- 1Center for Clinical Precision Pharmacy, The First Affiliated Hospital of Guangdong Pharmaceutical University, Guangzhou, China
- 2School of Clinical Pharmacy, Guangdong Pharmaceutical University, Guangzhou, China
- 3Department of Biomedical Science, City University of Hong Kong, Hong Kong, Hong Kong, SAR China
- 4Department of Colorectal Surgery, The Affiliated TCM Hospital of Guangzhou Medical University, Guangzhou, China
- 5School of Clinical Integrative Chinese and Western Medicine, Guangzhou Medical University, Guangzhou, China
- 6Department of Surgery, Maoming Hospital of Traditional Chinese Medicine, Maoming, China
- 7Department of Electrical Engineering, City University of Hong Kong, Hong Kong, Hong Kong, SAR China
Pelvic cancer radiotherapy may cause chronic radiation proctitis (CRP) that adversely affects patient’s quality of life, especially in patients with prolonged hematochezia. However, previous studies of radiation enteropathy mainly focused on acute irradiation hazards, and the detailed pathogenesis process and mechanism of prolonged hematochezia associated with radiation-induced toxicity remain unclear. In this study, we characterized the gut microbiota of 32 female CRP patients with or without hematochezia. Differential patterns of dysbiosis were observed. The abundance of Peptostreptococcaceae, Eubacterium, and Allisonella was significantly higher in CRP patients with hematochezia, while the compositions of the Lachnospiraceae, Megasphera, Megamonas, and Ruminococcaceae were lower in the microbiota of non-hematochezia patients. Functional prediction suggested significant difference in the expression of mineral absorption and the arachidonic acid metabolism proteins between hematochezia and non-hematochezia patients, possibly interdependent on radiation-induced inflammation. This study provides new insight into the altered composition and function of gut microbiota in patients with hematochezia, implying the potential use of probiotics and prebiotics for assessment and treatment of CRP.
Introduction
Pelvic radiotherapy is one of the most important treatments of gynecologic tumors. Chronic radiation proctitis (CRP) is a well-known complication of pelvic radiotherapy that occurs in 5% to 20% of the cancer survivors 6 months to several years later (1). Unlike acute radiation enteropathy (RE), which presents with acute diarrhea and requires immediate termination of radiotherapy (2), CRP commonly manifests hematochezia, which adversely affects patient’s quality of life in the long term. Prolonged, recurrent rectal inflammatory and bleeding lead to weakness, anemia, and a series of mental effects including anxiety and depression, especially in female patients (3, 4). Current treatments of CRP including high doses of 5-amin-osalicylic acid enemas, endoscopic thermal formalin therapy, and argon plasma coagulation rely on medical equipment and professional physicians (5–7). Development of daily oral drugs for improving CRP symptoms with better compliance are under the spotlight (8).
With the deepening understanding of intestinal homeostasis, prebiotics and probiotics have been proposed to be the future treatment option of an array of chronic diseases. The significance of the liver–microbiome axis has been recognized as a major modulator of liver diseases (9, 10). Also, gut microbial dysbiosis has been reported to play crucial roles in a series of gastrointestinal disorders including inflammatory bowel diseases (IBD), irritable bowel syndrome (IBS), and RE. A multi-omics study of the gut microbial ecosystem described both host and microbial activities with characteristic taxonomic that caused functional and biochemical shifts in IBD (11). DNA fingerprinting and cloning-sequencing techniques revealed that acute postradiotherapy diarrhea might be associated with patient’s initial microbial composition (12). Higher counts of Clostridium IV, Roseburia, and Phascolarctobacterium are significantly associated with RE (13). Lactobacillus rhamnosus GG (LGG) protection of intestinal epithelium from radiation injury was illustrated by a rodent model. The LGG-mediated radioprotection is reported to be dependent on MyD88, TLR-2, and COX-2 (14). However, little is known about the interaction between gut microbiota and prolonged proctorrhagia after irradiation.
In the present study, we set out to characterize the gut microbiota profiles of CRP patients and demonstrated the specific dysbiosis in CRP patients with hematochezia.
Materials and Methods
Ethics Statement and Patient Information
This study was approved by the Ethics Board at Guangzhou Hospital of TCM. Samples were collected from 32 female outpatients under a proctoscope. Informed consent was obtained from all subjects. The patients received pelvic radiotherapy 1 to 2 years prior to the onset of chronic radiation proctitis. No antibiotic has been adopted by any patient for at least 3 months. Thirteen patients complained of hematochezia for at least three times per day, and bleeding was observed under a proctoscope. These patients were classified as the H group. Sixteen patients who complained of diarrhea with no or little blood in stool were classified as the NH group (Table 1). Samples from three healthy volunteers were collected in parallel. Vegetarian, patients complicated with irritable bowel disease or genetic hemorrhagic disease, and patients who have received antibiotics or steroid treatment in the past 3 months were excluded from this study.
DNA Extraction
Total DNA was extracted from a 0.25-g sample by a QIAamp PowerFecal DNA Kit (QIAGEN, DE). The DNA concentration and purity were determined by Multiskan™ GO (Thermo Fisher Scientific, US), and the integrity of the DNA was determined by agarose gel electrophoresis.
16S rRNA Gene Sequencing
PCR was performed on the V4 region (515F-806R) of the 16S rRNA gene of the sample bacteria by priming different indexes at both ends. The reaction system of PCR was performed using 10 μl of KAPA HiFi HotStart ReadyMix (KAPA Biosystems, USA), 2 μl of DNA (30 ng/μl), and 1 μl of forward and reverse primer (10 μM). The forward primer of 16S was 5’-gtgccagcmgccgcggtaa-3’ and the reverse primer was 5’-ggactacnvgggtwtctaat-3’. The following thermal cycling program was used: an initial denaturation step at 95°C for 3 min followed by 30 cycles of 95°C for 20 s, 60°C for 30 s (annealing/synthesis step), 72°C for 30 s (extension), and finally 72°C for a 10-min extension (9, 12, 15). The PCR products were purified with the AxyPrep™ PCR Cleanup Kit (Axygen, USA), and the concentration was determined with Qubit 3.0 (Thermo Fisher Scientific, USA). Equal amounts of each sample were mixed together to form a sequencing library. QSEP100 (Bioptic, CHN) and ABI7300 quantitative PCR (Thermo Fisher Scientific, USA) were used to detect and quantify the insertion fragment and concentration of the library, respectively. The tested libraries were Paired-End 150 bp (PE150) sequenced using the Illumina MiniSeq platform (16).
Bioinformatic Analysis
Sequences generated from Illumina sequencing were analyzed with MOTHUR (version 1.39.5) for data cleaning and chimera removal, identification of operational taxonomic units (OTU), taxonomic assignment, and community comparison by adapting its standard operational procedure (17). Sequences were realigned with the SILVA-compatible alignment database (http://www.mothur.org/w/images/9/98/Silva.bacteria.zip). The 3% dissimilarity cutoff value was used for assigning an OTU. Shannon’s diversity, Simpson’s diversity, ACE, and Chao I richness indices were generated with the MOTHUR program. Output matrixes were further analyzed by principal coordinates analysis (PcoA) based on UniFrac distance (18, 19) and linear discriminant analysis (LDA) effect size (LEfSe) to identify differences in relative abundance at different taxonomic levels (20). Gene function and metabolic pathway prediction was conducted by PICRUSt (19).
