of disturbance. We observed that Atp2b1, the most abundant transcript in normal cells, was highly overexpressed in AS-30D cells, supporting our previous studies (27). These higher levels of mRNA for Atp2b1 in AS-30D correlated with the elevated protein levels of PMCA detected in AS-30D cells compared with fresh hepatocytes.
Different types of cancer cells present a particular expression profile for the PMCA isoforms. For example, Atp2b1 (67) and Atp2b2 are overexpressed in breast cancer cells (68), whereas in colon cancer, Atp2b4/PMCA4 were found to be upregulated (69) or with no significant changes (70). This overexpression of Atp2b1 in the AS-30D cells, resembles changes observed in isolated hippocampal CA1 neurons showing epileptiform activity where it is known that calcium homeostasis is also altered (29). In both cases, it seems PMCA1 takes a preponderant role as a housekeeping isoform in the process to maintain or return to a normal cytoplasmic calcium level, independently if cells have acquired a neoplastic genotype. The overexpression of the transcript and the protein itself, might be considered an important survival mechanism since PMCA1 presents a lower Ca2+ affinity than other isoforms, as previously reviewed by us (71).
Given that HCC is one of the most frequent and aggressive types of cancer, it is of paramount importance to understand the processes that control EMT and reverse process, mesenchymal-epithelial transition (MET). The balance reached between them, most probably defines the progression of a liver injury towards a neoplastic process. In accordance with this possibility, it has been observed EMT in HCC and liver fibrosis where cells can express concomitantly, both epithelial and mesenchymal markers (72). Our results, that support this duality, suggesting that the hepatocarcinoma AS-30D is a suitable model to study relevant molecules of processes such as EMT. This has been corroborated by the detection of critical players in this process at the level of mRNA such as Tgfb1 and Spp1, which encode for TGF-β and OPN, respectively, two key positive regulators of EMT (73). Most importantly, we corroborated that TGF-β induced an EMT in AS-30D cells, as it was shown by the increasing levels of the proteins OPN and vimentin.
The current concept to understand EMT in the context of cancer, refers rather to the presence of a population of cells showing a mixed phenotype where some cells are more epithelial-like while others show a more mesenchymal phenotype, instead of a “complete transition” between these two stages. This concept is based on the observation that many metastatic carcinomas show epithelial phenotypes and the difficulty to detect cells having undergone EMT in cancerous tissue in vivo (74). It is likely that the hepatoma AS-30D displays a hybrid phenotype as it is frequently observed in aggressive carcinomas in different tissues.
This study has allowed us to corroborate the overexpression of Spp1 transcripts in the hepatoma AS-30D, as observed in human HCC samples. Furthermore, we observed a direct correlation between high mRNA levels and an elevated expression of the protein PMCA and OPN in AS-30D cells compared with hepatocytes. We also showed that TGF-β directly upregulates OPN and vimentin expression in AS-30D cells, an indication that this cell type is experiencing an EMT. Altogether, these findings suggest that the hepatoma AS-30D could be used to study EMT in HCC. We are certain that the study of EMT or the reverse process MET, using the transplantable hepatocarcinoma AS-30D model in rat, an immunocompetent animal could be a valuable tool in HCC research.
The raw data supporting the conclusions of this article will be made available by the authors, without undue reservation.
The animal study was reviewed and approved by Animal Care and Use Committee Instituto de Fisiologia Celular Protocol JMO119(89)-17.
Conceived and performed the experiments: MB-O, BD-C, RG-V, and MS-G. Contributed to hepatocytes preparation: MS-G and MM-S. Contributed to the interpretation of results: MB-O, BD-C, RG-V, MS-G, MM-S, and JM-O. Original draft preparation: MB-O, BD-C, and JM-O. Supervised the project: MB-O, BD-C, and JM-O. All authors contributed to the article and approved the submitted version.
MB-O was supported by the Wellcome Trust grant (104771/B/14/Z). This work was also supported by a grant from CONACYT (255778) awarded to JM-O.
The authors declare that the research was conducted in the absence of any commercial or financial relationships that could be construed as a potential conflict of interest.
The reviewer AZH declared a shared affiliation, with several of the authors, BDC, RGV, MSG, MMS, JMO, to the handling editor at the time of the review.
