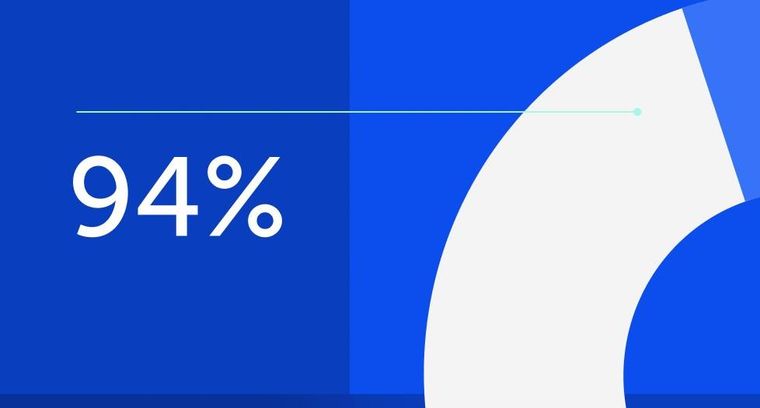
94% of researchers rate our articles as excellent or good
Learn more about the work of our research integrity team to safeguard the quality of each article we publish.
Find out more
REVIEW article
Front. Oncol., 28 April 2021
Sec. Genitourinary Oncology
Volume 11 - 2021 | https://doi.org/10.3389/fonc.2021.643065
This article is part of the Research TopicInfluences in the Progression of Renal Cell CarcinomaView all 15 articles
Context: The number of prognostic markers for clear cell renal cell carcinoma (ccRCC) has been increasing regularly over the last 15 years, without being integrated and compared.
Objective: Our goal was to perform a review of prognostic markers for ccRCC to lay the ground for their use in the clinics.
Evidence Acquisition: PubMed database was searched to identify RNA and protein markers whose expression level was reported as associated with survival of ccRCC patients. Relevant studies were selected through cross-reading by two readers.
Evidence Synthesis: We selected 249 studies reporting an association with prognostic of either single markers or multiple-marker models. Altogether, these studies were based on a total of 341 distinct markers and 13 multiple-marker models. Twenty percent of these markers were involved in four biological pathways altered in ccRCC: cell cycle, angiogenesis, hypoxia, and immune response. The main genes (VHL, PBRM1, BAP1, and SETD2) involved in ccRCC carcinogenesis are not the most relevant for assessing survival.
Conclusion: Among single markers, the most validated markers were KI67, BIRC5, TP53, CXCR4, and CA9. Of the multiple-marker models, the most famous model, ClearCode34, has been highly validated on several independent datasets, but its clinical utility has not yet been investigated.
Patient Summary: Over the years, the prognosis studies have evolved from single markers to multiple-marker models. Our review highlights the highly validated prognostic markers and multiple-marker models and discusses their clinical utility for better therapeutic care.
Clear cell renal cell carcinoma (ccRCC) is the most common histological subtype of kidney cancers, accounting for around 85% of all renal cell carcinomas (1). Although localized ccRCC can be treated by partial or total surgical ablation of the kidney, advanced ccRCC remains a clinical challenge, with 5-year overall survival (OS) rates of 0–20% (2). Over 90% of ccRCC cases have undergone a loss of heterozygosity of the chromosome 3p, where notably VHL is located. Moreover, VHL is mutated in 70% of ccRCC tumors and hypermethylated in 15% (3), and inactivating VHL mutation is considered the main driver of ccRCC carcinogenesis (4). Loss of VHL leads to activation of hypoxia-inducible factors and subsequently to vascular endothelial growth factor (VEGF)-mediated angiogenesis (5). Therefore, tyrosine kinase inhibitors with antiangiogenic properties have become a crucial treatment option for ccRCC patients (6).
Over the past decades, a large series of studies have aimed at finding prognostic markers for ccRCC in order to identify patients who were at higher risk of relapse and death. During this time, technologies have largely evolved—from surface proteins measured by single-molecule immunohistochemistry (IHC) to reverse-transcription quantitative polymerase chain reaction (RTQ-PCR) for mRNA and RNA sequencing (RNA-seq) for mRNA or long non-coding RNAs. Studies gradually incorporated more cases, while assessing an ever-larger number of putative targets, many focusing on angiogenesis-related targets, especially in the context of antiangiogenic therapies (7). Others emphasized immune-based approaches (8), as immune infiltration of tumors is a common prognostic factor in many different types of malignancies (9). Here, we aimed at reviewing prognostic markers that have been proposed for ccRCC during the 15 years between 2003 and 2018 through a thorough analysis of over 2,700 records from the literature.
A literature search was carried out using PubMed database to identify prognostic expression markers from studies published between 2003 and 2018. The PubMed query was: (clear cell renal cell carcinoma) AND (prognosis OR cancer prognosis OR cancer survival) AND (human OR Homo sapiens) AND (expression OR transcription OR transcriptome OR immunohistochemistry OR IHC). The search was conducted in December 2018.
The following inclusion criteria were applied: original article (not reviews, editorials, conference abstracts); English language; research was performed on human ccRCC tissue samples; and association of the expression level of candidate genes with patient survival was investigated in multivariate analyses in several independent datasets. The following patient survivals were considered: OS, progression-free survival (PFS), relapse-free survival (RFS), disease-free survival (DFS), cancer-specific survival (CSS), and metastasis-free survival (MFS). The studies describing transcriptome-based clusters of samples associated with patient survival were included. Case reports were excluded as well as studies performed on metastatic or advanced cohorts only. Two authors (SJ and FP) evaluated the titles and the abstracts of all 2,730 publications identified by the search strategy, and all 550 publications thought to be potentially relevant were retrieved in full (Figure 1A). The same authors then assessed full publications for eligibility. Any study was included in the review with the agreement of both authors.
Figure 1. (A) Consort diagram showing the selection process of studies included in the literature review. (B) Distribution of the studies investigating one marker, several markers, or multiple-marker models. (C) Venn diagram of the distribution of technologies used to quantify the expression level of the 341 genes. IHC, immunohistochemistry; TMA, tissue microarray; RNA-seq, RNA sequencing; RTQ-PCR, reverse-transcription quantitative polymerase chain reaction. (D) Distribution of the number of studies according to the type of biomaterial over the years: Frozen samples and/or formalin-fixed paraffin-embedded (FFPE) samples. The blue line indicates the number of studies using The Cancer Genome Atlas (TCGA) dataset as training or validation dataset.
The analyses were performed using R software version 3.5.2. We carried out hypergeometric tests on signaling pathways of three databases [Kyoto Encyclopedia of Genes and Genomes (KEGG), Gene Ontology (GO), and Reactome] using the reviewed prognostic markers. We used the gene lists with the most significant hypergeometric test p-values to illustrate the prognostic markers within the four mentioned pathways: angiogenesis (GO), hypoxia (Reactome), cell cycle (KEGG), and immunity (Reactome).
The PubMed query identified 2,730 publications (Figure 1A). Upon review, 2,180 publications were first excluded after title and abstract reading as being irrelevant to the present study, not available, or lacking validation. Of the remaining 550 publications, 301 studies were excluded due to the absence of validation on independent datasets or because of duplicate publication. Thus, the final total number of studies included in the present review was 249 (Figure 1A and Supplementary Table 1).
The 249 selected studies reported 341 distinct prognostic markers, 321 related to coding genes (mRNAs/proteins) and 20 to non-coding RNAs (six long non-coding RNAs, 14 microRNAs). While 169 out of the 249 studies (67.9%) focused on the prognostic impact of a single marker, the 80 remaining studies integrated multiple-marker analyses, 13 of them providing mathematical models computing a risk score (Figure 1B). Forty-one percent of markers (45/111) used in single-marker analyses were integrated in multiple-marker models. In the original publications, the expression levels of the prognostic markers were characterized using different technologies mainly represented by IHC, tissue microarray (TMA), RTQ-PCR, microarrays, and RNA-seq technologies (Figure 1C). These technologies exploit different types of biomaterials: IHC/TMA technologies generally use formalin-fixed paraffin-embedded (FFPE) samples and quantify a marker at a proteomic level, while RTQ-PCR, microarrays, and RNA-seq use frozen samples and quantify markers at a transcriptomic level. IHC and TMA were the most common identification methodologies used (143/341 targets, 41.9%). Among the 123 out of the 341 markers (36%) identified by two or more methodologies, 50 markers were validated both at the protein level (by IHC/TMA) and at the RNA level (by RTQ-PCR, RNA-seq, or microarrays) (Supplementary Table 1).
Over the years, we have remarked an increase in the number of analyzed frozen samples (Figure 1D). This increase is linked, on the one hand, to the growing accessibility to high-throughput technologies (microarrays and then RNA-seq) and, on the other hand, to the public datasets available in genomics data repositories such as Gene Expression Omnibus (GEO), array express, or the GDC data portal of The Cancer Genome Atlas (TCGA) Program. Eighty percent of studies using public datasets as training and/or validation sets use TCGA cohort composed of 532 ccRCC samples (Figure 1D). Our review excluded studies resulting from the analysis of familial cohorts and of advanced or metastatic cohorts. The studied cohorts essentially included unselected samples from ccRCC patients who have had a radical or partial nephrectomy, and the samples are primary tumors.
As previously stated, we excluded markers identified in a single study on a single cohort. Among the 341 prognostic markers, 250 markers were validated on internal datasets (Figure 2A). The 86 markers confirmed in two or more independent studies can be found in Supplementary Table 2. Seven markers were found in six or more studies (Figure 2B): KI67 (10–22), BIRC5 (23–32), TP53 (14, 18, 21, 33–39), CXCR4 (40–46), CA9 (47–53), miR-21 (54–59), and EZH2 (60–65). Some of them exceed the mere field of ccRCC. For instance, BIRC5 is able to inhibit cell death and is upregulated in most, if not all, cancers (66); TP53 is implicated in DNA damage repair and is mutated in a large portion of cancers (67). Of the 17 most reported prognostic markers, some are more tightly related to ccRCC, such as VHL (68–71), PBRM1 (72–75), CA9 (47–53), or CAV1 (76–79).
