- 1Department Pathogens & Inflammation-EPILAB EA4266, University of Bourgogne France-Comté, Besançon, France
- 2Molecular Cancer and Pharmaceutical Biology Laboratory, Lebanese University, Beyrouth, Lebanon
- 3Department of Pathology, CHRU Besançon, Besançon, France
- 4Institute for Clinical Virology, Ulm University, Ulm, Germany
- 5Department of Virology, CHRU Besancon, Besancon, France
A growing body of evidence addressing the involvement of human cytomegalovirus (HCMV) in malignancies had directed attention to the oncomodulation paradigm. HCMV-DB infected human mammary epithelial cells (HMECs) in culture showed the emergence of clusters of rapidly proliferating, spheroid-shaped transformed cells named CTH (CMV-Transformed HMECs) cells. CTH cells assessment suggests a direct contribution of HCMV to oncogenesis, from key latent and lytic genes activating oncogenic pathways to fueling tumor evolution. We hypothesized that the presence of HCMV genome in CTH cells is of pivotal importance for determining its oncogenic potential. We previously reported the detection of a long non-coding (lnc) RNA4.9 gene in CTH cells. Therefore, we assessed here the presence of UL69 gene, located nearby and downstream of the lncRNA4.9 gene, in CTH cells. The HCMV UL69 gene in CTH cells was detected using polymerase chain reaction (PCR) and sequencing of UL69 gene was performed using Sanger method. The corresponding amino acid sequence was then blasted against the UL69 sequence derived from HCMV-DB genome using NCBI Protein BLAST tool. A 99% identity was present between the nucleotide sequence present in CTH cells and HCMV-DB genome. UL69 transcript was detected in RNA extracts of CTH cells, using a reverse transcription polymerase chain reaction (RT-PCR) assay, and pUL69 protein was identified in CTH lysates using western blotting. Ganciclovir-treated CTH cells showed a decrease in UL69 gene detection and cellular proliferation. In CTH cells, the knockdown of UL69 with siRNA was assessed by RT-qPCR and western blot to reveal the impact of pUL69 on HCMV replication and CTH cell proliferation. Finally, UL69 gene was detected in breast cancer biopsies. Our results indicate a close link between the UL69 gene detected in the HCMV-DB isolate used to infect HMECs, and the UL69 gene present in transformed CTH cells and tumor biopsies, further highlighting a direct role for HCMV in breast tumor development.
Introduction
Human cytomegalovirus (HCMV) is a large double-stranded DNA virus of the Betaherpesvirinae subfamily, with a genome length of approximately 230 kbp (1). HCMV genome encodes for more than 700 short open reading frames (sORFs) and is composed of two unique regions, each flanked by inverted repeats (2, 3). During the lytic cycle, active viral genome replication results in the release of new viral particles, in contrast to the latency phase, during which a limited number of viral gene products are expressed. Latency is established depending on cell type-specified mechanisms related to transcriptional silencing unlike the reactivation phase triggered by diverse stimuli, for instance infection, inflammation, and injury (4). Studies have shown that HCMV activates pro-oncogenic pathways in infected cells, resulting in a modified cellular phenotype that favors transformation (5, 6). Indeed, HCMV gained a considerable attention in cancer due to many factors that could underlie its potential oncogenic role. The latter includes supporting proliferative signals, evading growth suppression while stimulating metastasis and invasion, permitting replicative immortality and angiogenesis, resisting cell death, escaping immune destruction, as well as inducing genome variability including mutations, and tumor stimulating inflammation (7, 8).
We previously observed that the infection with the clinical strain HCMV-DB resulted in the transformation of HMECs in culture, with the appearance of clusters of spheroid cells that contributed to the formation of CMV-transformed HMECs or CTH cells (6). When xenografted in NOD SCID gamma (NSG) mice, CTH cells underwent unchecked proliferation and gave rise to tumors (6). To determine the potential causal role of HCMV in the transformation of CTH cells, it is critical to detect the presence of HCMV genome in those transformed cells. Since the two oncogenic herpesviruses discovered so far, Epstein-Barr virus (EBV) and Kaposi’s sarcoma-associated herpesvirus (KSHV) exploit both latency and low lytic viral replication to fuel the oncogenic process (9–11), we decided to study HCMV genes and proteins expressed in CTH cells, even at low levels. In this context, studies have shown that few HCMV genes such as UL123 and UL122 immediate early genes, UL7, UL111A, UL112, US28, US33, UL135, UL136, and lncRNA4.9 could be responsible for the tumorigenic phenotype observed in some human malignancies (12). Previously, we detected the presence of a 126 bp amplicon corresponding to the sequence of lncRNA4.9 gene (nt95592- nt95717) of HCMV-DB strain in CTH cells (6). Since the previously detected lncRNA4.9 is a non-coding sequence (6), we decided to further assess the presence of the nearby coding HCMV gene UL69, which might be a part of the transformation machinery.
Herein, we detected the presence of UL69 gene in CTH cells using extensive PCR analysis and sequencing. Using phylogenic analysis, we confirmed that the detected UL69 gene in CTH cells matches the UL69 gene sequence of HCMV-DB strain originally used to infect and transform HMECs into CTH cells by 99%. At the proteomic level, the pUL69 sequence present in CTH cells differs from the HCMV-DB original pUL69 only in two amino acid point mutations. At the transcriptome level, we detected the transcript of UL69 and pUL69 in CTH cells. Besides, in agreement with a coding role for UL69 HCMV gene, we detected the expression of the pUL69 in CTH cells in vitro. Upon UL69 knockdown using siRNA, we observed a decrease in viral replication and CTH proliferation. In vivo, we identified UL69 gene with a high frequency in biopsies of breast cancer patients.
Materials and Methods
Isolation and Culture of CTH Cells
Several clusters of spheroid-cells were observed in HCMV-DB infected HMECs around day 20 post-infection in some of the cultures. These clusters were gently detached and the floating detached cells named CTH cells were cultured in HMEC Ready medium (Cat#12752010, Gibco, Grand Island, NY) for numerous passages, currently >150 passages (6). CTH cells used in this study were cultivated for approximately 410 days. HMECs cultures were infected with HCMV-DB at MOI of 1 as previously reported (13). An inverted light microscope (Olympus, Japan) was used to count cells in CTH cultures.
Detection of the UL69 Gene in CTH Cells Using PCR and qPCR Assay
Total DNA derived from uninfected HMECs, HCMV-DB infected HMECs, CTH, and MRC5 cells was extracted using a DNA extraction Kit (EZNA Blood DNA Kit, Omega BIO-TEK, Norcross, GA, USA). The presence of the HCMV sequence spanning the UL69 gene was determined by qualitative PCR assay. The list of primer sets used to screen for the presence of UL69 from HCMV-DB genome in CTH cells is described in Supplementary Table 1. Primer sets were provided by Eurogentec (Seraing, Belgium). Several Taq polymerases were used depending on the size and GC percent content of the amplified fragment. Dream Taq polymerase, KAPA hot start Taq polymerase, and KAPA fast Taq polymerase were ordered from Thermofisher (EP0701) and Sigma-Aldrich (KK1512 and KK4601) respectively. HCMV-DB DNA and lysates of HCMV-DB infected HMECs were used as positive controls. As negative controls, both uninfected HMECs and uninfected MRC5 cells were tested in parallel. Amplified products were electrophoresed in 2% agarose gel stained with Sybr green I nucleic acid stain. As an equal loading control, β-globin gene was amplified (sense: 5′-TCCCCTCCTACCCCTACTTTCTA-3′; antisense: 5′TGCCTGGACTAATC TGCAAGAG-3′). Using real-time qPCR assay, UL69 load was measured in ganciclovir-treated (20 µM for 4 days) and untreated CTH cultures in parallel with the cell counting.