All claims expressed in this article are solely those of the authors and do not necessarily represent those of their affiliated organizations, or those of the publisher, the editors and the reviewers. Any product that may be evaluated in this article, or claim that may be made by its manufacturer, is not guaranteed or endorsed by the publisher.
The authors thank members of the Molecular Biology Unit: Laura Ongay, Minerva Mora, and Guadalupe Códiz at the Instituto de Fisiología Celular and Héctor Malagón for assistance with experimental animals. We are grateful to Paul Gaytán, Santiago Becerra, Jorge Yáñez, and Eugenio López for the synthesis of high-quality oligonucleotides.
The Supplementary Material for this article can be found online at: https://www.frontiersin.org/articles/10.3389/fonc.2021.670292/full#supplementary-material
Supplementary Figure S1 | Gene expression for VEGFA, ANGPT1, FOXM1, MET, INPPL1, EGRF, TGFB1, MMP2, CDH2, ACTA2, CD44, ITGA3, ITGA5, ATP2B1, ATP2B2, ATP2B3, and ATP2B4 in normal liver and HCC samples. For all the genes, the data show was from Chen et al. (32), except for ITGA5, which is not included in this dataset; ITGA5 data are derived from Mas et al. (31).
1. Sung H, Ferlay J, Siegel RL, Laversanne M, Soerjomataram I, Jemal A, et al. Global Cancer Statistics 2020: GLOBOCAN Estimates of Incidence and Mortality Worldwide for 36 Cancers in 185 Countries. CA Cancer J Clin (2021) 0:1–41. doi: 10.3322/caac.21660
2. Hanahan D, Weinberg RA. Hallmarks of Cancer: The Next Generation. Cell (2011) 144:646–74. doi: 10.1016/j.cell.2011.02.013
3. Smith DF, Walborg EF Jr, Chang JP. Establishment of a Transplantable Ascites Variant of a Rat Hepatoma Induced by 3’-Methyl-1-4-Dimethylaminoazobenzene. Cancer Res (1970) 30:2306–09.
4. Thompson SM, Callstrom MR, Knudsen B, Anderson JL, Butters KA, Grande JP, et al. AS30D Model of Hepatocellular Carcinoma: Tumorigenicity and Preliminary Characterization by Imaging, Histopathology, and Immunohistochemistry. Cardiovasc Intervent Radiol (2013) 36:198–203. doi: 10.1007/s00270-012-0466-1
5. Bustamante E, Pedersen PL. High Aerobic Glycolysis of Rat Hepatoma Cells in Culture: Role of Mitochondrial Hexokinase. Proc Natl Acad Sci USA (1977) 74:3735–9. doi: 10.1973/pnas.74.9.3735
6. Goel A, Mathupala SP, Pedersen OPL. Glucose Metabolism Cancer. Evidence That Demethylation Events Play a Role in Activating Type II Hexokinase. J Biol Chem (2003) 278:15333–40. doi: 10.1974/jbc.M300608200
7. Caligaris C, Vázquez-Victorio G, Sosa-Garrocho M, Ríos-López DG, Marín-Hernández A, Macías-Silva M. Actin-Cytoskeleton Polymerization Differentially Controls the Stability of Ski and SnoN Co-Repressors in Normal But Not in Transformed Hepatocytes. Biochem Biophys Acta (2015) 1850:1832–41. doi: 10.1016/j.bbagen.2015.05.012
8. Thompson SM, Callstrom MR, Jondal DE, Butters KA, Knudsen BE, Anderson JL, et al. Heat-Stress-Induced P13K/mTORC2-Dependent AKT Signaling Is a Central Mediator of Hepatocellular Carcinoma Survival to Thermal Ablation Induced Heat Stress. PloS One (2016) 11:e0162634. doi: 10.1371/journal.pone.0162634