Statistics and Data Visualization
The analysis of variance (ANOVA) test was used to assess the differences in the similarity index and the number of bands among the three groups: controls, patients with hematochezia, and patients without hematochezia. The Mann–Whitney U test was used when we analyzed difference between two groups using GraphPad prism 8 (21). Krona was used for visualization of microbiome community (22).
Results
Patients and Sample Collection
Thirty-two female participants were assigned into two groups: group NH, meaning chronic radiative proctitis (CRP) patients with no or mild hematochezia (hematochezia frequency < twice per week and/or no fresh blood observed under proctoscope, n = 16); and group H, meaning CRP patients with hematochezia (hematochezia frequency > twice per week and/or fresh blood was found under proctoscope, n = 13) (Table 1). Three patients were excluded as they presented with rectal ulcers (diameter > 1 mm) and/or symptoms of bacterial infection. The age of the 29 patients ranged from 28 to 71 years, and no significant difference was found between the two groups. Patients have received a total dose of 48 to 54 Gy irradiation as for radiotherapy of uterus or cervical carcinoma but have not received antibiotic treatment for at least 3 months. Frequency of uterus or cervix cancer was similar between two groups. Three samples from healthy adults were collected and analyzed in parallel.
α- Diversity of Gut Microbiota in CRP Patients
To investigate the ecological complexity of the gut microbiota of CRP patients, α-diversity analysis within each group was conducted using Community richness calculators Chao1 estimator and ACE estimator as well as Community diversity calculators the Simpson index and the Shannon index in MOTHUR. As shown in Figure 1, the Community richness in CRP patients with hematochezia (Mean of Chao1 = 1065; Mean of ACE = 1738) as compared with CRP patients without hematochezia (Mean of Chao1 = 943.0; Mean of ACE = 1,488) was not statistically different (p > 0.1). Neither Community richness in healthy volunteer was distinguished (Supplementary Figure S1). This result suggests that the overall complexity of gut microbiota may not be a sensitive indicator for CRP.
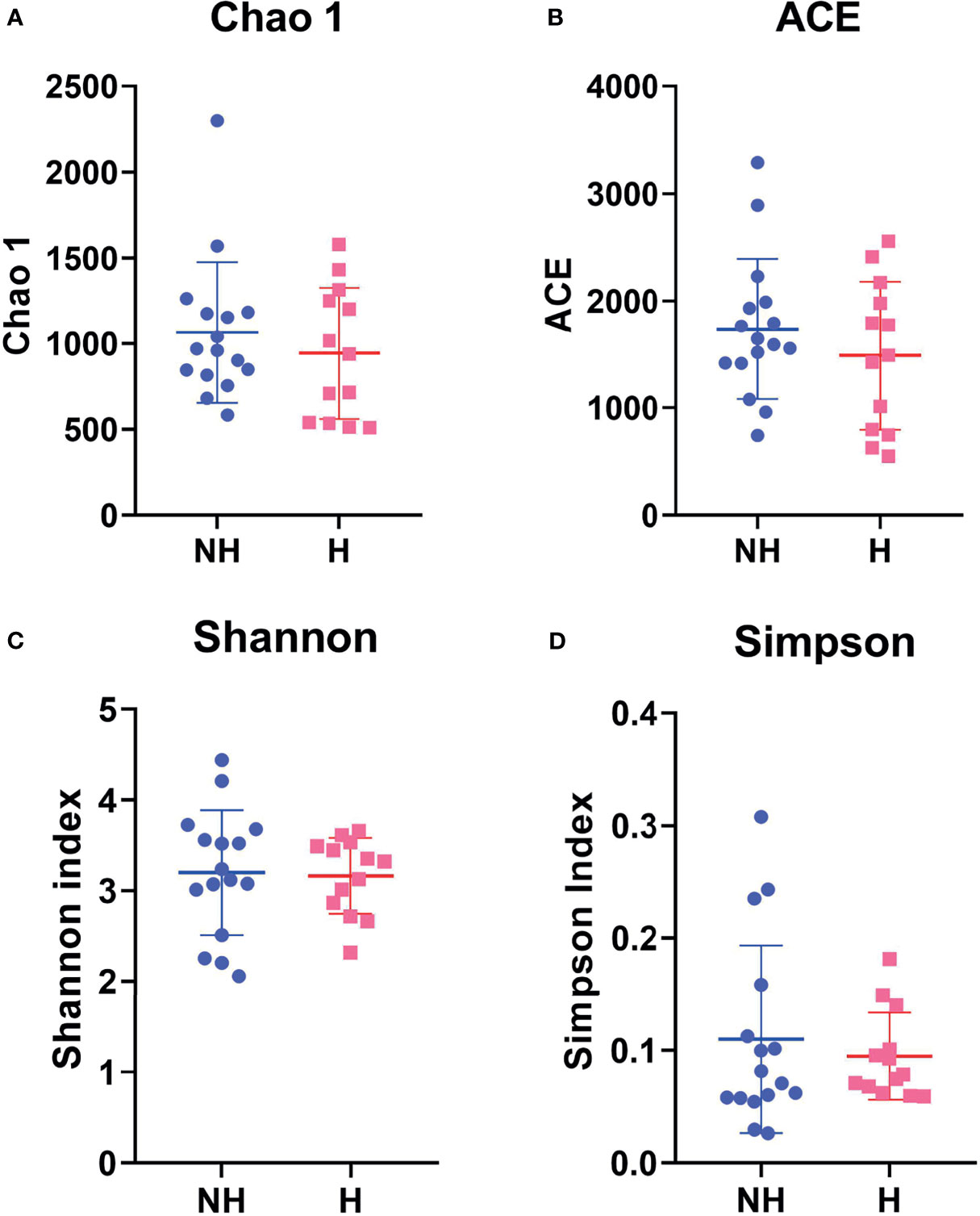
Figure 1 Comparative α-diversity analysis by (A) Chao1, (B) ACE estimator, (C) Shannon, and (D) Simpson Index indicating microbiome differences between non-hematochezia (NH) and hematochezia (H) subjects. Error bars indicate SEM.
Altered β-diversity of Gut Microbiota in CRP Patients
The 16S rRNA gene amplicon data contained 1,005 OTUs, 133 families, and 342 genera. Venn diagram was generated to illustrate the assigned OTUs in each group. About 70% of the OTUs were shared in CRP patients while 27% and 29% of the taxonomic units were unique in the H group and NH group, respectively (Figure 2A). Ordination analysis including Principal Component Analysis (PCA), Principal Co-ordinates Analysis (PCoA), and Non-Metric Multi-Dimensional Scaling (NMDS) were conducted to measure the difference in bacterial community composition in two dimensions. The first principal component (PC1) of PCA and PCoA largely distinguished changes among healthy controls and CRP patients with hematochezia (Figure 2B and Supplementary Figure S2). Inter-individual variation accounted for part of the variance, which was consistent with previous studies (9, 11). In addition, the heatmaps demonstrate differential composition of microbiota in three levels (Supplementary Figure S3). The β-diversity of gut microbiota in CRP patients suggests that a proportion of bacteria population is related to hematochezia.