Figure 2. (A) Barplot of the number of markers cited in one or more studies. (B) Barplot of the most investigated prognostic markers. In orange are indicated prognostic markers specific to clear cell renal cell carcinoma (ccRCC). (C) Barplot of the number of studies investigating markers involved in the main biological pathways: angiogenesis, immunity, cell cycle, and hypoxia. Pies on the right represent the proportion of prognostic markers in the pathway. (D) Distribution of the studies assessing the prognostic value of genes on chromosome 3p over the years. (E) Barplot of the number of studies integrating clinical covariates. ECOG, Eastern Cooperative Oncology Group; VI, vascular invasion; BMI, body mass index; SSIGN, Stage, Size, Grade, and Necrosis; LVI, lymphovascular invasion; MVD, microvessel density; MSKCC, Memorial Sloan Kettering Cancer Center.
Of the 341 reviewed prognostic markers, 20% are involved in biological pathways altered in ccRCC (80) of which the main ones are cell cycle, angiogenesis, hypoxia, and immune response (Figure 2C), which are involved in classical hallmarks of cancer (81).
VHL inactivation, through mutation, hypermethylation, and/or loss of heterozygosity (3), is regarded as the key genetic event leading to ccRCC formation (4). This dysregulation of the Von Hippel–Lindau pathway leads to HIF1α-HIF1β transcription factor activation and increased hypoxia response and neoangiogenesis through VEGF signaling. Expectedly, hypoxia and angiogenesis pathways are importantly represented in the list of prognostic markers that have been identified in the literature (Figure 2C). Sixty-nine studies listed at least one gene associated with angiogenesis, and 35 such genes were identified: AAMP (82), ANPEP (83), APOLD1 (82), B4GALT2 (84), C5 (85), CAV1 (76–79), CCL2 (86, 87), CCR2 (87), CEACAM1 (82), CTGF (83), CTNNB1 (88–91), CX3CL1 (82), CXCL10 (92), CXCL12 (42), CXCR2 (93), CXCR4 (40–46), EPAS1 (94–96), FLT1 (14), GPX1 (82), HIF1A (52, 97, 98), HPSE (99, 100), IL6 (82), JAG1 (101, 102), MMP2 (103), NOS3 (82), NOTCH1 (102, 104, 105), NRP1 (95), PDGFRB (106, 107), PTEN (108–110), SERPINE1 (111–113), SETD2 (74, 114, 115), TGFBR2 (116, 117), THSD7A (118), VASH1 (100, 119), VEGFA (50, 120, 121). Six markers of hypoxia [ARNT (95), CA9 (47–53), EPAS1 (94–96), HIF1A (52, 97, 98), VEGFA (50, 120, 121), and VHL (68–71)], representing about 30% of the genes involved in the hypoxia pathway, are reported as prognostic markers in 18 publications. Of note, three of these markers (EPAS1, HIF1A, and VEGFA) also belong to the angiogenesis pathway.
ccRCC tumors exhibit a rather low mutational burden compared to other tumor types (122). Nonetheless, they have been one of the first tumor types for which immunotherapy with high-dose IL-2 has proved efficient (123), although their responsiveness to immune checkpoint blockade remains rather low, below 30% (124). Moreover, immunity has been repeatedly associated with clinical outcome for this pathology (8). We found 59 articles identifying at least one gene related to immunity (among other pathways) as a prognostic factor in ccRCC. Twenty-nine such genes were identified: AKT1 (77, 108), ANAPC5 (125), ARF1 (82), BCL2 (39, 126), C5 (85), CARD9 (127), CCR2 (87), CD274 (26, 128, 128–130), CD4 (118, 131, 132), CD44 (100, 133), CDH1 (63, 103, 134, 135), CDK1 (136), CDKN1A (17, 18, 137), CDKN1B (138–141), CIITA (127), DEFB1 (83, 142), ICOS (118, 143), IKBKE (144), IL5RA (145), IL6 (82), MDM2 (34), NCF2 (127), PAK1 (146), PSMD9 (18, 147), PTEN (108–110), RCHY1 (148), TLR3 (95), VCAM1 (95, 149), and VHL (68–71) (Figure 2C). Some of them were independently reported by several publications. For instance, CXCR4 (40–46), which encodes a receptor for the lymphocyte chemoattractant CXCL12, was identified by seven publications. ICOS, a T cell co-stimulatory molecule, was reported as prognostic biomarker by two publications.
Expression of cell cycle-related gene signatures or proteins is generally a marker of the presence of highly proliferative cells and is therefore widely regarded as a biomarker of aggressive malignancy and poor prognosis (150, 151). Here, we have observed 11 such genes, reported in 20 publications: ANAPC5 (125), CCND1 (18), CDC7 (125), CDK1 (136), CDKN1A (17, 18, 137), CDKN1B (138–141), CDKN1C (18), CDKN2A (18, 152), GADD45G (127), MDM2 (34), and TP53 (14, 18, 21, 33–39).
Over 90% of sporadic ccRCC displays a deletion of chromosome 3p. The ccRCC key event is the alteration of the tumor-suppressor gene VHL (3p25-p26). Its prognostic impact was mainly studied a few years ago (between 2003 and 2007; Figure 2D), but the results were not very significant and often associated with specific subcellular locations (69, 70). Its validation as a prognostic marker was then neglected until recently in a study investigating the cumulative roles of PBRM1 and VHL as risk factors (71).
Whole exome sequencing helped to identify the three other frequently mutated genes on chromosome 3p: PBRM1 (~40%), SETD2 (~12%), and BAP1 (~10%) (71). Several studies investigated their prognostic values since 2014. Studies about PBRM1 (71, 72, 74, 75) were contradictory. While authors of the study (75) validate PBRM1 as an independent predictor of PFS but not of OS, Jiang et al. (74) and Högner et al. (71) showed opposite results. The prognostic value of BAP1 was dependent on the cellular localization (153) but was only validated by bivariate Cox models (154) or in combination with the expression of PBRM1 (72). Finally, SETD2 was studied in three studies (74, 114, 115) in combination or not with the expression of H3K36me3, and all results agreed with the prognostic role of SETD2.
In conclusion, despite the high rate of alterations of these four genes on chromosome 3p and their role in ccRCC carcinogenesis and progression, their prognostic value may be ambiguous, explaining why these markers were not in the most reported prognostic markers, except VHL.
Our review focused on independent prognostic markers, meaning that their prognostic impacts evaluated by Cox models remain significant after inclusion of other clinical and/or molecular covariates. Here, 242 out of the 249 studies integrated one or more clinical covariates, while seven studies (22, 74, 121, 130, 132, 155, 156) concluded the independence of the prognostic markers only from comparisons with other molecular markers. The studies using one or more bivariate Cox models were integrated in our review despite the lack of a global multivariate Cox model. The selected studies focused on different types of survival: OS, CSS, PFS, DFS, RFS, or MFS. When filled in, the starting times used to compute survival data may also differ between studies using either date at diagnosis or date at surgery. All these aspects make a direct comparison of prognostic values difficult.
Figure 2E summarizes the clinical covariates used in the 242 studies. The most represented clinical covariates are age, stage, and grade in adequacy with their known prognostic value. The clinical covariates specific to ccRCC such as Fuhrman grade or Stage, Size, Grade, and Necrosis score (SSIGN score) were also represented but to a lesser extent.
The authors of the reviewed studies present multivariate Cox models in the goal to validate the independence of the molecular predictors they studied. In our point of view, they validate above all the use of clinical and molecular covariates to better predict the survival of patients with ccRCC.
Eighty out of the 249 selected studies investigated the prognostic value of several markers. We distinguish multi-marker analyses (n = 67 studies; Figure 1B) evaluating several independent predictors in multivariate Cox models and multiple-marker model analyses (n = 13 studies) providing a mathematical model that computes a risk score (36, 54, 55, 57, 82, 83, 95, 125, 135, 136, 149, 157, 158). These multiple-marker models were calibrated against a given technology used to quantify expression values (RTQ-PCR, RNA-seq, or nanostring) and used from 2 to 34 markers (Supplementary Table 3). Ten out of these multiple-marker models provide a mathematical formula, represented by a weighted sum of the expression values of each prognostic marker with or without clinical covariates. Three studies provided models based on microRNA expression (54, 55, 57). Mlcochova et al. (135) focused on genes involved in epithelial–mesenchymal transition, and Yang et al. (136) computed a risk model using genes in interaction with the nucleotide degrading enzyme gene RNASEH2A. Two unsupervised classifications based on whole transcriptome proposed prognostic ccRCC subtyping (157, 158). Brannon et al. (157) identified two subtypes (ccA and ccB), and Beuselinck et al. (158) proposed four subtype names ccrcc1 to ccrcc4. The two classifications identified subtypes related to a worse prognosis (Brannon: ccB; Beuselinck: ccrcc1 and ccrcc4). The classification by Beuselinck et al. (158) was also related to response to antiangiogenic treatment by sunitinib. The now well-established clinico-molecular prognostic model (95), ClearCode34, was built from the classification by Brannon et al. (157) added to clinical covariates (stage and Fuhrman grade). It is the single risk model using clinical and molecular markers.