Sequencing of the UL69 Gene Present in CTH Cells
Fragments amplified by PCR were sequenced using the Sanger method (Genoscreen, Lille, France; GATC, Köln, Germany). PCR products were sent either directly after amplification or after gel cutting to harvest the band of the expected size (in case of several bands). Supplementary Table 1 includes the primers used for sequencing. PCR amplifications and Sanger’s sequencing were repeated twice. The obtained sequences were prepared using the BIOEDIT software (14). Sequences were compared against the sequence of the HCMV-DB strain (accession number KT959235) (13) using NCBI nucleotide blast tool (https://blast.ncbi.nlm.nih.gov/BlastAlign.cgi).
Detection of the UL69 Transcript by RT-PCR
RNA was extracted from CTH cells using RNA extraction kit (EZNA Total RNA Kit I, Omega BIO-TEK). Following DNAse I treatment for 30 minutes (ThermoFisher), cDNA was synthesized from extracted RNA using Superscript IV First-strand synthesis kit (ThermoFisher) as per manufacturer instructions. cDNA was then used as a template for qualitative PCR amplification using UL69 primers and Dream Taq polymerase (ThermoFisher) as described above. Lysates of HCMV-DB infected HMECs were used as positive controls. The amplified product was electrophoresed in 2% agarose gel stained with Sybr green I nucleic acid stain.
UL69 knockdown with siRNA performed on CTH cells was assessed by the quantification of UL69 RNA transcripts. Following RNA extraction and DNAse I treatment as described above, cDNA synthesis was performed. Real-time qPCR detection of UL69 was performed using KAPA SYBR FAST Master Mix (KAPA BIOSYSTEMS, KK4601) and UL69 gene primers (sense: 5’-GGGATGTCGATGACTCCCTTC-3’; antisense: 5’- GTCGCTATTGGATCTCACCGT-3’), according to the manufacturers’ instructions. Real-time qPCR reactions were activated at 95°C for 10 minutes and then 50 cycles (15 s at 95°C and 1 min at 60°C) were conducted using a Stratagene Mx3005P thermocycler (Agilent Technology, Santa Clara, CA, USA). The results were recorded and analyzed using MxPro qPCR software.
Western Blotting
Western blot was performed as described previously (13). Both controls and CTH cells were lysed and proteins were separated by electrophoresis on SDS-PAGE gel. Proteins were transferred to a nitrocellulose membrane. After blocking in 5% milk, the membrane was incubated overnight with 1 µg/ml anti-pUL69 polyclonal antibody (kindly provided by Dr. Stamminger, Erlangen University, Germany). Blots were developed with the ECL detection kit (Amersham Biosciences, Piscataway, NJ).
The siRNA-mediated knockdown of UL69 was assessed by western blot. Cellular extracts of transfected CTH cells with scramble siRNA and UL69 siRNA were prepared at day 1 post-transfection. In addition, cellular extracts of MRC5 infected with HCMV-DB and transfected with scramble siRNA and UL69 siRNA were prepared 24 hours post-transfection. β-actin was used as a control to normalize sample loading.
Flow Cytometry Analysis
The proliferation of CTH cells was assessed using the measurement of Ki67 antigen expression by intracellular flow cytometry as described previously (15).
Soft Agar Assay
Colony formation in soft agar seeded with ganciclovir-treated (20 µM for 4 days) and untreated CTH cells was assayed. Ganciclovir-treated and untreated CTH cells were incubated for 14 days in the semisolid agar medium. Colonies were observed under an Olympus microscope as previously reported (6).
Transfection Assay
A total of 0.25×106 cells (HMECs and CTH cells) were transfected with 1-2 µg total plasmid DNA, namely plasmids pUL69, empty pcDNA3.1 (16) and pGFP (17) using JetPEI transfection reagent (Polyplus Transfection, Illkirch, France) as per manufacturers’ protocol. pGFP-positive cells were gated and Ki67Ag expression was measured 24 hours post-transfection in pGFP-positive cells using flow cytometry.
RNA Interference
HCMV-DB infected MRC5 and CTH cells were transfected with scramble siRNA or UL69 siRNA (sense:ACUCAGCCGUUUGAUCGAATT; anti-sense:UUCGAUCAAAC GGCUGAGUTG) (Life Technologies, Carlsbad, CA, USA) using Lipofectamine RNAiMAX (Life Technologies) according to the manufacturer’s protocol. Transfection efficiency was monitored 24 hours post-transfection for HCMV-DB infected MRC5 cells by western blot and for CTH cells by RT-qPCR and western blot as described above.
Determination of Amino Acid Sequences
Amino acid sequence derived from UL69 gene present in CTH cells was obtained using the MBS translator software (http://insilico.ehu.es/translate/) (18). The UL69 amino acid sequence existing in CTH cells was blasted against the pUL69 sequences derived from the HCMV-DB genome (KT959235) (13) using NCBI protein blast tool (https://blast.ncbi.nlm.nih.gov/Blast.cgi).
Phylogenic Analysis
Multi-sequences alignments (MSA) were performed using CLUSTAL W. Phylogenic tree was obtained using the neighbor-joining method and MEGA7 software (http://www.megasoftware.net/) as previously described (6).
Measurement of HCMV Growth
Viral replication was assessed by the quantification of HCMV load following DNA extraction (EZNA Blood DNA Kit, D3392-02, Omega BIO-TEK) by real-time qPCR detection using KAPA SYBR FAST Master Mix (KAPA BIOSYSTEMS, KK4601) and primers specific for the IE1 gene (sense: 5’-CGACGTTCCTGCAGACTATG-3’; antisense: 5’-TCCTCGGTCACTTGTTCAAA-3’) according to the manufacturer’s protocol. Real-time qPCR reactions were activated at 95°C for 10 minutes, followed by 50 cycles (15 seconds at 95°C and 1 minute at 60°C) using a Stratagene Mx3005P thermocycler (Agilent). Results were collected and analyzed using MxPro qPCR software.
Detection of UL69 DNA in Breast Tumor Tissue
All patients who were admitted to Besançon University Hospital (Besançon, France) gave their written informed consent to participate in the study according to the Helsinki declaration. The study was approved by the local ethics committees of Besançon University Hospital (Besançon, France) and the French Research Ministry (AC-2015-2496, CNIL n°1173545, NF-S-96900 n°F2015). Genomic DNA isolated from biopsies of breast cancer patients (n=30) was provided by the regional tumor bank (BB-0033-00024 Tumorothèque Régionale de Franche-Comté). Paired adjacent healthy tissue (n=29) was utilized in the study. 29 paired adjacent healthy samples match 29 tumor samples, but only one of the tumor samples lacks the corresponding healthy tissue. Breast tissues from non-tumor breast disease (n=4) were used as control. For histological grading of breast cancer biopsies, the Elston-Ellis grade was used with the three factors of grading: tubule formation, nuclear pleomorphism, and mitotic count. Each factor is given a score of 1 to 3 (1 being the best; 3 the worst) and the scores of all the three factors are then added up to give a total of 3 to 9 points indicating the tumor grade. Tumor grade is allocated on the following basis: 3 to 5 points: grade I (well-differentiated); 6 to 7 points: grade II (moderately differentiated); 8 to 9 points: grade III (poorly differentiated) (19). The presence of HCMV was determined by quantitative PCR using UL69 primers (sense: 5’-GGGATGTCGATGACTCCCTTC-3’; antisense: 5’- GTCGCTATTGGATCTCACCGT-3’) as reported previously (6).