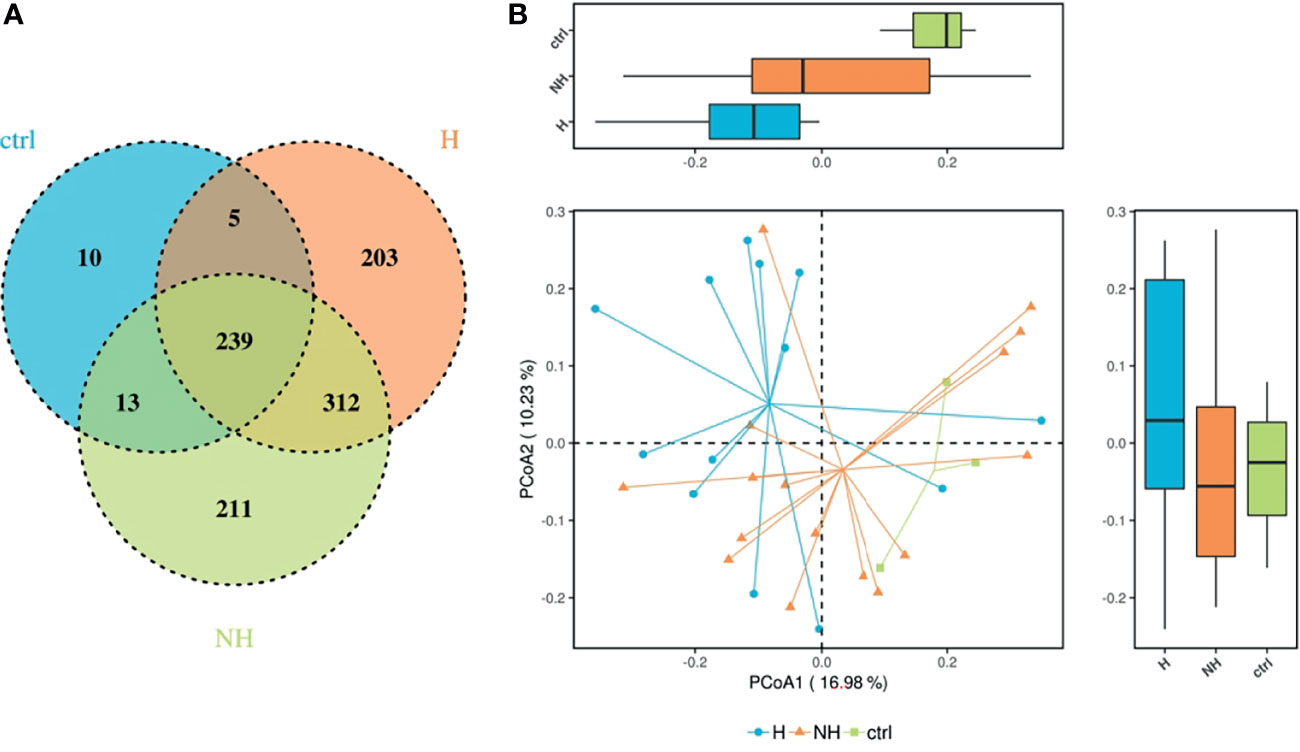
Figure 2 Differential composition of gut microbiome in CRP patients. (A) Venn diagram representing the operational taxonomic units (OTUs) in samples obtained in healthy volunteers (crtl), CRP patients with hematochezia (H), and those without hematochezia (NH). (B) PCoA based on UniFrac matrix showing the differential microbiota composition. Boxes represent the 25th to 75th percentile of the distribution; the median is shown as a thick line in the box.
Different Bacterial Taxa Between the Hematochezia and Non-Hematochezia Group
To further study the effect of microbiota composition on CPR symptom, the OTU matrixes were clustered into three cohorts. Hematochezia and non-hematochezia groups were isolated and visualized by Krona (Supplementary Figure S4). Taxonomy of the filtered bacteria with more than 2% of total abundance is shown in Figure 3. Changed compositions of more than twofold were observed in Fusobacteriales and Selenomonadales orders; Peptostreptococcaceae, Fusobacteriaceae, Verrucomicrobiaceae, Prevotellaceace, and Veilonelaceae families; and Peptostreptococcus, Akkermansia, Prevotella, and Megamonas genera.
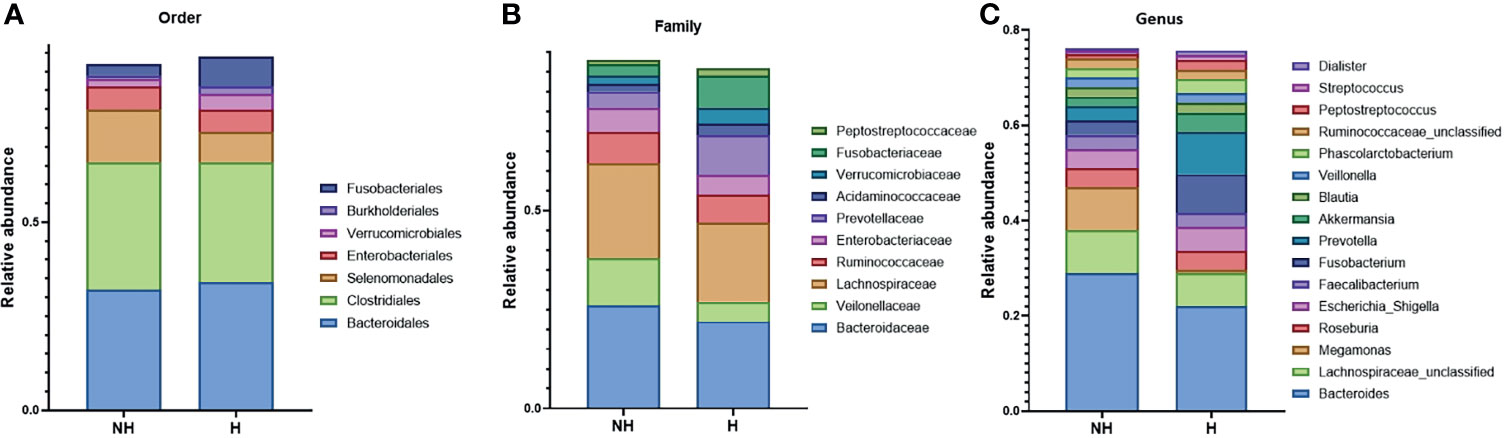
Figure 3 The relative abundance of the bacterial taxa with more than 2% of the abundance. (A) The seven most abundant bacteria in the order level. (B) The 10 most abundant bacteria in the family level. (C) The 16 most abundant bacteria in the genus level.