We propose a sub-selection of the most validated prognostic predictors with a potential clinical utility. About the single prognostic markers, we focused on the prognostic markers validated on more than seven independent studies (Figure 2B), validated on fewer technologies based on FFPE and frozen samples. We imposed a prognostic impact independent of the classical clinical covariates (stage, Fuhrman grade, age, gender, and grade) on a large set of samples. The five markers (KI67, BIRC5, TP53, CXCR4, and CA9) validated on seven or more independent studies were validated on FFPE and frozen samples and compared with a large set of clinical covariates. KI67 and BIRC5 remained the more confident given their validation on more than 5,000 samples. The quantification of KI67 was used as secondary objective in two clinical trials on ccRCC patients (NCT03575611 and NCT01253668) to evaluate response to treatment. Three clinical trials in ccRCC patients (NCT02787915, NCT00197860, and NCT01924156) integrated the use of BIRC5 to assess survival or response to BIRC5-loaded dendritic cell vaccines.
We have been less strict on the sub-selection of multiple-marker models, as these models are newer. We focused on gene models validated at least in one independent study (Supplementary Table 3) and whose clinical use has been evaluated in a clinical trial. Six out of the 13 multiple-marker models were validated in external datasets, but only the clinical utility of the 16-gene assay of Rini et al. (82) and the ccrcc classification of Beuselinck et al. (158) were tested in a phase III (NCT00375674) and a phase II (NCT02960906) clinical trial, respectively.
In this review, we conducted an extensive analysis of the literature on prognostic markers in ccRCC over the last 15 years. Published studies evolved according to technological progresses. The oldest studies mainly focused on the prognosis impact of single genes known to be involved in the ccRCC carcinogenesis such as VHL or HIF1A, mostly validated by IHC. Over the years, the high-throughput technologies allowed the prognosis analysis of the whole transcriptome as well as the integration of non-coding RNA as microRNA and long non-coding RNA showing promising results that still require further validations.
We identified 20% of the 341 reviewed prognostic markers as involved in biological pathways altered in ccRCC of which the main ones are cell cycle, angiogenesis, hypoxia, and immune response. Interestingly, the prognostic immune genes are mainly related to inflammation such as IKBKE that plays a role in regulating inflammatory responses to viral infection (159), the well-known proinflammatory cytokine interleukin-6 (IL6) (160), the complement C5 (161), or the receptor of hyaluronic acid, CD44, involved in inflammation and tissue regeneration (162). These inflammatory markers are mainly related to poor prognosis in agreement with inflammation being a cancer-fueling factor (163).
Among single markers, the most validated markers (KI67, BIRC5, TP53, CXCR4, and CA9) exceed the mere field of ccRCC, except CA9. Two of them, KI67 and BIRC5, were validated on the largest sets of samples, and they are beginning to be used in clinical trials. Among the 13 multiple-marker models proposed in the literature, the most validated ones are the 16-gene assay and the ccrcc1-4 subtyping. Paradoxically, the most famous model, ClearCode34, has been highly validated on several independent datasets, but its clinical utility has not yet been investigated. This review highlights the prognostic molecular predictors that should be investigated in more detail to improve therapeutic care and recommends to focus on the most validated markers or models (KI67, BIRC5, the 16-gene assay, and the ccrcc1-4 subtyping) to be quantified on FFPE samples for an easier clinical use. An important preliminary test should be first to ensure the reproducibility of the quantification on several samples of the same tumor to avoid contradictory conclusions.
Our review has some limitations. Several sources of heterogeneity make difficult the comparison between studies. First, the start points used to compute the survival delays (diagnosis vs. surgery) as well as the type of events (OS, MFS, RFS…) may differ. Another limitation is that we kept markers with a prognostic impact on OS but not RFS or inversely, as well as markers recurrently found to be prognostic even if other studies showed no significance. That is the case of genes mostly studied given their role in the ccRCC carcinogenesis like VHL and BAP1 (164, 165). All the markers proposed in the review were validated in multivariate models in at least two datasets. According to the studies, the multivariate models integrated several genes and/or clinicopathological covariates, but the available clinical annotations strongly differ too. Finally, the increased use of abdominal imaging has resulted in an increase in the number of small renal incidentaloma in recent decades (166). Consequently, the clinical characteristics of the patient cohorts evolved over the 15 years, with an increase of the proportion of early stages. All these heterogeneity sources can explain why some markers can be found to be significantly associated with survival in some studies and not in others. In this context, it is important to note that some markers, notably the ccrcc1-4 molecular subtyping, proved to be related to older therapies, notably antiangiogenic drugs (158), but their predictive power is being considered also for more recent immunotherapies with immune checkpoint inhibitors, as illustrated in clinical trials [BIONIKK trial, NCT02960906 (167)].
In addition to the gene expression markers reviewed here, methylation markers such as the ones that were reviewed by Joosten et al. (168) and mutational markers as the ones reviewed by Mitchell et al. (169) can also be considered and could be integrated in multi-omics prognostic models. Combining the prognostic impact of these omics could improve the accuracy of survival prediction. Altogether, the present comprehensive analysis paves the way to robust and accurate evaluation of the risk of relapse and death for patients with ccRCC.
FP: conceptualization, methodology, validation, formal analysis, investigation, resources, data curation, writing—review and editing, and funding acquisition. MA and AR: conceptualization, writing—review and editing, and funding acquisition. WF and CS-F: conceptualization, writing—review and editing, supervision, and project administration. SJ: conceptualization, methodology, validation, formal analysis, investigation, resources, data curation, writing—review and editing, funding acquisition, supervision, and project administration. All authors contributed to the article and approved the submitted version. All authors listed have made substantial, direct, and intellectual contribution to the work and approved it for publication.
The authors declare that the research was conducted in the absence of any commercial or financial relationships that could be construed as a potential conflict of interest.
The Supplementary Material for this article can be found online at: https://www.frontiersin.org/articles/10.3389/fonc.2021.643065/full#supplementary-material
Supplementary Table 1. Studies included in the literature review, identified by their PubMed ID. The second column indicates the technologies and the third column the prognostic markers.
Supplementary Table 2. List of the 87 prognostic markers validated on one or more independent studies, identified by the PubMed ID.
Supplementary Table 3. Summary of the 13 multi-marker models.
1. Jemal A, Siegel R, Ward E, Murray T, Xu J, Thun MJ. Cancer statistics, 2007. CA Cancer J Clin. (2007) 57:43–66. doi: 10.3322/canjclin.57.1.43
2. Ballarin R, Spaggiari M, Cautero N, De Ruvo N, Montalti R, Longo C, et al. Pancreatic metastases from renal cell carcinoma: the state of the art. World J Gastroenterol. (2011) 17:4747–56. doi: 10.3748/wjg.v17.i43.4747
3. Sato Y, Yoshizato T, Shiraishi Y, Maekawa S, Okuno Y, Kamura T, et al. Integrated molecular analysis of clear-cell renal cell carcinoma. Nat Genet. (2013) 45:860–7. doi: 10.1038/ng.2699
4. Turajlic S, Xu H, Litchfield K, Rowan A, Horswell S, Chambers T, et al. Deterministic evolutionary trajectories influence primary tumor growth: TRACERx renal. Cell. (2018) 173:595–610.e11. doi: 10.1016/j.cell.2018.03.043
5. Casuscelli J, Vano Y-A, Fridman WH, Hsieh JJ. Molecular classification of renal cell carcinoma and its implication in future clinical practice. Kidney Cancer. (2017) 1:3–13. doi: 10.3233/KCA-170008
6. Lee C-H, Motzer RJ. Kidney cancer in 2016: the evolution of anti-angiogenic therapy for kidney cancer. Nat Rev Nephrol. (2017) 13:69–70. doi: 10.1038/nrneph.2016.194
7. D'Aniello C, Berretta M, Cavaliere C, Rossetti S, Facchini BA, Iovane G, et al. Biomarkers of prognosis and efficacy of anti-angiogenic therapy in metastatic clear cell renal cancer. Front Oncol. (2019) 9:1400. doi: 10.3389/fonc.2019.01400
8. Vano Y-A, Petitprez F, Giraldo NA, Fridman WH, Sautès-Fridman C. Immune-based identification of cancer patients at high risk of progression. Curr Opin Immunol. (2018) 51:97–102. doi: 10.1016/j.coi.2018.03.005
9. Fridman WH, Zitvogel L, Sautès–Fridman C, Kroemer G. The immune contexture in cancer prognosis and treatment. Nat Rev Clin Oncol. (2017) 14:717–34. doi: 10.1038/nrclinonc.2017.101
10. Visapää H, Bui M, Huang Y, Seligson D, Tsai H, Pantuck A, et al. Correlation of Ki-67 and gelsolin expression to clinical outcome in renal clear cell carcinoma. Urology. (2003) 61:845–50. doi: 10.1016/S0090-4295(02)02404-4
11. Rioux-Leclercq N, Delcros J-G, Bansard J-Y, Epstein JI, Patard J-J, Lobel B, et al. Immunohistochemical analysis of tumor polyamines discriminates high-risk patients undergoing nephrectomy for renal cell carcinoma. Hum Pathol. (2004) 35:1279–84. doi: 10.1016/j.humpath.2004.07.002
12. Kankuri M, Söderström K-O, Pelliniemi T-T, Vahlberg T, Pyrhönen S, Salminen E. The association of immunoreactive p53 and Ki-67 with T-stage, grade, occurrence of metastases and survival in renal cell carcinoma. Anticancer Res. (2006) 26:3825–33.
