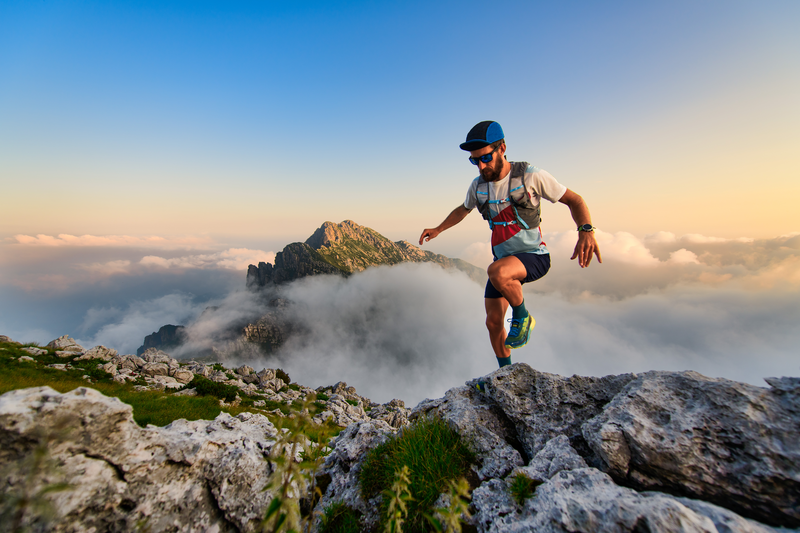
94% of researchers rate our articles as excellent or good
Learn more about the work of our research integrity team to safeguard the quality of each article we publish.
Find out more
SYSTEMATIC REVIEW article
Front. Oncol. , 28 April 2021
Sec. Cancer Immunity and Immunotherapy
Volume 11 - 2021 | https://doi.org/10.3389/fonc.2021.651944
Background: This study aimed to systematically evaluate and compare the efficacy and safety of consolidative hematopoietic stem cell transplantation (HSCT) after CD19 chimeric antigen receptor T (CAR-T) therapy with non-HSCT in the treatment of acute lymphoblastic leukemia (ALL).
Methods: The PubMed, Embase, Cochrane Library and Web of Science databases were searched for clinical trials. Pooled hazard ratios (HRs) for overall survival (OS), relapse rate, and leukemia-free survival (LFS) as well as overall incidence rates for transplant-related mortality (TRM), acute graft-versus-host disease (aGVHD), chronic graft-versus-host disease (cGVHD), and infections were calculated using Stata software.
Results: We screened 3,441 studies and identified 19 eligible studies with 690 patients. Among the patients who achieved complete remission (CR) after CD19 CAR-T therapy, consolidative HSCT was beneficial for OS (HR = 0.34, 95% CI, 0.17–0.68, P = 0.003), the relapse rate (HR = 0.16, 95% CI, 0.10–0.25, P < 0.001), and LFS (HR = 0.15, 95% CI, 0.08–0.28, P < 0.001). For patients who achieved MRD-negative (neg) CR after CD19 CAR-T therapy, consolidative HSCT was beneficial for OS (0.57, 95% CI, 0.33–0.99, P = 0.045), the relapse rate (0.14, 95% CI, 0.06–0.31, P < 0.001), and LFS (0.21, 95% CI, 0.12–0.35, P < 0.001). Regarding safety, we calculated pooled incidence rates for TRM (8%, 95% CI, 0.02–0.15), aGVHD (44%, 95% CI, 0.23–0.67), cGVHD (36%, 95% CI, 0.17–0.56), and infections (39%, 95% CI, 0.03–0.83).
Conclusions: Compared with non-HSCT treatment, consolidative HSCT after CD19 CAR-T therapy for R/R B-ALL patients can prolong OS and LFS and reduce the risk of relapse. The incidence rates for adverse events are acceptable. More high-quality randomized controlled trials are required to avoid bias and further determine the efficacy of HSCT.
B-cell acute lymphoblastic leukemia (B-ALL) is one of the most common hematological malignancies in children and young adults. Patients with refractory or relapsed (R/R) B-ALL are usually resistant to traditional therapy and have a poor prognosis. Novel therapeutic strategies to improve prognosis have been widely studied, and chimeric antigen receptor (CAR)-T cell therapy is a promising and potent approach. T cells with engineered CAR molecules recognize antigens on tumor cells via single-chain variable fragments (scFv) without MHC restriction. High complete remission (CR) rates were reported in different clinical trials of CD19 CAR-T therapy; in 2017, the first CD19 CAR-T product—tisagenlecleucel—was approved by the Food and Drug Administration (FDA).
However, relapse is a challenge for CAR-T therapy, with rates ranging from 20 to 70% during sufficiently long follow-up periods (1). A combinatorial treatment modality might be needed to enhance the antitumor effect. Allogeneic hematopoietic stem cell transplantation (allo-HSCT), the only potentially curative therapy for R/R B-ALL (2, 3), is considered a promising option. CAR-T cells may be used as a bridge to HSCT because they can induce a high CR rate. HSCT rebuilds the patient’s hematopoietic and immune system. Genetic differences between patients and donors will induce graft versus leukemia (GVL) effects, thus maintaining the patient’s long-term remission and reducing the relapse rate. However, whether to administer HSCT after CAR-T therapy remains controversial. Because CAR-T cells can survive and maintain their therapeutic effect in vivo for a long time, patients may have similar outcomes regardless of whether they undergo subsequent consolidative HSCT. Furthermore, transplant-associated complications, particularly lethal complications, may severely affect the patient prognosis. Thus, whether bridging HSCT benefits ALL patients remains unclear.