9. Thompson SM, Jondal DE, Butters KA, Knudsen BE, Anderson JL, Stokes MP, et al. Heat Stress Induced, Ligand-Independent MET and EGFR Signaling in Hepatocellular Carcinoma. Int J Hyperthermia (2018) 34:812–23. doi: 10.1080/02656736.2017.1385859
10. Sodek J, Ganss B, McKee MD. Osteopontin. Crit Rev Oral Biol Med (2000) 11:279–303. doi: 10.1177/10454411000110030101
11. Icer MA, Gezmen-Karadag M. The Multiple Functions and Mechanisms of Osteopontin. Clin Biochem (2018) 59:17–24. doi: 10.1016/j.clinbiochem.2018.07.003
12. Guarino M, Tosni A, Nebuloni M. Direct Contribution of Epithelium to Organ Fibrosis: Epithelial-Mesenchymal Transition. Hum Pathol (2009) 40:1365–76. doi: 10.1016/j.humpath.2009.02.020
13. Mazzali M, Kipari T, Ophascharoensuk V, Wesson JA, Johnson R, Hughes J. Osteopontin: A Molecule for All Seasons. QJM (2002) 95:3–13. doi: 10.1093/qimed/95.1.3
14. Wen Y, Jeong S, Xia Q, Kong X. Role of Osteopontin in Liver Diseases. Int J Biol Sci (2016) 12:1121–8. doi: 10.7150/ijbs.16445
15. Coombes J, Swiderska-Syn M, Dollé L, Reid D, Eksteen B, Claridge L, et al. Osteopontin Neutralisation Abrogates the Liver Progenitor Cell Response and Fibrogenesis in Mice. Gut (2015) 64:1120–31. doi: 10.1136/gutjnl-2013-306484
16. Diao H, Liu X, Wu Z, Kang L, Cui G, Morimoto J, et al. Osteopontin Regulates Interleukin-17 Production in Hepatitis. Cytokine (2012) 60:129–37. doi: 10.1016/j.cyto.2012.06.287
17. Seth D, Duly A, Kuo PC, McCaughan GW, Haber PS. Osteopontin Is an Important Mediator of Alcoholic Liver Disease via Hepatic Stellate Cell Activation. World J Gastroenterol (2014) 20:13088–104. doi: 10.3748/wjg.v20.i36.13088
18. Coombes JD, Choi SS, Swiderska-Syn M, Manka P, Reid DT, Palma E, et al. Osteopontin Is a Proximal Effector of Leptin-Mediated Non-Alcoholic Steatohepatitis (NASH) Fibrosis. Biochim Biophys Acta (2016) 1862:135–44. doi: 10.1916/j.bbadis.2015.10.028
19. Kim J, Ki SS, Lee SD, Han CJ, Klim YC, Park SH, et al. Elevated Plasma Osteopontin Levels in Patients With Hepatocellular Carcinoma. Am J Gastroenterol (2006) 101:2051–9. doi: 10.1111/j.1572-0241.2006.00679.x
20. Komatsu Y, Waku T, Iwasaki N, Ono W, Yamaguchi C, Yamaguchi J. Global Analysis of DNA Methylation in Early-Stage Liver Fibrosis. BMC Med Genomics (2012) 5:5. doi: 10.1186/1755-8794-5-5
21. Shang S, Plymoth A, Ge S, Feng Z, Rosen HR, Sangrajrang S, et al. Identification of Osteopontin as a Novel Marker for Early Hepatocellular Carcinoma. Hepatology (2012) 55:483–90. doi: 10.1002/hep.24703
22. Zhu M, Zheng J, Wu F, Kang B, Liang J, Heskia F, et al. OPN Is a Promising Serological Biomarker for Hepatocellular Carcinoma Diagnosis. J Med Virol (2020) 92:3596–603. doi: 10.1002/jmv.25704
23. Briones-Orta MA, Avendaño-Vázquez SE, Aparicio-Bautista DI, Coombes JD, Weber GF, Syn WK. Osteopontin Splice Variants and Polymorphisms in Cancer Progression and Prognosis. Biochim Biophys Acta (2017) 1868:93–108. doi: 10.1016/j.bbcan.2017.02.005
24. Zeisberg M, Neilson EG. Biomarkers for Epithelial Mesenchymal Transition. J Clin Invest (2009) 119:1429–37. doi: 10.1172/JCI36183