13. Tollefson MK, Thompson RH, Sheinin Y, Lohse CM, Cheville JC, Leibovich BC, et al. Ki-67 and coagulative tumor necrosis are independent predictors of poor outcome for patients with clear cell renal cell carcinoma and not surrogates for each other. Cancer. (2007) 110:783–90. doi: 10.1002/cncr.22840
14. Klatte T, Seligson DB, LaRochelle J, Shuch B, Said JW, Riggs SB, et al. Molecular signatures of localized clear cell renal cell carcinoma to predict disease-free survival after nephrectomy. Cancer Epidemiol Biomark Prev. (2009) 18:894–900. doi: 10.1158/1055-9965.EPI-08-0786
15. Miralles A, Bracamonte L, Soncul H, Diaz del Castillo R, Akhtar R, Bors V, et al. Cardiac tumors: clinical experience and surgical results in 74 patients. Ann Thorac Surg. (1991) 52:886–95. doi: 10.1016/0003-4975(91)91241-M
16. Sakai I, Miyake H, Takenaka A, Fujisawa M. Expression of potential molecular markers in renal cell carcinoma: impact on clinicopathological outcomes in patients undergoing radical nephrectomy. BJU Int. (2009) 104:942–6. doi: 10.1111/j.1464-410X.2009.08591.x
17. Weber T, Meinhardt M, Zastrow S, Wienke A, Fuessel S, Wirth MP. Immunohistochemical analysis of prognostic protein markers for primary localized clear cell renal cell carcinoma. Cancer Invest. (2013) 31:51–9. doi: 10.3109/07357907.2012.749267
18. Gayed BA, Youssef RF, Bagrodia A, Kapur P, Darwish OM, Krabbe L-M, et al. Prognostic role of cell cycle and proliferative biomarkers in patients with clear cell renal cell carcinoma. J Urol. (2013) 190:1662–7. doi: 10.1016/j.juro.2013.06.037
19. Gayed BA, Youssef RF, Bagrodia A, Darwish OM, Kapur P, Sagalowsky A, et al. Ki67 is an independent predictor of oncological outcomes in patients with localized clear-cell renal cell carcinoma. BJU Int. (2014) 113:668–73. doi: 10.1111/bju.12263
20. Abel EJ, Bauman TM, Weiker M, Shi F, Downs TM, Jarrard DF, et al. Analysis and validation of tissue biomarkers for renal cell carcinoma using automated high-throughput evaluation of protein expression. Hum Pathol. (2014) 45:1092–9. doi: 10.1016/j.humpath.2014.01.008
21. Zheng K, Zhu W, Tan J, Wu W, Yang S, Zhang J. Retrospective analysis of a large patient sample to determine p53 and Ki67 expressions in renal cell carcinoma. J. BUON. (2014) 19:512–6.
22. Kim SH, Park WS, Park EY, Park B, Joo J, Joung JY, et al. The prognostic value of BAP1, PBRM1, pS6, PTEN, TGase2, PD-L1, CA9, PSMA, and Ki-67 tissue markers in localized renal cell carcinoma: a retrospective study of tissue microarrays using immunohistochemistry. PLoS ONE. (2017) 12:e0179610. doi: 10.1371/journal.pone.0179610
23. Parker AS, Kosari F, Lohse CM, Houston Thompson R, Kwon ED, Murphy L, et al. High expression levels of survivin protein independently predict a poor outcome for patients who undergo surgery for clear cell renal cell carcinoma. Cancer. (2006) 107:37–45. doi: 10.1002/cncr.21952
24. Kosari F, Parker AS, Kube DM, Lohse CM, Leibovich BC, Blute ML, et al. Clear cell renal cell carcinoma: gene expression analyses identify a potential signature for tumor aggressiveness. Clin Cancer Res. (2005) 11:5128–39. doi: 10.1158/1078-0432.CCR-05-0073
25. Byun S-S, Yeo WG, Lee SE, Lee E. Expression of survivin in renal cell carcinomas: association with pathologic features and clinical outcome. Urology. (2007) 69:34–7. doi: 10.1016/j.urology.2006.09.024
26. Krambeck AE, Dong H, Thompson RH, Kuntz SM, Lohse CM, Leibovich BC, et al. Survivin and b7-h1 are collaborative predictors of survival and represent potential therapeutic targets for patients with renal cell carcinoma. Clin Cancer Res. (2007) 13:1749–56. doi: 10.1158/1078-0432.CCR-06-2129
27. Parker AS, Lohse CM, Leibovich BC, Cheville JC, Sheinin YM, Kwon ED. Comparison of digital image analysis versus visual assessment to assess survivin expression as an independent predictor of survival for patients with clear cell renal cell carcinoma. Hum Pathol. (2008) 39:1176–84. doi: 10.1016/j.humpath.2007.12.009
28. Zamparese R, Pannone G, Santoro A, Lo Muzio L, Corsi F, Pedicillo MC, et al. Survivin expression in renal cell carcinoma. Cancer Invest. (2008) 26:929–35. doi: 10.1080/07357900802017553
29. Lei Y, Geng Z, Guo-Jun W, He W, Jian-Lin Y. Prognostic significance of survivin expression in renal cell cancer and its correlation with radioresistance. Mol Cell Biochem. (2010) 344:23–31. doi: 10.1007/s11010-010-0525-3
30. Shi Z-G, Li S-Q, Li Z-J, Zhu X-J, Xu P, Liu G. Expression of vimentin and survivin in clear cell renal cell carcinoma and correlation with p53. Clin Transl Oncol. (2015) 17:65–73. doi: 10.1007/s12094-014-1199-1
31. Ma C, Lu B, Sun E. Clinicopathological and prognostic significance of survivin expression in renal cancer patients: a meta-analysis. Postgrad Med J. (2017) 93:186–92. doi: 10.1136/postgradmedj-2016-134105
32. Pu Z, Wang Q, Xie H, Wang G, Hao H. Clinicalpathological and prognostic significance of survivin expression in renal cell carcinoma: a meta-analysis. Oncotarget. (2017) 8:19825–33. doi: 10.18632/oncotarget.15082
33. Zigeuner R, Ratschek M, Rehak P, Schips L, Langner C. Value of p53 as a prognostic marker in histologic subtypes of renal cell carcinoma: a systematic analysis of primary and metastatic tumor tissue. Urology. (2004) 63:651–5. doi: 10.1016/j.urology.2003.11.011
34. Noon AP, Polański R, El-Fert AY, Kalirai H, Shawki H, Campbell F, et al. Combined p53 and MDM2 biomarker analysis shows a unique pattern of expression associated with poor prognosis in patients with renal cell carcinoma undergoing radical nephrectomy. BJU Int. (2012) 109:1250–7. doi: 10.1111/j.1464-410X.2011.10433.x
35. Abolhasani M, Salarinejad S, Asgari M. P53 and MDM2 over-expression and five-year survival of kidney cancer patients undergoing radical nephrectomy–Iranian experience. Asian Pac J Cancer Prev. (2015) 16:5043–7. doi: 10.7314/APJCP.2015.16.12.5043
36. Zhan Y, Guo W, Zhang Y, Wang Q, Xu X, Zhu L. A five-gene signature predicts prognosis in patients with kidney renal clear cell carcinoma. Comput Math Methods Med. (2015) 2015:842784. doi: 10.1155/2015/842784
37. Cho DS, Joo HJ, Oh DK, Kang JH, Kim YS, Lee KB, et al. Cyclooxygenase-2 and p53 expression as prognostic indicators in conventional renal cell carcinoma. Yonsei Med J. (2005) 46:133–140. doi: 10.3349/ymj.2005.46.1.133
38. Shvarts O, Seligson D, Lam J, Shi T, Horvath S, Figlin R, et al. p53 is an independent predictor of tumor recurrence and progression after nephrectomy in patients with localized renal cell carcinoma. J Urol. (2005) 173:725–8. doi: 10.1097/01.ju.0000152354.08057.2a
39. Phuoc NB, Ehara H, Gotoh T, Nakano M, Yokoi S, Deguchi T, et al. Immunohistochemical analysis with multiple antibodies in search of prognostic markers for clear cell renal cell carcinoma. Urology. (2007) 69:843–8. doi: 10.1016/j.urology.2007.01.069
40. D'Alterio C, Consales C, Polimeno M, Franco R, Cindolo L, Portella L, et al. Concomitant CXCR4 and CXCR7 expression predicts poor prognosis in renal cancer. Curr Cancer Drug Targets. (2010) 10:772–81. doi: 10.2174/156800910793605839
41. D'Alterio C, Cindolo L, Portella L, Polimeno M, Consales C, Riccio A, et al. Differential role of CD133 and CXCR4 in renal cell carcinoma. Cell Cycle Georget Tex. (2010) 9:4492–500. doi: 10.4161/cc.9.22.13680
42. Wang L, Chen W, Gao L, Yang Q, Liu B, Wu Z, et al. High expression of CXCR4, CXCR7 and SDF-1 predicts poor survival in renal cell carcinoma. World J Surg Oncol. (2012) 10:212. doi: 10.1186/1477-7819-10-212
43. Li G, Badin G, Zhao A, Gentil-Perret A, Tostain J, Péoc'h M, et al. Prognostic value of CXCR4 expression in patients with clear cell renal cell carcinoma. Histol Histopathol. (2013) 28:1217–22. doi: 10.14670/HH-28.1217
44. Chen D, Gassenmaier M, Maruschke M, Riesenberg R, Pohla H, Stief CG, et al. Expression and prognostic significance of a comprehensive epithelial-mesenchymal transition gene set in renal cell carcinoma. J Urol. (2014) 191:479–86. doi: 10.1016/j.juro.2013.08.052
45. An H, Xu L, Zhu Y, Lv T, Liu W, Liu Y, et al. High CXC chemokine receptor 4 expression is an adverse prognostic factor in patients with clear-cell renal cell carcinoma. Br J Cancer. (2014) 110:2261–8. doi: 10.1038/bjc.2014.179
46. Li X, Huang Y, Xia J, Chen N, Wei Q, Li X, et al. CXCR4 expression in patients with high-risk locally advanced renal cell carcinoma can independently predict increased risk of disease progression and poor overall survival. Asian Pac J Cancer Prev. (2011) 12:3313–8.