Recently, an increasing number of clinical trials have compared the outcomes of patients with and without transplantation after CD19 CAR-T therapy. This study aimed to evaluate the efficacy and safety of HSCT after CAR-T therapy to treat R/R B-ALL and provide more objective data for optimal clinical practices.
This study was conducted in accordance with the Preferred Reporting Items for Systematic Reviews and Meta-analyses (PRISMA) reporting guidelines (4) and was registered in PROSPERO on July 5, 2020. The registration number is CRD42020182281.
We searched the PubMed, Embase, Cochrane Library and Web of Science databases using the key terms “receptor, chimeric antigen,” “hematologic malignancy,” and “hematopoietic stem cell transplantation.” All relevant studies published from 01 January 2011 to 03 August 2020 were searched without restriction on country or article type. The reference lists of all the selected articles were independently screened to identify additional studies.
The inclusion criteria were as follows: (1) clinical trials; (2) patients with a diagnosis of relapsed/refractory B-lineage ALL without other hematological malignancies; (3) patients receiving CD19 CAR-T therapy followed by consolidative HSCT; (4) reported necessary information, such as clear grouping of patients and follow-up data; (5) studies conducted from 2011 to the present. Unpublished gray literature, commentaries, letters, reviews, and editorials were excluded. Studies with the same clinical trial number were excluded or analyzed for different outcomes.
The data were independently extracted by two reviewers (SC and ZZ) using a standardized collection form. XXu confirmed the data and adjudicated any remaining discrepancies. The hazard ratios (HRs) of six trials (5–10) selected for the efficacy analysis were not reported; thus, they were extracted based on data in waterfall plots using WebPlotDigitizer (version 4.2). The relapse rate and survival status were converted into HR by SPSS (version 25). The data from one trial (11) presented in the Kaplan-Meier curve were extracted using the automatic point-finding method of the Engauge Digitizer (version 11.1) and Photoshop (version 2020), and then the data were entered into an HR calculation spreadsheet template developed by Sydes and Tierney (12). The estimated HR and 95% confidence interval (CI), under the assumption of uniform within-interval censoring, were used in the analysis. Data concerning transplant-related adverse events were extracted in the text, and the total number of target events in the HSCT group were collected.
All statistical analyses were conducted using Stata version 16.0 and Stata version 14.0 (StataCorp, College Station, TX, USA). The pooled HR was used to describe the outcome indicators of OS, relapse and LFS. The command “metaprop” (13) was used in Stata to analyze the rates of transplant-related adverse events. The 95% CI was selected, and P < 0.05 indicated statistically significant results. We used Cochran’s Q test and I2 statistic to assess heterogeneity between studies. In the former, P < 0.1 indicated significant heterogeneity. If I2 < 50%, the fixed-effects model was selected; if I2 ≥ 50%, the random-effects model was chosen due to substantial heterogeneity. Sensitivity analysis was performed to evaluate the stability of the results.
The Newcastle-Ottawa scale was selected to evaluate cohort study quality. The modified Institute of Health Economics (IHE) tool was adopted to evaluate the study quality of single-arm studies (14). We assessed publication bias using funnel plots, Begg’s test, and Egger’s test and defined significant publication bias as a P value <0.05.
The flow diagram of the study selection process is shown in Figure 1. In total, 3,441 articles were retrieved from four databases and other sources (668 from PubMed, 1,375 from Web of Science, 1,134 from Embase, 263 from Cochrane Library, 1 from other sources), of which 19 (1, 5–11, 15–25) were included for quantitative analysis. The 19 studies were from 17 different clinical trials, and studies from the same trials were analyzed for different outcomes. Of the 19 studies with 690 patients, 11 reported data related to efficacy outcomes, and 14 reported data concerning safety outcomes. Eleven (1, 5–11, 16, 19, 24) studies were cohort studies, and 8 (15, 17, 18, 20–23, 25) were single-arm studies.
All 11 studies enrolled for efficacy analysis were cohort studies. The basic information of the 11 enrolled studies is shown in Table 1; 586 R/R ALL patients received CD19 CAR-T infusion. Additionally, 521 patients achieved CR/CRi, and 456 achieved MRD-negative (neg) CR. Regarding the costimulatory domain of CAR-T cells, three studies used CD28, seven used 4-1BB, and one adopted both CD28 and 4-1BB. A total of 266 patients were bridged to HSCT after achieving CR, and 218 were bridged to HSCT after achieving MRD-neg CR. The median time between CAR-T infusion and HSCT was within 3 months for the eight studies that reported the median interval time. Ten of the 11 studies performed allogeneic transplantation, and one (16) performed allogeneic or autologous transplantation.
All 14 studies enrolled for safety analysis were non-randomized, and their basic information is listed in Table 2. A total of 328 patients were bridged to HSCT in the 14 studies. Of the 10 studies reporting the median time between CAR-T therapy and HSCT, the interval time was within 3 months. All 14 studies reported TRM with an incidence rate ranging from 0 to 50%. The specific causes of TRM were reported in five studies and included GVHD, infections, veno-occlusive disease (VOD), and lung diseases. The most common causes were GVHD (n = 6) and infections (n = 5). Adverse events related to HSCT included aGVHD, cGVHD, infections, and VOD, etc. Five studies mentioned aGVHD, with an incidence rate ranging from 23.3 to 73.7%; of which the incidence rate of severe aGVHD (grade III/IV) ranged from 0 to 21.1%. Six studies mentioned cGVHD with an incidence rate from 9.5 to 88.9%. In total, five studies reported pathogens of infections, including bacteria, fungi, and viruses. Among them, 36 cases of cytomegalovirus (CMV) infection and 32 cases of Epstein–Barr virus (EBV) infection were reported.