Statistical Analysis
The reported values represent the mean and SD of independent experiments. Statistical analysis was performed using Mann Whitney U test and differences were considered significant at a value of p-value < 0.05. Microsoft Excel was used for plot construction. Correlation analysis was done using Spearman test (Statistical software SPSS 23). Based on Spearman’s rank correlation coefficient (rho), all bivariate correlation analyses express the strength of association between two variables where r = +1 (positive correlation) and the value r = -1 (negative correlation). Results were interpreted according to the degree of association as strong (0.7–1), moderate (0.5–0.7), or low (0.3–0.5), taking significant correlation (p-value < 0.05) values into consideration.
Results
Presence of HCMV-DB UL69 Gene Signature in CTH Cells
Since we previously identified lncRNA4.9 sequences in CTH cells (7), we screened for the presence of the downstream and nearby UL69 gene using primers spanning the complete gene region (Figure 1A, Supplementary Table 1). Using PCR assay, we first detected two HCMV-DB sequences in CTH cells corresponding to 160 bp and 245 bp UL69 gene amplicons (Figure 1B), indicating that the complete UL69 gene could be present in CTH cells. After demonstrating the presence of UL69 genomic sequences in CTH cells, we used several primers sets to span the complete UL69 gene (Table 1). Overall, we were able to detect the existence of a set of viral sequences in CTH cells that corresponds to the complete UL69 gene (nt99397- nt101619) (Figure 1B, Table 1). UL69 amplicons of the same size were also amplified in positive controls, including HMECs infected acutely with HCMV-DB and HCMV-DB viral stock (Figure 1B), whereas no HCMV sequences were detected in uninfected HMECs and uninfected MRC5 cells used as negative controls (Figure 1B).
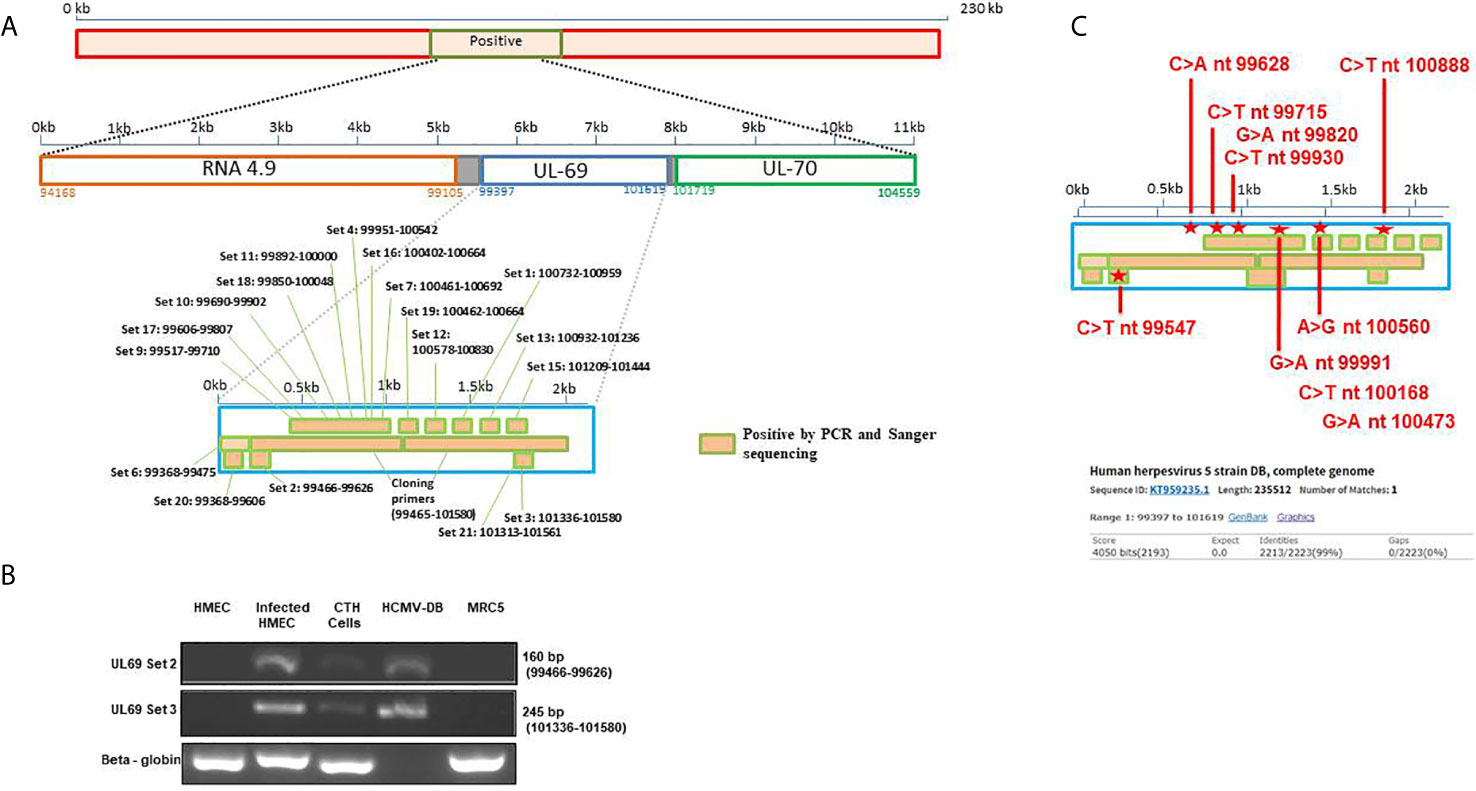
Figure 1 Detection of UL69 gene in CTH cells. (A) Screening strategy used to detect HCMV UL69 sequences in CTH cells using PCR assay. (B) UL69 amplicons detection in CTH cells using conventional PCR. Uninfected HMECs and MRC5 cells were used as negative controls; HCMV-DB viral stock and infected HMECs were considered as positive controls. β-globin was used as an internal control. Agarose gels show results representative of three independent experiments. The complete primer sets used are described in Supplementary Table 1. (C) Detection of nucleotide point mutations in HCMV-DB UL69 sequence present in CTH cells compared to wild-type HCMV-DB virus.
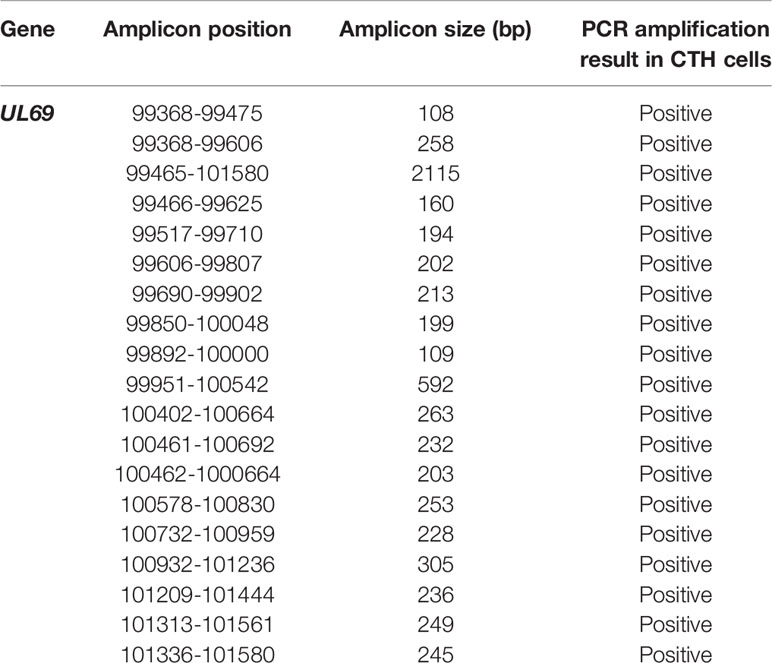
Table 1 Amplicon positions and sizes of the UL69 HCMV-DB gene screened in CTH cells using qualitative and/or quantitative PCR.