47. Li G, Feng G, Gentil-Perret A, Genin C, Tostain J. CA9 gene expression in conventional renal cell carcinoma: a potential marker for prediction of early metastasis after nephrectomy. Clin Exp Metastasis. (2007) 24:149–55. doi: 10.1007/s10585-007-9064-z
48. Sandlund J, Oosterwijk E, Grankvist K, Oosterwijk-Wakka J, Ljungberg B, Rasmuson T. Prognostic impact of carbonic anhydrase IX expression in human renal cell carcinoma. BJU Int. (2007) 100:556–60. doi: 10.1111/j.1464-410X.2007.07006.x
49. Patard J-J, Fergelot P, Karakiewicz PI, Klatte T, Trinh Q-D, Rioux-Leclercq N, et al. Low CAIX expression and absence of VHL gene mutation are associated with tumor aggressiveness and poor survival of clear cell renal cell carcinoma. Int J Cancer. (2008) 123:395–400. doi: 10.1002/ijc.23496
50. Phuoc NB, Ehara H, Gotoh T, Nakano M, Kamei S, Deguchi T, et al. Prognostic value of the co-expression of carbonic anhydrase IX and vascular endothelial growth factor in patients with clear cell renal cell carcinoma. Oncol Rep. (2008) 20:525–30.
51. Chamie K, Klöpfer P, Bevan P, Störkel S, Said J, Fall B, et al. Carbonic anhydrase-IX score is a novel biomarker that predicts recurrence and survival for high-risk, nonmetastatic renal cell carcinoma: data from the phase III ARISER clinical trial. Urol Oncol. (2015) 33:204.e25–33. doi: 10.1016/j.urolonc.2015.02.013
52. Cros J, Sbidian E, Posseme K, Letierce A, Guettier C, Benoît G, et al. Nestin expression on tumour vessels and tumour-infiltrating macrophages define a poor prognosis subgroup of pt1 clear cell renal cell carcinoma. Virchows Arch Int J Pathol. (2016) 469:331–7. doi: 10.1007/s00428-016-1973-2
53. Ingels A, Hew M, Algaba F, de Boer OJ, van Moorselaar RJA, Horenblas S, et al. Vimentin over-expression and carbonic anhydrase IX under-expression are independent predictors of recurrence, specific and overall survival in non-metastatic clear-cell renal carcinoma: a validation study. World J Urol. (2017) 35:81–7. doi: 10.1007/s00345-016-1854-y
54. Vergho D, Kneitz S, Rosenwald A, Scherer C, Spahn M, Burger M, et al. Combination of expression levels of miR-21 and miR-126 is associated with cancer-specific survival in clear-cell renal cell carcinoma. BMC Cancer. (2014) 14:25. doi: 10.1186/1471-2407-14-25
55. Fritz HKM, Lindgren D, Ljungberg B, Axelson H, Dahlbäck B. The miR(21/10b) ratio as a prognostic marker in clear cell renal cell carcinoma. Eur J Cancer. (2014) 50:1758–65. doi: 10.1016/j.ejca.2014.03.281
56. Ge Y-Z, Wu R, Xin H, Zhu M, Lu T-Z, Liu H, et al. A tumor-specific microRNA signature predicts survival in clear cell renal cell carcinoma. J Cancer Res Clin Oncol. (2015) 141:1291–9. doi: 10.1007/s00432-015-1927-0
57. Christinat Y, Krek W. Integrated genomic analysis identifies subclasses and prognosis signatures of kidney cancer. Oncotarget. (2015) 6:10521–31. doi: 10.18632/oncotarget.3294
58. Tang K, Xu H. Prognostic value of meta-signature miRNAs in renal cell carcinoma: an integrated miRNA expression profiling analysis. Sci Rep. (2015) 5:10272. doi: 10.1038/srep10272
59. Liang B, Zhao J, Wang X. A three-microRNA signature as a diagnostic and prognostic marker in clear cell renal cancer: an in silico analysis. PLoS ONE. (2017) 12:e0180660. doi: 10.1371/journal.pone.0180660
60. Hinz S, Weikert S, Magheli A, Hoffmann M, Engers R, Miller K, et al. Expression profile of the polycomb group protein enhancer of Zeste homologue 2 and its prognostic relevance in renal cell carcinoma. J Urol. (2009) 182:2920–5. doi: 10.1016/j.juro.2009.08.014
61. Wagener N, Macher-Goeppinger S, Pritsch M, Hüsing J, Hoppe-Seyler K, Schirmacher P, et al. Enhancer of zeste homolog 2 (EZH2) expression is an independent prognostic factor in renal cell carcinoma. BMC Cancer. (2010) 10:524. doi: 10.1186/1471-2407-10-524
62. Liu L, Xu Z, Zhong L, Wang H, Jiang S, Long Q, et al. Prognostic value of EZH2 expression and activity in renal cell carcinoma: a prospective study. PLoS ONE. (2013) 8:e81484. doi: 10.1371/journal.pone.0081484
63. Liu L, Xu Z, Zhong L, Wang H, Jiang S, Long Q, et al. Enhancer of zeste homolog 2 (EZH2) promotes tumour cell migration and invasion via epigenetic repression of E-cadherin in renal cell carcinoma. BJU Int. (2016) 117:351–62. doi: 10.1111/bju.12702
64. Wang Y, Chen Y, Geng H, Qi C, Liu Y, Yue D. Overexpression of YB1 and EZH2 are associated with cancer metastasis and poor prognosis in renal cell carcinomas. Tumour Biol. (2015) 36:7159–66. doi: 10.1007/s13277-015-3417-z
65. Ho TH, Kapur P, Eckel-Passow JE, Christie A, Joseph RW, Serie DJ, et al. Multicenter validation of enhancer of zeste homolog 2 expression as an independent prognostic marker in localized clear cell renal cell carcinoma. J Clin Oncol. (2017) 35:3706–13. doi: 10.1200/JCO.2017.73.3238
66. Wheatley SP, Altieri DC. Survivin at a glance. J Cell Sci. (2019) 132:jcs223826. doi: 10.1242/jcs.223826
67. Olivier M, Hollstein M, Hainaut P. TP53 mutations in human cancers: origins, consequences, and clinical use. Cold Spring Harb Perspect Biol. (2010) 2:a001008. doi: 10.1101/cshperspect.a001008
68. Parker AS, Cheville JC, Lohse CM, Igel T, Leibovich BC, Blute ML. Loss of expression of Von Hippel-Lindau tumor suppressor protein associated with improved survival in patients with early-stage clear cell renal cell carcinoma. Urology. (2005) 65:1090–5. doi: 10.1016/j.urology.2004.12.040
69. Schraml P, Hergovich A, Hatz F, Amin MB, Lim SD, Krek W, et al. Relevance of nuclear and cytoplasmic Von Hippel Lindau protein expression for renal carcinoma progression. Am J Pathol. (2003) 163:1013–20. doi: 10.1016/S0002-9440(10)63461-X
70. Di Cristofano C, Minervini A, Menicagli M, Salinitri G, Bertacca G, Pefanis G, et al. Nuclear expression of hypoxia-inducible factor-1alpha in clear cell renal cell carcinoma is involved in tumor progression. Am J Surg Pathol. (2007) 31:1875–81. doi: 10.1097/PAS.0b013e318094fed8
71. Högner A, Krause H, Jandrig B, Kasim M, Fuller TF, Schostak M, et al. PBRM1 and VHL expression correlate in human clear cell renal cell carcinoma with differential association with patient's overall survival. Urol Oncol. (2018) 36:94.e1–e14. doi: 10.1016/j.urolonc.2017.10.027
72. da Costa WH, da Cunha IW, Fares AF, Bezerra SM, Shultz L, Clavijo DA, et al. Prognostic impact of concomitant loss of PBRM1 and BAP1 protein expression in early stages of clear cell renal cell carcinoma. Urol Oncol. (2018) 36:24.e1–e8. doi: 10.1016/j.urolonc.2018.01.002
73. da Costa WH, Rezende M, Carneiro FC, Rocha RM, da Cunha IW, Carraro DM, et al. Polybromo-1 (PBRM1), a SWI/SNF complex subunit is a prognostic marker in clear cell renal cell carcinoma. BJU Int. (2014) 113:E157–63. doi: 10.1111/bju.12426
74. Jiang W, Dulaimi E, Devarajan K, Parsons T, Wang Q, O'Neill R, et al. Intratumoral heterogeneity analysis reveals hidden associations between protein expression losses and patient survival in clear cell renal cell carcinoma. Oncotarget. (2017) 8:37423–34. doi: 10.18632/oncotarget.16965
75. Nam SJ, Lee C, Park JH, Moon KC. Decreased PBRM1 expression predicts unfavorable prognosis in patients with clear cell renal cell carcinoma. Urol Oncol. (2015) 33:340.e9–16. doi: 10.1016/j.urolonc.2015.01.010
76. Campbell L, Gumbleton M, Griffiths DFR. Caveolin-1 overexpression predicts poor disease-free survival of patients with clinically confined renal cell carcinoma. Br J Cancer. (2003) 89:1909–13. doi: 10.1038/sj.bjc.6601359
77. Campbell L, Jasani B, Edwards K, Gumbleton M, Griffiths DFR. Combined expression of caveolin-1 and an activated AKT/mTOR pathway predicts reduced disease-free survival in clinically confined renal cell carcinoma. Br J Cancer. (2008) 98:931–40. doi: 10.1038/sj.bjc.6604243