Eight studies reported data related to OS, in which patients achieved CR before receiving consolidative HSCT post-CAR-T therapy (Figure 2A). The pooled HR was 0.34 (95% CI, 0.17–0.68, P = 0.003), indicating a significantly better OS for patients who received consolidative HSCT. Based on the moderate heterogeneity (I2 = 65.71%, P = 0.005), the DerSimonian-Laird random-effects model was selected.
Figure 2 Forest plots of efficacy outcomes comparing consolidative HSCT and non-HSCT using pooled hazard ratios (HRs). (A) Overall survival (OS) analysis for patients achieving CR after CAR-T therapy; (B) OS analysis for patients achieving MRD-neg CR after CAR-T therapy; (C) Relapse rate analysis for patients achieving CR after CAR-T therapy; (D) Relapse rate analysis for patients achieving MRD-neg CR after CAR-T therapy; (E) Leukemia-free survival (LFS) analysis for patients achieving CR after CAR-T therapy; (F) LFS analysis for patients achieving MRD-neg CR after CAR-T therapy.
Five studies were enrolled for analysis when the inclusion criteria of the HSCT group only included patients achieving MRD-neg CR before consolidative HSCT therapy (Figure 2B). The pooled HR was 0.57 (95% CI, 0.33–0.99, P = 0.045), and there was low heterogeneity (I2 = 8.87%, P = 0.356). The pooled HR indicated a significantly better OS for patients who received HSCT after achieving MRD-neg CR.
Six studies reported sufficient data regarding relapse in the two groups, in which patients received HSCT after achieving CR (Figure 2C). The pooled HR was 0.16 (95% CI, 0.10–0.25, P < 0.001) (I2 = 0.00%, P = 0.950). Thus, the patients who were bridged into HSCT had a significantly decreased likelihood of relapse.
Four studies were analyzed when the inclusion criteria of the HSCT group only included patients achieving MRD-neg CR before consolidative HSCT therapy (Figure 2D). The pooled HR was 0.14 (95% CI, 0.06–0.31, P < 0.001) (I2 = 0.00%, P = 0.535). The pooled HR indicated a significantly lower risk of relapse in the HSCT group after MRD-neg CR was achieved.
Eight studies reported data related to LFS, in which patients achieved CR before receiving consolidative HSCT (Figure 2E). The pooled HR was 0.15 (95% CI, 0.08–0.28, P < 0.001). Based on the moderate heterogeneity (I2 = 55.66%, P = 0.027), the DerSimonian-Laird random-effects model was chosen. The pooled HR showed that the LFS results of the HSCT group were significantly better than those of the non-HSCT group.
Five studies were enrolled when the inclusion criteria of the HSCT group only included patients who achieved MRD-neg CR before consolidative HSCT therapy (Figure 2F). The pooled HR was 0.21 (95% CI, 0.12–0.35, P < 0.001), and there was low heterogeneity (I2 = 0.00%, P = 0.438). The pooled HR indicated significantly better LFS in the HSCT group after achieving MRD-neg CR.
OS was analyzed in six studies using the 4-1BB costimulatory domain of CAR-T cells (Figure S1A). The pooled HR for the 4-1BB cases was 0.32 (95% CI, 0.21–0.49, P < 0.001). The relapse rate was analyzed by five studies based on the 4-1BB costimulatory domain of CAR-T cells (Figure S1B). The pooled HR for 4-1BB cases was 0.16 (95% CI, 0.10–0.26, P < 0.001). LFS was analyzed by five studies based on the 4-1BB costimulatory domain of CAR-T cells (Figure S1C). The results revealed a pooled HR of 0.24 (95% CI, 0.16-0.35, P < 0.001) for 4-1BB cases.
The results confirmed the significant efficacy of consolidative HSCT in 4-1BB cases, such that the treatment prolonged OS, reduced the relapse rate, and increased LFS, while the efficacy of this treatment in CD28 cases remained unclear because of the scarcity of studies. Thus, we could not perform subgroup analysis of 4-1BB and CD28.
Fourteen studies reported data concerning transplant-related mortality (Figure 3A). The pooled HR of the incidence rate of TRM was 0.08 (95% CI, 0.02–0.15). The DerSimonian-Laird random-effects model was selected based on the significant heterogeneity (I2 = 62.87%, P < 0.01).
Figure 3 Forest plots of safety outcomes of consolidative HSCT using pooled incidence rates. (A) Pooled transplant-related mortality rates; (B) Pooled acute graft-versus-host disease rates; (C) Pooled chronic graft-versus-host disease rates; (D) Pooled infection rates.
Five studies reported data concerning acute GVHD (Figure 3B). The pooled incidence rate of acute GVHD was 0.44 (95% CI, 0.23–0.67). Based on the significant heterogeneity (I2 = 81.40%, P < 0.01), the DerSimonian-Laird random-effects model was selected.
Six studies assessed chronic GVHD and were enrolled in this analysis (Figure 3C). The pooled incidence rate of chronic GVHD was 0.36 (95% CI, 0.17–0.56). The DerSimonian-Laird random-effects model was selected based on the significant heterogeneity (I2 = 82.47%, P < 0.01).
Only three studies reported data related to infections after bridging to HSCT (Figure 3D). The pooled incidence rate of infections was 0.39 (95% CI, 0.03–0.83). Because of the significant heterogeneity (I2 = 89.74%, P < 0.01), the DerSimonian-Laird random-effects model was selected.