With the linear discriminant analysis (LDA) on effective size (LefSe), we generated a global view of gut microbiota and revealed 25 bacteria taxa with differential relative abundance in both NH and H groups (LDA score > 2, Figure 4). The LDA result was consistent with the Krona plots. The Peptostreptococcaceae, Eubacterium, and Allisonella were enriched from the hematochezia group, while the Ruminococcaceae, Megasphera, and Megamonas were distinguished from the non-hematochezia group. Dominant population of Bacteroidaceae and Lachnospiraceae in each group depend on species. OTU 35, 54, 162, 134, and 212 of Lachnospiraceae were significant in the non-hematochezia group. OTU 797 and 993 of Bacteroidaceae were found in the hematochezia group.
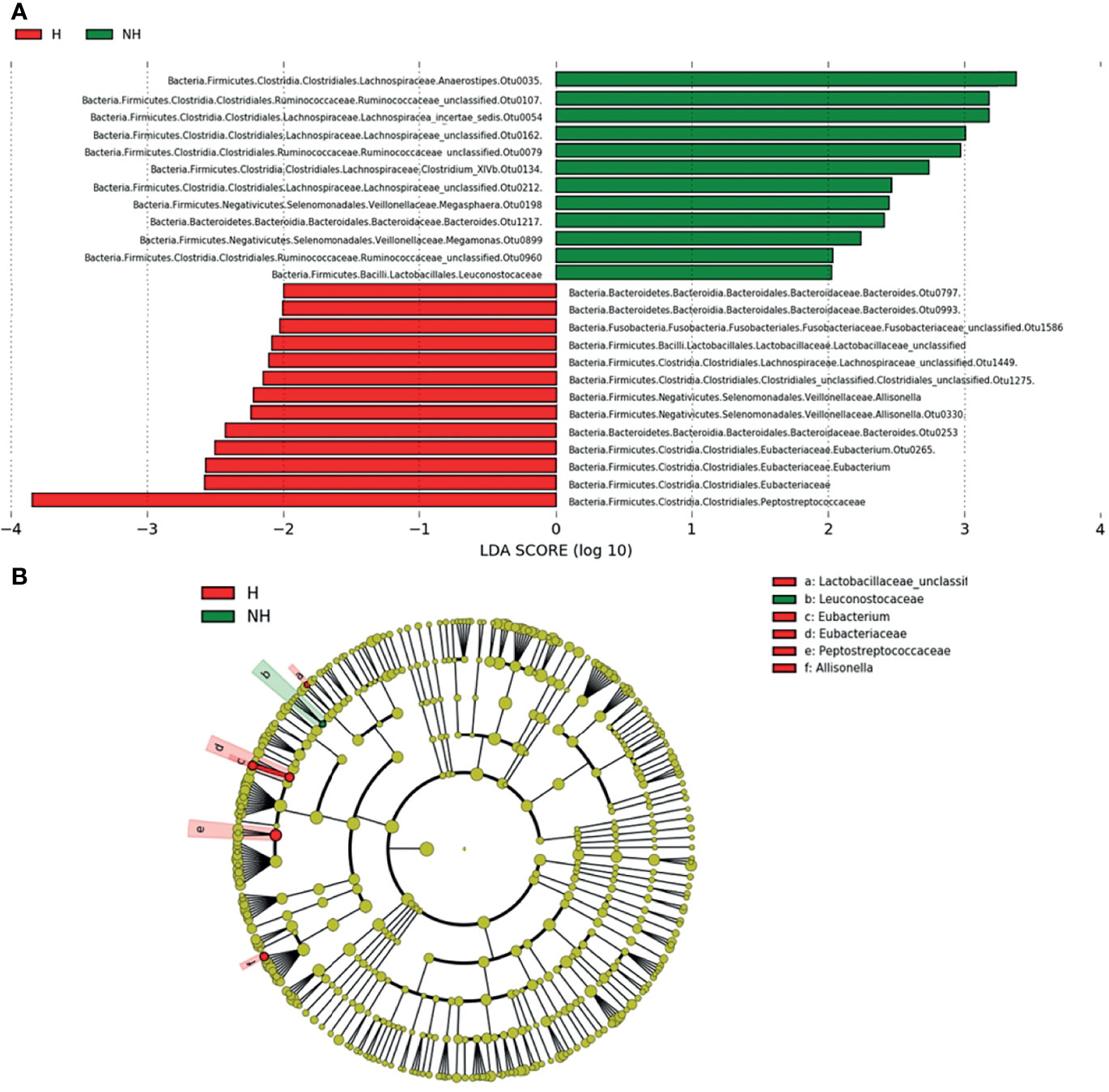
Figure 4 LDA score (A) and evolutionary diagram (B), with circles representing taxonomic levels from phylum to genus/species. Each small circle at a different classification level represents a classification at that level, and the diameter of the small circle is proportional to the relative abundance. The species with no significant difference were yellow, while the differential species were colored as indicated.
Peptostreptococcus is the most abundant genus within the Peptostreptococcaceae family (accounts for >70% of the family in the H group; 84% in the NH group, Supplementary Figure S4), which met the highest LDA score in the hematochezia group. We compared the relative abundance of Peptostreptococcus OTU45 from each group and found that the data distribution was similar with that of Peptostreptococcaceae (Figure 5), which indicated that the abundance of Peptostreptococcus contributed to dysbiosis in hematochezia patients. In contrast, the relative abundance of Lachnospiraceae OTU212 was significantly higher in the non-hematochezia group, occupying 0.1% of the rectal microbiome. In addition, Bacteroidaceae OTU993, Lachnospiraceae OTU1449, Fusobacteriaceae OTU1586, and Clostridiales OTU1275 were uniquely found from the hematochezia group. Bacteroidaceae OTU217 and Megamonas OTU899 were identified from the non-hematochezia group (Figure 5, Supplementary Figure S5).