78. Steffens S, Schrader AJ, Blasig H, Vetter G, Eggers H, Tränkenschuh W, et al. Caveolin 1 protein expression in renal cell carcinoma predicts survival. BMC Urol. (2011) 11:25. doi: 10.1186/1471-2490-11-25
79. Ruan H, Li X, Yang H, Song Z, Tong J, Cao Q, et al. Enhanced expression of caveolin-1 possesses diagnostic and prognostic value and promotes cell migration, invasion and sunitinib resistance in the clear cell renal cell carcinoma. Exp Cell Res. (2017) 358:269–78. doi: 10.1016/j.yexcr.2017.07.004
80. Sanchez DJ, Simon MC. Genetic and metabolic hallmarks of clear cell renal cell carcinoma. Biochim Biophys Acta Rev Cancer. (2018) 1870:23–31. doi: 10.1016/j.bbcan.2018.06.003
81. Hanahan D, Weinberg RA. Hallmarks of cancer: the next generation. Cell. (2011) 144:646–74. doi: 10.1016/j.cell.2011.02.013
82. Rini B, Goddard A, Knezevic D, Maddala T, Zhou M, Aydin H, et al. A 16-gene assay to predict recurrence after surgery in localised renal cell carcinoma: development and validation studies. Lancet Oncol. (2015) 16:676–85. doi: 10.1016/S1470-2045(15)70167-1
83. Büttner F, Winter S, Rausch S, Hennenlotter J, Kruck S, Stenzl A, et al. Clinical utility of the S3-score for molecular prediction of outcome in non-metastatic and metastatic clear cell renal cell carcinoma. BMC Med. (2018) 16:108. doi: 10.1186/s12916-018-1088-5
84. Zhang H, Liu Y, Xie H, Fu Q, Liu Z, Zhu Y, et al. Beta-1,4-galactosyltransferase II predicts poor prognosis of patients with non-metastatic clear-cell renal cell carcinoma. Tumour Biol. (2017) 39:1010428317691417. doi: 10.1177/1010428317691417
85. Xi W, Liu L, Wang J, Xia Y, Bai Q, Xiong Y, et al. Enrichment of C5a-C5aR axis predicts poor postoperative prognosis of patients with clear cell renal cell carcinoma. Oncotarget. (2016) 7:80925–34. doi: 10.18632/oncotarget.13108
86. Yang Y, Zhai C, Chang Y, Zhou L, Shi T, Tan C, et al. High expression of chemokine CCL2 is associated with recurrence after surgery in clear-cell renal cell carcinoma. Urol Oncol. (2016) 34:238.e19–26. doi: 10.1016/j.urolonc.2015.11.026
87. Wang Z, Xie H, Zhou L, Liu Z, Fu H, Zhu Y, et al. CCL2/CCR2 axis is associated with postoperative survival and recurrence of patients with non-metastatic clear-cell renal cell carcinoma. Oncotarget. (2016) 7:51525–34. doi: 10.18632/oncotarget.10492
88. Aaltomaa S, Lipponen P, Kärjä V, Lundstedt S, Lappi J, Kosma V-M. The expression and prognostic value of alpha-, beta- and gamma-catenins in renal cell carcinoma. Anticancer Res. (2004) 24:2407–13.
89. Krabbe L-M, Westerman ME, Bagrodia A, Gayed BA, Darwish OM, Haddad AQ, et al. Dysregulation of β-catenin is an independent predictor of oncologic outcomes in patients with clear cell renal cell carcinoma. J Urol. (2014) 191:1671–7. doi: 10.1016/j.juro.2013.11.052
90. Kovacs G, Billfeldt NK, Farkas N, Dergez T, Javorhazy A, Banyai D, et al. Cytoplasmic expression of β-catenin is an independent predictor of progression of conventional renal cell carcinoma: a simple immunostaining score. Histopathology. (2017) 70:273–80. doi: 10.1111/his.13059
91. Lian X, Duan X, Wu X, Li C, Chen S, Wang S, et al. Expression and clinical significance of Von Hippel-Lindau downstream genes: Jade-1 and β-catenin related to renal cell carcinoma. Urology. (2012) 80:485.e7–13. doi: 10.1016/j.urology.2012.02.024
92. Liu W, Liu Y, Fu Q, Zhou L, Chang Y, Xu L, et al. Elevated expression of IFN-inducible CXCR3 ligands predicts poor prognosis in patients with non-metastatic clear-cell renal cell carcinoma. Oncotarget. (2016) 7:13976–83. doi: 10.18632/oncotarget.7468
93. An H, Xu L, Chang Y, Zhu Y, Yang Y, Chen L, et al. CXC chemokine receptor 2 is associated with postoperative recurrence and survival of patients with non-metastatic clear-cell renal cell carcinoma. Eur J Cancer. (2015) 51:1953–61. doi: 10.1016/j.ejca.2015.06.125
94. Biswas S, Charlesworth PJS, Turner GDH, Leek R, Thamboo PT, Campo L, et al. CD31 angiogenesis and combined expression of HIF-1α and HIF-2α are prognostic in primary clear-cell renal cell carcinoma (CC-RCC), but HIFα transcriptional products are not: implications for antiangiogenic trials and HIFα biomarker studies in primary CC-RCC. Carcinogenesis. (2012) 33:1717–25. doi: 10.1093/carcin/bgs222
95. Brooks SA, Brannon AR, Parker JS, Fisher JC, Sen O, Kattan MW, et al. ClearCode34: a prognostic risk predictor for localized clear cell renal cell carcinoma. Eur Urol. (2014) 66:77–84. doi: 10.1016/j.eururo.2014.02.035
96. Wrzesiński T, Szelag M, Cieślikowski WA, Ida A, Giles R, Zodro E, et al. Expression of pre-selected TMEMs with predicted ER localization as potential classifiers of ccRCC tumors. BMC Cancer. (2015) 15:518. doi: 10.1186/s12885-015-1530-4
97. Lidgren A, Hedberg Y, Grankvist K, Rasmuson T, Vasko J, Ljungberg B. The expression of hypoxia-inducible factor 1alpha is a favorable independent prognostic factor in renal cell carcinoma. Clin Cancer Res. (2005) 11:1129–35.
98. Schultz L, Chaux A, Albadine R, Hicks J, Kim JJ, De Marzo AM, et al. Immunoexpression status and prognostic value of mTOR and hypoxia-induced pathway members in primary and metastatic clear cell renal cell carcinomas. Am J Surg Pathol. (2011) 35:1549–56. doi: 10.1097/PAS.0b013e31822895e5
99. Ren J, Liu H, Yan L, Tian S, Li D, Xu Z. Microvessel density and heparanase over-expression in clear cell renal cell cancer: correlations and prognostic significances. World J Surg Oncol. (2011) 9:158. doi: 10.1186/1477-7819-9-158
100. Mikami S, Oya M, Shimoda M, Mizuno R, Ishida M, Kosaka T, et al. Expression of heparanase in renal cell carcinomas: implications for tumor invasion and prognosis. Clin Cancer Res. (2008) 14:6055–61. doi: 10.1158/1078-0432.CCR-08-0750
101. Wu K, Xu L, Zhang L, Lin Z, Hou J. High Jagged1 expression predicts poor outcome in clear cell renal cell carcinoma. Jpn J Clin Oncol. (2011) 41:411–6. doi: 10.1093/jjco/hyq205
102. Liu Z, Fu Q, Fu H, Wang Z, Xu L, An H, et al. A three-molecule score based on Notch pathway predicts poor prognosis in non-metastasis clear cell renal cell carcinoma. Oncotarget. (2016) 7:68559–70. doi: 10.18632/oncotarget.11849
103. Fukata S, Inoue K, Kamada M, Kawada C, Furihata M, Ohtsuki Y, et al. Levels of angiogenesis and expression of angiogenesis-related genes are prognostic for organ-specific metastasis of renal cell carcinoma. Cancer. (2005) 103:931–42. doi: 10.1002/cncr.20887
104. An H, Zhu Y, Xu L, Chen L, Lin Z, Xu J. Notch1 predicts recurrence and survival of patients with clear-cell renal cell carcinoma after surgical resection. Urology. (2015) 85:483.e9–e14. doi: 10.1016/j.urology.2014.10.022
105. Hu J, Zhang L-C, Song X, Lu J-R, Jin Z. KRT6 interacting with notch1 contributes to progression of renal cell carcinoma, and aliskiren inhibits renal carcinoma cell lines proliferation in vitro. Int J Clin Exp Pathol. (2015) 8:9182–8.
106. Shim M, Song C, Park S, Choi S-K, Cho YM, Kim C-S, et al. Prognostic significance of platelet-derived growth factor receptor-β expression in localized clear cell renal cell carcinoma. J Cancer Res Clin Oncol. (2015) 141:2213–20. doi: 10.1007/s00432-015-2019-x
107. Frödin M, Mezheyeuski A, Corvigno S, Harmenberg U, Sandström P, Egevad L, et al. Perivascular PDGFR-β is an independent marker for prognosis in renal cell carcinoma. Br J Cancer. (2017) 116:195–201. doi: 10.1038/bjc.2016.407
108. Pantuck AJ, Seligson DB, Klatte T, Yu H, Leppert JT, Moore L, et al. Prognostic relevance of the mTOR pathway in renal cell carcinoma: implications for molecular patient selection for targeted therapy. Cancer. (2007) 109:2257–67. doi: 10.1002/cncr.22677
109. Zhu C, Wei J, Tian X, Li Y, Li X. Prognostic role of PPAR-γ and PTEN in the renal cell carcinoma. Int J Clin Exp Pathol. (2015) 8:12668–77.