The 11 cohort studies were evaluated using the Newcastle-Ottawa Scale (Table S1) and were all categorized as low risk. The eight single-arm studies were evaluated using the modified Institute of Health Economics (IHE) risk of bias tool and were also categorized as low risk (Figure S2).
We performed publication bias analyses using funnel plots, Egger’s test, and Begg’s test. The scattered points of the funnel plots were symmetrically distributed. The P values of Egger’s and Begg’s tests for all outcomes were >0.05, suggesting no significant publication bias. Begg’s plots are shown in Figure S3.
Using different models did not affect the efficacy outcomes but did affect the safety outcomes. No small-sample effect was observed for the efficacy analysis, and a potential small-sample effect was observed for the safety analysis.
To evaluate the stability of the analysis, the studies were excluded one by one according to the order of Newcastle-Ottawa Scale scores and IHE assessment outcomes. Sensitivity analysis proved the analysis of relapse stable. However, confounding factors caused instability in other outcome indicators. Two studies (11, 24) showed heterogeneity in OS, one study showed heterogeneity in LFS (24), two studies showed heterogeneity in TRM (1, 11), one study showed heterogeneity in aGVHD (6), and two studies showed heterogeneity in cGVHD (8, 15). Regarding infections, excluding any study would alter the result because only three studies were included in the analysis.
To our knowledge, this is the first study to systematically analyze the efficacy and safety of consolidative HSCT after CD19 CAR-T therapy for patients with R/R B-ALL. Our findings suggest that bridging to HSCT after CAR-T therapy is an efficient and relatively safe method that prolongs OS and LFS and reduces the relapse risk without obviously increasing the risks of adverse events.
Achieving molecular remission is considered an ideal outcome for ALL patients and is superior to hematologic remission. Our study indicates that patients who received consolidative HSCT after achieving CR or MRD-neg CR had a longer OS and LFS and a lower risk of relapse. Because no adequate MRD grouping information was available and the sample data of MRD-positive CR patients were too small, it was difficult to compare the results of MRD-neg CR patients and MRD-positive CR patients by subgroup analysis directly. Some studies suggest that, after the administration of CAR-T cells, patients achieving MRD-neg CR might have a longer OS and LFS, while patients achieving MRD-positive CR had a higher chance of relapse (11, 27). Additionally, the state of MRD-neg CR could greatly benefit the outcomes of HSCT (26, 28, 29). In the enrolled studies, Zhao et al. (1) reported a longer OS and LFS of MRD-neg CR patients than those of MRD-positive CR patients, and Gu et al. (6) drew a similar conclusion. Thus, a patient’s state of MRD-neg CR is a strong indicator of a better prognosis after HSCT. However, this finding may not indicate that MRD-positive patients are unsuitable for HSCT. Because the risk of relapse is higher in these patients, they may require consolidative HSCT to improve the prognosis (30). Studies with larger sample sizes are needed to verify the outcomes of MRD-positive patients. Notably, qPCR may be more sensitive than FCM in MRD detection (5); thus, the detection method must be standardized.
Previous studies have shown that CD28 CAR-T cells can lead to robust expansion initially but short persistence and high relapse rates. By contrast, 4-1BB CAR-T cells have a prolonged duration in vivo and antitumor effects. Thus, we analyzed the 4-1BB costimulatory domain and further confirmed the prolonged OS and LFS, as well as lower risks of relapse for the 4-1BB group after HSCT. Because few studies meeting the inclusion criteria adopted CD28 as a costimulatory domain, we were unable to perform subgroup analysis of 4-1BB and CD28. In the future, more randomized studies are needed to determine the effect of the CAR costimulatory domain on the post-HSCT outcomes of patients.
Safety was a focus of this novel therapy. It was previously believed that CAR-T infusion and subsequent conditioning therapy might induce synergetic immune dysregulation and immunosuppression (31, 32), increasing the incidence of transplant-related complications. Severe CRS and long-term pancytopenia may also increase the risk of infection. Our study showed that the overall TRM rate was 8%, the aGVHD rate was 44%, the cGVHD rate was 36%, and the infection rate was 39%. The incidence rates of these complications were tolerable and not higher than those of patients receiving HSCT alone (33–37). In addition, Gu et al. (6) found that the 2-year treatment-related mortality did not differ significantly between the consolidative HSCT and non-HSCT groups [14.3% (95% CI, 7.6–21%) vs. 9.8% (95% CI, 3.2–16.4%); p = 0.804]. Furthermore, the reduction of TRM is a key issue and may benefit from the increasingly developed transplant techniques and maturity of preventive and curative measures for complications of HSCT and CAR-T.
Presently, the application of HSCT in the treatment of hematological malignancies is relatively mature, and there are no considerable technical obstacles at most transplant institutions. As a combinatorial regimen, maximizing the synergistic effects of CAR-T therapy and HSCT is important and is influenced by factors such as the patient status, donor sources, and transplantation timing, etc. The associated data in our review cannot be separated to perform subgroup analysis and draw quantitative conclusions. We will discuss those important factors based on trials enrolled in our study to optimize therapeutic regimens.
