- 1Department of Pancreatic and Biliary Surgery, The First Affiliated Hospital of Harbin Medical University, Harbin, China
- 2Key Laboratory of Hepatosplenic Surgery, Ministry of Education, Harbin, China
Pancreatic cancer (PC) is a malignant tumor with high invasiveness, easy metastatic ability, and chemoresistance. Patients with PC have an extremely low survival rate due to the difficulty in early diagnosis. It is estimated that nearly 90% of PC cases are caused by environmental risk factors. Approximately 50% of PC cases are induced by an unhealthy diet, which can be avoided. Given this large attribution to diet, numerous studies have assessed the relationship between various dietary factors and PC. This article reviews three beneficial diets: a ketogenic diet (KD), a Mediterranean diet (MD), and a low-sugar diet. Their composition and impact mechanism are summarized and discussed. The associations between these three diets and PC were analyzed, and we aimed to provide more help and new insights for the prevention and treatment of PC.
Introduction
Pancreatic cancer (PC) is known for its insidious onset, invasive fast-growing nature, and poor prognosis (1). Although radical resection is currently the primary therapeutic option, most patients miss the appropriate time due to its unspecific early clinical manifestation. Although great progress has been made in radiotherapy (RT), chemotherapy, and immunotherapy in recent years, the desired results have not yet been achieved (2). Avoiding high-risk factors is the first and most crucial step to change its incidence. Current evidence suggests that up to one-third of cancer deaths can be prevented by reducing risk factors, and an unhealthy diet is one of the most crucial factors (3). Dietary composition affects tumor growth and progression and creates potential synergies or antagonisms between new or existing therapeutics (4). Diet can affect tumor growth through various mechanisms that alter cancer cell metabolism (Figure 1). The components within the diet could even improve the prognosis by affecting drug efficacy and resistance (5). Research on the relationship between dietary composition and cancer risk is becoming increasingly crucial. This review stresses several different kinds of typical diets, and the effects of some components within the diet are analyzed in PC treatment. The aim is to deepen the knowledge about the role of diets in PC and the underlying mechanisms, which provides evidence for further developing PC prevention strategies. Second, it is hoped that this study can fill the gaps in the treatment methods of PC and improve the treatment effect and patient survival rate. Finally, we aim to promote multidisciplinary prospective research to advance the field.
The Ketogenic Diet (KD)
There is no precise definition of the KD. Thus, many studies have defined it as any diet that leads to an increase in blood ketones (6), for example, diets in which more than 50% of total calories are from fat (7). However, the KD is generally accepted to be characterized by high fat, moderate protein, and very low carbohydrate composition. The classical KD contains four parts fat, one part carbohydrate, and one part protein (4:1:1) (8). It delivers 90% of its calories from fat, 8% from protein, and only 2% from carbohydrates. However, the KD used in the clinical setting has a ratio of fat to carbohydrate and fat to protein of at least 2:1 and 3:1 (9). The development of the KD has been relatively completed thus far. All-liquid and parenteral KDs have been studied (10), thus, the diet is often started on an outpatient basis (11).
The KD is regarded as a metabolic therapy widely used in the treatment of epilepsy (12). Recent studies have confirmed the therapeutic potential of the KD in many pathological conditions over the last decade, including diabetes, polycystic ovary syndrome, acne, neurological diseases, malignant tumors, and the amelioration of respiratory and cardiovascular disease risk factors (13, 14). A growing number of preclinical studies suggest that the KD as a dietary intervention is a potent anticancer therapy (15).
Various investigators have used the term “therapeutic ketosis”, which implies the achievement of plasma ketone body levels in the 2-7 mmol/L range, comparable to concentrations found in subjects maintained on various KDs (16). The KD leads to an increase in ketone bodies without restricting energy intake, a clear advantage in the cancer setting (17). The KD suppresses the Warburg effect by inducing tumor starvation, which is generally considered its anticancer mechanism (18). The Warburg effect is characterized as mainly adopting glycolysis as an energy source to maintain tumor cell growth and biosynthesis in cancers (19). Glucose dependency and lactate production are recognized as two key features related to the aggressive capacity and invasive potential of cancer cells (20). Normal cells readily use ketones as an alternate energy source and induce a shift in cellular energy supply from glucose to fatty acids and ketones to protect against hypoglycemia when ketone body levels rise (21). Cancer cells are incapable of ketone body metabolism due to their acquired metabolic inflexibility (22). As a result, ketone bodies cannot be consumed, and tumor development is inhibited (23). Many studies have confirmed that the KD has a positive effect on various types of cancer and could retard the progression of gastric cancer, prostate cancer, and brain cancer in mouse models (24, 25). In addition, the KD was found to eliminate tumor growth by inhibiting angiogenesis and reducing tumor volume in preclinical trials (26). This ability was linked to the reduction in glucose availability, insulin, and circulating insulin-like growth factor (IGF)-1 levels (27, 28). Ketosis has been confirmed to be inversely associated with serum insulin levels and correlated with stable disease or partial remission (29).
Husain et al. (30) confirmed that the KD could decrease the activation of natural killer cells, improve the numbers of myeloid-derived suppressor cells (MDSCs) in a PC mouse model, and enhance the host immune response to tumor cells. This suggested that several mechanisms could support the effectiveness of the KD in cancer treatment, far beyond the originally proposed inhibition of glucose/insulin signaling, including oxidative stress, mitochondrial metabolism, and inflammation (31). The increased oxidative stress and reactive oxygen species (ROS) production are attributable to mitochondrial damage (32). In addition, chronic inflammation from sustained hyperglycemia also represents a major source of ROS production in tumors (33). Stafford et al. (34) reported that the KD reduced the rate of tumor growth and prolonged survival with reduced ROS production in cancer cells. Ketosis protects against oxidative stress in healthy tissues by simultaneously enhancing endogenous antioxidant capacity and decreasing ROS production (35). Moreover, cancer cells are inefficient in metabolizing toxic substances (28). These factors allowed the KD to selectively inhibit metabolism in cancer cells but not in normal cells.
Surgery remains the primary treatment for PC, and radical tumor resection can considerably reduce the risk of cancer recurrence and increase the 5-year survival rate (36). However, patients who undergo pancreatectomy are more susceptible to malnourishment and weight loss due to complications, such as pancreatic fistula and delayed gastric emptying (37). It has been suggested that the KD improves meal compliance, satisfaction, and the energy intake rate in post-pancreatectomy patients without increasing complications of the digestive system. It is a safe way to increase energy and nutrient intake in pancreato-biliary cancer patients after surgery (38, 39). Unfortunately, only 20-30% of PC patients are candidates for surgical resection, as most are diagnosed with locally advanced PC or metastatic PC (40). As a result, chemotherapy must be conferred as a survival advantage for those patients. Recent guidelines recommend gemcitabine monotherapy or gemcitabine-based combination therapies; however, even the FOLFIRINOX (5-fluorouracil, folinic acid [leucovorin], irinotecan, and oxaliplatin) regimen is considered as an option for some patients (41, 42). RT, chemotherapy, and current nonsurgical standard therapies for cancer therapies share a common mechanism, which is to kill cancer cells by enhancing the production of ROS (43). Therefore, the application of a KD during treatment would selectively enhance tumor cell versus normal cell sensitivity to RT and chemotherapy by inducing oxidative stress (44).
Metabolically supported chemotherapy (MSCT) is a novel approach targeting the dysregulated energy mechanism of tumor cells and has been generally combined with KD, hyperthermia (HT), and hyperbaric oxygen therapy (HBOT) in advanced PC patients (45, 46). The effects of MSCT (either gemcitabine based or FOLFIRINOX regimen administered concomitantly with induced hypoglycemia) plus the KD, HT, and HBOT combination have been investigated in a clinical trial. A total of 25 metastatic pancreatic ductal adenocarcinoma (PDAC) patients were enrolled, and past data were compared. The results showed that the overall median survival rate of patients receiving gemcitabine therapy alone was approximately 6.8 months, and that of the FOLFIRINOX group was 11.1 months. Encouragingly, the combination of the KD with MSCT achieved an overall median survival rate of 15.8 months and a progression-free survival rate of 12.9 months (Figure 2) (47). Talib et al. (48) demonstrated that a combination of the KD with melatonin could successfully inhibit cisplatin- and vincristine-resistant breast cancer, which indicates that the KD may improve the treatment effect of drug-resistant patients. In addition, there was evidence of increased survival in mice grafted with high-grade glioma, lung, or PC cells when mice received a KD in association with RT (49, 50). A phase I clinical trial of the KD and PC patients was also conducted, but it was terminated due to suboptimal oral KD compliance and poor tolerance (51). Another trial enrolled 70 cancer patients, half of whom received a KD during RT. The results revealed that the KD improves patient tolerance by improving muscle mass against cachexia (52). This indicated that the KD might be of unexpected value for PC patients at high risk of weight loss and receiving RT and chemotherapy.
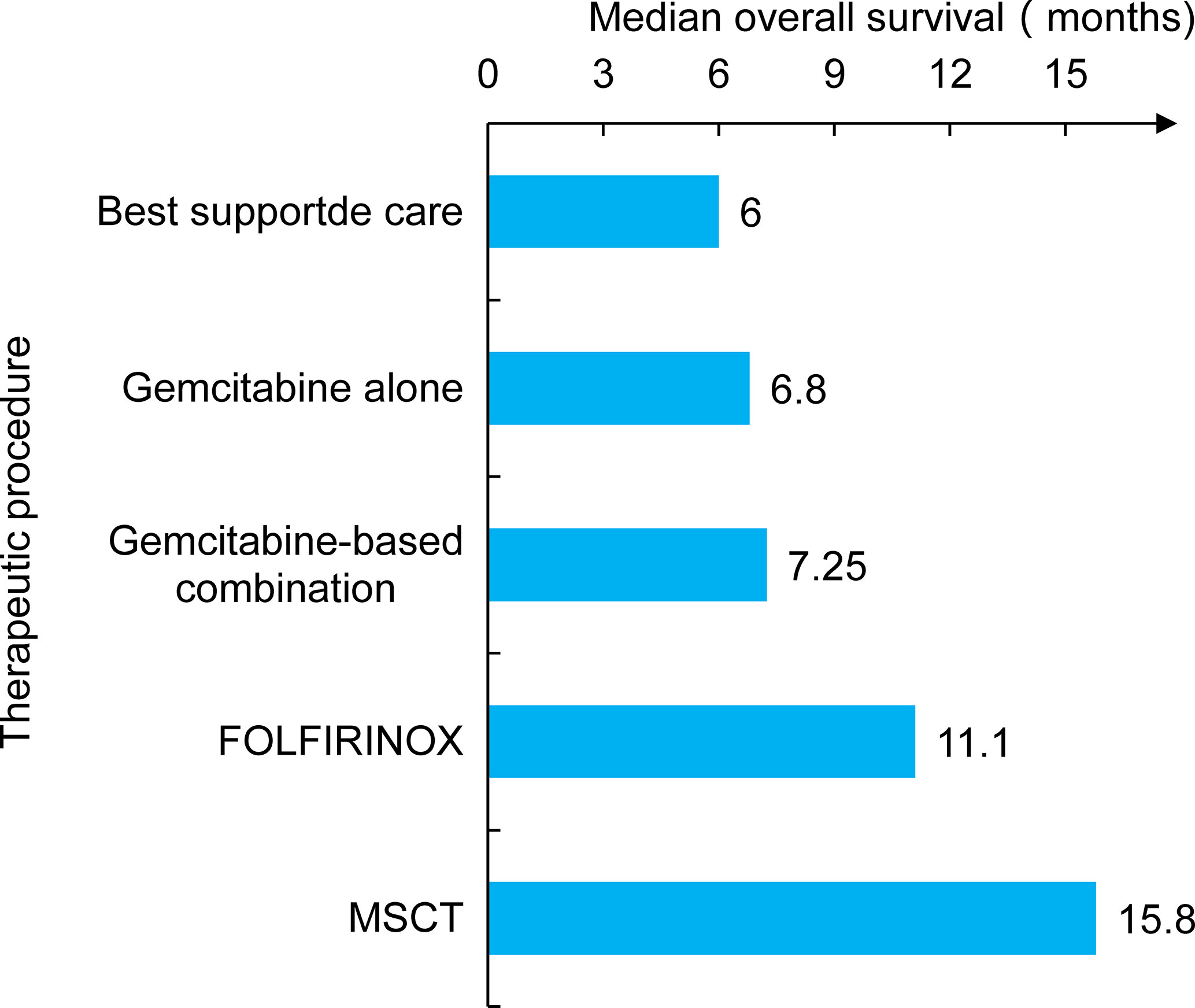
Figure 2 The study compared several treatment modalities, including earlier traditional care and chemotherapy. The patient’s survival time was significantly prolonged when combined chemotherapy with metabolic support and the KD (MSCT).