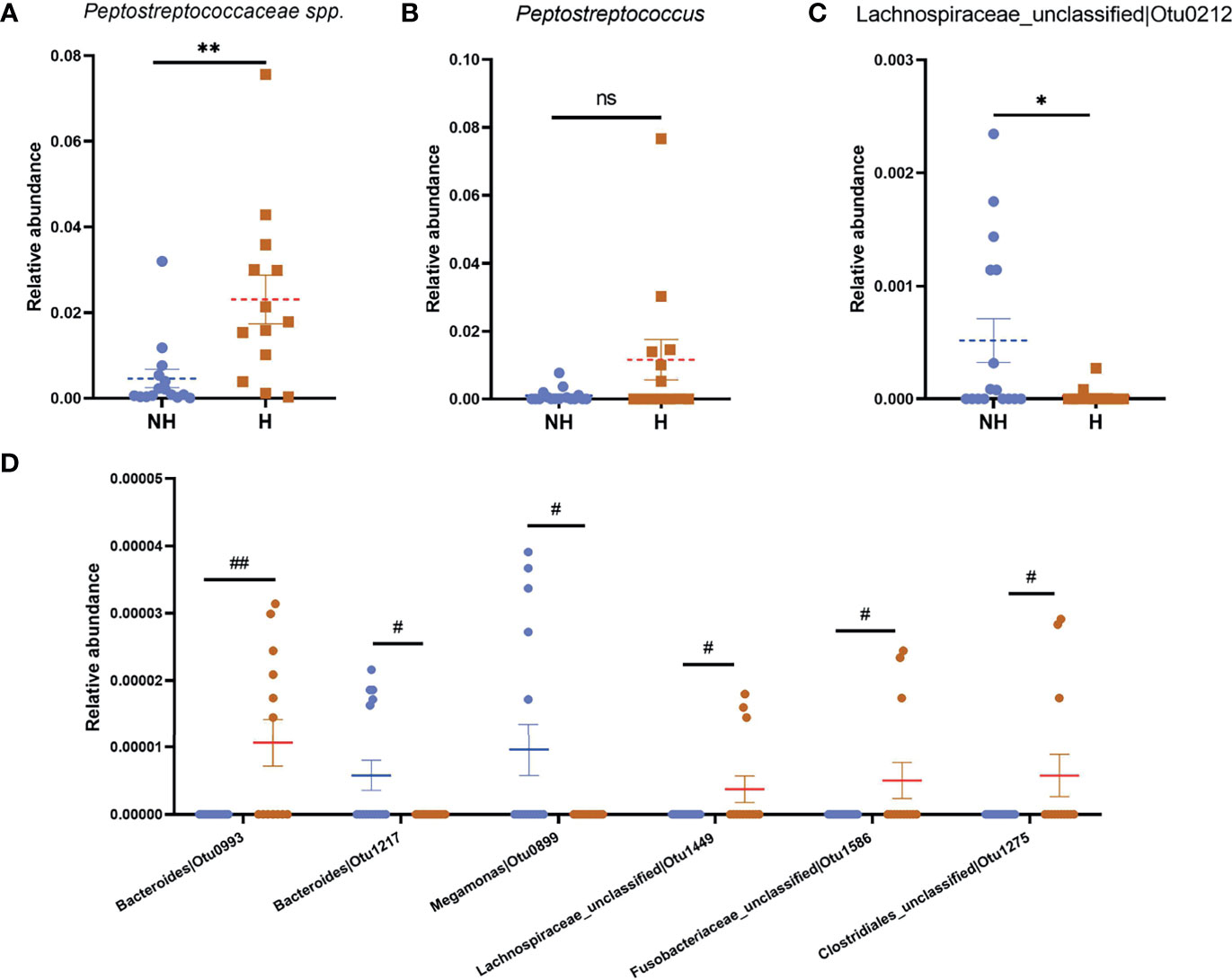
Figure 5 Significantly differential relative abundance of bacteria in Heamafecia patients. The relative abundance of Peptostreptococcaceae species (A), Peptostreptococcus OTU45 (B) and Lachnospiraceae OTU212 (C) are shown. 4 OTU were unique in H group while 2 OTU were found in NH group (D). Error bars indicate SEM. Blue dots: Relative abundance of bacteria in NH; brown squares: Relative abundance of bacteria in H group. ns, not significant, *p < 0.05, **p < 0.01, #qFDR < 0.1, ##qFDR < 0.05.
These data imply that the bacterial taxa in the hematochezia group is interfered and may alter the microenvironment in colorectum, leading to aggravation of chronic radiation proctitis symptoms.
Microbial Functional Dysbiosis in the Hematochezia Group
As the composition of microbiota community altered, functional and metabolic changes could be predicted based on previous molecular studies (23, 24). Four Clusters of Orthologous Groups (COG) with p < 0.01 and four Kyoto Encyclopedia of Genes and Genome (KEGG) orthology with p < 0.05 were identified (Figure 6). The Protease E (COG3340, Supplementary Table S1) was significantly increased in the hematochezia group, while the β-galactosidase βsubunit (COG2731), the Mannitol-1-phosphate/altronate dehydrogenases (COG246), and the Thymidine phosphorylase (COG213) were downregulated (Figure 6A). Notably, mineral absorption and the metabolism of arachidonic acid (ARA) were significantly higher in the hematochezia group according to the annotation of the KEGG orthology (Figure 6B). It is known that ARA is not only an important composition of bacterial cell membrane but also interacts with mammalian cell to improve biosynthesis of inflammatory mediators prostaglandin (PGE2 and PGI2). Also, phenylpropanoid biosynthesis and cyanoaminoacid metabolism showed significant difference. The functional dysbiosis in CRP patients with hematochezia suggested an exacerbated inflammation state caused by rectal dysbiosis.
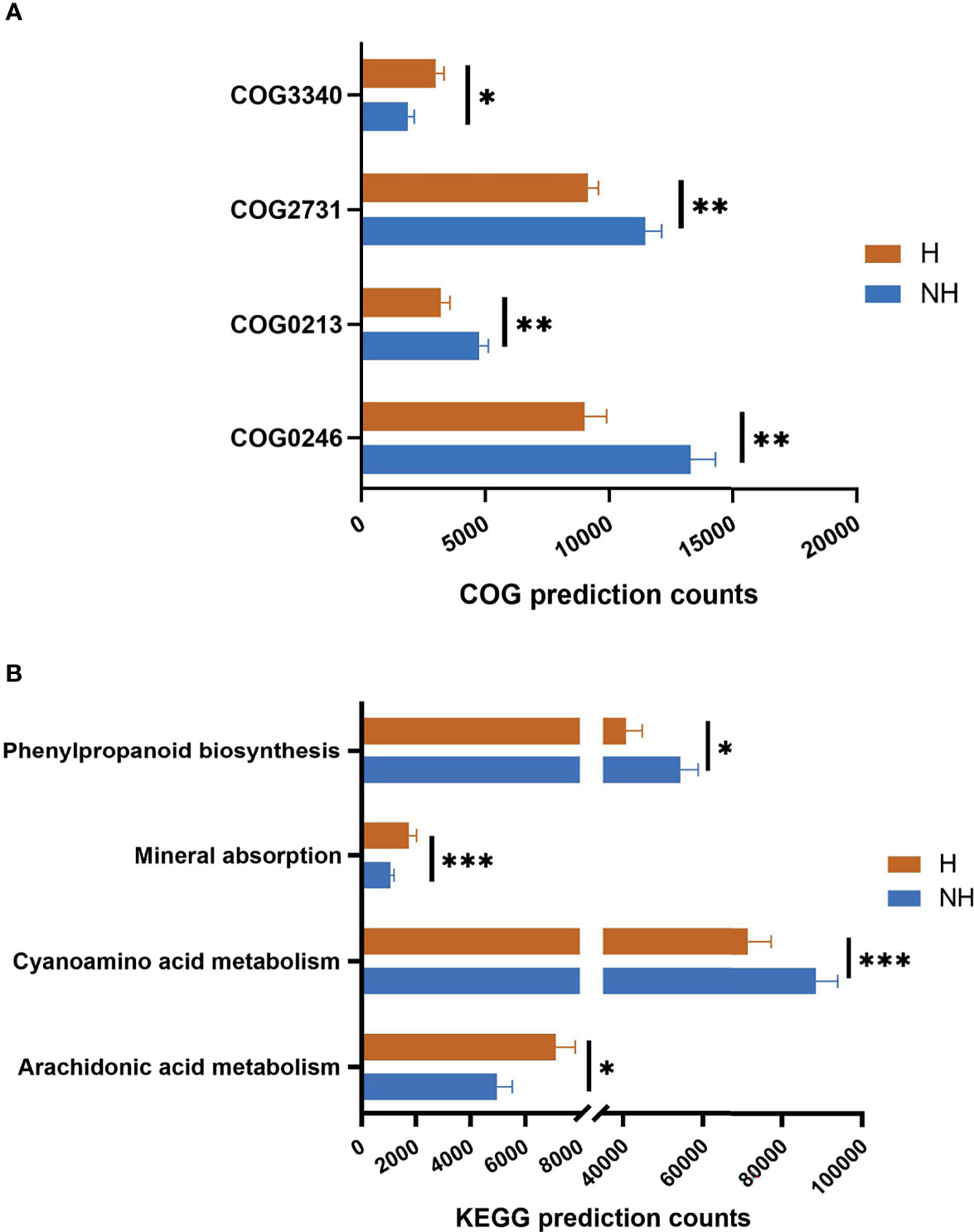
Figure 6 The distribution of functional categories. The distribution of (A) COG and (B) KEGG function with significant difference between two groups. The column length represents the corresponding samples in this metabolic pathway, and the error bar represents the standard error of abundance. *p < 0.05, **p < 0.01, ***p < 0.001.