110. Dai J, Lu Y, Wang J, Yang L, Han Y, Wang Y, et al. A four-gene signature predicts survival in clear-cell renal-cell carcinoma. Oncotarget. (2016) 7:82712–26. doi: 10.18632/oncotarget.12631
111. Ohba K, Miyata Y, Kanda S, Koga S, Hayashi T, Kanetake H. Expression of urokinase-type plasminogen activator, urokinase-type plasminogen activator receptor and plasminogen activator inhibitors in patients with renal cell carcinoma: correlation with tumor associated macrophage and prognosis. J Urol. (2005) 174:461–5. doi: 10.1097/01.ju.0000165150.46006.92
112. Zubac DP, Wentzel-Larsen T, Seidal T, Bostad L. Type 1 plasminogen activator inhibitor (PAI-1) in clear cell renal cell carcinoma (CCRCC) and its impact on angiogenesis, progression and patient survival after radical nephrectomy. BMC Urol. (2010) 10:20. doi: 10.1186/1471-2490-10-20
113. Fuessel S, Erdmann K, Taubert H, Lohse-Fischer A, Zastrow S, Meinhardt M, et al. Prognostic impact of urokinase-type plasminogen activator system components in clear cell renal cell carcinoma patients without distant metastasis. BMC Cancer. (2014) 14:974. doi: 10.1186/1471-2407-14-974
114. Liu W, Fu Q, An H, Chang Y, Zhang W, Zhu Y, et al. Decreased expression of SETD2 predicts unfavorable prognosis in patients with nonmetastatic clear-cell renal cell carcinoma. Medicine. (2015) 94:e2004. doi: 10.1097/MD.0000000000002004
115. Liu L, Guo R, Zhang X, Liang Y, Kong F, Wang J, et al. Loss of SETD2, but not H3K36me3, correlates with aggressive clinicopathological features of clear cell renal cell carcinoma patients. Biosci Trends. (2017) 11:214–20. doi: 10.5582/bst.2016.01228
116. Miyajima A, Asano T, Seta K, Asano T, Kakoi N, Hayakawa M. Loss of expression of transforming growth factor-beta receptor as a prognostic factor in patients with renal cell carcinoma. Urology. (2003) 61:1072–7. doi: 10.1016/S0090-4295(02)02553-0
117. Parker AS, Lohse CM, Wu K, Kreinest P, Copland JA, Hilton T, et al. Lower expression levels of the transforming growth factor beta receptor type II protein are associated with a less aggressive tumor phenotype and improved survival among patients with clear cell renal cell carcinoma. Hum Pathol. (2007) 38:453–61. doi: 10.1016/j.humpath.2006.08.029
118. Kawashima A, Kanazawa T, Goto K, Matsumoto M, Morimoto-Okazawa A, Iwahori K, et al. Immunological classification of renal cell carcinoma patients based on phenotypic analysis of immune check-point molecules. Cancer Immunol. Immunother. (2018) 67:113–25. doi: 10.1007/s00262-017-2060-5
119. Kanomata N, Sato Y, Miyaji Y, Nagai A, Moriya T. Vasohibin-1 is a new predictor of disease-free survival in operated patients with renal cell carcinoma. J Clin Pathol. (2013) 66:613–9. doi: 10.1136/jclinpath-2013-201444
120. Yildiz E, Gokce G, Kilicarslan H, Ayan S, Goze OF, Gultekin EY. Prognostic value of the expression of Ki-67, CD44 and vascular endothelial growth factor, and microvessel invasion, in renal cell carcinoma. BJU Int. (2004) 93:1087–93. doi: 10.1111/j.1464-410X.2004.04786.x
121. Liang Y-X, He H-C, Han Z-D, Bi X-C, Dai Q-S, Ye Y-K, et al. CD147 and VEGF expression in advanced renal cell carcinoma and their prognostic value. Cancer Invest. (2009) 27:788–93. doi: 10.1080/07357900802709167
122. Alexandrov LB, Nik-Zainal S, Wedge DC, Campbell PJ, Stratton MR. Deciphering signatures of mutational processes operative in human cancer. Cell Rep. (2013) 3:246–59. doi: 10.1016/j.celrep.2012.12.008
123. Jiang T, Zhou C, Ren S. Role of IL-2 in cancer immunotherapy. Oncoimmunology. (2016) 5:e1163462. doi: 10.1080/2162402X.2016.1163462
124. Nishino M, Ramaiya NH, Hatabu H, Hodi FS. Monitoring immune-checkpoint blockade: response evaluation and biomarker development. Nat Rev Clin Oncol. (2017) 14:655–68. doi: 10.1038/nrclinonc.2017.88
125. Li P, Ren H, Zhang Y, Zhou Z. Fifteen-gene expression based model predicts the survival of clear cell renal cell carcinoma. Medicine. (2018) 97:e11839. doi: 10.1097/MD.0000000000011839
126. Itoi T, Yamana K, Bilim V, Takahashi K, Tomita F. Impact of frequent Bcl-2 expression on better prognosis in renal cell carcinoma patients. Br J Cancer. (2004) 90:200–5. doi: 10.1038/sj.bjc.6601454
127. Tan W, Hildebrandt MAT, Pu X, Huang M, Lin J, Matin SF, et al. Role of inflammatory related gene expression in clear cell renal cell carcinoma development and clinical outcomes. J Urol. (2011) 186:2071–7. doi: 10.1016/j.juro.2011.06.049
128. Thompson RH, Gillett MD, Cheville JC, Lohse CM, Dong H, Webster WS, et al. Costimulatory B7-H1 in renal cell carcinoma patients: indicator of tumor aggressiveness and potential therapeutic target. Proc Natl Acad Sci USA. (2004) 101:17174–9. doi: 10.1073/pnas.0406351101
129. Thompson RH, Kuntz SM, Leibovich BC, Dong H, Lohse CM, Webster WS, et al. Tumor B7-H1 is associated with poor prognosis in renal cell carcinoma patients with long-term follow-up. Cancer Res. (2006) 66:3381–5. doi: 10.1158/0008-5472.CAN-05-4303
130. Parker AS, Leibovich BC, Lohse CM, Sheinin Y, Kuntz SM, Eckel-Passow JE, et al. Development and evaluation of BioScore: a biomarker panel to enhance prognostic algorithms for clear cell renal cell carcinoma. Cancer. (2009) 115:2092–103. doi: 10.1002/cncr.24263
131. Bromwich EJ, McArdle PA, Canna K, McMillan DC, McNicol A-M, Brown M, et al. The relationship between T-lymphocyte infiltration, stage, tumour grade and survival in patients undergoing curative surgery for renal cell cancer. Br. J. Cancer. (2003) 89:1906–8. doi: 10.1038/sj.bjc.6601400
132. Siddiqui SA, Frigola X, Bonne-Annee S, Mercader M, Kuntz SM, Krambeck AE, et al. Tumor-infiltrating Foxp3-CD4+CD25+ T cells predict poor survival in renal cell carcinoma. Clin Cancer Res. (2007) 13:2075–81. doi: 10.1158/1078-0432.CCR-06-2139
133. Lim SD, Young AN, Paner GP, Amin MB. Prognostic role of CD44 cell adhesion molecule expression in primary and metastatic renal cell carcinoma: a clinicopathologic study of 125 cases. Virchows Arch Int J Pathol. (2008) 452:49–55. doi: 10.1007/s00428-007-0530-4
134. Zhang X, Yang M, Shi H, Hu J, Wang Y, Sun Z, et al. Reduced E-cadherin facilitates renal cell carcinoma progression by WNT/β-catenin signaling activation. Oncotarget. (2017) 8:19566–76. doi: 10.18632/oncotarget.15361
135. Mlcochova H, Machackova T, Rabien A, Radova L, Fabian P, Iliev R, et al. Epithelial-mesenchymal transition-associated microRNA/mRNA signature is linked to metastasis and prognosis in clear-cell renal cell carcinoma. Sci Rep. (2016) 6:31852. doi: 10.1038/srep31852
136. Yang C-A, Huang H-Y, Yen J-C, Chang J-G. Prognostic value of RNASEH2A-, CDK1-, and CD151-related pathway gene profiling for kidney cancers. Int J Mol Sci. (2018) 19:1586. doi: 10.3390/ijms19061586
137. Wang C, Tang K, Li Z, Chen Z, Xu H, Ye Z. Targeted p21(WAF1/CIP1) activation by miR-1236 inhibits cell proliferation and correlates with favorable survival in renal cell carcinoma. Urol Oncol. (2016) 34:59.e23–34. doi: 10.1016/j.urolonc.2015.08.014
138. Pertia A, Nikoleishvili D, Trsintsadze O, Gogokhia N, Managadze L, Chkhotua A. Immunoreactivity of p27: cyclin D3, and Ki67 in conventional renal cell carcinoma. Int Urol Nephrol. (2009) 41:243–9. doi: 10.1007/s11255-008-9442-8
139. Pertia A, Nikoleishvili D, Trsintsadze O, Gogokhia N, Managadze L, Chkhotua A. Loss of p27(Kip1) CDKI is a predictor of poor recurrence-free and cancer-specific survival in patients with renal cancer. Int Urol Nephrol. (2007) 39:381–7. doi: 10.1007/s11255-006-9077-6
140. Liu Z, Fu Q, Lv J, Wang F, Ding K. Prognostic implication of p27Kip1, Skp2 and Cks1 expression in renal cell carcinoma: a tissue microarray study. J Exp Clin Cancer Res. (2008) 27:51. doi: 10.1186/1756-9966-27-51
141. Sgambato A, Camerini A, Genovese G, De Luca F, Viacava P, Migaldi M, et al. Loss of nuclear p27(kip1) and α-dystroglycan is a frequent event and is a strong predictor of poor outcome in renal cell carcinoma. Cancer Sci. (2010) 101:2080–6. doi: 10.1111/j.1349-7006.2010.01644.x