As reported in most preclinical studies, the process of cancer-induced cachexia can be reversed by a KD (53, 54). The incidence of cachexia in PC is 80%, characterized as a complex metabolic syndrome associated with the advanced disease stages (55). Surendra et al. (56) demonstrated that reversal of the metabolic syndrome induced by ketone bodies might be related to the levels of c-Myc in PC. The ketone body-reduced c-Myc expression suggests that the KD is beneficial to PC. Greene et al. (57) found that a KD decreased body mass without adverse effects on skeletal muscle and muscle strength. In addition, Andrew et al. (58) demonstrated that R/S1,3‐butanediol acetoacetate diester, a novel synthetic ketone diester, attenuates tumor burden indices by diminishing anorexia, altering systemic metabolism, and mitigating catabolism in a cancer‐independent multifactorial inflammation-induced atrophy environment. This indicated that ketone bodies participate in anticatabolic effects by eliminating multifactorial atrophy. Nakamura et al. (59) postulated that elevated blood ketone levels might have antitumor effects by promoting the maintenance of body weight and muscle mass, leading to a reduction in inflammation. KD treatment of cachexia aims to maintain skeletal muscle mass and physical performance, avoid interruption of scheduled anticancer treatments, and improve quality of life (60).
The KD might indirectly affect the efficacy of immunotherapy by affecting the metabolism of the host. Flint et al. (61) indicated that the metabolism of calorie-deficient mice would be altered to develop hypoketonemia, which triggered a marked rise in glucocorticoid levels, affecting the effectiveness of immunotherapy in PDAC. They conducted further studies on this view and found that the increased level of ketone bodies in PDAC mice inhibited the systemic metabolic stress response, reducing the suppression of immunotherapy (62). Another study found that glucose-dependent CD8+ tumor-infiltrating lymphocytes (TILs) could undergo a competitive disadvantage for nutrients that might negatively affect their immune function. However, the KD significantly reduces the expression of the inhibitory ligand PD-1 (PD-L1) on CD8+ T cells (63). Additionally, mice fed a KD presented lower expression of PD-L1 in tumor cells that notoriously inhibited CD8+ T cell activity (64). These results suggested that the KD may alter tumor-mediated T cell suppression by reducing the number of cells susceptible to the PD-1 inhibitory pathway. A recent study demonstrated that the enhancement of lipid catabolism in CD8+ T cells increases the efficacy of immunotherapy within a tumor microenvironment low in carbohydrates (65). Mounting evidence has highlighted that nutrient modulation also improves cancer immune surveillance so that severe immunosuppression could be avoided or even that the immune response or immune-based cancer therapies could be potentiated through patient microbiota remodeling (66). The convergence of host metabolism and antitumor immunity may offer a platform for investigations of new combination therapies.
Most experiments have shown that the KD is safe (37–39). The side effects caused by the KD, including constipation, fatigue, leg cramps, vomiting, lack of energy, and hunger, are reversible (67, 68). In addition, the KD should be low in proteins (mainly vegetable proteins) and carbohydrates (non-starchy vegetables) and high in lipids [mainly unprocessed vegetable oils rich in polyunsaturated fatty acids (PUFAs)] (16, 21, 69). It is worth noting that the classic artificial KD may be deficient in vitamins and minerals (70). Adequate supplementation of these micronutrients is essential and should be planned and monitored by physicians and qualified dieticians (71). The paleolithic ketogenic diet (PKD) is based on animal fat, meat, and offal with a fat: protein ratio of about 2:1 (72). The PKD differs from the classical KD in that it excludes food components that are not available in preagricultural times, and it supplies optimal amounts of micronutrients (73). Tóth et al. have proven that the PKD has a considerable effect on soft palate cancer, rectal cancer, glioblastoma multiforme, and cervical intraepithelial neoplasia (72–75). The researchers assume that this diet is evolutionarily advantageous for humans and has superior effectiveness compared to the KD in cancer management (72, 73). Thus, the PKD provides hopes for refractory cancer therapy and we do believe that further studies should be conducted to explore the possible mechanisms of PKD in the treatment of cancer and other chronic diseases.
In most preclinical models, the overall beneficial effects of the KD were suggested (17, 24, 25). However, most of the data took advantage of the mouse model, limiting the translation to preclinical studies (53, 54). Many clinical trials have involved only a few patients, and in some cases, a control group was not included (23, 29). A high level of heterogeneity among studies prevents the formulation of conclusions. In addition, apart from the earliest study on two pediatric oncology patients, other studies on KD were limited in follow-up duration (23). There is no clear evidence available demonstrating long-term benefits of KD, as regards hard clinical endpoints, such as survival or mortality. However, due to the special biological characteristics of PC, many therapies were not very effective. Therefore, it is currently impractical to discuss whether KD can affect the survival or mortality of PC. Researchers should focus on the effects of combining KD with other therapies, such as chemotherapy or neoadjuvant therapy. We are eager to know whether the combination could decrease the tumor stage, reduce the distant metastasis, or even relieve the side effects of such kinds of treatments. Furthermore, high-quality randomized controlled trials should be taken into account to gain more evidence for bringing KD into clinical practice.
The Mediterranean Diet (MD)
The Composition of the MD
The MD originates in the food cultures of ancient civilizations that developed around the Mediterranean Basin, and this term is used today to describe the traditional dietary habits of countries neighboring the Mediterranean Sea, mainly Greece and southern Italy (76, 77). Its detailed components contain fruits, vegetables, and whole grains in every meal. Olive oil, tree nuts, and seeds are consumed every day. The aim is to eat fish, seafood, and legume products at least twice a week. Meat and eggs are consumed infrequently and in small quantities, and processed meat and sweets are practically nonexistent (Figure 3) (78, 79). This diet contains 40% to 50% carbohydrates (approximately 80% coming from complex carbohydrates such as whole grain bread), 10% to 20% protein (particularly fish), and 30% to 40% fat (mainly from polyunsaturated ω-3 FAs) (80, 81). Higher adherence to the MD can extend lifespan and seems to have an inverse association with the risk of gastrointestinal cancers, including PC, by affecting gut microbes, hormone receptors, fat, obesity, and other aspects (82–84). However, some limitations could be found in these studies. First, the distinction between different food groups is imprecise and overlapping. For instance, the vegetable group includes beans, and the legume group contains peanuts that are often covered by the nut group. Second, many foods are not associated with the same category, although similar biologically active substances are present. It is difficult to classify them according to their specific effects (85). Therefore, we will discuss the bioactive compounds that have greater impacts on PC in the natural products in the MD rather than categorize them by the types or species.
ω-3 FAs and PC
Many epidemiological studies have accumulated evidence regarding the effect of ω-3 PUFAs, namely, α-linolenic acid, eicosapentaenoic acid (EPA), and docosahexaenoic acid, in cardiovascular disease, metabolic syndrome, cancer, and immune system disorders (86, 87). It is estimated that the minimum human requirements are 0.2% of daily energy intake for ω-3 PUFAs (88). The typical MD recommends consuming marine fish that provide ω-3 FAs and eating vegetables, nuts, and virgin olive oil to complement derived ω-3 FAs (89). Recent studies have shown that ω-3 PUFAs inhibit cancer cell growth in colorectal cancer (CRC) and cholangiocarcinoma (90, 91). Song et al. (92) found that ω-3 PUFAs inhibited PC growth by reducing the β-catenin expression and T cell factor/lymphoid-enhancing factor reporter activity. A study found that a 5% fish oil (FO)-supplemented diet rich in ω-3 FAs significantly suppressed tumor growth by inducing ROS accumulation and caspase-8-dependent cell death (93). In addition, the accumulated EPA in the pancreas was shown to concomitantly promote autophagy and diminish its ability to induce apoptotic cell death (94). The combination of EPA with an autophagy inhibitor may be a useful strategy in increasing the therapeutic effectiveness in PC. ω-3 FAs also manifest synergistically with chemotherapeutic agents and enhance tumor radiosensitivity (95). Two clinical trials found that gemcitabine plus an ω-3-rich lipid emulsion improved the response rate and disease control rate (96) and improved PC patient prognosis (97, 98). Another study investigated whether the application of ω-3 FAs significantly prolonged the median survival time (MST) of patients (99). Hering et al. (100) demonstrated that ω-3 FAs plus gemcitabine inhibited gemcitabine-induced NF-κB activation, restored apoptosis, and reduced mortality due to gemcitabine chemoresistance. Similar to the KD, ω-3 FAs can also improve the skeletal muscle mass of PC patients against cachexia and simultaneously reduce the side effects caused by chemotherapy (101). The mucositis induced by chemotherapy-induced peripheral neuropathy and intestinal microbial dysbiosis manifests as inflammation from the mouth to the anus and neuropathic pain, respectively (102, 103). These side effects may cause weight loss, generalized infection, and longer hospitalization times (104, 105). Barber et al. (106) demonstrated that taking FO-enriched nutritional supplements could stabilize the appetite and weight of PC patients. The evidence to date demonstrates that ω-3 FAs may decrease cancer risk by affecting genetic variants of inflammatory pathways, oxidative stress, and tumor apoptosis and are also a high potential strategy for the treatment of PC (107, 108). According to the recommendations from the Dietary Guidelines Advisory Committee in 2015, no upper limit was given for dietary fat intake, and saturated fatty acids should be replaced by PUFAs (109). Therefore, sticking to the MD is the right choice to consume adequate PUFAs in a daily diet.
Micronutrients and PC
Water-soluble vitamins, including folic acid and vitamin C, are well represented in the MD. Vitamin C, also known as ascorbic acid, acts as an antioxidant and is found mainly in citrus fruits, broccoli, spinach, cauliflower, and sweet and white potatoes (110). The combination of hydrogen peroxide and ascorbate in the extracellular fluid has been shown to result in the formation of ROS, which selectively kill tumor cells (111). A study showed that combinations of gemcitabine and vitamin C could inhibit PC tumor growth and demonstrated a gemcitabine dose-sparing effect, even with PC that was unresponsive to gemcitabine (112). Espey (113) and Monti (114) et al. revealed that PDAC patients tolerated the treatment well and showed a decrease in the size of tumors when the intravenous infusion of vitamin C was combined with gemcitabine. It is worth noting that the mechanisms of high-dose intravenous vitamin C are distinct from orally administered vitamin C. Intravenous vitamin C in pharmacologic doses can produce peak plasma concentrations that are several hundredfold higher than those from maximal oral doses (115). A high intake of dietary vitamin C mitigates the risk of PDAC from meat-derived mutagen exposure (116). Another water-soluble vitamin, folic acid, also known as vitamin B9, has a closer relationship with PC compared with other B vitamins. Chittiboyina et al. (117) found that the mean levels of folate in red blood cells were significantly lower in PC patients. Marley et al. (118) demonstrated that dietary folate intake was associated with a reduced PC risk.