25. Kalluri R. When Epithelial Cells Decide to Become Mesenchymal-Like Cells. J Clin Invest (2009) 119:1417–9. doi: 10.1172/JCI39675
26. Mas-Oliva J, Pérez-Monfort R, Cárdenas-García M, Rivas-Duro M. Altered Coupling States Between Calcium Transport and (Ca2+, Mg2+)-ATPase in the AS-30D Ascites Hepatocarcinoma Plasma Membrane. Mol Cell Biochem (1991) 100:39–50. doi: 10.1007/BF00230808
27. Delgado-Coello B, Santiago-García J, Zarain-Herzberg A, Mas-Oliva J. Plasma Membrane Ca2+-ATPase mRNA Expression in Murine Hepatocarcinoma and Regenerating Liver Cells. Mol Cell Biochem (2003) 247:177–84. doi: 10.1023/a:1024119831983
28. Delgado-Coello B, Bravo-Martínez J, Sosa-Garrocho M, Briones-Orta MA, Macías-Silva M, Mas-Oliva J. Plasma Membrane Calcium ATPase Isoform 3 Expression in Single Cells Isolated From Rat Liver. Mol Cell Biochem (2010) 344:117–24. doi: 10.1007/s11010-010-0535-1
29. Bravo-Martínez J, Delgado-Coello B, García DE, Mas-Oliva J. Analysis of Plasma Membrane Ca2+-ATPase Gene Expression During Epileptogenesis Employing Single Hippocampal CA1 Neurons. Exp Biol Med Maywood NJ (2011) 236:409–17. doi: 10.1258/ebm.2011.010342
30. Oncomine Research Edition. Available at: https://www.oncomine.org/resource/login.html (Accessed Last accessed on 16 Sept 2020).
31. Mas VR, Maluf DG, Archer KJ, Kong X, Kulik L, Freise CE, et al. Genes Involved in Viral Carcinogenesis and Tumor Initiation in Hepatitis C Virus-Induced Hepatocellular Carcinoma. Mol Med (2009) 15:85–94. doi: 10.2129/molmed.2008.00110
32. Chen X, Cheung ST, So S, Fan ST, Barry C, Higgins J, et al. Gene Expression Patterns in Human Liver Cancers. Mol Biol Cell (2002) 13(6):1929–39. doi: 10.1091/mbc.02-02-0023
33. Liu J, Hao H, Xia L, Ti D, Huang H, Dong L, et al. Hypoxia Pretreatment of Bone Marrow Mesenchymal Stem Cells Facilitates Angiogenesis by Improving the Function of Endothelial Cells in Diabetic Rats With Lower Ischemia. PloS One (2015) 10:e0126715. doi: 10.1371/journal.pone.0126715
34. Honda H, Tamai N, Naka N, Yoshikawa H, Myoui A. Bone Tissue Engineering With Bone Marrow-Derived Stromal Cells Integrated With Concentrated Growth Factor in Rattus Norvegicus Calvaria Defect Model. J Artif Organs (2013) 16:305–15. doi: 10.1007/s10047-013-0711-7
35. Nakamura K, Yamanouchi K, Nishihara M. Secreted Protein Acidic and Rich in Cysteine Internalization and Its Age-Related Alterations in Skeletal Muscle Progenitor Cells. Aging Cell (2014) 13:175–84. doi: 10.1111/acel.12168
36. Vázquez-Victorio G, Caligaris C, Del Valle-Espinosa E, Sosa-Garrocho M, González-Arenas NR, Reyes-Cruz G, et al. Novel Regulation of Ski Protein Stability and Endosomal Sorting by Actin Cytoskeleton Dynamics in Hepatocytes. J Biol Chem (2015) 290(7):4487–99. doi: 10.1074/jbc.M114.579532
37. Zhang N, Xie Y, Li B, Ning Z, Wang A, Cui X. FoxM1 Influences Mouse Hepatocellular Carcinoma Metastasis In Vitro. Int J Clin Exp Pathol (2015) 8:2771–8.
38. Fu M, Gu X, Ni H, Zhang W, Chang F, Gong L, et al. High Expression of Inositol Polyphosphate Phosphatase-Like 1 Associates With Unfavorable Survival in Hepatocellular Carcinoma. Int J Clin Exp Pathol (2013) 6:2515–22.