Functional analysis of rectal microbiota was also compared with healthy volunteers (Supplementary Figure S6). The Fe-S protein (COG1600), glutaredoxins (COG4545), and glutathione peroxidase (COG386) (25) were predicted to be significantly increased in both of the CRP groups. Mineral absorption and ARA metabolism in healthy controls were further reduced, compared with samples from CRP patients.
Discussion
Rectal bleeding is the most common symptom of chronic radiation proctitis (CRP) that lays heavy medical burden to the patients. In this pilot study, we took gut microbiota samples from CRP patients under a proctoscope and revealed an altered microbial ecosystem in CRP patients with hematochezia.
CRP patients are mostly outpatients in surgery clinic seeking for remission of rectal irritation and hematochezia. It is believed that the signs and symptoms of CRP are largely contributed by interstitial fibrosis and occlusive endovascular inflammation (5, 6). Proctoscope examination provides a clearer vision of rectal mucus in a relatively convenient and cost-effective way (1). It is suggested that regular proctoscope could be beneficial to patients with radiation proctitis (3). Here, we included patients with an array of criteria including patients’ observation of blood in stool, digital rectal examination (DRE), and doctors’ observation under a proctoscope. A systematic history collection with multiple categories is helpful for not only research purposes but also outpatient management.
Dysbiosis has been demonstrated to be radiation-induced enteric inflammatory, although most of the research focused on acute enteritis. In a previous study on irradiation-inferred microbiome, Clostridium IV, Roseburia, and Phascolarctobacterium (13) were enriched. In this study, we found that relative abundances of these bacterium were similar between H and NH groups, implying a specific bacterium involves in prolonged bleeding. To our knowledge, this is the first study digging into the association between radiation-induced dysbiosis and chronic hemorrhage. A limitation of this study is the lack of pre-radiotherapy microbiome data, which could provide the baseline individual variation for better causative analysis and facilitate identification of biomarker for prognosis of pelvic cancer radiotherapy. A multicenter study with a larger sample size including male patients who survived other pelvic cancer is expected.
In this population of CRP patients, hematochezia is associated with an increased proportion of Peptostreptococcaceae, and Peptostreptococcus OTU45 is of most abundance in this family. High density of Peptostreptococcaceae was displayed in the intestine of humans with non-alcoholic fatty liver disease, suggesting the connection between Peptostreptococcaceae and impaired mucosal immune function (15). Dysregulated inflammation could be resulting from stress response. Gao et al. utilized a chronic stress mouse model to investigate the role of gut microbiota in stress-induced inflammatory bowel disease (IBD). They found that Peptostreptococcaceae were one of the inflammation-promoting OTUs and could be eliminated by antibiotics (26), which had also been considered as radiation response modifier. Clathrin adapter AP-1B is the epithelium-specific basolateral targeting factor that polarizes the epithelial cells, maintaining homeostasis of the gastrointestinal immune system. AP-1B was demonstrated to be the key factor of Peptostreptococcaceae abundance, which was significantly increased in Aplm2-/- colitis mice, but the detailed mechanism remains unclear (27). These lines of evidence suggest that Peptostreptococcaceae is highly associated with gastrointestinal inflammation, which may subsequently influence epithelial cell repair capacity or proliferation rate.
Diminished composition of Lachnospiraceae is also known to be related to gastrointestinal inflammation. In patients with IBD and acute colitis, Lachnospiraceae, Ruminococcus spp., Faecalibacterium spp., and Roseburia spp. were consistently depleted (28), suggesting that they are crucial gastrointestinal probiotics. Here,we observed that Lachnospiraceae OTU212 in the hematochezia group was significantly reduced, compared to that in the non-hematochezia group. Our LDA result also showed that genus Lachnospiraceae_FCS20 was higher in healthy controls, compared with CRP patients. Interestingly, Lachnospiraceae bacterium may contribute to the development of diabetes in obese mice (29) and calorie restriction resulted in lower Lachnospiraceae proportion (30). CRP with prolonged hematochezia and radiation enteritis patients have a relatively thin physique. However, the causation of specific Lachnospiraceae insufficiency and the decrease of body weight/mass index or diet intake remain to be elucidated.
Microbiota produce vitamins, energy sources, and amino acids by degradation and processing of diet-derived substrates. In this study, the differential mineral absorption, ARA metabolism, and Protease E between H and NH groups were predicted by PICRUSt. One possible explanation is that the micro-environment with continuous bleeding favors the high-metabolic-activity bacterium. The Grxs, FeS, and GPx upregulated in CRP patients are key regulators of cell redox and electron transport that are involved in various processes of biosynthesis and detoxification (31). Glutaredoxins (Grxs)-ligated Fe-S cluster participates in oxidative signaling (32) and haem synthesis (33), which may be compensatory to the prolonged anemia. The Grxs system also acts as an efficient electron donor to plasma glutathione peroxidase (GPx) (25). GPx is known as one of the major enzymes that prevent oxidative stress by catalyzing glutathione (GSH) into glutathione disulfide (GSSG). The increase of Grxs, FeS, and GPx may result from a high oxidative micro-environment after irradiation. In addition, GPx together with ARA enriched in the hematochezia group is capable of regulating prostaglandin PGE2 and PGI2, which promote gut inflammation (34). A prospective study of ARA and/or GPx effect on inflammation and hemorrhage in a post-irradiation model is expected in the future.