Lipid-soluble vitamins are complemented by abundant provitamin A (α- and β-carotene, β-cryptoxanthin) found in yellow-orange-red fruits and vegetables (119). Several meta-analyses have investigated whether dietary vitamin A has an inverse association with PC risk (120, 121). However, the single prospective study showed no association between vitamin A intake and the risk of PC (122). Another lipid-soluble vitamin, vitamin D, is found in oily fish (123). It participates in antitumor effects through the AMPK and PI3K/Akt pathways in PC and inhibits the expression of the cell cycle-related proteins CDKN1A (p21) and CDK1 (124, 125). Altieri et al. (126) found that the immunomodulatory and endocrine regulatory effects of vitamin D are related to the development of diabetes and PC. Camara et al. (127) believed that a lack of sunlight caused vitamin D deficiency, which improved the mortality of PC. Moreover, higher concentrations of vitamin D are suggested to increase the risk of PC (128).
Vitamin E is a group of naturally occurring and potent antioxidants that includes 4 tocopherols and 4 tocotrienols (α-, β-, δ-, and γ-) (129). It has been discovered to inhibit breast cancer, colon cancer, lung cancer, and hepatocellular carcinomas (130, 131). The main sources are vegetable oils and nuts that are frequently consumed in the MD (119). Husain et al. (132) found that tocotrienols inhibit PC by eliminating NF-κB activity. In addition, taking 200 to 1600 mg/day vitamin E δ-tocotrienol before surgery can significantly induce apoptosis in neoplastic cells (133).
Selenium (Se) intake from aquatic and dairy products is seriously insufficient in almost all regions (134). However, large amounts of whole grains, which are good sources of Se, including whole meal pasta and wheat, sourdough bread, stoneground wheat bread, and brown rice, are recommended by the MD (135). Higher levels of Se appeared protective for both mutated and KRAS wild-type PDAC (136). Amaral et al. (137) found that high concentrations of Se were inversely associated with the risk of exocrine PC. Two studies have shown that supplementing Se during cisplatin therapy reduces myelosuppression and nephrotoxicity, suggesting that optimal levels of Se could aid in the toxicity profile related to chemotherapy (138, 139).
Current research on the relationship between these micronutrients and PC does not show a strong association. Hence, a more meaningful approach would be to incorporate certain trace elements into a risk stratification scheme for the selection and surveillance control examination to complement existing PC screening and diagnostic procedures and improve the overall design of future micronutrient clinical trials for PC.
Polyphenols and PC
Dietary antioxidants counteract oxidation processes and prevent chronic diseases related to oxidative stress (140). Natural antioxidants from plants, including vitamins and phenolic compounds, were suggested to suppress PC, breast cancer, and prostate cancer (141, 142). The phenolic compounds that are closely related to PC include curcumin, resveratrol (RV), and sulforaphane (SFN) (143). Some studies suggest that adding more plant-based condiments, such as curcumin, to the diet could reduce sodium intake (144). Dhillon et al. (145) found that peripheral mononuclear cells isolated from curcumin-treated patients showed reduced activation of NF-κB and that tolerance to gemcitabine and erlotinib was increased. Another clinical trial showed that curcumin improved the MST of gemcitabine-resistant patients and overall survival (146). Li et al. (147) found that SFN could regulate the self-renewal of PC stem cells through the modulation of the Hedgehog pathway. Naumann et al. (148) observed that, when combined with RT, it exerts more distinct DNA damage and pancreatic tumor growth inhibition. In addition, the most striking observation was the ability of SFN to potentiate the activity of several classes of anticancer agents, including paclitaxel, docetaxel, and gemcitabine, through additive and synergistic effects (149). SFN has been explored as a plant-derived histone deacetylase inhibitor in the treatment of PC (150). It is hoped that the novel approach circumvents herculean cancer chemoresistance and alleviates toxicity, the main drawback of monotherapy (151).
RV has been detected in more than 70 plant species, including red grapes, peanuts, and berries (152). It has been shown to target various signaling pathways in PC, such as Hedgehog, leukotriene A4 hydrolase, macrophage inhibitory cytokine-1, and STAT3 (153). Cui et al. (154) demonstrated that RV inhibited the proliferation of PC cells by inducing apoptotic cell death and enhanced the sensitivity to gemcitabine (155). Furthermore, the special effect of RV improves the DNA-damaging effect of paclitaxel in epididymal sperm. This discovery can benefit male cancer patients (156). Verdura et al. (157) demonstrated that the ability of RV to directly target PD-L1 interferes with its stability and trafficking, ultimately impeding its targeting to the cancer cell plasma membrane. However, it did not appear to interfere with blood cell count or splenocyte or macrophage function (158). Available evidence suggests that RV combined with anti-PD-1/PD-L1 blockade treatment can improve the effectiveness of breast cancer immunotherapy (159). This unforeseen immunomodulatory mechanism of RV might illuminate new approaches to restore T-cell function by targeting the PD-1/PD-L1 immunologic checkpoint with natural polyphenols and provide a new perspective for PC immunotherapy.
Red Meat and PC
Meat intake was considered as a risk factor for PC, but more epidemiological studies should be further explored (160). Some studies believe that its carcinogenicity comes from animal carcinogens, such as heterocyclic amines (HCAs) and benzo(a)pyrene (BaP) (161). However, other studies believe that advanced glycation end products (AGEs) are the main carcinogens. AGEs are a heterogeneous group of compounds present in uncooked foods as well as in food cooked at high temperatures (162). AGEs are associated with insulin resistance, oxidative stress, PC, and chronic inflammation in patients with diabetes (163). AGEs markedly accelerated tumor development in a mouse model of Kras-driven PDAC (164). Jiao et al. (165) observed that higher consumption of red meat increased the risk of PC. Larsson et al. (166) suggested that substituting poultry for red meat might reduce the risk of PC. In addition, some studies refuted the red meat-cancer causality and believed that highly processed meat has a higher risk of causing cancer (167). Thus, future prospective studies should also assess cooking practices and processing methods concerning the risk of PC, not just the type of meat. The serious issue with MD studies is that most research focuses on the prevention rather than the treatment of PC (168). Future studies should shift from using the MD for the prevention of PC to the treatment of PC patients. A recent study proposed a D. I. E. T project to identify the best diet for immunotherapy enhancement against tumors and discussed the dietary patterns affecting immune function. This project proposed adhering to a healthy diet such as the MD, vegetarian, or KD. The proposed supplements are suitable for application in immunotherapy, including ω-3 FAs and polyphenols, which means there is potential for diet in immunotherapy (169).
The Low-Sugar Diet
Increasing epidemiological evidence has indicated the association between diabetes and pancreatic malignancy, but the mechanism is still unclear (170). Emerging molecular studies suggest that hyperglycemia, obesity-associated hyperinsulinemia, and chronic inflammation in diabetes might be involved in PC proliferation and metastasis (171). The hallmark characteristic of type 2 diabetes (T2D) is hyperglycemia, but hyperglycemia can also occur at a pre-T2D level with a higher-than-normal blood sugar (BG) level and not reach the threshold for diagnosing T2D (172). Hyperglycemic episodes increase the risk of adverse events and outcomes for cancer patients with or without T2D (173). Several studies have shown that high glucose activates epidermal growth factor receptor (EGFR), which participates in PC progression (174–176).
The glycemic index (GI) and the glycemic load (GL) were proposed as measurements of carbohydrate quality and quantity (177). A low-GI and an energy-restricted diet containing moderate amounts of carbohydrates may reduce body weight and control glucose and insulin metabolism (178). Those following the low-sugar and low-GI diet should consume large amounts of vegetables rich in fiber and phytonutrients (179), avoid the intake of carbohydrate-rich foods, such as bread, noodles, pasta, and starchy vegetables, as in the Western diet (180, 181), and cakes, candy, biscuits, and sugar-sweetened beverages are forbidden (182). Turati et al. (183) found that high-GL diets may have an adverse effect on blood glucose levels, insulin, and IGFs, resulting in an increased risk of PC. Hu et al. (184) believed that the consumption of sugar, candy, honey, and jam was positively associated with PC. Larsson et al. (185) found that the consumption of added sugar, soft drinks, and sweetened fruit soups or stewed fruit was positively associated with the risk of PC. Other studies have shown that diets high in fructose and sucrose increase the risk of PC, especially for women with a high body mass index or low levels of physical activity (186, 187).
Approximately more than 50% of all patients with PDAC develop diabetes before their cancer diagnosis (188). Diabetes or impaired glucose tolerance is present in more than two-thirds of PC patients (189). The relationship between hyperglycemia, diabetes, and PC is getting closer. Hyperglycemia has been demonstrated to promote the perineural invasion of PC and liver metastasis in vivo (190, 191). Kesh et al. (192) observed that microbial dysbiosis caused by hyperglycemia was associated with increased resistance to chemotherapeutic compounds in a T2D animal model. Furthermore, high glucose may promote immune escape under a hyperglycemic tumor microenvironment in PC (193). A high-sugar diet brings adverse effects and leads to a poor prognosis. However, some researchers have suggested that a high sugar intake will not increase the risk of PC, with the limitation that patients without diabetes were enrolled in this study (194). Therefore, future studies should explore the relationship between diabetes, abnormal BG, and PC. Meanwhile, a low-sugar diet is more specific and standardized and plays a more targeted role in diabetic patients and people with abnormal BG.
Conclusion
The existing studies on diet and PC are encouraging, but research is still in its infancy (Table 1). Research comparing these three diets was even rarer. However, these three diets have a lot in common. The low-sugar diet recommends rejecting refined carbohydrates and excessive sugar intake, which also includes overprocessed meat (180–182). In addition, a low-sugar diet is a low-GI diet, and some researchers believe that the MD is also a low-GI diet (78). In terms of the effect of hyperglycemia on tumors, the mechanism was consistent across these three diets. The mechanism of the KD involves increased oxidative stress and ROS production, which are all related to the hyperglycemic state of the tumor, similar to a low-sugar diet (31–33, 193). Furthermore, views about the types of fatty acids in all three diets were basically the same (15, 16, 21, 80, 81). There have even been studies combining the KD with ω-3 FAs (54).
Although diet has been used to improve immunotherapy and enhance the efficacy of chemotherapy or RT, it is still regarded as a nutritional supplement. Tóth et al. (72–75) have pointed out that nonsurgical therapies might hinder the effects of metabolic therapies and might even lead to the tumor progression in several studies. Therefore, the consideration of using diet therapy as a stand-alone treatment may bring unexpected results. The depth of knowledge about the relationship between diet and cancer is far from sufficient. How these diets bring changes at the cellular level or whether these diets can reduce the risk of PC in the entire family by reducing the risk of the first-generation population and then through genetic variation is still obscure. More clinical trials and more detailed multi-sample studies are needed to better explain the dietary patterns in tumor prevention and treatment. Future research should focus on combining diets with treatment and better popularizing beneficial diets.
Author Contributions
This review was drafted by YZ and designed by LL. TZ, WY, HZC, XG, GL, HC, and YW conducted the literature search and identified eligible studies. YZ and LL wrote and critically revised the manuscript. BS supervised and reviewed the manuscript. All authors contributed to the article and approved the submitted version.
Funding
This work was supported by The National Natural Science Foundation of China (81800572, 81670583, 81871974, 81702384).
Conflict of Interest
The authors declare that the research was conducted in the absence of any commercial or financial relationships that could be construed as a potential conflict of interest.
References
1. Ren H, Wang CF. Diagnosis, Treatment and Prognosis Evaluation of Pancreatic Cancer by Microrna. Zhonghua Zhong Liu Za Zhi (2020) 42(11):903–6. doi: 10.3760/cma.j.cn112152-20190313-00152 Chinese.