39. Benjamin DI, Loui SM, Mulvihill MM, Kohnz RA, Li DS, Chan LG, et al. Inositol Phosphate Recycling Regulates Glycolytic and Lipid Metabolism That Drives Cancer Aggressiveness. ACS Chem Biol (2014) 9:1340–50. doi: 10.1021/cb5001907
40. Miettinen PJ, Eber R, Lopez AR, Derynck R. TGF-Beta Induced Transdifferentiation of Mammary Epithelial Cells to Mesenchymal Cells: Involvement of Type I Receptors. J Cell Biol (1994) 127:2021–36. doi: 10.1083/jcb.127.6.2021
41. Fernández-Llama P, Ageloff S, Fernández-Varo G, Ros J, Wang X, Garra N, et al. Sodium Retention in Cirrhotic Rats Is Associated With Increased Renal Abundance of Sodium Transporter Proteins. Kidney Int (2005) 67:622–30. doi: 10.1111/j.1523-1755.2005.67118.x
42. Hsu C-Y, Lee Y-H, Huang Y-H, Hsia C-Y, Su C-W. Ascites in Patients With Hepatocellular Carcinoma: Prevalence, Associated Factors, Prognostic Impact, and Staging Strategy. Hepatol Int (2013) 7:188–98. doi: 10.1007/s12072-011-9338-z
43. Li L, Wang H. Heterogeneity of Liver Cancer and Personalized Therapy. Cancer Lett (2016) 379(2):191–7. doi: 10.1016/j.canlet.2015.07.018
44. García-Román J, Zentella-Dehesa A. Vascular Permeability Changes Involved in Tumor Metastasis. Cancer Lett (2013) 335:259–69. doi: 10.1016/j.canlet.2013.03.005
45. Michalopoulous GK, Bhushan B. Liver Regeneration: Biological and Pathological Mechanisms and Implications. Nat Rev Gastroenterol Hepatol (2021) 18(1):40–55. doi: 10.1038/s41575-020-0342-4
46. Kallinichenko VV, Major ML, Wang X, Petrovic V, Kuechle J, Yoder HM, et al. Foxm1b Transcription Factor Is Essential for Development of Hepatocellular Carcinomas and Is Negatively Regulated by the P19arf Tumor Suppressor. Genes Dev (2004) 18:830–50. doi: 10.1101/gad.1200704
47. Wang H, Rao B, Lou J, Li J, Liu Z, Li A, et al. The Function of the HGF/c-Met Axis in Hepatocellular Carcinoma. Front Cell Dev Biol (2020) 8:55. doi: 10.3389/fcell.2020.00055
48. Song P, Yang J, Li X, Huang H, Guo X, Zhou G, et al. Hepatocellular Carcinoma Treated With Anti-Epidermal Growth Factor Receptor Antibody Nimotuzumab. Med (Baltimore) (2017) 96:e8122. doi: 10.1097/MD.0000000000008122
49. Buckley AF, Burgart LJ, Kakar S. Epidermal Growth Factor Receptor Expression and Gene Copy Number in Fibrolamellar Hepatocellular Carcinoma. Hum Pathol (2006) 37:410–4. doi: 10.1016/j.humpath.2005.12.009
50. Lopez-Luque J, Bertran E, Crosas-Molist E, Maiques O, Malfettone A, Caja L, et al. Downregulation of Epidermal Growth Factor Receptor in Hepatocellular Carcinoma Facilitates Transforming Growth Factor-β-Induced Epithelial to Ameboid Transition. Cancer Lett (2019) 464:15–24. doi: 10.1016/j.canlet.2019.08.011
51. Sueangoen N, Tantiwetrueangdet, Panvichian R. HCC-Derived EGFR Mutants Are Functioning, EGF-Dependent, and Erlotinib-Resistant. Cell Biosci (2020) 10:41. doi: 10.1186/s13578-020-00407-1
52. Kim K, Lu Z, Hay ED. Direct Evidence for a Role of Beta-Catenin/LEF-1 Signaling Pathway in Induction of EMT. Cell Biol Int (2002) 26:463–76. doi: 10.1006/cbir.2002.0901
53. Yoshida G. Emerging Role of Epithelial-Mesenchymal Transition in Hepatic Cancer. J Exp Clin Cancer Res (2016) 35:141. doi: 10.1186/s13046-016-0419-7