Regarding the patient status, age and pretransplantation history can influence choices concerning whether to undergo HSCT. Although we cannot propose a specific age limit, younger patients may benefit more from consolidative HSCT than elderly patients. Zhao et al. (1) proposed that age is an independent prognostic factor and that patients younger than 40 years had a significantly better prognosis (LFS, HR= 4.706, 95% CI, 1.6301–13.586; P = 0.004). Jiang et al. (8) reported an age older than 70 years as an exclusion criterion for HSCT. Regarding the pretransplantation status, receiving two or more transplantations may lead to a high relapse rate and incidence of complications after HSCT (38), and a pretransplant history before CAR-T cell infusion can also dampen the efficacy of a second transplantation after CAR-T cell infusion (26). Many patients with a transplant history have been excluded from many trials included in our study (1, 6, 8, 39). More studies are needed to determine whether patients with a history of transplantation can benefit from consolidative HSCT after CAR-T therapy. Other exclusion criteria included infections or complications, relapse from blinatumomab (40, 41), heavy tumor burden before CAR-T treatment (11, 42) and other contraindications (7, 8).
Regarding donor choices, in addition to HLA-identical donors, unrelated matched donors and haploidentical donors are gradually being accepted. In particular, for haplo-HSCT, almost all patients can obtain stable donor sources in a short time. The efficacy of haplo-HSCT is similar to that of HLA-matched HSCT (35); in our included trials, haplo-HSCT is the most common choice (Table 2). The graft can also be further optimized to improve the safety of transplantation. Using CD34 selected T-cell deletion (TCD) may be a feasible solution. Previously, it was believed that the depletion of CD34-positive T cells could result in slow immune recovery and a high infection rate (43). However, in the study of Fabrizio et al. (17), CD34-selected TCD allo-HSCT significantly reduced TRM without affecting disease control ability. If transplant-related risks are high and allo-HSCT is inappropriate, auto-HSCT may also be a strategy (16).
The timing of transplantation should also be considered because the optimal timing of HSCT after CAR-T infusion may greatly influence the outcome and safety of this therapy. Shadman et al. (22) found that a longer bridging time may deteriorate patient prognosis. Those bridging to allo-HSCT ≥80 days were associated with a higher risk of death (HR= 4.01, 95% CI, 1.14–14.0; P = 0.03) and higher non-relapse mortality (HR= 4.4, 95% CI, 0.54–21.1; P = 0.19) than those transplanted within 80 days, consistent with the study at another center (17). Over time, CAR-T cells may gradually fail, and the risk of relapse will increase accordingly (20). Therefore, after patients stabilize from CAR-T therapy, they should bridge to HSCT as soon as possible to continuously suppress tumor cells and improve the long-term prognosis of patients.
Our meta-analysis has some limitations. First, because few randomized controlled trials exist for CAR-T therapy due to its novelty, some bias may have been introduced because of the nature of our study. Patients in the HSCT group may achieve better outcomes, partly because fitter patients were more likely to be chosen for transplant. Measures were taken to reduce such bias. For example, we compared the HSCT versus non-HSCT groups based on both achieving CR or MRD-neg CR, a strategy that should reduce bias to a certain extent. Second, the limited number of included studies and small sample sizes of several studies may compromise the accuracy of the results, also resulting in an unclear conclusion of the CD28 subgroup. Third, the analysis was not sufficiently thorough because of incomplete information, including the age, pretransplantation history, donor, timing, and conditioning therapy of each group. Thus, we cannot provide detailed recommendations for consolidative HSCT. Despite these limitations, our study still provides guidance for clinical practice and directions for future research.
Our study suggests that CD19 CAR-T cell therapy is an effective and safe bridge to HSCT. HSCT after CAR-T therapy can prolong OS and LFS and reduce the risk of relapse. MRD-neg CR after CAR-T cell therapy is a good prognostic indicator for HSCT. The incidence rates for adverse events did not increase significantly, and the safety of the combination therapy was acceptable. More randomized clinical trials with longer follow-up durations are needed to confirm these findings.
The original contributions presented in the study are included in the article/Supplementary Material. Further inquiries can be directed to the corresponding authors.
XXu, YL, and ST contributed to the study concept and design. SC, ZZ, ZH, XXiao, SH, and XXu contributed to the literature search, data collection, and data analysis. XXu, SC, ZZ, XXiao, and SH contributed to the drafting and review of the final manuscript. All authors contributed to the article and approved the submitted version.
This research was supported by grants from the Clinical Research Startup Program of Southern Medical University by High-level University Construction Funding of Guangdong Provincial Department of Education (No. LC2016ZD027), Guangdong Science and Technology Department (No. 2017A020215183), the Major Program for Health Medical Collaborative Innovation of Guangzhou (No. 201704020216), Natural Science Foundation of Guangdong Province, China (No. 2018B030311042), Frontier Research Program of Guangzhou Regenerative Medicine and Health Guangdong Laboratory (No. 2018GZR110105014), National Students' Platform for Innovation and Entrepreneurship Training Program of China (202012121025) and Special Funds for the Cultivation of Guangdong College Students’ Scientific and Technological Innovation (No. pdjh2021b0101).
The authors declare that the research was conducted in the absence of any commercial or financial relationships that could be construed as a potential conflict of interest.
XXu, SC, ZZ, XXiao, SH, and ZH are members of the Small Small bird (SSb) group and acknowledge the strong support from SSb.
The Supplementary Material for this article can be found online at: https://www.frontiersin.org/articles/10.3389/fonc.2021.651944/full#supplementary-material
Supplementary Table 1 | NOS assessment outcomes of the 11 cohort studies.
Supplementary Figure 1 | Forest plots of efficacy outcomes of consolidative HSCT after 4-1BB costimulatory CAR-T infusion. (A) Overall survival (OS) analysis; (B) Relapse rate analysis; (C) Leukemia-free survival (LFS) analysis.
Supplementary Figure 2 | IHE assessment outcomes of the 8 single-arm studies.