2. Haller SD, Monaco ML, Essani K. The Present Status of Immuno-Oncolytic Viruses in the Treatment of Pancreatic Cancer. Viruses (2020) 12(11):1318. doi: 10.3390/v12111318
3. Mehra K, Berkowitz A, Sanft T. Diet, Physical Activity, and Body Weight in Cancer Survivorship. Med Clin North Am (2017) 101(6):1151–65. doi: 10.1016/j.mcna
4. Lien EC, Vander Heiden MG. A Framework for Examining How Diet Impacts Tumour Metabolism. Nat Rev Cancer (2019) 19(11):651–61. doi: 10.1038/s41568-019-0198-5
5. Zheng J, Guinter MA, Merchant AT, Wirth MD, Zhang J, Stolzenberg-Solomon RZ, et al. Dietary Patterns and Risk of Pancreatic Cancer: A Systematic Review. Nutr Rev (2017) 75(11):883–908. doi: 10.1093/nutrit/nux038
6. Le HH, Johnson EL. Going Keto? Say βHB-Ye Bye to Your Gut Bifidobacteria. Cell Host Microbe (2020) 28(1):3–5. doi: 10.1016/j.chom.2020.06.012
7. Schwartz K, Chang HT, Nikolai M, Pernicone J, Rhee S, Olson K, et al. Treatment of Glioma Patients With Ketogenic Diets: Report of Two Cases Treated With an IRB-approved Energy-Restricted Ketogenic Diet Protocol and Review of the Literature. Cancer Metab (2015) 3:3. doi: 10.1186/s40170-015-0129-1
8. Martin-McGill KJ, Bresnahan R, Levy RG, Cooper PN. Ketogenic Diets for Drug-Resistant Epilepsy. Cochrane Database Syst Rev (2020) 6:CD001903. doi: 10.1002/14651858.CD001903.pub5
9. Weber DD, Aminzadeh-Gohari S, Tulipan J, Catalano L, Feichtinger RG, Kofler B. Ketogenic Diet in the Treatment of Cancer - Where do We Stand? Mol Metab (2020) 33:102–21. doi: 10.1016/j.molmet.2019.06.026
10. Kossoff EH, McGrogan JR, Freeman JM. Benefits of an All-Liquid Ketogenic Diet. Epilepsia (2004) 45(9):1163. doi: 10.1111/j.0013-9580.2004.18504.x
11. van der Louw E, Olieman J, Poley MJ, Wesstein T, Vehmeijer F, Catsman-Berrevoets C, et al. Outpatient Initiation of the Ketogenic Diet in Children With Pharmacoresistant Epilepsy: An Effectiveness, Safety and Economic Perspective. Eur J Paediatr Neurol (2019) 23(5):740–8. doi: 10.1016/j.ejpn.2019.06.001
12. Lyons L, Schoeler NE, Langan D, Cross JH. Use of Ketogenic Diet Therapy in Infants With Epilepsy: A Systematic Review and Meta-Analysis. Epilepsia (2020) 61(6):1261–81. doi: 10.1111/epi.16543
13. Kumar S, Behl T, Sachdeva M, Sehgal A, Kumari S, Kumar A, et al. Implicating the Effect of Ketogenic Diet as a Preventive Measure to Obesity and Diabetes Mellitus. Life Sci (2021) 264:118661. doi: 10.1016/j.lfs.2020.118661
14. O’Neill BJ. Effect of Low-Carbohydrate Diets on Cardiometabolic Risk, Insulin Resistance, and Metabolic Syndrome. Curr Opin Endocrinol Diabetes Obes (2020) 27(5):301–7. doi: 10.1097/MED.0000000000000569
15. Shingler E, Perry R, Mitchell A, England C, Perks C, Herbert G, et al. Dietary Restriction During the Treatment of Cancer: Results of a Systematic Scoping Review. BMC Cancer (2019) 19(1):811. doi: 10.1186/s12885-019-5931-7
16. Hashim SA, VanItallie TB. Ketone Body Therapy: From the Ketogenic Diet to the Oral Administration of Ketone Ester. J Lipid Res (2014) 55(9):1818–26. doi: 10.1194/jlr.R046599
17. Klement RJ, Champ CE, Otto C, Kämmerer U. Anti-Tumor Effects of Ketogenic Diets in Mice: A Meta-Analysis. PloS One (2016) 11(5):e0155050. doi: 10.1371/journal.pone.0155050
18. Charlot A, Conrad O, Zoll J. Le Régime Cétogène : Une Stratégie Alimentaire Efficace En Complément Des Traitements Contre Le Cancer ? [Ketogenic Diet: A New Nutritional Strategy for Cancer Therapy?]. Biol Aujourdhui (2020) 214(3-4):115–23. doi: 10.1051/jbio/2020014 French.
19. Wang QQ, Li MX, Li C, Gu XX, Zheng MZ, Chen LX, et al. Natural Products and Derivatives Targeting at Cancer Energy Metabolism: A Potential Treatment Strategy. Curr Med Sci (2020) 40(2):205–17. doi: 10.1007/s11596-020-2165-5
20. Gillies RJ, Robey I, Gatenby RA. Causes and Consequences of Increased Glucose Metabolism of Cancers. J Nucl Med (2008) 49 Suppl2:24S–42S. doi: 10.2967/jnumed.107.047258
21. Feng S, Wang H, Liu J, Aa J, Zhou F, Wang G. Multi-Dimensional Roles of Ketone Bodies in Cancer Biology: Opportunities for Cancer Therapy. Pharmacol Res (2019) 150:104500. doi: 10.1016/j.phrs.2019.104500
22. Seyfried TN, Kiebish MA, Marsh J, Shelton LM, Huysentruyt LC, Mukherjee P. Metabolic Management of Brain Cancer. Biochim Biophys Acta (2011) 1807(6):577–94. doi: 10.1016/j.bbabio.2010.08.009
23. Nebeling LC, Miraldi F, Shurin SB, Lerner E. Effects of a Ketogenic Diet on Tumor Metabolism and Nutritional Status in Pediatric Oncology Patients: Two Case Reports. J Am Coll Nutr (1995) 14(2):202–8. doi: 10.1080/07315724.1995.10718495
24. Otto C, Kaemmerer U, Illert B, Muehling B, Pfetzer N, Wittig R, et al. Growth of Human Gastric Cancer Cells in Nude Mice is Delayed by a Ketogenic Diet Supplemented With Omega-3 Fatty Acids and Medium-Chain Triglycerides. BMC Cancer (2008) 8:122. doi: 10.1186/1471-2407-8-122
25. Kim HS, Masko EM, Poulton SL, Kennedy KM, Pizzo SV, Dewhirst MW, et al. Carbohydrate Restriction and Lactate Transporter Inhibition in a Mouse Xenograft Model of Human Prostate Cancer. BJU Int (2012) 110(7):1062–9. doi: 10.1111/j.1464-410X.2012.10971.x
26. Zhou W, Mukherjee P, Kiebish MA, Markis WT, Mantis JG, Seyfried TN. The Calorically Restricted Ketogenic Diet, an Effective Alternative Therapy for Malignant Brain Cancer. Nutr Metab (Lond) (2007) 4:5. doi: 10.1186/1743-7075-4-5
27. Hursting SD, Dunlap SM, Ford NA, Hursting MJ, Lashinger LM. Calorie Restriction and Cancer Prevention: A Mechanistic Perspective. Cancer Metab (2013) 1(1):10. doi: 10.1186/2049-3002-1-10
28. Klement RJ, Fink MK. Dietary and Pharmacological Modification of the Insulin/IGF-1 System: Exploiting the Full Repertoire Against Cancer. Oncogenesis (2016) 5(2):e193. doi: 10.1038/oncsis.2016.2
29. Fine EJ, Segal-Isaacson CJ, Feinman RD, Herszkopf S, Romano MC, Tomuta N, et al. Targeting Insulin Inhibition as a Metabolic Therapy in Advanced Cancer: A Pilot Safety and Feasibility Dietary Trial in 10 Patients. Nutrition (2012) 28(10):1028–35. doi: 10.1016/j.nut.2012.05.001
30. Husain Z, Huang Y, Seth P, Sukhatme VP. Tumor-Derived Lactate Modifies Antitumor Immune Response: Effect on Myeloid-Derived Suppressor Cells and NK Cells. J Immunol (2013) 191(3):1486–95. doi: 10.4049/jimmunol.1202702
31. Barrea L, Caprio M, Tuccinardi D, Moriconi E, Di Renzo L, Muscogiuri G, et al. Obesity Programs of Nutrition, Education, Research and Assessment (OPERA) Group. Could Ketogenic Diet “Starve” Cancer? Emerging Evidence. Crit Rev Food Sci Nutr (2020) 4:1–22. doi: 10.1080/10408398.2020.1847030
32. Hamanaka RB, Chandel NS. Mitochondrial Reactive Oxygen Species Regulate Cellular Signaling and Dictate Biological Outcomes. Trends Biochem Sci (2010) 35(9):505–13. doi: 10.1016/j.tibs.2010.04.002
33. Fruehauf JP, Meyskens FL Jr. Reactive Oxygen Species: A Breath of Life or Death? Clin Cancer Res (2007) 13(3):789–94. doi: 10.1158/1078-0432.CCR-06-2082
34. Stafford P, Abdelwahab MG, Kim DY, Preul MC, Rho JM, Scheck AC. The Ketogenic Diet Reverses Gene Expression Patterns and Reduces Reactive Oxygen Species Levels When Used as an Adjuvant Therapy for Glioma. Nutr Metab (Lond) (2010) 7:74. doi: 10.1186/1743-7075-7-74
35. Maalouf M, Rho JM, Mattson MP. The Neuroprotective Properties of Calorie Restriction, the Ketogenic Diet, and Ketone Bodies. Brain Res Rev (2009) 59(2):293–315. doi: 10.1016/j.brainresrev.2008.09.002
36. Stark AP, Sacks GD, Rochefort MM, Donahue TR, Reber HA, Tomlinson JS, et al. Long-Term Survival in Patients With Pancreatic Ductal Adenocarcinoma. Surgery (2016) 159(6):1520–7. doi: 10.1016/j.surg.2015.12.024
37. Gilliland TM, Villafane-Ferriol N, Shah KP, Shah RM, Tran Cao HS, Massarweh NN, et al. Nutritional and Metabolic Derangements in Pancreatic Cancer and Pancreatic Resection. Nutrients (2017) 9(3):243. doi: 10.3390/nu9030243
38. Ok JH, Lee H, Chung HY, Lee SH, Choi EJ, Kang CM, et al. The Potential Use of a Ketogenic Diet in Pancreatobiliary Cancer Patients After Pancreatectomy. Anticancer Res (2018) 38(11):6519–27. doi: 10.21873/anticanres.13017
39. Kang CM, Yun B, Kim M, Song M, Kim YH, Lee SH, et al. Postoperative Serum Metabolites of Patients on a Low Carbohydrate Ketogenic Diet After Pancreatectomy for Pancreatobiliary Cancer: A Nontargeted Metabolomics Pilot Study. Sci Rep (2019) 9(1):16820. doi: 10.1038/s41598-019-53287-y
40. Bortesi L, Pesci A, Bogina G, Castelli P, Zamboni G. Ductal Adenocarcinoma of the Pancreas. Surg Pathol Clin (2011) 4(2):487–521. doi: 10.1016/j.path.2011.03.007
41. Tempero MA, Malafa MP, Al-Hawary M, Asbun H, Bain A, Behrman SW, et al. Pancreatic Adenocarcinoma, Version 2.2017, NCCN Clinical Practice Guidelines in Oncology. J Natl Compr Canc Netw (2017) 15(8):1028–61. doi: 10.6004/jnccn.2017.0131
42. Kieler M, Unseld M, Bianconi D, Schindl M, Kornek GV, Scheithauer W, et al. Impact of New Chemotherapy Regimens on the Treatment Landscape and Survival of Locally Advanced and Metastatic Pancreatic Cancer Patients. J Clin Med (2020) 9(3):648. doi: 10.3390/jcm9030648
43. Wang J, Yi J. Cancer Cell Killing Via ROS: To Increase or Decrease, That is the Question. Cancer Biol Ther (2008) 7(12):1875–84. doi: 10.4161/cbt.7.12.7067