The dysbiosis could be related to the change of gene expression in glandular and/or epithelial cells induced by irradiation and inflammation (35). Thus, a combination of larger-scale metagenomic data, transcriptome profiles, and proteomic profile of an inflammatory factor study is expected to provide a comprehensive view of post-irradiation gut–microbiome interaction (36). In summary, this is a pilot study revealing the altered gut microbiota in CRP with hematochezia, providing a fundamental evidence for developing and evaluating treatments for CRP.
Data Availability Statement
The datasets presented in this study can be found in online repositories. The names of the repository/repositories and accession number(s) can be found at: https://www.ncbi.nlm.nih.gov/genbank/, PRJNA682045.
Ethics Statement
The studies involving human participants were reviewed and approved by the Ethics Board at the Affiliated TCM Hospital of Guangzhou Medical University. The patients/participants provided their written informed consent to participate in this study.
Author Contributions
JL and LL conceived the study. LL contributed to the literature search, preparation of figures and panels, and writing of the manuscript. CC, BC, and XL contributed to sample collection clinical data analysis. CD contributed to programing data analysis. All authors have seen and agreed on the final submitted version of the manuscript.
Funding
This article is an independent research funded by the the Affiliated TCM Hospital of Guangzhou Medical University (2018A01) and Guangdong Provincial Administration of Traditional Chinese Medicine (20211293).
Conflict of Interest
The authors declare that the research was conducted in the absence of any commercial or financial relationships that could be construed as a potential conflict of interest.
Publisher’s Note
All claims expressed in this article are solely those of the authors and do not necessarily represent those of their affiliated organizations, or those of the publisher, the editors and the reviewers. Any product that may be evaluated in this article, or claim that may be made by its manufacturer, is not guaranteed or endorsed by the publisher.
Acknowledgments
We would like to thank Ms. Nana Xiao from Guangzhou University of Chinese Medicine for her enthusiastic involvement in documentation works.
Supplementary Material
The Supplementary Material for this article can be found online at: https://www.frontiersin.org/articles/10.3389/fonc.2021.637265/full#supplementary-material
References
1. Rustagi T, Mashimo H. Endoscopic Management of Chronic Radiation Proctitis. World J Gastroenterol (2011) 17:4554–62. doi: 10.3748/wjg.v17.i41.4554
2. Gerassy-Vainberg S, Blatt A, Danin-Poleg Y, Gershovich K, Sabo E, Nevelsky A, et al. Radiation Induces Proinflammatory Dysbiosis: Transmission of Inflammatory Susceptibility by Host Cytokine Induction. Gut (2018) 67(1):97–107. doi: 10.1136/gutjnl-2017-313789
3. Zinicola R, Rutter MD, Falasco G, Brooker JC, Cennamo V, Contini S, et al. Haemorrhagic Radiation Proctitis: Endoscopic Severity may be Useful to Guide Therapy. Int J Colorectal Dis (2003) 18(5):439–44. doi: 10.1007/s00384-003-0487-y
4. Ramakrishnaiah VPN, Krishnamachari S. Chronic Haemorrhagic Radiation Proctitis: A Review. World J Gastrointest Surg (2016) 8(7):483. doi: 10.4240/wjgs.v8.i7.483
5. Vanneste BGL, De Voorde LV, De Ridder RJ, Van Limbergen EJ, et al. Chronic Radiation Proctitis: Tricks to Prevent and Treat (2015). Available at: https://www.ncbi.nlm.nih.gov/pmc/articles/PMC4575375/pdf/384_2015_Article_2289.pdf.
7. Gul YA, Prasannan S, Jabar FM, Shaker ARH, Moissinac K. Pharmacotherapy for Chronic Hemorrhagic Radiation Proctitis. World J Surg (2002) 26(12):1499–502. doi: 10.1007/s00268-002-6529-8
8. Ferreira MR, Muls A, Dearnaley DP, Andreyev HJN. Microbiota and Radiation-Induced Bowel Toxicity: Lessons From Inflammatory Bowel Disease for the Radiation Oncologist. Lancet Oncol (2014) 15(3):e139–47. doi: 10.1016/S1470-2045(13)70504-7
9. Wei Y, Li Y, Yan L, Sun C, Miao Q, Wang Q, et al. Alterations of Gut Microbiome in Autoimmune Hepatitis. Gut (2019) 1–9. doi: 10.1136/gutjnl-2018-317836
10. Depommier C, Everard A, Druart C, Plovier H, Van Hul M, Vieira-Silva S, et al. Supplementation With Akkermansia Muciniphila in Overweight and Obese Human Volunteers: A Proof-of-Concept Exploratory Study. Nat Med (2019) 25:1096–103. Nature Publishing Group. doi: 10.1038/s41591-019-0495-2
11. Lloyd-Price J, Arze C, Ananthakrishnan AN, Schirmer M, Avila-Pacheco J, Poon TW, et al. Multi-Omics of the Gut Microbial Ecosystem in Inflammatory Bowel Diseases. Nature (2019) 569(7758):655–62. doi: 10.1038/s41586-019-1237-9
12. Manichanh C, Varela E, Martinez C, Antolin M, Llopis M, Dor J, et al. The Gut Microbiota Predispose to the Pathophysiology of Acute Postradiotherapy Diarrhea. Am J Gastroenterol (2008) 103(7):1754–61. doi: 10.1111/j.1572-0241.2008.01868.x
13. Reis Ferreira M, Andreyev HJN, Mohammed K, Truelove L, Gowan SM, Li J, et al. Microbiota- and Radiotherapy-Induced Gastrointestinal Side-Effects (MARS) Study: A Large Pilot Study of the Microbiome in Acute and Late-Radiation Enteropathy. Clin Cancer Res (2019) 25(21):6487–500. doi: 10.1158/1078-0432.CCR-19-0960
14. Ciorba MA, Riehl TE, Rao MS, Moon C, Ee X, Nava GM, et al. Lactobacillus Probiotic Protects Intestinal Epithelium From Radiation Injury in a TLR-2/Cyclo-Oxygenase-2-Dependent Manner. Gut (2012) 61(6):829–38. doi: 10.1136/gutjnl-2011-300367
15. Jiang W, Wu N, Wang X, Chi Y, Zhang Y, Qiu X, et al. Dysbiosis Gut Microbiota Associated With Inflammation and Impaired Mucosal Immune Function in Intestine of Humans With Non-Alcoholic Fatty Liver Disease. Sci Rep (2015) 5:8096. doi: 10.1038/srep08096
16. Purcell RV, Visnovska M, Biggs PJ, Schmeier S, Frizelle FA. Distinct Gut Microbiome Patterns Associate With Consensus Molecular Subtypes of Colorectal Cancer. Available at: https://www.nature.com/articles/s41598-017-11237-6.pdf.