Supplementary Figure 3 | Begg’s plots of all outcome indicators.
1. Zhao H, Wei J, Wei G, Luo Y, Shi J, Cui Q, et al. Pre-transplant MRD negativity predicts favorable outcomes of CAR-T therapy followed by haploidentical HSCT for relapsed/refractory acute lymphoblastic leukemia: a multi-center retrospective study. J Hematol Oncol (2020) 13:42. doi: 10.1186/s13045-020-00873-7
2. Jabbour E, Short NJ, Jorgensen JL, Yilmaz M, Ravandi F, Wang SA, et al. Differential impact of minimal residual disease negativity according to the salvage status in patients with relapsed/refractory B-cell acute lymphoblastic leukemia. Cancer (2017) 123:294–302. doi: 10.1002/cncr.30264
3. Stein A, Palmer J, Tsai N, Al Malki M, Aldoss I, Ali H, et al. Phase I Trial of Total Marrow and Lymphoid Irradiation Transplantation Conditioning in Patients with Relapsed/Refractory Acute Leukemia. Biol Blood Marrow Transplant (2017) 23:618–24. doi: 10.1016/j.bbmt.2017.01.067
4. Moher D, Liberati A, Tetzlaff J, Altman DG. Preferred reporting items for systematic reviews and meta-analyses: the PRISMA statement. BMJ (2009) 21:b2535. doi: 10.1136/bmj.b2535
5. Gardner R, Finney O, Annesley C, Brakke H, Summers C, Leger K, et al. Intent-to-treat leukemia remission by CD19 CAR T cells of defined formulation and dose in children and young adults. Blood (2017) 129:3322–31. doi: 10.1182/blood-2017-02-769208
6. Gu B, Shi BY, Zhang X, Zhou SY, Chu JH, Wu XJ, et al. Allogeneic haematopoietic stem cell transplantation improves outcome of adults with relapsed/refractory Philadelphia chromosome-positive acute lymphoblastic leukemia entering remission following CD19 chimeric antigen receptor T cells. Bone Marrow Transplant (2020) 56(1):91–100. doi: 10.1038/s41409-020-0982-6
7. Jacoby E, Bielorai B, Avigdor A, Itzhaki O, Hutt D, Nussboim V, et al. Locally produced CD19 CAR T cells leading to clinical remissions in medullary and extramedullary relapsed acute lymphoblastic leukemia. Am J Hematol (2018) 93:1485–92. doi: 10.1002/ajh.25274
8. Jiang H, Li C, Yin P, Guo T, Liu L, Xia L, et al. Anti-CD19 chimeric antigen receptor-modified T-cell therapy bridging to allogeneic hematopoietic stem cell transplantation for relapsed/refractory B-cell acute lymphoblastic leukemia: An open-label pragmatic clinical trial. Am J Hematol (2019) 94:1113–22. doi: 10.1002/ajh.25582
9. Turtle CJ, Hanafi LA, Berger C, Gooley TA, Cherian S, Hudecek M, et al. CD19 CAR-T cells of defined CD4+:CD8+ composition in adult B cell ALL patients. J Clin Invest (2016) 126:2123–38. doi: 10.1172/JCI85309
10. Wang J, Mou N, Yang Z, Li Q, Jiang Y, Meng J, et al. Efficacy and safety of humanized anti-CD19-CAR-T therapy following intensive lymphodepleting chemotherapy for refractory/relapsed B acute lymphoblastic leukaemia. Br J Haematol (2020) 191(2):212–22. doi: 10.1111/bjh.16623
11. Park JH, Riviere I, Gonen M, Wang X, Senechal B, Curran KJ, et al. Long-Term Follow-up of CD19 CAR Therapy in Acute Lymphoblastic Leukemia. N Engl J Med (2018) 378:449–59. doi: 10.1056/NEJMoa1709919
12. Tierney JF, Stewart LA, Ghersi D, Burdett S, Sydes MR. Practical methods for incorporating summary time-to-event data into meta-analysis. Trials (2007) 8:16. doi: 10.1186/1745-6215-8-16
13. Victoria N, Marc AR, Marc AE. Metaprop: a Stata command toperform meta-analysis of binomial data. Arch Public Health (2014) 72:39. doi: 10.1186/2049-3258-72-39
14. Grigor EJM, Fergusson DA, Haggar F, Kekre N, Atkins H, Shorr R, et al. Efficacy and safety of chimeric antigen receptor T-cell (CAR-T) therapy in patients with haematological and solid malignancies: protocol for a systematic review and meta-analysis. BMJ Open (2017) 7:e019321. doi: 10.1136/bmjopen-2017-019321
15. Ai H, Yin Q, Wang Q, Fu Y, Wei X, Song Y. [Safety and efficacy of patients with refractory B-lymphoblastic leukemia treated with anti-CD19 CAR-T cell bridging to allogeneic hematopoietic stem cell transplantation]. Zhonghua xue ye xue za zhi (2020) 41:239–44. doi: 10.3760/cma.j.issn.0253-2727.2020.03.010
16. Cao J, Wang G, Cheng H, Wei C, Qi K, Sang W, et al. Potent anti-leukemia activities of humanized CD19-targeted Chimeric antigen receptor T (CAR-T) cells in patients with relapsed/refractory acute lymphoblastic leukemia. Am J Hematol (2018) 9:851–8. doi: 10.1002/ajh.25108
17. Fabrizio VA, Kernan NA, Boulad F, Cancio M, Allen J, Higman M, et al. Low toxicity and favorable overall survival in relapsed/refractory B-ALL following CAR T cells and CD34-selected T-cell depleted allogeneic hematopoietic cell transplant. Bone Marrow Transplant (2020) 55:2160–9. doi: 10.1038/s41409-020-0926-1