44. Plotti F, Terranova C, Luvero D, Bartolone M, Messina G, Feole L, et al. Diet and Chemotherapy: The Effects of Fasting and Ketogenic Diet on Cancer Treatment. Chemotherapy (2020) 65(3-4):77–84. doi: 10.1159/000510839
45. İyikesici MS, Slocum AK, Slocum A, Berkarda FB, Kalamian M, Seyfried TN. Efficacy of Metabolically Supported Chemotherapy Combined With Ketogenic Diet, Hyperthermia, and Hyperbaric Oxygen Therapy for Stage IV Triple-Negative Breast Cancer. Cureus (2017) 9(7):e1445. doi: 10.7759/cureus.1445
46. Seyfried TN, Flores R, Poff AM, D’Agostino DP, Mukherjee P. Metabolic Therapy: A New Paradigm for Managing Malignant Brain Cancer. Cancer Lett (2015) 356(2 Pt A):289–300. doi: 10.1016/j.canlet.2014.07.015
47. Iyikesici MS. Long-Term Survival Outcomes of Metabolically Supported Chemotherapy With Gemcitabine-Based or FOLFIRINOX Regimen Combined With Ketogenic Diet, Hyperthermia, and Hyperbaric Oxygen Therapy in Metastatic Pancreatic Cancer. Langzeitüberlebens-Outcomes Der Metabolisch Unterstützten Chemotherapie Mit Gemcitabin Oder FOLFIRINOX in Kombination Mit Ketogener Ernährung, Hyperthermie Und Hyperbarer Sauerstofftherapie Beim Metastasierenden Pankreaskarzinom. Complement Med Res (2020) 27(1):31–9. doi: 10.1159/000502135
48. Zahra A, Fath MA, Opat E, Mapuskar KA, Bhatia SK, Ma DC, et al. Consuming a Ketogenic Diet While Receiving Radiation and Chemotherapy for Locally Advanced Lung Cancer and Pancreatic Cancer: The University of Iowa Experience of Two Phase 1 Clinical Trials. Radiat Res (2017) 187(6):743–54. doi: 10.1667/RR14668.1
49. Allen BG, Bhatia SK, Buatti JM, Brandt KE, Lindholm KE, Button AM, et al. Ketogenic Diets Enhance Oxidative Stress and Radio-Chemo-Therapy Responses in Lung Cancer Xenografts. Clin Cancer Res (2013) 19(14):3905–13. doi: 10.1158/1078-0432.CCR-12-0287
50. Abdelwahab MG, Fenton KE, Preul MC, Rho JM, Lynch A, Stafford P, et al. The Ketogenic Diet is an Effective Adjuvant to Radiation Therapy for the Treatment of Malignant Glioma. PloS One (2012) 7(5):e36197. doi: 10.1371/journal.pone.0036197
51. Klement RJ, Sweeney RA. Impact of a Ketogenic Diet Intervention During Radiotherapy on Body Composition: II. Protocol of a Randomised Phase I Study (KETOCOMP). Clin Nutr ESPEN (2016) 12:e1–6. doi: 10.1016/j.clnesp.2015.11.001
52. Talib WH. A Ketogenic Diet Combined With Melatonin Overcomes Cisplatin and Vincristine Drug Resistance in Breast Carcinoma Syngraft. Nutrition (2020) 72:110659. doi: 10.1016/j.nut.2019.110659
53. Morscher RJ, Aminzadeh-Gohari S, Feichtinger RG, Mayr JA, Lang R, Neureiter D, et al. Inhibition of Neuroblastoma Tumor Growth by Ketogenic Diet and/or Calorie Restriction in a CD1-Nu Mouse Model. PloS One (2015) 10(6):e0129802. doi: 10.1371/journal.pone.0129802
54. Hao GW, Chen YS, He DM, Wang HY, Wu GH, Zhang B. Growth of Human Colon Cancer Cells in Nude Mice is Delayed by Ketogenic Diet With or Without Omega-3 Fatty Acids and Medium-Chain Triglycerides. Asian Pac J Cancer Prev (2015) 16(5):2061–8. doi: 10.7314/apjcp.2015.16.5.2061
55. Sun Y, Zhang B, Han Y, Jiang Y, Zhuang Q, Gong Y, et al. Survey of Cachexia in Digestive System Cancer Patients and Its Impact on Clinical Outcomes. Zhonghua Wei Chang Wai Ke Za Zhi (2014) 17(10):968–71. doi: CNKI:SUN:ZWCW.0.2014-10-007
56. Shukla SK, Gebregiworgis T, Purohit V, Chaika NV, Gunda V, Radhakrishnan P, et al. Metabolic Reprogramming Induced by Ketone Bodies Diminishes Pancreatic Cancer Cachexia. Cancer Metab (2014) 2:18. doi: 10.1186/2049-3002-2-18
57. Greene DA, Varley BJ, Hartwig TB, Chapman P, Rigney M. A Low-Carbohydrate Ketogenic Diet Reduces Body Mass Without Compromising Performance in Powerlifting and Olympic Weightlifting Athletes. J Strength Cond Res (2018) 32(12):3373–82. doi: 10.1519/JSC.0000000000002904
58. Koutnik AP, Poff AM, Ward NP, DeBlasi JM, Soliven MA, Romero MA, et al. Ketone Bodies Attenuate Wasting in Models of Atrophy. J Cachexia Sarcopenia Muscle (2020) 10:1002. doi: 10.1002/jcsm.12554
59. Nakamura K, Tonouchi H, Sasayama A, Ashida K. A Ketogenic Formula Prevents Tumor Progression and Cancer Cachexia by Attenuating Systemic Inflammation in Colon 26 Tumor-Bearing Mice. Nutrients (2018) 10(2):206. doi: 10.3390/nu10020206
60. Baracos VE, Martin L, Korc M, Guttridge DC, Fearon KCH. Cancer-Associated Cachexia. Nat Rev Dis Primers (2018) 4:17105. doi: 10.1038/nrdp.2017.105
61. Flint TR, Fearon DT, Janowitz T. Connecting the Metabolic and Immune Responses to Cancer. Trends Mol Med (2017) 23(5):451–64. doi: 10.1016/j.molmed.2017.03.001
62. Flint TR, Janowitz T, Connell CM, Roberts EW, Denton AE, Coll AP, et al. Tumor-Induced IL-6 Reprograms Host Metabolism to Suppress Anti-Tumor Immunity. Cell Metab (2016) 24(5):672–84. doi: 10.1016/j.cmet.2016.10.010
63. Lettieri-Barbato D, Aquilano K. Pushing the Limits of Cancer Therapy: The Nutrient Game. Front Oncol (2018) 8:148. doi: 10.3389/fonc.2018.00148
64. Lussier DM, Woolf EC, Johnson JL, Brooks KS, Blattman JN, Scheck AC. Enhanced Immunity in a Mouse Model of Malignant Glioma is Mediated by a Therapeutic Ketogenic Diet. BMC Cancer (2016) 16:310. doi: 10.1186/s12885-016-2337-7
65. Zhang Y, Kurupati R, Liu L, Zhou XY, Zhang G, Hudaihed A, et al. Enhancing CD8+ T Cell Fatty Acid Catabolism Within a Metabolically Challenging Tumor Microenvironment Increases the Efficacy of Melanoma Immunotherapy. Cancer Cell (2017) 32(3):377–91.e9. doi: 10.1016/j.ccell.2017.08.004
66. Siska PJ, Rathmell JC. T Cell Metabolic Fitness in Antitumor Immunity. Trends Immunol (2015) 36(4):257–64. doi: 10.1016/j.it.2015.02.007
67. Mitchell T, Clarke L, Goldberg A, Bishop KS. Pancreatic Cancer Cachexia: The Role of Nutritional Interventions. Healthcare (Basel) (2019) 7(3):89. doi: 10.3390/healthcare7030089
68. Neal EG, Chaffe H, Schwartz RH, Lawson MS, Edwards N, Fitzsimmons G, et al. The Ketogenic Diet for the Treatment of Childhood Epilepsy: A Randomised Controlled Trial. Lancet Neurol (2008) 7(6):500–6. doi: 10.1016/S1474-4422(08)70092-9
69. Sremanakova J, Sowerbutts AM, Burden S. A Systematic Review of the Use of Ketogenic Diets in Adult Patients With Cancer. J Hum Nutr Diet (2018) 31(6):793–802. doi: 10.1111/jhn.12587
70. Ressel GW, American Cancer Society. American Cancer Society Releases Guidelines on Nutrition and Physical Activity for Cancer Prevention. Am Fam Physician (2002) 66(8):1555, 1559–60, 1562. doi: 10.1016/S0095-4543(02)00043-X
71. Kossoff EH. International Consensus Statement on Clinical Implementation of the Ketogenic Diet: Agreement, Flexibility, and Controversy. Epilepsia (2008) 49 Suppl 8:11–3. doi: 10.1111/j.1528-1167.2008.01823.x
72. Tóth C, Clemens Z. Treatment of Rectal Cancer With the Paleolithic Ketogenic Diet: A 24-Months Follow-Up. Am J Med Case Rep (2017) 5:205–16. doi: 10.12691/ajmcr-5-8-3
73. Tóth C, Clemens Z. Halted Progression of Soft Palate Cancer in a Patient Treated With the Paleolithic Ketogenic Diet Alone: A 20-Months Follow-Up. Am J Med Case Rep (2016) 4:288–92. doi: 10.12691/ajmcr-4-8-8
74. Tóth C, Schimmer M, Clemens Z. Complete Cessation of Recurrent Cervical Intraepithelial Neoplasia (CIN) by the Paleolithic Ketogenic Diet: A Case Report. J Cancer Res Treat (2018) 6:1–5. doi: 10.12691/jcrt-6-1-1
75. Tóth C, Dabóczi A, Chanrai M, Schimmer M, Horváth K, Clemens Z. 4-Year Long Progression-Free and Symptom-Free Survival of a Patient With Recurrent Glioblastoma Multiforme: A Case Report of the Paleolithic Ketogenic Diet (PKD) Used as a Stand-Alone Treatment After Failed Standard Oncotherapy. Preprints (2019). doi: 10.20944/preprints201912.0264.v2
76. Lăcătușu CM, Grigorescu ED, Floria M, Onofriescu A, Mihai BM. The Mediterranean Diet: From an Environment-Driven Food Culture to an Emerging Medical Prescription. Int J Environ Res Public Health (2019) 16(6):942. doi: 10.3390/ijerph16060942
77. Dernini S. The Erosion and the Renaissance of the Mediterranean Diet: A Sustainable Cultural Resource. Quaderns la Mediterrània (2011) 16:75–82. doi: 10.1017/S1368980011002527
78. Davis C, Bryan J, Hodgson J, Murphy K. Definition of the Mediterranean Diet; a Literature Review. Nutrients (2015) 7(11):9139–53. doi: 10.3390/nu7115459
79. Hernáez Á, Estruch R. The Mediterranean Diet and Cancer: What Do Human and Molecular Studies Have to Say About it? Nutrients (2019) 11(9):2155. doi: 10.3390/nu11092155
80. Urquiaga I, Echeverría G, Dussaillant C, Rigotti A. Origen, Componentes Y Posibles Mecanismos De Acción De La Dieta Mediterránea. Rev Med Chil (2017) 145(1):85–95. doi: 10.4067/S0034-98872017000100012
81. Martínez-González MA, Salas-Salvadó J, Estruch R, Corella D, Fitó M, Ros E. Benefits of the Mediterranean Diet: Insights From the PREDIMED Study. Prog Cardiovasc Dis (2015) 58(1):50–60. doi: 10.1016/j.pcad.2015.04.003
82. Schwingshackl L, Schwedhelm C, Galbete C, Hoffmann G. Adherence to Mediterranean Diet and Risk of Cancer: An Updated Systematic Review and Meta-Analysis. Nutrients (2017) 9(10):1063. doi: 10.3390/nu9101063
83. Bosetti C, Turati F, Dal Pont A, Ferraroni M, Polesel J, Negri E, et al. The Role of Mediterranean Diet on the Risk of Pancreatic Cancer. Br J Cancer (2013) 109(5):1360–6. doi: 10.1038/bjc.2013.345