Detection of Nucleotide Point Mutations in UL69 Gene Present in CTH Cells Compared to the Original HCMV-DB Strain
To definitively confirm the presence of UL69 gene in CTH cells, obtained UL69 amplicons covering the complete gene were sequenced using the Sanger’s method. UL69 sequences present in CTH cells were then compared to the HCMV-DB genome sequence (KT959235) by using BLAST analysis. We detected ten nucleotide point mutations in the UL69 sequence obtained from CTH cells compared to the parental HCMV-DB sequence (Figure 1C, Table 2, Supplementary Figure 1). Thoroughly, a 99% identity was established between the UL69 nucleotide sequence present in CTH cells and HCMV-DB genome (Figure 1C, Supplementary Figure 1).
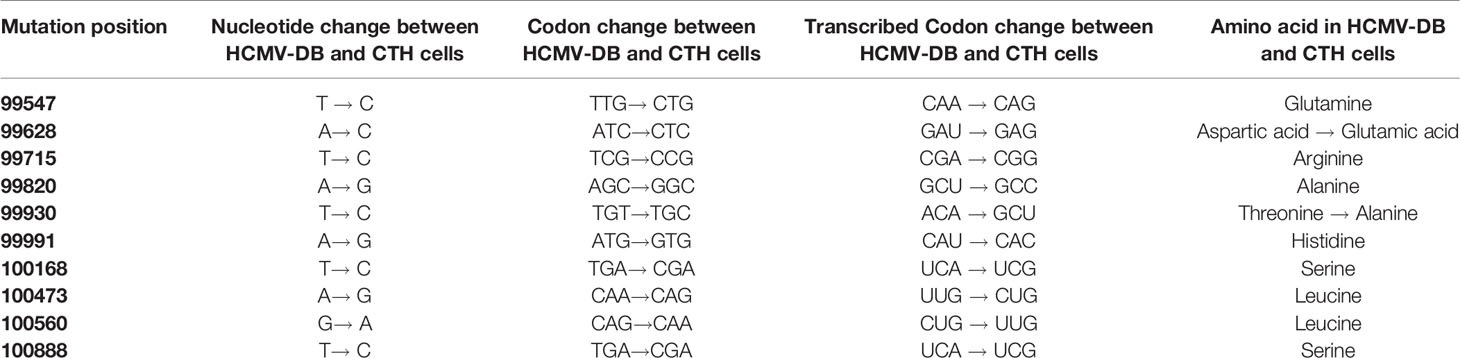
Table 2 Detection of nucleotide point mutations in the UL69 gene present in CTH cells compared to the HCMV-DB.
Detection of the UL69 Transcript and Protein in CTH Cells
Following the detection of UL69 sequence in CTH cells, we subsequently assessed the presence of UL69 transcript and pUL69 protein in CTH cells. Using RT-PCR assay, we detected UL69 transcript in RNA extracts of CTH cells (Figure 2A). We next analyzed the presence of pUL69 protein in CTH cells using western blotting. MRC5 cells infected with HCMV-DB were used as a positive control (Figure 2B). Despite its low levels, pUL69 protein was detected in CTH cells lysates at the expected size (82 kDa) (Figure 2B, red arrow). Intriguingly, one additional band of approximately 60 kDa was observed in CTH lysates compared to HCMV-infected MRC5 cells (Figure 2B, unidentified band). Amino acid sequence derived from UL69 gene present in CTH cells was obtained using the MBS translator software (http://insilico.ehu.es/translate/) (18). UL69 amino acid sequence existing in CTH cells was blasted against pUL69 sequence derived from the HCMV-DB genome (KT959235) (13) using NCBI Protein BLAST tool (https://blast.ncbi.nlm.nih.gov/Blast.cgi). Two residue mutations were detected in pUL69 protein in CTH cells compared to the original HCMV-DB strain. The first mutation is at position 564, where the amino acid threonine (T) in HCMV-DB is changed to alanine (A) in CTH cells. The second one is at position 664, where the amino acid aspartic acid (D) is replaced by glutamic acid (E) (Figure 2C, Table 2).
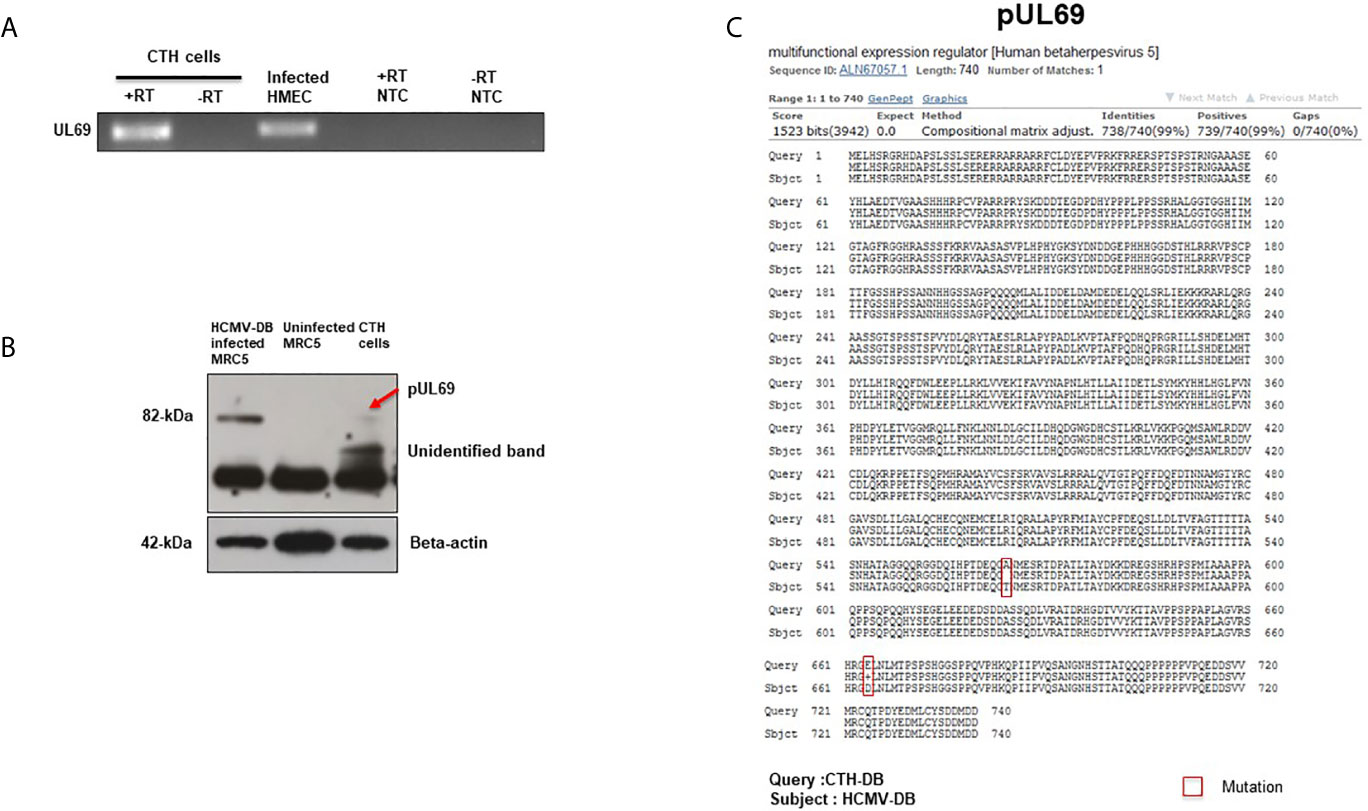
Figure 2 Detection of UL69 transcript and pUL69 protein in CTH cells. (A) RT-PCR assay to detect the presence of UL69 transcript in RNA extracts of CTH cells in the presence (+) and absence (-) of reverse transcriptase (RT). Infected HMECs were used as a positive control and no template control (NTC) as a negative control. (B) Detection of pUL69 protein in CTH cells by western blot. (C) Two residue point mutations present in pUL69 in CTH cells when compared to the original pUL69 from HCMV-DB isolate.