18. Hu Y, Wu Z, Luo Y, Shi J, Yu J, Pu C, et al. Potent Anti-leukemia activities of Chimeric antigen Receptor-modified T cells against CD19 in Chinese patients with relapsed/refractory acute lymphocytic leukemia. Clin Cancer Res (2017) 23:3297–306. doi: 10.1158/1078-0432.CCR-16-1799
19. Lee DW, Stetler-Stevenson M, CM Y, NN S, Delbrook C, Yates B, et al. Long-Term Outcomes Following CD19 CAR T Cell Therapy for B-ALL Are Superior in Patients Receiving a Fludarabine/Cyclophosphamide Preparative Regimen and Post-CAR Hematopoietic Stem Cell Transplantation. Blood (2016) 128:218. doi: 10.1182/blood.V128.22.218.218
20. Pan J, Yang JF, Deng BP, Zhao XJ, Zhang X, Lin YH, et al. High efficacy and safety of low-dose CD19-directed CAR-T cell therapy in 51 refractory or relapsed B acute lymphoblastic leukemia patients. Leukemia (2017) 31:2587–93. doi: 10.1038/leu.2017.145
21. Qasim W, Ciocarlie O, Adams S, Inglott S, Murphy C, Rivat C, et al. Preliminary Results of UCART19, an Allogeneic Anti-CD19 CAR T-Cell Product in a First-in-Human Trial (PALL) in Pediatric Patients with CD19+Relapsed/Refractory B-Cell Acute Lymphoblastic Leukemia. Blood (2017), 130(Suppl_1):1271. doi: 10.1182/blood.V130.Suppl_1.887.887
22. Shadman M, Gauthier J, Hay KA, Voutsinas JM, Milano F, Li A, et al. Safety of allogeneic hematopoietic cell transplant in adults after CD19-targeted CAR T-cell therapy. Blood Adv (2019) 3:3062–9. doi: 10.1182/bloodadvances.2019000593
23. Summers C, Annesley C, Bleakley M, Dahlberg A, Jensen MC, Gardner R. Long Term Follow-up after SCRI-CAR19v1 Reveals Late Recurrences As Well As a Survival Advantage to Consolidation with HCT after CAR T Cell Induced Remission. Blood (2018) 132(Suppl_1):967. doi: 10.1182/blood-2018-99-115599
24. Zhang X, Lu XA, Yang J, Zhang G, Li J, Song L, et al. Efficacy and safety of anti-CD19 CAR T-cell therapy in 110 patients with B-cell acute lymphoblastic leukemia with high-risk features. Blood Adv (2020) 4:2325–38. doi: 10.1182/bloodadvances.2020001466
25. Zuo Y, Jia Y, Wu J, Wang J, Lu A, Dong L, et al. Chimeric antigen receptors T cells for treatment of 48 relapsed or refractory acute lymphoblastic leukemia children: long term follow-up outcomes. Zhonghua xue ye xue za zhi (2019) 40:270–5. doi: 10.3760/cma.j.issn.0253-2727.2019.04.002
26. Pavlů J, Labopin M, Niittyvuopio R, Socié G, Yakoub-Agha I, Wu D, et al. Measurable residual disease at myeloablative allogeneic transplantation in adults with acute lymphoblastic leukemia: a retrospective registry study on 2780 patients from the acute leukemia working party of the EBMT. J Hematol Oncol (2019) 12:108. doi: 10.1186/s13045-019-0790-x
27. Hay KA, Gauthier J, Hirayama AV, Voutsinas JM, Wu Q, Li D, et al. Factors associated with durable EFS in adult B-cell ALL patients achieving MRD-negative CR after CD19 CAR T-cell therapy. Blood (2019) 133:1652–63. doi: 10.1182/blood-2018-11-883710
28. Lovisa F, Zecca M, Rossi B, Campeggio M, Magrin E, Giarin E, et al. Pre- and post-transplant minimal residual disease predicts relapse occurrence in children with acute lymphoblastic leukaemia. Br J Haematol (2018) 180:680–93. doi: 10.1111/bjh.15086
29. Zhao X, Liu Y, Xu L, Wang Y, Zhang X, Chen H, et al. Minimal residual disease status determined by multiparametric flow cytometry pretransplantation predicts the outcome of patients with ALL receiving unmanipulated haploidentical allografts. Am J Hematol (2019) 94:512–21. doi: 10.1002/ajh.25417
30. Frank MJ, Hossain N, Bukhari A, Dean E, Spiegel JY, Claire GK, et al. Detectable Circulating Tumor DNA 28 Days after the CD19 CAR T-Cell Therapy, Axicabtagene Ciloleucel, Is Associated with Poor Outcomes in Patients with Diffuse Large B-Cell Lymphoma. Blood (2019) 134(Supplement_1):884. doi: 10.1182/blood-2019-132057
31. Gust J, Hay KA, Hanafi LA, Li D, Myerson D, Gonzalez-Cuyar LF, et al. Endothelial Activation and Blood-Brain Barrier Disruption in Neurotoxicity after Adoptive Immunotherapy with CD19 CAR-T Cells. Cancer Discovery (2017) 7:1404–19. doi: 10.1158/2159-8290.CD-17-0698
32. Lee DW, Santomasso BD, Locke FL, Ghobadi A, Turtle CJ, Brudno JN, et al. ASTCT Consensus Grading for Cytokine Release Syndrome and Neurologic Toxicity Associated with Immune Effector Cells. Biol Blood Marrow Transplant (2019) 25:625–38. doi: 10.1016/j.bbmt.2018.12.758