84. Zhao Z, Yin Z, Pu Z, Zhao Q. Association Between Consumption of Red and Processed Meat and Pancreatic Cancer Risk: A Systematic Review and Meta-Analysis. Clin Gastroenterol Hepatol (2017) 15(4):486–93.e10. doi: 10.1016/j.cgh.2016.09.143
85. D’Alessandro A, Lampignano L, De Pergola G. Mediterranean Diet Pyramid: A Proposal for Italian People. A Systematic Review of Prospective Studies to Derive Serving Sizes. Nutrients (2019) 11(6):1296. doi: 10.3390/nu11061296
86. De Caterina R. N-3 Fatty Acids in Cardiovascular Disease. N Engl J Med (2011) 364(25):2439–50. doi: 10.1056/NEJMra1008153
87. Kremmyda LS, Tvrzicka E, Stankova B, Zak A. Fatty Acids as Biocompounds: Their Role in Human Metabolism, Health and Disease: A Review. Part 2: Fatty Acid Physiological Roles and Applications in Human Health and Disease. BioMed Pap Med Fac Univ Palacky Olomouc Czech Repub (2011) 155(3):195–218. doi: 10.5507/bp.2011.052
88. Liu X, Kris-Etherton PM, West SG, Lamarche B, Jenkins DJ, Fleming JA, et al. Effects of Canola and High-Oleic-Acid Canola Oils on Abdominal Fat Mass in Individuals With Central Obesity. Obesity (Silver Spring) (2016) 24(11):2261–8. doi: 10.1002/oby.21584
89. Scoditti E, Capurso C, Capurso A, Massaro M. Vascular Effects of the Mediterranean Diet-Part II: Role of Omega-3 Fatty Acids and Olive Oil Polyphenols. Vascul Pharmacol (2014) 63(3):127–34. doi: 10.1016/j.vph.2014.07.001
90. Calviello G, Resci F, Serini S, Piccioni E, Toesca A, Boninsegna A, et al. Docosahexaenoic Acid Induces Proteasome-Dependent Degradation of Beta-Catenin, Down-Regulation of Survivin and Apoptosis in Human Colorectal Cancer Cells Not Expressing COX-2. Carcinogenesis (2007) 28(6):1202–9. doi: 10.1093/carcin/bgl254
91. Lim K, Han C, Xu L, Isse K, Demetris AJ, Wu T. Cyclooxygenase-2-derived Prostaglandin E2 Activates Beta-Catenin in Human Cholangiocarcinoma Cells: Evidence for Inhibition of These Signaling Pathways by Omega 3 Polyunsaturated Fatty Acids. Cancer Res (2008) 68(2):553–60. doi: 10.1158/0008-5472.CAN-07-2295
92. Song KS, Jing K, Kim JS, Yun EJ, Shin S, Seo KS, et al. Omega-3-polyunsaturated Fatty Acids Suppress Pancreatic Cancer Cell Growth In Vitro and In Vivo Via Downregulation of Wnt/Beta-catenin Signaling. Pancreatology (2011) 11(6):574–84. doi: 10.1159/000334468
93. Kang KS, Wang P, Yamabe N, Fukui M, Jay T, Zhu BT. Docosahexaenoic Acid Induces Apoptosis in MCF-7 Cells In Vitro and In Vivo Via Reactive Oxygen Species Formation and Caspase 8 Activation. PloS One (2010) 5(4):e10296. doi: 10.1371/journal.pone.0010296
94. Fukui M, Kang KS, Okada K, Zhu BT. EPA, an Omega-3 Fatty Acid, Induces Apoptosis in Human Pancreatic Cancer Cells: Role of ROS Accumulation, Caspase-8 Activation, and Autophagy Induction. J Cell Biochem (2013) 114(1):192–203. doi: 10.1002/jcb.24354
95. Wendel M, Heller AR. Anticancer Actions of Omega-3 Fatty Acids–Current State and Future Perspectives. Anticancer Agents Med Chem (2009) 9(4):457–70. doi: 10.2174/1871520610909040457
96. Arshad A, Isherwood J, Mann C, Cooke J, Pollard C, Runau F, et al. Intravenous ω-3 Fatty Acids Plus Gemcitabine. JPEN J Parenter Enteral Nutr (2017) 41(3):398–403. doi: 10.1177/0148607115595221
97. Nakagawa T, Ma BY, Uemura K, Oka S, Kawasaki N, Kawasaki T. Role of Mannan-Binding Protein, MBP, in Innate Immunity. Anticancer Res (2003) 23(6a):4467–71. doi: 10.1007/s10625-005-0227-x
98. Arshad A, Chung W, Isherwood J, Steward W, Metcalfe M, Dennison A. Restoration of Mannose-Binding Lectin Complement Activity is Associated With Improved Outcome in Patients With Advanced Pancreatic Cancer Treated With Gemcitabine and Intravenous ω-3 Fish Oil. JPEN J Parenter Enteral Nutr (2014) 38(2):214–9. doi: 10.1177/0148607113476304
99. Isherwood J, Arshad A, Chung WY, Runau F, Cooke J, Pollard C, et al. Myeloid Derived Suppressor Cells are Reduced and T Regulatory Cells Stabilised in Patients With Advanced Pancreatic Cancer Treated With Gemcitabine and Intravenous Omega 3. Ann Transl Med (2020) 8(5):172. doi: 10.21037/atm.2020.02.02
100. Hering J, Garrean S, Dekoj TR, Razzak A, Saied A, Trevino J, et al. Inhibition of Proliferation by Omega-3 Fatty Acids in Chemoresistant Pancreatic Cancer Cells. Ann Surg Oncol (2007) 14(12):3620–8. doi: 10.1245/s10434-007-9556-8
101. Abe K, Uwagawa T, Haruki K, Takano Y, Onda S, Sakamoto T, et al. Effects of ω-3 Fatty Acid Supplementation in Patients With Bile Duct or Pancreatic Cancer Undergoing Chemotherapy. Anticancer Res (2018) 38(4):2369–75. doi: 10.21873/anticanres.12485
102. Zhang AC, De Silva MEH, MacIsaac RJ, Roberts L, Kamel J, Craig JP, et al. Omega-3 Polyunsaturated Fatty Acid Oral Supplements for Improving Peripheral Nerve Health: A Systematic Review and Meta-Analysis. Nutr Rev (2020) 78(4):323–41. doi: 10.1093/nutrit/nuz054
103. Watson H, Mitra S, Croden FC, Taylor M, Wood HM, Perry SL, et al. A Randomised Trial of the Effect of Omega-3 Polyunsaturated Fatty Acid Supplements on the Human Intestinal Microbiota. Gut (2018) 67(11):1974–83. doi: 10.1136/gutjnl-2017-314968
104. Keefe DM. Intestinal Mucositis: Mechanisms and Management. Curr Opin Oncol (2007) 19(4):323–7. doi: 10.1097/CCO.0b013e3281214412
105. Barasch A, Peterson DE. Risk Factors for Ulcerative Oral Mucositis in Cancer Patients: Unanswered Questions. Oral Oncol (2003) 39(2):91–100. doi: 10.1016/s1368-8375(02)00033-7
106. Barber MD, Fearon KC, Tisdale MJ, McMillan DC, Ross JA. Effect of a Fish Oil-Enriched Nutritional Supplement on Metabolic Mediators in Patients With Pancreatic Cancer Cachexia. Nutr Cancer (2001) 40(2):118–24. doi: 10.1207/S15327914NC402_7
107. Werner K, Küllenberg de Gaudry D, Taylor LA, Keck T, Unger C, Hopt UT, et al. Dietary Supplementation With n-3-fatty Acids in Patients With Pancreatic Cancer and Cachexia: Marine Phospholipids Versus Fish Oil - a Randomized Controlled Double-Blind Trial. Lipids Health Dis (2017) 16(1):104. doi: 10.1186/s12944-017-0495-5
108. Yurko-Mauro K, Van Elswyk M, Teo L. A Scoping Review of Interactions Between Omega-3 Long-Chain Polyunsaturated Fatty Acids and Genetic Variation in Relation to Cancer Risk. Nutrients (2020) 12(6):E1647. doi: 10.3390/nu12061647
109. Trumbo PR. Review of the Scientific Evidence Used for Establishing US Policies on Added Sugars. Nutr Rev (2019) 3:nuz014. doi: 10.1093/nutrit/nuz014
110. Gerber M. Biofactors in the Mediterranean Diet. Clin Chem Lab Med (2003) 41(8):999–1004. doi: 10.1515/CCLM.2003.153
111. Chen Q, Espey MG, Krishna MC, Mitchell JB, Corpe CP, Buettner GR, et al. Pharmacologic Ascorbic Acid Concentrations Selectively Kill Cancer Cells: Action as a Pro-Drug to Deliver Hydrogen Peroxide to Tissues. Proc Natl Acad Sci U S A (2005) 102(38):13604–9. doi: 10.1073/pnas.0506390102
112. Espey MG, Chen P, Chalmers B, Drisko J, Sun AY, Levine M, et al. Pharmacologic Ascorbate Synergizes With Gemcitabine in Preclinical Models of Pancreatic Cancer. Free Radic Biol Med (2011) 50(11):1610–9. doi: 10.1016/j.freeradbiomed.2011.03.007
113. Monti DA, Mitchell E, Bazzan AJ, Littman S, Zabrecky G, Yeo CJ, et al. Phase I Evaluation of Intravenous Ascorbic Acid in Combination With Gemcitabine and Erlotinib in Patients With Metastatic Pancreatic Cancer. PloS One (2012) 7(1):e29794. doi: 10.1371/journal.pone.0029794
114. Welsh JL, Wagner BA, van’t Erve TJ, Zehr PS, Berg DJ, Halfdanarson TR, et al. Pharmacological Ascorbate With Gemcitabine for the Control of Metastatic and Node-Positive Pancreatic Cancer (PACMAN): Results From a Phase I Clinical Trial. Cancer Chemother Pharmacol (2013) 71(3):765–75. doi: 10.1007/s00280-013-2070-8
115. Padayatty SJ, Sun H, Wang Y, Riordan HD, Hewitt SM, Katz A, et al. Vitamin C Pharmacokinetics: Implications for Oral and Intravenous Use. Ann Intern Med (2004) 140(7):533–7. doi: 10.7326/0003-4819-140-7-200404060-00010
116. Li D, Tang H, Wei P, Zheng J, Daniel CR, Hassan MM. Vitamin C and Vitamin E Mitigate the Risk of Pancreatic Ductal Adenocarcinoma From Meat-Derived Mutagen Exposure in Adults in a Case-Control Study. J Nutr (2019) 149(8):1443–50. doi: 10.1093/jn/nxz081
117. Chittiboyina S, Chen Z, Chiorean EG, Kamendulis LM, Hocevar BA. The Role of the Folate Pathway in Pancreatic Cancer Risk. PloS One (2018) 13(2):e0193298. doi: 10.1371/journal.pone.0193298
118. Marley AR, Fan H, Hoyt ML, Anderson KE, Zhang J. Intake of Methyl-Related Nutrients and Risk of Pancreatic Cancer in a Population-Based Case-Control Study in Minnesota. Eur J Clin Nutr (2018) 72(8):1128–35. doi: 10.1038/s41430-018-0228-5
119. Bibiloni MDM, Julibert A, Bouzas C, Martínez-González MA, Corella D, Salas-Salvadó J, et al. Nut Consumptions as a Marker of Higher Diet Quality in a Mediterranean Population at High Cardiovascular Risk. Nutrients (2019) 11(4):754. doi: 10.3390/nu11040754
120. Zhang T, Chen H, Qin S, Wang M, Wang X, Zhang X, et al. The Association Between Dietary Vitamin A Intake and Pancreatic Cancer Risk: A Meta-Analysis of 11 Studies. Biosci Rep (2016) 36(6):e00414. doi: 10.1042/BSR20160341
121. Huang X, Gao Y, Zhi X, Ta N, Jiang H, Zheng J. Association Between Vitamin A, Retinol and Carotenoid Intake and Pancreatic Cancer Risk: Evidence From Epidemiologic Studies. Sci Rep (2016) 6:38936. doi: 10.1038/srep38936