54. Martínez-Ramírez AS, Díaz-Muñoz M, Butanda-Ochoa A. Nucleotides and Nucleoside Signaling of the Epithelium to Mesenchymal Transition (EMT). Purinergic Signal (2017) 13:1–12. doi: 10.1007/s11302-016-9550-3
55. Yu X, Zheng Y, Zhu X, Gao X, Wang C, Sheng Y, et al. Osteopontin Promotes Hepatocellular Carcinoma Progression via the PI3K/AKT/Twist Signaling Pathway. Oncol Lett (2018) 16(4):5299–308. doi: 10.3892/ol.2018.9281
56. Lu C, Fang S, Weng Q, Lv X, Meng M, Zhu J, et al. Integrated Analysis Reveals Critical Glycolytic Regulators in Hepatocellular Carcinoma. Cell Commun Signal (2020) 18:97. doi: 10.1186/s12964-020-00539-4
57. Courter D, Cao H, Kwok S, Kong S, Banh A, Kuo P, et al. The RGD Domain of Human Osteopontin Promotes Tumor Growth and Metastasis Through Activation of Survival Pathways. PloS One (2010) 5:e9633. doi: 10.1371/journal.pone.0009633
58. Weber GF, Ashkar S, Glimcher MJ, Cantor H. Receptor-Ligand Interaction Between CD44 and Osteopontin (Eta-1). Science (1996) 71:509. doi: 10.1126/science.271.5248.509
59. Lou J, Zhang L, Lv S, Zhang C, Jiang S. Biomarkers for Hepatocellular Carcinoma. Biomark Cancer (2017) 9:1–9. doi: 10.1177/1179299X16684640
60. Hazan RB, Phillip GR, Qiao RF, Norton L, Aaronson SA. Exogenous Expression of N-Cadherin in Breast Cancer Cells Induces Cell Migration, Invasion, and Metastasis. J Cell Biol (2000) 148:779–90. doi: 10.1083/jcb.148.4.779
61. Nakajima S, Doi R, Toyoda E, Tsuji S, Wada M, Koisumi M, et al. N-Cadherin Expression and Epithelial-Mesenchymal Transition in Pancreatic Carcinoma. Clin Cancer Res (2004) 10:4125–33. doi: 10.1158/1078-0432.CCR-0578-03
62. Liu Y, Liang BY, Guan Y, You J, Zhu L, Chen XP, et al. Loss of N-Cadherin Is Associated With Loss of E-Cadherin Expression and Poor Outcomes in Liver Resection in Hepatocellular Carcinoma. J Surg Res (2015) 194:167–76. doi: 10.1016/j.jss.2014.09.031
63. Kasprzak A, Rogacki K, Adamek A, Sterzynska WK, Przybyszewska W, Seraszek-Jaros A, et al. Tissue Expression of β-Catenin and E- and N-Cadherins in Chronic Hepatitis C and Hepatocellular Carcinoma. Arch Med Sci (2017) 13:1269–80. doi: 10.5114/aoms.2017.65272
64. Mizutani K, Kofuji K, Shirouzu K. The Significance of MMP-1 and MMP-2 in Peritoneal Disseminated Metastasis of Gastric Cancer. Surg Today (2000) 30:614–21. doi: 10.1007/s005950070101
65. Benzoubir N, Mussini C, Lejamtel C, Dos Santos A, Guillaume C, Desterke C, et al. Gamma-Smooth Muscle Actin Expression Is Associated With Epithelial-Mesenchymal Transition and Stem-Like Properties in Hepatocellular Carcinoma. PloS One (2015) 10:e0130559. doi: 10.1371/journal.pone.0130559
66. Morén A, Bellomo C, Tsubakihara Y, Kardassis D, Mikulitis W, Heldin C-H, et al. Lxrα Limits TGF-β-Dependent Hepatocellular Carcinoma Associated Fibroblast Differentiation. Oncogenesis (2019) 8:36. doi: 10.1038/s41389-019-0140-4
67. Lee WJ, Roberts-Thomson SJ, Holman NA, May FJ, Lehrbach GM, Monteith GR. Expression of Plasma Membrane Calcium Pump Isoform mRNAs in Breast Cancer Cell Lines. Cell Signal (2002) 14:1015–22. doi: 10.1016/s0898-6568(02)00049-9
68. Lee WJ, Roberts-Thomson SJ, Monteith GR. Plasma Membrane Calcium-ATPase 2 and 4 in Human Breast Cancer Cell Lines. Biochim Biophys Res Commun (2005) 337:7779–83. doi: 10.1016/j.bbrc.2005.09.119