142. Rabjerg M, Bjerregaard H, Halekoh U, Jensen BL, Walter S, Marcussen N. Molecular characterization of clear cell renal cell carcinoma identifies CSNK2A1, SPP1 and DEFB1 as promising novel prognostic markers. APMIS. (2016) 124:372–83. doi: 10.1111/apm.12519
143. Giraldo NA, Becht E, Vano Y, Petitprez F, Lacroix L, Validire P, et al. Tumor-infiltrating and peripheral blood T-cell immunophenotypes predict early relapse in localized clear cell renal cell carcinoma. Clin Cancer Res. (2017) 23:4416–28. doi: 10.1158/1078-0432.CCR-16-2848
144. Hildebrandt MAT, Tan W, Tamboli P, Huang M, Ye Y, Lin J, et al. Kinome expression profiling identifies IKBKE as a predictor of overall survival in clear cell renal cell carcinoma patients. Carcinogenesis. (2012) 33:799–803. doi: 10.1093/carcin/bgs018
145. Bamias A, Chorti M, Deliveliotis C, Trakas N, Skolarikos A, Protogerou B, et al. Prognostic significance of CA 125, CD44, and epithelial membrane antigen in renal cell carcinoma. Urology. (2003) 62:368–73. doi: 10.1016/S0090-4295(03)00264-4
146. Zhu Y, Xu L, An H, Liu W, Wang Z, Xu J. p21-activated kinase 1 predicts recurrence and survival in patients with non-metastatic clear cell renal cell carcinoma. Int J Urol. (2015) 22:447–53. doi: 10.1111/iju.12715
147. Hedberg Y, Ljungberg B, Roos G, Landberg G. Expression of cyclin D1, D3, E, and p27 in human renal cell carcinoma analysed by tissue microarray. Br J Cancer. (2003) 88:1417–23. doi: 10.1038/sj.bjc.6600922
148. Wu XR, Sha JJ, Liu DM, Chen YH, Yang GL, Zhang J, et al. High expression of P53-induced Ring-h2 protein is associated with poor prognosis in clear cell renal cell carcinoma. Eur J Surg Oncol. (2013) 39:100–6. doi: 10.1016/j.ejso.2012.10.004
149. Yao M, Huang Y, Shioi K, Hattori K, Murakami T, Sano F, et al. A three-gene expression signature model to predict clinical outcome of clear cell renal carcinoma. Int J Cancer. (2008) 123:1126–32. doi: 10.1002/ijc.23641
150. Williams GH, Stoeber K. The cell cycle and cancer. J Pathol. (2012) 226:352–64. doi: 10.1002/path.3022
151. Otto T, Sicinski P. Cell cycle proteins as promising targets in cancer therapy. Nat Rev Cancer. (2017) 17:93–115. doi: 10.1038/nrc.2016.138
152. Ikuerowo SO, Kuczyk MA, von Wasielewski R, Shittu OB, Jonas U, Machtens S, et al. p16INK4a expression and clinicopathologic parameters in renal cell carcinoma. Eur Urol. (2007) 51:732–7; discussion: 738. doi: 10.1016/j.eururo.2006.08.010
153. Joseph RW, Kapur P, Serie DJ, Eckel-Passow JE, Parasramka M, Ho T, et al. Loss of BAP1 protein expression is an independent marker of poor prognosis in patients with low-risk clear cell renal cell carcinoma. Cancer. (2014) 120:1059–67. doi: 10.1002/cncr.28521
154. Minardi D, Lucarini G, Milanese G, Di Primio R, Montironi R, Muzzonigro G. Loss of nuclear BAP1 protein expression is a marker of poor prognosis in patients with clear cell renal cell carcinoma. Urol Oncol. (2016) 34:338.e11–18. doi: 10.1016/j.urolonc.2016.03.006
155. Chang P, Bing Z, Tian J, Zhang J, Li X, Ge L, et al. Comprehensive assessment gene signatures for clear cell renal cell carcinoma prognosis. Medicine. (2018) 97:e12679. doi: 10.1097/MD.0000000000012679
156. Cao Y-W, Liu Y, Dong Z, Guo L, Kang E-H, Wang Y-H, et al. Monocarboxylate transporters MCT1 and MCT4 are independent prognostic biomarkers for the survival of patients with clear cell renal cell carcinoma and those receiving therapy targeting angiogenesis. Urol Oncol. (2018) 36:311.e15–25. doi: 10.1016/j.urolonc.2018.03.014
157. Brannon AR, Reddy A, Seiler M, Arreola A, Moore DT, Pruthi RS, et al. Molecular stratification of clear cell renal cell carcinoma by consensus clustering reveals distinct subtypes and survival patterns. Genes Cancer. (2010) 1:152–63. doi: 10.1177/1947601909359929
158. Beuselinck B, Job S, Becht E, Karadimou A, Verkarre V, Couchy G, et al. Molecular subtypes of clear cell renal cell carcinoma are associated with sunitinib response in the metastatic setting. Clin Cancer Res. (2015) 21:1329–39. doi: 10.1158/1078-0432.CCR-14-1128
159. DeLuca C, Kwon H, Lin R, Wainberg M, Hiscott J. NF-kappaB activation and HIV-1 induced apoptosis. Cytokine Growth Factor Rev. (1999) 10:235–53. doi: 10.1016/S1359-6101(99)00015-5
160. Kumari N, Dwarakanath BS, Das A, Bhatt AN. Role of interleukin-6 in cancer progression and therapeutic resistance. Tumour Biol. (2016) 37:11553–72. doi: 10.1007/s13277-016-5098-7
161. Roumenina LT, Daugan MV, Noe R, Petitprez F, Vano YA, Sanchez-Salas R, et al. Tumor cells hijack macrophage-produced complement C1q to promote tumor growth. Cancer Immunol Res. (2019) 7:1091–105. doi: 10.1158/2326-6066.CIR-18-0891
162. Litwiniuk M, Krejner A, Speyrer MS, Gauto AR, Grzela T. Hyaluronic acid in inflammation and tissue regeneration. Wounds Compend Clin Res Pract. (2016) 28:78–88.
163. Murata M. Inflammation and cancer. Environ Health Prev Med. (2018) 23:50. doi: 10.1186/s12199-018-0740-1
164. Kim BJ, Kim JH, Kim HS, Zang DY. Prognostic and predictive value of VHL gene alteration in renal cell carcinoma: a meta-analysis and review. Oncotarget. (2017) 8:13979–85. doi: 10.18632/oncotarget.14704
165. Joseph RW, Kapur P, Serie DJ, Parasramka M, Ho TH, Cheville JC, et al. Clear cell renal cell carcinoma subtypes identified by BAP1 and PBRM1 expression. J Urol. (2016) 195:180–7. doi: 10.1016/j.juro.2015.07.113
166. van Oostenbrugge TJ, Fütterer JJ, Mulders PFA. Diagnostic imaging for solid renal tumors: a pictorial review. Kidney Cancer. (2018) 2:79–93. doi: 10.3233/KCA-180028
167. Vano Y, Elaidi RT, Bennamoun M, Chevreau CM, Borchiellini D, Pannier D, et al. LBA25 Results from the phase II biomarker driven trial with nivolumab (N) and ipilimumab or VEGFR tyrosine kinase inhibitor (TKI) in naïve metastatic kidney cancer (m-ccRCC) patients (pts): the BIONIKK trial. Ann Oncol. (2020) 31:S1157. doi: 10.1016/j.annonc.2020.08.2254
168. Joosten SC, Deckers IA, Aarts MJ, Hoeben A, van Roermund JG, Smits KM, et al. Prognostic DNA methylation markers for renal cell carcinoma: a systematic review. Epigenomics. (2017) 9:1243–57. doi: 10.2217/epi-2017-0040
Keywords: prognostic markers, clear cell renal cell carcinoma (ccRCC), multivariate analysis, independent datasets, cox models
Citation: Petitprez F, Ayadi M, de Reyniès A, Fridman WH, Sautès-Fridman C and Job S (2021) Review of Prognostic Expression Markers for Clear Cell Renal Cell Carcinoma. Front. Oncol. 11:643065. doi: 10.3389/fonc.2021.643065
Received: 17 December 2020; Accepted: 17 February 2021;
Published: 28 April 2021.
Edited by:
Elena Ranieri, University of Foggia, ItalyReviewed by:
Philipp Ivanyi, Hannover Medical School, GermanyCopyright © 2021 Petitprez, Ayadi, de Reyniès, Fridman, Sautès-Fridman and Job. This is an open-access article distributed under the terms of the Creative Commons Attribution License (CC BY). The use, distribution or reproduction in other forums is permitted, provided the original author(s) and the copyright owner(s) are credited and that the original publication in this journal is cited, in accordance with accepted academic practice. No use, distribution or reproduction is permitted which does not comply with these terms.
*Correspondence: Catherine Sautès-Fridman, Y2F0aGVyaW5lLmZyaWRtYW5AY3JjLmp1c3NpZXUuZnI=; Sylvie Job, c3lsdmllLmpvYkBpbnNlcm0uZnI=
†These authors share last authorship
Disclaimer: All claims expressed in this article are solely those of the authors and do not necessarily represent those of their affiliated organizations, or those of the publisher, the editors and the reviewers. Any product that may be evaluated in this article or claim that may be made by its manufacturer is not guaranteed or endorsed by the publisher.
Research integrity at Frontiers
Learn more about the work of our research integrity team to safeguard the quality of each article we publish.