122. Stolzenberg-Solomon RZ, Pietinen P, Taylor PR, Virtamo J, Albanes D. Prospective Study of Diet and Pancreatic Cancer in Male Smokers. Am J Epidemiol (2002) 155(9):783–92. doi: 10.1093/aje/155.9.783
123. Su Q, Rowley KG, Itsiopoulos C, O’Dea K. Identification and Quantitation of Major Carotenoids in Selected Components of the Mediterranean Diet: Green Leafy Vegetables, Figs and Olive Oil. Eur J Clin Nutr (2002) 56(11):1149–54. doi: 10.1038/sj.ejcn.1601472
124. Chiang KC, Chen TC. Vitamin D for the Prevention and Treatment of Pancreatic Cancer. World J Gastroenterol (2009) 15(27):3349–54. doi: 10.3748/wjg.15.3349
125. Li L, Shang F, Zhu Y, Sun Y, Sudi RS. Modulation of VDR and Cell Cycle-Related Proteins by Vitamin D in Normal Pancreatic Cells and Poorly Differentiated Metastatic Pancreatic Cancer Cells. Nutr Cancer (2019) 71(5):818–24. doi: 10.1080/01635581.2018.1521445
126. Altieri B, Grant WB, Della Casa S, Orio F, Pontecorvi A, Colao A, et al. Vitamin D and Pancreas: The Role of Sunshine Vitamin in the Pathogenesis of Diabetes Mellitus and Pancreatic Cancer. Crit Rev Food Sci Nutr (2017) 57(16):3472–88. doi: 10.1080/10408398.2015.1136922
127. Camara AB, Brandao IA. The Role of Vitamin D and Sunlight Incidence in Cancer. Anticancer Agents Med Chem (2019) 19(11):1418–36. doi: 10.2174/1389557519666190312123212
128. Weinstein SJ, Stolzenberg-Solomon RZ, Kopp W, Rager H, Virtamo J, Albanes D. Impact of Circulating Vitamin D Binding Protein Levels on the Association Between 25-Hydroxyvitamin D and Pancreatic Cancer Risk: A Nested Case-Control Study. Cancer Res (2012) 72(5):1190–8. doi: 10.1158/0008-5472.CAN-11-2950
129. Sylvester PW, Shah SJ. Mechanisms Mediating the Antiproliferative and Apoptotic Effects of Vitamin E in Mammary Cancer Cells. Front Biosci (2005) 10:699–709. doi: 10.2741/1565
130. Wada S, Satomi Y, Murakoshi M, Noguchi N, Yoshikawa T, Nishino H. Tumor Suppressive Effects of Tocotrienol In Vivo and In Vitro. Cancer Lett (2005) 229(2):181–91. doi: 10.1016/j.canlet.2005.06.036
131. Xu WL, Liu JR, Liu HK, Qi GY, Sun XR, Sun WG, et al. Inhibition of Proliferation and Induction of Apoptosis by Gamma-Tocotrienol in Human Colon Carcinoma HT-29 Cells. Nutrition (2009) 25(5):555–66. doi: 10.1016/j.nut.2008.10.019
132. Husain K, Francois RA, Yamauchi T, Perez M, Sebti SM, Malafa MP. Vitamin E δ-Tocotrienol Augments the Antitumor Activity of Gemcitabine and Suppresses Constitutive NF-κB Activation in Pancreatic Cancer. Mol Cancer Ther (2011) 10(12):2363–72. doi: 10.1158/1535-7163.MCT-11-0424
133. Springett GM, Husain K, Neuger A, Centeno B, Chen DT, Hutchinson TZ, et al. A Phase I Safety, Pharmacokinetic, and Pharmacodynamic Presurgical Trial of Vitamin E δ-Tocotrienol in Patients With Pancreatic Ductal Neoplasia. EBioMedicine (2015) 2(12):1987–95. doi: 10.1016/j.ebiom.2015.11.025
134. Wang J, Yang L, Li H, Li Y, Wei B. Dietary Selenium Intake Based on the Chinese Food Pagoda: The Influence of Dietary Patterns on Selenium Intake. Nutr J (2018) 17(1):50. doi: 10.1186/s12937-018-0358-6
135. Olza J, Aranceta-Bartrina J, González-Gross M, Ortega RM, Serra-Majem L, Varela-Moreiras G, et al. Reported Dietary Intake and Food Sources of Zinc, Selenium, and Vitamins a, E and C in the Spanish Population: Findings From the ANIBES Study. Nutrients (2017) 9(7):697. doi: 10.3390/nu9070697
136. Gómez-Tomás Á, Pumarega J, Alguacil J, Amaral AFS, Malats N, Pallarès N, et al. Concentrations of Trace Elements and KRAS Mutations in Pancreatic Ductal Adenocarcinoma. Environ Mol Mutagen (2019) 60(8):693–703. doi: 10.1002/em.22296
137. Amaral AF, Porta M, Silverman DT, Milne RL, Kogevinas M, Rothman N, et al. Pancreatic Cancer Risk and Levels of Trace Elements. Gut (2012) 61(11):1583–8. doi: 10.1136/gutjnl-2011-301086
138. Han X, Li J, Brasky TM, Xun P, Stevens J, White E, et al. Antioxidant Intake and Pancreatic Cancer Risk: The Vitamins and Lifestyle (VITAL) Study. Cancer (2013) 119(7):1314–20. doi: 10.1002/cncr.27936
139. Gray A, Dang BN, Moore TB, Clemens R, Pressman P. A Review of Nutrition and Dietary Interventions in Oncology. SAGE Open Med (2020) 8:2050312120926877. doi: 10.1177/2050312120926877
140. Cömert ED, Gökmen V. Evolution of Food Antioxidants as a Core Topic of Food Science for a Century. Food Res Int (2018) 105:76–93. doi: 10.1016/j.foodres.2017.10.056
141. Lourenço SC, Moldão-Martins M, Alves VD. Antioxidants of Natural Plant Origins: From Sources to Food Industry Applications. Molecules (2019) 24(22):4132. doi: 10.3390/molecules24224132
142. Aggarwal BB, Bhardwaj A, Aggarwal RS, Seeram NP, Shishodia S, Takada Y. Role of Resveratrol in Prevention and Therapy of Cancer: Preclinical and Clinical Studies. Anticancer Res (2004) 24(5A):2783–840. doi: 10.1016/j.fcr.2013.08.015
143. Subramaniam D, Kaushik G, Dandawate P, Anant S. Targeting Cancer Stem Cells for Chemoprevention of Pancreatic Cancer. Curr Med Chem (2018) 25(22):2585–94. doi: 10.2174/0929867324666170127095832
144. Rodrigues JF, Junqueira G, Gonçalves CS, Carneiro JD, Pinheiro AC, Nunes CA. Elaboration of Garlic and Salt Spice With Reduced Sodium Intake. Acad Bras Cienc (2014) 86(4):2065–75. doi: 10.1590/0001-3765201420130464
145. Dhillon N, Aggarwal BB, Newman RA, Wolff RA, Kunnumakkara AB, Abbruzzese JL, et al. Phase II Trial of Curcumin in Patients With Advanced Pancreatic Cancer. Clin Cancer Res (2008) 14(14):4491–9. doi: 10.1158/1078-0432.CCR-08-0024
146. Kanai M, Yoshimura K, Asada M, Imaizumi A, Suzuki C, Matsumoto S, et al. A Phase I/II Study of Gemcitabine-Based Chemotherapy Plus Curcumin for Patients With Gemcitabine-Resistant Pancreatic Cancer. Cancer Chemother Pharmacol (2011) 68(1):157–64. doi: 10.1007/s00280-010-1470-2
147. Li SH, Fu J, Watkins DN, Srivastava RK, Shankar S. Sulforaphane Regulates Self-Renewal of Pancreatic Cancer Stem Cells Through the Modulation of Sonic hedgehog-GLI Pathway. Mol Cell Biochem (2013) 373(1-2):217–27. doi: 10.1007/s11010-012-1493-6
148. Naumann P, Liermann J, Fortunato F, Schmid TE, Weber KJ, Debus J, et al. Sulforaphane Enhances Irradiation Effects in Terms of Perturbed Cell Cycle Progression and Increased DNA Damage in Pancreatic Cancer Cells. PloS One (2017) 12(7):e0180940. doi: 10.1371/journal.pone.0180940
149. Kamal MM, Akter S, Lin CN, Nazzal S. Sulforaphane as an Anticancer Molecule: Mechanisms of Action, Synergistic Effects, Enhancement of Drug Safety, and Delivery Systems. Arch Pharm Res (2020) 43(4):371–84. doi: 10.1007/s12272-020-01225-2
150. Paul B, Li Y, Tollefsbol TO. The Effects of Combinatorial Genistein and Sulforaphane in Breast Tumor Inhibition: Role in Epigenetic Regulation. Int J Mol Sci (2018) 19(6):1754. doi: 10.3390/ijms19061754
151. Ganai SA, Rashid R, Abdullah E, Altaf M. Plant Derived Inhibitor Sulforaphane in Combinatorial Therapy Against Therapeutically Challenging Pancreatic Cancer. Anticancer Agents Med Chem (2017) 17(3):365–73. doi: 10.2174/1871520616666160607004729
152. Athar M, Back JH, Tang X, Kim KH, Kopelovich L, Bickers DR, et al. Resveratrol: A Review of Preclinical Studies for Human Cancer Prevention. Toxicol Appl Pharmacol (2007) 224(3):274–83. doi: 10.1016/j.taap.2006.12.025
153. Mo W, Xu X, Xu L, Wang F, Ke A, Wang X, et al. Resveratrol Inhibits Proliferation and Induces Apoptosis Through the Hedgehog Signaling Pathway in Pancreatic Cancer Cell. Pancreatology (2011) 11(6):601–9. doi: 10.1159/000333542
154. Cui J, Sun R, Yu Y, Gou S, Zhao G, Wang C. Antiproliferative Effect of Resveratrol in Pancreatic Cancer Cells. Phytother Res (2010) 24(11):1637–44. doi: 10.1002/ptr.3157
155. Harikumar KB, Kunnumakkara AB, Sethi G, Diagaradjane P, Anand P, Pandey MK, et al. Resveratrol, a Multitargeted Agent, can Enhance Antitumor Activity of Gemcitabine In Vitro and in Orthotopic Mouse Model of Human Pancreatic Cancer. Int J Cancer (2010) 127(2):257–68. doi: 10.1002/ijc.25041
156. Ili P, Sari F, Bucak MN, Öztürk C, Güngör Ş, Ataman MB. DNA Damaging Effect of Paclitaxel in the Epididymal Sperms as a Chemotherapeutic Agent and Possible Remedies to Prevent This Effect: A Study on Reproductive Potential of Male Cancer Patients of Reproductive Age. Theriogenology (2019) 132:201–11. doi: 10.1016/j.theriogenology.2019.04.015
157. Verdura S, Cuyàs E, Cortada E, Brunet J, Lopez-Bonet E, Martin-Castillo B, et al. Resveratrol Targets PD-L1 Glycosylation and Dimerization to Enhance Antitumor T-cell Immunity. Aging (Albany NY) (2020) 12(1):8–34. doi: 10.18632/aging.102646
158. Soto BL, Hank JA, Darjatmoko SR, Polans AS, Yanke EM, Rakhmilevich AL, et al. Anti-Tumor and Immunomodulatory Activity of Resveratrol In Vitro and its Potential for Combining With Cancer Immunotherapy. Int Immunopharmacol (2011) 11(11):1877–86. doi: 10.1016/j.intimp.2011.07.019
159. Hsieh TC, Wu JM. Tumor PD-L1 Induction by Resveratrol/Piceatannol may Function as a Search, Enhance, and Engage (“See”) Signal to Facilitate the Elimination of “Cold, non-Responsive” Low PD-L1-Expressing Tumors by PD-L1 Blockade. Int J Mol Sci (2019) 20(23):5969. doi: 10.3390/ijms20235969