Phylogenetic Analysis of UL69 Gene and pUL69 Protein Present in CTH Cells
UL69 gene present in CTH cells was compared to its counterpart present in HCMV-DB genome, in parallel to comparison with ten other clinical and laboratory-adapted HCMV strains described previously (Figure 3) (5). We observed that UL69 genomic sequence in CTH cells was mostly analogous to the UL69 gene of TB40/E clinical strain than to that of the parental HCMV-DB strain (Figure 3A), indicating a shift of the UL69 genomic sequence from HCMV-DB strain toward TB40/E strain. Similarly, at the protein level, we observed a shift from pUL69 protein of HCMV-DB strain toward TB40/E strain in CTH cells (Figure 3B). This analysis was performed over three independent experiments.
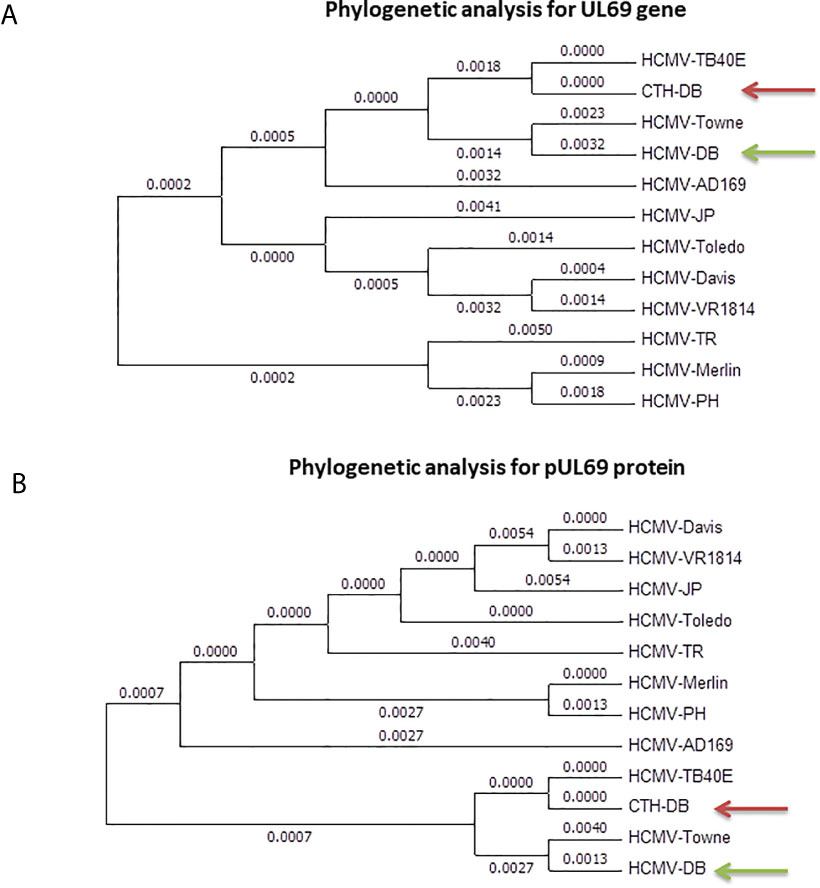
Figure 3 Phylogenetic analysis of UL69 gene and pUL69 protein present in CTH cells. Phylogenetic analysis of UL69 gene (A) and pUL69 protein (B) present in CTH cells compared to the original HCMV-DB strain in addition to other clinical and laboratory-adapted HCMV strains. This analysis was performed over three independent experiments.
Ganciclovir Treatment of CTH Cells Decreases Both UL69 Gene Detection and Cell Proliferation
Since UL69 and the previously described lncRNA4.9 genes are detected in CTH cells, we assessed the presence of UL69 gene in culture supernatants of CTH cells. We detected a very low viral load (around 2 log copies/ml) in culture supernatants of CTH cells, indicating the incidence of a relatively slow viral lytic cycle rate. Upon ganciclovir treatment, a 95% decrease in the viral load was detected in CTH cultures as measured by UL69 quantification, further indicating that HCMV is replicating in CTH cells. Interestingly, parallel to the UL69 decrease, ganciclovir treatment diminished the CTH cell count in culture by 41%, the CTH cell proliferation by 54%, and the size of the colonies observed in soft agar (Figures 4A–D).