33. Cassaday RD, Storer BE, Sorror ML, Sandmaier BM, Guthrie KA, Maloney DG, et al. Long-term outcomes of patients with persistent indolent B cell malignancies undergoing nonmyeloablative allogeneic transplantation. Biol Blood Marrow Transplant (2015) 21:281–7. doi: 10.1016/j.bbmt.2014.10.024
34. Rosko A, Wang HL, de Lima M, Sandmaier B, Khoury HJ, Artz A, et al. Reduced intensity conditioned allograft yields favorable survival for older adults with B-cell acute lymphoblastic leukemia. Am J Hematol (2017) 92:42–9. doi: 10.1002/ajh.24575
35. Salvatore D, Labopin M, Ruggeri A, Battipaglia G, Ghavamzadeh A, Ciceri F, et al. Outcomes of hematopoietic stem cell transplantation from unmanipulated haploidentical versus matched sibling donor in patients with acute myeloid leukemia in first complete remission with intermediate or high-risk cytogenetics: a study from the Acute Leukemia Working Party of the European Society for Blood and Marrow Transplantation. Haematologica (2018) 103:1317–28. doi: 10.3324/haematol.2018.189258
36. Wang Y, Wu DP, Liu QF, Xu LP, Liu KY, Zhang XH, et al. Low-dose post-transplant cyclophosphamide and anti-thymocyte globulin as an effective strategy for GVHD prevention in haploidentical patients. J Hematol Oncol (2019) 12:88. doi: 10.1186/s13045-019-0781-y
37. Wingard JR, Hsu J, Hiemenz JW. Hematopoietic stem cell transplantation: an overview of infection risks and epidemiology. Hematol Oncol Clin North Am (2011) 251:101–16. doi: 10.1016/j.hoc.2010.11.008
38. Bouziana S, Bouzianas D. Exploring the Dilemma of Allogeneic Hematopoietic Cell Transplantation after Chimeric Antigen Receptor T Cell Therapy: To Transplant or Not? Biol Blood Marrow Transplant (2020) 26:e183–91. doi: 10.1016/j.bbmt.2020.04.003
39. Zhang Y, Chen H, Song Y, Tan X, Zhao Y, Liu X, et al. Chimeric antigens receptor T cell therapy as a bridge to haematopoietic stem cell transplantation for refractory/relapsed B-cell acute lymphoblastic leukemia. Br J Haematol (2020) 189:146–52. doi: 10.1111/bjh.16339
40. Gardner R, Wu D, Cherian S, Fang M, Hanafi LA, Finney O, et al. Acquisition of a CD19-negative myeloid phenotype allows immune escape of MLL-rearranged B-ALL from CD19 CAR-T-cell therapy. Blood (2016) 127:2406–10. doi: 10.1182/blood-2015-08-665547
41. Nagel I, Bartels M, Duell J, Oberg HH, Ussat S, Bruckmueller H, et al. Hematopoietic stem cell involvement in BCR-ABL1-positive ALL as a potential mechanism of resistance to blinatumomab therapy. Blood (2017) 130:2027–31. doi: 10.1182/blood-2017-05-782888
42. Zhang X, Yang J, Shi Y, Song D, Li J, Lu X-A, et al. Analysis of Factors Predicting Treatment Response of 254 Patients Who Received CD19-Targeted CAR-T Cell Therapy for Relapsed/Refractory (R/R) B-Cell Acute Lymphoblastic Leukemia (B-ALL). Blood (2019) 134(Suppl_1):224. doi: 10.1182/blood-2019-125503
Keywords: meta-analysis, acute lymphoblastic leukemia, CD19, hematopoietic stem-cell transplantation, chimeric antigen receptor T (CAR-T)
Citation: Xu X, Chen S, Zhao Z, Xiao X, Huang S, Huo Z, Li Y and Tu S (2021) Consolidative Hematopoietic Stem Cell Transplantation After CD19 CAR-T Cell Therapy for Acute Lymphoblastic Leukemia: A Systematic Review and Meta-analysis. Front. Oncol. 11:651944. doi: 10.3389/fonc.2021.651944
Received: 11 January 2021; Accepted: 24 March 2021;
Published: 28 April 2021.
Edited by:
Jose A. Garcia-Sanz, Consejo Superior de Investigaciones Científicas (CSIC), SpainReviewed by:
He Huang, Zhejiang University, ChinaCopyright © 2021 Xu, Chen, Zhao, Xiao, Huang, Huo, Li and Tu. This is an open-access article distributed under the terms of the Creative Commons Attribution License (CC BY). The use, distribution or reproduction in other forums is permitted, provided the original author(s) and the copyright owner(s) are credited and that the original publication in this journal is cited, in accordance with accepted academic practice. No use, distribution or reproduction is permitted which does not comply with these terms.
*Correspondence: Yuhua Li, bGl5dWh1YTIwMTFnekAxNjMuY29t; Sanfang Tu, ZG9jdG9ydHV0dUAxNjMuY29t
†These authors have contributed equally to this work
Disclaimer: All claims expressed in this article are solely those of the authors and do not necessarily represent those of their affiliated organizations, or those of the publisher, the editors and the reviewers. Any product that may be evaluated in this article or claim that may be made by its manufacturer is not guaranteed or endorsed by the publisher.
Research integrity at Frontiers
Learn more about the work of our research integrity team to safeguard the quality of each article we publish.