17. Schloss PD, Westcott SL, Ryabin T, Hall JR, Hartmann M, Hollister EB, et al. Introducing Mothur: Open-Source, Platform-Independent, Community-Supported Software for Describing and Comparing Microbial Communities. Appl Environ Microbiol (2009) 75(23):7537–41. doi: 10.1128/AEM.01541-09
18. Ahn J, Sinha R, Pei Z, Dominianni C, Wu J, Shi J, et al. Human Gut Microbiome and Risk for Colorectal Cancer. J Natl Cancer Inst (2013) 105(24):1907–11. doi: 10.1093/jnci/djt300
19. Langille MGI, Zaneveld J, Caporaso JG, McDonald D, Knights D, Reyes JA, et al. Predictive Functional Profiling of Microbial Communities Using 16S rRNA Marker Gene Sequences. Nat Biotechnol (2013) 31(9):814–21. doi: 10.1038/nbt.2676
20. Segata N, Izard J, Waldron L, Gevers D, Miropolsky L, Garrett WS, et al. Metagenomic Biomarker Discovery and Explanation. Genome Biol (2011) 12:R60. doi: 10.1186/gb-2011-12-6-r60
21. Moulton CR, House MJ, Lye V, Tang CI, Krawiec M, Joseph DJ, et al. Prostate External Beam Radiotherapy Combined With High-Dose-Rate Brachytherapy: Dose-Volume Parameters From Deformably-Registered Plans Correlate With Late Gastrointestinal Complications. Radiat Oncol (2016) 11(1):144. doi: 10.1186/s13014-016-0719-2
22. Ondov BD, Bergman NH, Phillippy AM. Interactive Metagenomic Visualization in a Web Browser. BMC Bioinformatics (2011) 12(1):1–10. doi: 10.1186/1471-2105-12-385
23. Heintz-Buschart A, Wilmes P. Human Gut Microbiome: Function Matters. Trends Microbiol (2018) 26(7):563–74. doi: 10.1016/j.tim.2017.11.002
24. Arnold JW, Roach J, Azcarate-Peril MA. Emerging Technologies for Gut Microbiome Research. Trends Microbiol (2016) 24(11):887–901. doi: 10.1016/j.tim.2016.06.008
25. Björnstedt M, Xue J, Huang W, Åkesson B, Holmgren A. The Thioredoxin and Glutaredoxin Systems are Efficient Electron Donors to Human Plasma Glutathione Peroxidase. J Biol Chem (1994) 269(47):29382–4. doi: 10.1016/S0021-9258(18)43889-6
26. Gao X, Cao Q, Cheng Y, Zhao D, Wang Z, Yang H, et al. Chronic Stress Promotes Colitis by Disturbing the Gut Microbiota and Triggering Immune System Response. Proc Natl Acad Sci U S A (2018) 115(13):E2960–9. doi: 10.1073/pnas.1806622115www.pnas.org
27. Jangid A, Fukuda S, Seki M, Horiuchi T, Suzuki Y, Taylor TD, et al. Association of Colitis With Gut-Microbiota Dysbiosis in Clathrin Adapter AP-1B Knockout Mice. PloS One (2020) 15(3):e0228358. doi: 10.1371/journal.pone.0228358. Blachier F, Editor.
28. Suchodolski JS. Gastrointestinal Microbiota. In: Washabau RJ, Day MJ, editors. Canine and Feline Gastroenterology. W.B. Saunders (2013). p. 32–41.
29. Kameyama K, Itoh K. Intestinal Colonization by a Lachnospiraceae Bacterium Contributes to the Development of Diabetes in Obese Mice. Microbes Environ (2014) 29(4):427–30. doi: 10.1264/jsme2.ME14054
30. Wang S, Huang M, You X, Zhao J, Chen L, Wang L, et al. Gut Microbiota Mediates the Anti-Obesity Effect of Calorie Restriction in Mice. Sci Rep (2018) 8(1):13037. doi: 10.1038/s41598-018-31353-1
31. Mitsou EK, Detopoulou M, Kakali A, Fragopoulou E, Nomikos T, Antonopoulou S, et al. Mining Possible Associations of Faecal A. Muciniphila Colonisation Patterns With Host Adiposity and Cardiometabolic Markers in an Adult Population. Benef Microbes (2019) 10(7):741–9. doi: 10.3920/BM2019.0033
32. Couturier J, Przybyla-Toscano J, Roret T, Didierjean C, Rouhier N. The Roles of Glutaredoxins Ligating Fe-S Clusters: Sensing, Transfer or Repair Functions? Biochim Biophys Acta - Mol Cell Res (2015) 1853:1513–27. Elsevier. doi: 10.1016/j.bbamcr.2014.09.018
33. Wingert RA, Galloway JL, Barut B, Foott H, Fraenkel P, Axe JL, et al. Deficiency of Glutaredoxin 5 Reveals Fe-S Clusters are Required for Vertebrate Haem Synthesis. Nature (2005) 436(7053):1035–9. doi: 10.1038/nature03887
34. Meskini N, Nemoz G, Chapuy P, Haond P, Pageaux JF, Vericel E, et al. Glutathione Peroxidase Activity Ahnd Metabolism of Arachidonic Acid in Peripheral Blood Mononuclear Cells From Elderly Subjects. Clin Sci (1993) 85(2):203–11. doi: 10.1042/cs0850203
35. Colon J, Hsieh N, Ferguson A, Kupelian P, Seal S, Jenkins DW, et al. Cerium Oxide Nanoparticles Protect Gastrointestinal Epithelium From Radiation-Induced Damage by Reduction of Reactive Oxygen Species and Upregulation of Superoxide Dismutase 2. Nanomed Nanotechnol Biol Med (2010) 6(5):698–705. doi: 10.1016/j.nano.2010.01.010
Keywords: chronic radiation proctitis, hematochezia, gut microbiota, Peptostreptococcaceae, Lachnospiraceae
Citation: Liu L, Chen C, Liu X, Chen B, Ding C and Liang J (2021) Altered Gut Microbiota Associated With Hemorrhage in Chronic Radiation Proctitis. Front. Oncol. 11:637265. doi: 10.3389/fonc.2021.637265
Received: 25 March 2021; Accepted: 20 September 2021;
Published: 15 October 2021.
Edited by:
Pelagia G. Tsoutsou, Geneva University Hospitals (HUG), SwitzerlandReviewed by:
Giovanni Cestaro, Azienda Ulss 5 Polesana, ItalyJustyna Chałubińska-Fendler, Military Institute of Medicine, Poland
Copyright © 2021 Liu, Chen, Liu, Chen, Ding and Liang. This is an open-access article distributed under the terms of the Creative Commons Attribution License (CC BY). The use, distribution or reproduction in other forums is permitted, provided the original author(s) and the copyright owner(s) are credited and that the original publication in this journal is cited, in accordance with accepted academic practice. No use, distribution or reproduction is permitted which does not comply with these terms.
*Correspondence: Jinjun Liang, MjAxOTYyMDQwMkBnemhtdS5lZHUuY24=