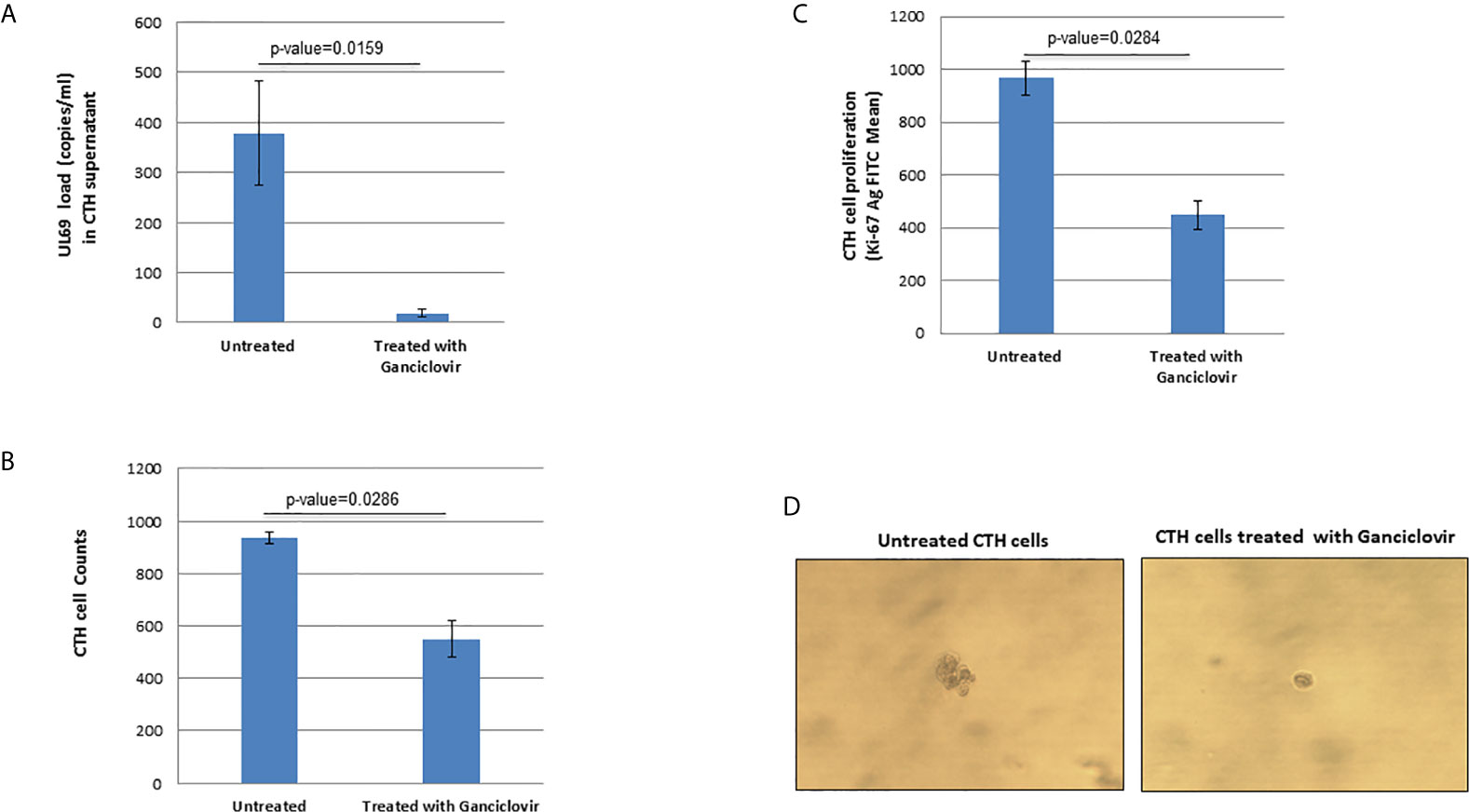
Figure 4 Ganciclovir treatment of CTH cells decreases UL69 viral load, cell count, and cellular proliferation in culture and reduces colony formation in soft agar. (A) Untreated and ganciclovir-treated (20 µM for 4 days) CTH cells were compared for detection of UL69 gene by qPCR, (B) cell counting under the microscope (total magnification of 200x), (C) cellular proliferation as measured by Ki67 antigen quantification by flow cytometry, and (D) colony formation in soft agar.
pUL69 Favors HCMV Replication and CTH Cell Proliferation
To further clarify the role of UL69 protein in CTH cells, we treated CTH cells with UL69 siRNA and a scramble control. Knockdown of UL69 transcript and protein in CTH cells was monitored by RT-qPCR assay and western blot, respectively (Figures 5A, B). In addition, knockdown of UL69 protein was assessed in MRC5 cells acutely infected with HCMV-DB (Supplementary Figure 2A) in parallel with decreased HCMV replication in culture (Supplementary Figure 2B). Knockdown of UL69 decreased both HCMV growth in CTH cell culture (Figure 5C) and CTH cell proliferation (Figure 5D). In addition, after overexpressing pUL69 in HMECs and CTH cells, we observed an increase in cell proliferation at 24 hours post-transfection using Ki67Ag detection by flow cytometry (Figure 5E). Altogether, our data indicate that UL69 favors both HCMV replication and cell proliferation in CTH culture.
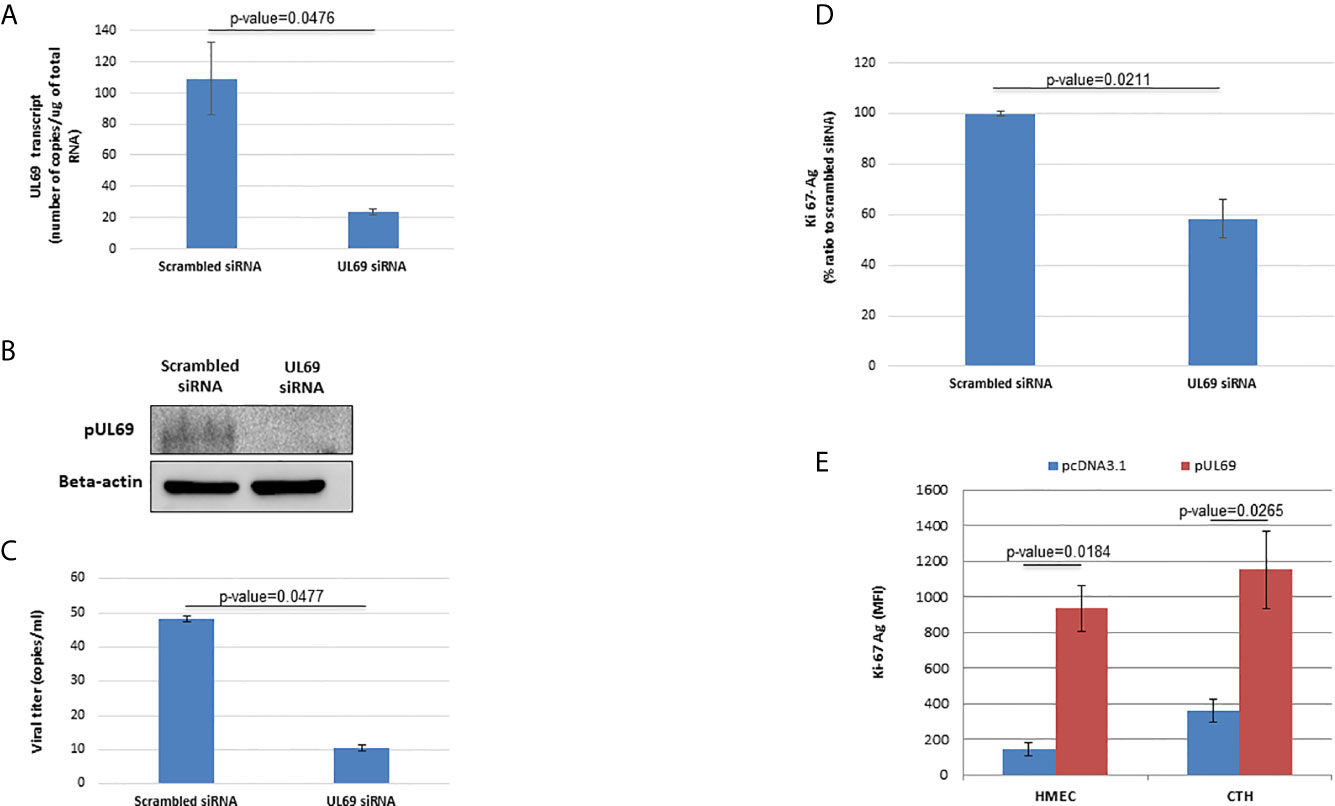
Figure 5 pUL69 favors HCMV replication and cellular proliferation in CTH cells. (A, B) Knockdown of UL69 transcript and protein in CTH cells was monitored by RT-qPCR assay (A) and western blot (B) respectively. CTH cells were treated with UL69 siRNA and a scramble control. RT-qPCR assay and western blot were performed as specified in Methods. (C) Knockdown of UL69 resulted in decreased HCMV replication in CTH cell cultures as measured by detection of IE1 in culture supernatants by qPCR assay. (D) UL69 knockdown decreased CTH cell proliferation as measured by Ki67Ag quantification (MFI, mean fluorescence intensity) by flow cytometry. (E) Overexpression of pUL69 favors the proliferation of HMECs and CTH cells. Increased cellular proliferation as measured by Ki67 antigen quantification (MFI) by flow cytometry in HMEC and CTH cells transfected with pUL69 plasmid. Empty pcDNA3.1 transfected cells were used as control. Results are means (± SD) of three independent experiments.