160. Anderson KE, Mongin SJ, Sinha R, Stolzenberg-Solomon R, Gross MD, Ziegler RG, et al. Pancreatic Cancer Risk: Associations With Meat-Derived Carcinogen Intake in the Prostate, Lung, Colorectal, and Ovarian Cancer Screening Trial (PLCO) Cohort. Mol Carcinog (2012) 51(1):128–37. doi: 10.1002/mc.20794
161. Stolzenberg-Solomon RZ, Cross AJ, Silverman DT, Schairer C, Thompson FE, Kipnis V, et al. Meat and Meat-Mutagen Intake and Pancreatic Cancer Risk in the NIH-AARP Cohort. Cancer Epidemiol Biomarkers Prev (2007) 16(12):2664–75. doi: 10.1158/1055-9965.EPI-07-0378
162. Ahmad S, Khan H, Siddiqui Z, Khan MY, Rehman S, Shahab U, et al. Ages, RAGEs and s-RAGE; Friend or Foe for Cancer. Semin Cancer Biol (2018) 49:44–55. doi: 10.1016/j.semcancer
163. Dariya B, Nagaraju GP. Advanced Glycation End Products in Diabetes, Cancer and Phytochemical Therapy. Drug Discov Today (2020) 5(9):1614–23. doi: 10.1016/j.drudis.2020.07.003
164. Menini S, Iacobini C, Vitale M, Pesce C, Pugliese G. Diabetes and Pancreatic Cancer-a Dangerous Liaison Relying on Carbonyl Stress. Cancers (Basel) (2021) 13(2):313. doi: 10.3390/cancers13020313
165. Jiao L, Stolzenberg-Solomon R, Zimmerman TP, Duan Z, Chen L, Kahle L, et al. Dietary Consumption of Advanced Glycation End Products and Pancreatic Cancer in the Prospective NIH-AARP Diet and Health Study. Am J Clin Nutr (2015) 101(1):126–34. doi: 10.3945/ajcn.114.098061
166. Larsson SC, Håkanson N, Permert J, Wolk A. Meat, Fish, Poultry and Egg Consumption in Relation to Risk of Pancreatic Cancer: A Prospective Study. Int J Cancer (2006) 118(11):2866–70. doi: 10.1002/ijc.21732
167. Santarelli RL, Pierre F, Corpet DE. Processed Meat and Colorectal Cancer: A Review of Epidemiologic and Experimental Evidence. Nutr Cancer (2008) 60(2):131–44. doi: 10.1080/01635580701684872
168. Di Daniele N, Noce A, Vidiri MF, Moriconi E, Marrone G, Annicchiarico-Petruzzelli M, et al. Impact of Mediterranean Diet on Metabolic Syndrome, Cancer and Longevity. Oncotarget (2017) 8(5):8947–79. doi: 10.18632/oncotarget.13553
169. Soldati L, Di Renzo L, Jirillo E, Ascierto PA, Marincola FM, De Lorenzo A. The Influence of Diet on Anti-Cancer Immune Responsiveness. J Transl Med (2018) 16(1):75. doi: 10.1186/s12967-018-1448-0
170. Song S, Wang B, Zhang X, Hao L, Hu X, Li Z, et al. Long-Term Diabetes Mellitus Is Associated With an Increased Risk of Pancreatic Cancer: A Meta-Analysis. PloS One (2015) 10(7):e0134321. doi: 10.1371/journal.pone.0134321
171. Er KC, Hsu CY, Lee YK, Huang MY, Su YC. Effect of Glycemic Control on the Risk of Pancreatic Cancer: A Nationwide Cohort Study. Medicine (Baltimore) (2016) 95(24):e3921. doi: 10.1097/MD.0000000000003921
172. American Diabetes Association. 2. Classification and Diagnosis of Diabetes: Standards of Medical Care in Diabetes-2020. Diabetes Care (2020) 43(Suppl 1):S14–31. doi: 10.2337/dc20-S002
173. Hammer M, Storey S, Hershey DS, Brady VJ, Davis E, Mandolfo N, et al. Hyperglycemia and Cancer: A State-of-the-Science Review. Oncol Nurs Forum (2019) 46(4):459–72. doi: 10.1188/19.ONF.459-472
174. Han L, Ma Q, Li J, Liu H, Li W, Ma G, et al. High Glucose Promotes Pancreatic Cancer Cell Proliferation Via the Induction of EGF Expression and Transactivation of EGFR. PloS One (2011) 6(11):e27074. doi: 10.1371/journal.pone.0027074
175. Liu H, Ma Q, Li J. High Glucose Promotes Cell Proliferation and Enhances GDNF and RET Expression in Pancreatic Cancer Cells. Mol Cell Biochem (2011) 347(1-2):95–101. doi: 10.1007/s11010-010-0617-0
176. Veit C, Genze F, Menke A, Hoeffert S, Gress TM, Gierschik P, et al. Activation of Phosphatidylinositol 3-Kinase and Extracellular Signal-Regulated Kinase is Required for Glial Cell Line-Derived Neurotrophic Factor-Induced Migration and Invasion of Pancreatic Carcinoma Cells. Cancer Res (2004) 64(15):5291–300. doi: 10.1158/0008-5472.CAN-04-1112
177. Sadeghi A, Sadeghi O, Khodadost M, Pirouzi A, Hosseini B, Saedisomeolia A. Dietary Glycemic Index and Glycemic Load and the Risk of Prostate Cancer: An Updated Systematic Review and Dose-Response Meta-Analysis. Nutr Cancer (2020) 72(1):5–14. doi: 10.1080/01635581.2019.1621356
178. Juanola-Falgarona M, Salas-Salvadó J, Ibarrola-Jurado N, Rabassa-Soler A, Díaz-López A, Guasch-Ferré M, et al. Effect of the Glycemic Index of the Diet on Weight Loss, Modulation of Satiety, Inflammation, and Other Metabolic Risk Factors: A Randomized Controlled Trial. Am J Clin Nutr (2014) 100(1):27–35. doi: 10.3945/ajcn.113.081216
179. Sreeja SR, Seo SS, Kim MK. Associations of Dietary Glycemic Index, Glycemic Load and Carbohydrate With the Risk of Cervical Intraepithelial Neoplasia and Cervical Cancer: A Case-Control Study. Nutrients (2020) 12(12):3742. doi: 10.3390/nu12123742
180. Barchitta M, Maugeri A, Quattrocchi A, Agrifoglio O, Scalisi A, Agodi A. The Association of Dietary Patterns With High-Risk Human Papillomavirus Infection and Cervical Cancer: A Cross-Sectional Study in Italy. Nutrients (2018) 10(4):469. doi: 10.3390/nu10040469
181. Murakami K, Sasaki S. Glycemic Index and Glycemic Load of the Diets of Japanese Adults: The 2012 National Health and Nutrition Survey, Japan. Nutrition (2018) 46:53–61. doi: 10.1016/j.nut.2017.08.012
182. Louie JC, Markovic TP, Ross GP, Foote D, Brand-Miller JC. Higher Glycemic Load Diet is Associated With Poorer Nutrient Intake in Women With Gestational Diabetes Mellitus. Nutr Res (2013) 33(4):259–65. doi: 10.1016/j.nutres.2013.02.008
183. Turati F, Galeone C, Gandini S, Augustin LS, Jenkins DJ, Pelucchi C, et al. High Glycemic Index and Glycemic Load are Associated With Moderately Increased Cancer Risk. Mol Nutr Food Res (2015) 59(7):1384–94. doi: 10.1002/mnfr.201400594
184. Hu J, La Vecchia C, Augustin LS, Negri E, de Groh M, Morrison H, et al. Glycemic Index, Glycemic Load and Cancer Risk. Ann Oncol (2013) 24(1):245–51. doi: 10.1093/annonc/mds235
185. Larsson SC, Bergkvist L, Wolk A. Consumption of Sugar and Sugar-Sweetened Foods and the Risk of Pancreatic Cancer in a Prospective Study. Am J Clin Nutr (2006) 84(5):1171–6. doi: 10.1093/ajcn/84.5.1171
186. Nöthlings U, Murphy SP, Wilkens LR, Henderson BE, Kolonel LN. Dietary Glycemic Load, Added Sugars, and Carbohydrates as Risk Factors for Pancreatic Cancer: The Multiethnic Cohort Study. Am J Clin Nutr (2007) 86(5):1495–501. doi: 10.1093/ajcn/86.5.1495
187. Michaud DS, Liu S, Giovannucci E, Willett WC, Colditz GA, Fuchs CS. Dietary Sugar, Glycemic Load, and Pancreatic Cancer Risk in a Prospective Study. J Natl Cancer Inst (2002) 94(17):1293–300. doi: 10.1093/jnci/94.17.1293
188. Pannala R, Leirness JB, Bamlet WR, Basu A, Petersen GM, Chari ST. Prevalence and Clinical Profile of Pancreatic Cancer-Associated Diabetes Mellitus. Gastroenterology (2008) 134(4):981–7. doi: 10.1053/j.gastro.2008.01.039
189. Andersen DK, Korc M, Petersen GM, Eibl G, Li D, Rickels MR, et al. Diabetes, Pancreatogenic Diabetes, and Pancreatic Cancer. Diabetes (2017) 66(5):1103–10. doi: 10.2337/db16-1477
190. Li J, Ma J, Han L, Xu Q, Lei J, Duan W, et al. Hyperglycemic Tumor Microenvironment Induces Perineural Invasion in Pancreatic Cancer. Cancer Biol Ther (2015) 16(6):912–21. doi: 10.1080/15384047.2015.1040952
191. Li W, Zhang L, Chen X, Jiang Z, Zong L, Ma Q. Hyperglycemia Promotes the Epithelial-Mesenchymal Transition of Pancreatic Cancer Via Hydrogen Peroxide. Oxid Med Cell Longev (2016) 2016:5190314. doi: 10.1155/2016/5190314
192. Kesh K, Mendez R, Abdelrahman L, Banerjee S, Banerjee S. Type 2 Diabetes Induced Microbiome Dysbiosis is Associated With Therapy Resistance in Pancreatic Adenocarcinoma. Microb Cell Fact (2020) 19(1):75. doi: 10.1186/s12934-020-01330-3
193. Duan Q, Li H, Gao C, Zhao H, Wu S, Wu H, et al. High Glucose Promotes Pancreatic Cancer Cells to Escape From Immune Surveillance Via AMPK-Bmi1-GATA2-MICA/B Pathway. J Exp Clin Cancer Res (2019) 38(1):192. doi: 10.1186/s13046-019-1209-9
Keywords: diet, ketogenic diet, low-sugar diet, Mediterranean diet, pancreatic cancer
Citation: Zhang Y, Zhang T, Yang W, Chen H, Geng X, Li G, Chen H, Wang Y, Li L and Sun B (2021) Beneficial Diets and Pancreatic Cancer: Molecular Mechanisms and Clinical Practice. Front. Oncol. 11:630972. doi: 10.3389/fonc.2021.630972
Received: 18 November 2020; Accepted: 19 April 2021;
Published: 28 May 2021.
Edited by:
Atrayee Basu Mallick, Thomas Jefferson University, United StatesReviewed by:
Ann-Katrin Kraeuter, Northumbria University, United KingdomZsófia Clemens, University of Pécs, Hungary
Copyright © 2021 Zhang, Zhang, Yang, Chen, Geng, Li, Chen, Wang, Li and Sun. This is an open-access article distributed under the terms of the Creative Commons Attribution License (CC BY). The use, distribution or reproduction in other forums is permitted, provided the original author(s) and the copyright owner(s) are credited and that the original publication in this journal is cited, in accordance with accepted academic practice. No use, distribution or reproduction is permitted which does not comply with these terms.
*Correspondence: Bei Sun, sunbei70@tom.com; Le Li, lile@hrbmu.edu.cn
†These authors have contributed equally to this work