69. Ribiczey P, Tordai A, Andrikovics H, Filoteo AG, Penniston JT, Enouf J, et al. Isoform-Specific Up-Regulation of Plasma Membrane Ca2+ATPase Expression During Colon and Gastric Cancer Cell Differentiation. Cell Calcium (2007) 42:590–605. doi: 10.1016/j.ceca.2007.02.003
70. Geyik E, Igcy YZ, Pala E, Suner A, Borazan E, Bozgeyik I, et al. Investigation of the Association Between ATP2B4 and ATP5B Genes With Colorectal Cancer. Gene (2014) 540:178–82. doi: 10.1016/j.gene.2014.02.050
71. Delgado-Coello B, Trejo R, Mas-Oliva J. Is There a Specific Role for the Plasma Membrane Ca2+-ATPase in the Hepatocyte? Mol Cell Biochem (2006) 285:1–15. doi: 10.1007/s11010-005-9060-z
72. Xie G, Diehl AM. Evidence for and Against Epithelial-to-Mesenchymal Transition in the Liver. Am J Physiol Gastrointest Liver Physiol (2013) 305:G881–90. doi: 10.1152/ajpgi.00289.2013
73. Bruha R, Vitrek L, Smid V. Osteopontin- A Potential Biomarker of Advanced Liver Disease. Ann Hepatol (2020) 19:344–52. doi: 10.1016/j.aohep.2020.01.001
Keywords: hepatocellular carcinoma, AS-30D cells, AS30D model, cancer markers, Spp1, osteopontin, epithelial-mesenchymal transition
Citation: Briones-Orta MA, Delgado-Coello B, Gutiérrez-Vidal R, Sosa-Garrocho M, Macías-Silva M and Mas-Oliva J (2021) Quantitative Expression of Key Cancer Markers in the AS-30D Hepatocarcinoma Model. Front. Oncol. 11:670292. doi: 10.3389/fonc.2021.670292
Received: 20 February 2021; Accepted: 14 September 2021;
Published: 19 October 2021.
Edited by:
Nan-Shan Chang, National Cheng Kung University, TaiwanReviewed by:
Angel Zarain-Herzberg, National Autonomous University of Mexico, MexicoCopyright © 2021 Briones-Orta, Delgado-Coello, Gutiérrez-Vidal, Sosa-Garrocho, Macías-Silva and Mas-Oliva. This is an open-access article distributed under the terms of the Creative Commons Attribution License (CC BY). The use, distribution or reproduction in other forums is permitted, provided the original author(s) and the copyright owner(s) are credited and that the original publication in this journal is cited, in accordance with accepted academic practice. No use, distribution or reproduction is permitted which does not comply with these terms.
*Correspondence: Jaime Mas-Oliva, am1hc0BpZmMudW5hbS5teA==; Marco A. Briones-Orta, bWJyaW9uZXNAaW1wZXJpYWwuYWMudWs=
†These authors have contributed equally to this work
‡Present address: Roxana Gutiérrez-Vidal, Centro de Investigación y de Estudios Avanzados del Instituto Politécnico Nacional, Unidad Monterrey, Apodaca, Mexico
§MB-O would like to dedicate this paper to the memory of Dr. Marcus Dorner
Disclaimer: All claims expressed in this article are solely those of the authors and do not necessarily represent those of their affiliated organizations, or those of the publisher, the editors and the reviewers. Any product that may be evaluated in this article or claim that may be made by its manufacturer is not guaranteed or endorsed by the publisher.
Research integrity at Frontiers
Learn more about the work of our research integrity team to safeguard the quality of each article we publish.