Detection of UL69 DNA Within Breast Cancer Biopsies
To further demonstrate the pathophysiological relevance of the CTH cell model in vivo, we assessed the expression of the UL69 gene in the genomic DNA obtained from human breast cancer biopsies and paired adjacent healthy tissue. Breast cancer biopsies were classified into luminal and basal-like biopsies based on the IHC analysis of a pathologist. Breast tissue from patients with non-tumor breast disease was used as control. Using quantitative PCR, UL69 gene was detected in 43.33% (n=13/30) and 75.85% (n=22/29) of biopsies from breast tumor and paired adjacent healthy breast tissue (Figure 6A) (p-value=0.01). In addition, UL69 gene was detected in 53.33% (n=8/15) and 33.33% (n=5/15) of biopsies from luminal and basal-like breast cancer, respectively (Figure 6A). In breast tissue taken from patients with non-tumor breast disease, we detected the UL69 gene in 50% of the cases in agreement with approximately 40%-50% of the French healthy adults harboring the virus (20) with a Ct value of 44.35. Among HCMV-positive (UL69 gene positive) biopsies, we did not find significant differences in the viral load between paired healthy breast tissue (Ct=44.24), tumor breast tissue (Ct=44.01), luminal breast biopsies (Ct=43.16), and basal-like breast biopsies (Ct=45.38) as measured by quantitative PCR (UL69 Ct value) (Figure 6B). In tumor biopsies, a significant positive correlation was detected between UL69 Ct value and tubule formation (rho=0.53, p-value=0.038). In luminal breast cancer biopsies, a significant positive correlation was also found between UL69 Ct value and tubule formation (rho=0.62, p-value=0.05). In addition, in basal-like breast cancer biopsies a significant negative correlation was observed between UL69 Ct value and nuclear pleomorphism (rho=-0.89, p-value=0.05) (Table 3).
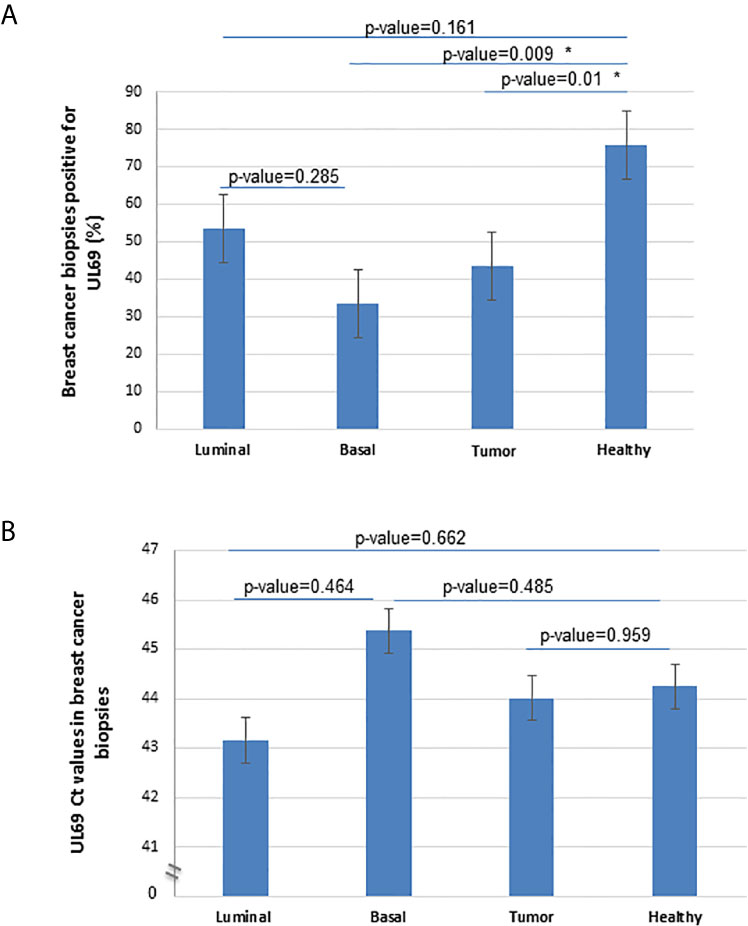
Figure 6 Detection of UL69 DNA in human breast cancer biopsies. (A) Percentage of UL69-positive samples (Ct<50) in breast tumor biopsies (n=30), including luminal (n=15) and basal-like (n=15) tumors, and in adjacent healthy paired breast tissue (n=29) using UL69 quantitative PCR measurement. (B) Quantification of HCMV UL69 DNA in breast tumor biopsies (n=30) compared to healthy adjacent paired breast tissue (n=29), and in patients with basal-like breast cancer (n=15) compared to patients with luminal breast cancer (n=15), as measured by quantitative PCR UL69 Ct value. * A statistically significant difference at p-value < 0.05. Histogram data are represented as mean ± SD of two independent experiments.

Table 3 Correlation between UL69 Ct value and tumor grade (Elston-Ellis), tubule formation, nuclear pleomorphism and mitotic count in breast cancer biopsies.
Discussion
Previously, we reported the transformation of HMECs infected with the HCMV-DB strain, namely the CTH cells (6). To substantiate the causative role of HCMV-DB in HMECs transformation, it is imperative to demonstrate the presence of coding HCMV-DB genes in CTH cells. One short DNA sequence related to the viral lncRNA4.9 was previously detected in CTH cells. In the present study, we assessed the presence of UL69 gene located downstream and nearby the lncRNA4.9. Upon sequencing the UL69 gene present in CTH cells, we detected ten nucleotide point mutations that resulted in two amino acid point mutations in pUL69 in comparison with HCMV-DB genome. Remarkably, pUL69 protein, along with its transcript, were detected in CTH cells. Moreover, UL69 gene was detected in breast cancer biopsies. Overall, our data denote the occurrence of the coding UL69 gene in CTH cells, with potential pathophysiological relevance in breast cancer.
Earlier, we aimed to determine HCMV-DB signature in CTH cells by whole exome sequencing (WES) adopting Sureselect target enrichment method (21). Using WES, we detected limited sequences of HCMV-DB genomic DNA in the CTH cells genome, where the observed signals were close to the “background noise”. Among those weak WES signals in CTH cells, we noticed some hits corresponding to lncRNA4.9 sequence of HCMV-DB. This was further confirmed by both qualitative and quantitative PCR that amplified a 126 bp fragment of lncRNA4.9 originating from HCMV-DB, as confirmed by Sanger’s sequencing (6).
In the present study, UL69 gene, its corresponding transcript, and pUL69 protein were detected in CTH cells confirming the availability of the UL69 gene. The UL69 gene covers a genomic region of 2.2 kb and encodes for 744 amino acids protein (pUL69) (22). pUL69 is a multifunctional regulatory protein belonging to the ICP27 herpesvirus family (23) which contains the ICP27 homology domain (IHD) (24). pUL69 could participate in the transformation of CTH cells via two distinct mechanisms: low viral replication levels in parallel to viral latency as already reported for the two oncoviruses (EBV and KSHV), and/or through a direct oncogenic potential of the viral protein (9–11, 25). Those two mechanisms might not be exclusive to each other. In agreement with low levels of viral replication in sustained CTH transformation by HCMV, we observed decreased levels of UL69 gene in CTH cells treated with ganciclovir. In parallel, this was accompanied by a decrease in CTH cell count in culture, indicating reduced CTH cell proliferation, in addition to a lower number of colony formation in soft agar. Although we cannot exclude the role of other viral proteins in the maintenance of the CTH cell transformation throughout a prolonged cell culture, UL69 gene and pUL69 might contribute to the exquisite balance between low viral replication and the transformation process. Thus, comparable to EBV and KSHV, HCMV low replication level could fuel oncogenesis, where pUL69 could be part of this process since it targets several stages of the lytic viral cycle such as transcription, mRNA nuclear export, and translation. Indeed, pUL69 is involved in gene transcription and binds via its IHD domain to the cellular transcriptional elongation factor SPT6 which favors chromatin remodeling. Upon knocking-down SPT6, an approximately 90% inhibition of the wild-type replication was detected, thus highlighting the significance of SPT6-UL69 binding in HCMV replication (24, 26, 27). Besides, pUL69 possesses binding motifs for UAP56/URH49 known as cellular mRNA adaptor proteins (28). pUL69 binding to the cellular helicase UAP56 has a role in transcriptional elongation and also promotes the mRNA nuclear export (16, 29). Additionally, pUL69 contains nuclear export and localization sequences that play a vital role in facilitating the shuttle between the nucleus and the cytoplasm (24). Finally, to modulate translation in HCMV-infected cells, pUL69 interacts with part of the cap-binding complex, eIF4A1, and poly (A) binding protein (30). The detection of low levels of pUL69 protein and low viral replication rate in CTH cells are in line with the previously described constrained viral replication in sustained long term cultures of cancerous cell lines infected acutely with HCMV (31, 32). In fact, it has been shown that persistent HCMV infection of tumor cells may lead to the selection of novel virus variants characterized by changes in the coding sequences for virus regulatory proteins that have lost their ability to induce cell cycle arrest. Thus, persistent HCMV infection of tumor cells results in the development of mutant viral variants, that grow slowly and yield lower amounts of progeny virus compared to the wild-type viral strains originally used for infection (31, 33).
Based on UL69 knockdown (Figures 5A–D) and overexpression (Figure 5E) experiments, we observed that UL69 favors both HCMV replication and cell proliferation in CTH cultures. Enhanced cell proliferation could be involved in the sustained growth of CTH cells in culture. In contrast to our data in CTH cells, a pUL69-mediated cell cycle block in G1/S phase has been reported previously in acutely HCMV-infected MRC5 cells (34, 35). The difference in the cell type used (fibroblast versus epithelial cells) and in acute (MRC5 cells) versus chronic (CTH cells) infection could explain the observed discrepancies. In addition, cells with disrupted cell cycle tend to control mechanisms in a similar manner to tumor cells; the function of virus regulatory proteins may depend on the internal landscape of tumor cells including p53 and Rb expression/mutation (36). For example, HCMV IE2 protein induces a G1/S block in human cells with wild-type pRb, but not in the human Rb-deficient osteosarcoma cell line Saos-2. Similarly, sustained HCMV infection did not induce cell cycle arrest in T89G glioblastoma cells with disrupted p53 signaling, but virus antigen-positive cells continued to divide (37). Interestingly, we previously reported both a functional defect of p53 and Rb downregulation in CTH cells infected with HCMV-DB (6). These findings indicate that the effects of HCMV on cell cycle and cell proliferation may depend on both the landscape of the internal cellular environment (e.g. p53, Rb) and the properties of virus regulatory proteins such as pUL69 (38). Although we did not yet decipher the molecular mechanism(s) involved in HCMV transformation and the role played by pUL69 (alone or in conjunction with other viral proteins) in this phenomenon, pUL69 favors sustained proliferation of CTH cells in parallel with low sustained viral growth in culture which have been reported as key elements for the transforming capabilities of the two oncogenic herpesviruses EBV and KSHV (25).
In addition to the role of pUL69 in low level viral replication detected in CTH cell cultures, it might also be directly involved in the oncogenic process fueled by HCMV. Notably, the functions of several cellular proteins involved in anti-viral responses and oncogenesis are under the control of certain modifications caused by the small ubiquitin-like modifiers (SUMOs), referred to as SUMOylation. These modifications can change the stability or localization of a specific protein and are capable of enhancing protein-protein interactions through the binding process of SUMOs to the SUMO-interacting sequences (SIMs) (39, 40). SUMOylation acts as a fundamental controller of cell cycle progression, genome stability, senescence, gene expression, stress, and innate immune responses (39, 41, 42). SUMO signal transduction has been identified as a key factor in the development of various cancer types, and SUMOylation was shown to be highly upregulated in several malignancies including breast cancer (39, 43–45). A recent report indicates that pUL69 has a SUMO E3 ligase activity and it can potentially bind to SUMO and Ubc9 (46). Besides, pUL69 takes over the induction of p53 SUMOylation when in complex with Ubc9 and SAE (46). Moreover, in Kaposi’s sarcoma-associated herpesvirus (KSHV), K-bZIP represents a SUMO2/3-specific E3 ligase, leading to its own SUMOylation in addition to that of p53 and Rb (47). Since pUL69 protein present in CTH cells possesses an E3 ligase activity, it might induce the inactivation of p53 through SUMOylation, thereby potentially leading to the development of cancer. In addition, a virally-encoded deSUMOylase activity is required for HCMV reactivation from latency (48). In line with the fact that SUMOylation is involved in HCMV latency, it is often considered as a prerequisite for cellular transformation by herpes oncoviruses (7, 25). It should be pointed out that the binding of pUL69 to PRMT6 could be another mechanism elucidating its role in cancer development. We previously noted the upregulation of PRMT6 gene expression in HCMV-infected HMECs (5). The binding of pUL69 to PRMT6 could favor oncogenesis as already reported with the upregulated expression of PRMT6 in prostate cancer (17, 49). Therefore, the multifunctional pUL69 protein might play a complex role in controlling CTH cell transformation. Future studies adopting UL69 deletion and/or point mutation constructs and UL69-defective HCMV might further assess its direct (or indirect) role in oncogenesis.
It is worth noting that in macrophages, interferon (IFN) production is inhibited through ICP27‐mediated interaction with the active stimulator of interferon genes (STING) signalosome. Knowing that UL69 protein represents the HCMV homolog of ICP27, it compromises the STING signaling pathway and type I IFN production, which might favor viral immune evasion and further cancer cell proliferation and migration (50, 51). Furthermore, a previous study indicated a potential role of the viral immuno-modulator cytokine IL-10 during HCMV infection in enhancing breast tumor development and metastasis (52). Likewise, HCMV IL-10 exposed MCF-7 human breast cancer cells showed a significant up-regulation of matrix metalloproteinase-10 (MMP-10) gene expression, thus promoting excessive proliferation and tissue invasion (53).
Although lncRNA4.9 has been shown to physically interact with the HCMV latent viral chromosome (54), we previously reported the presence of HCMV lncRNA4.9 gene in breast cancer biopsies (6). In the present study, we detected the presence of the nearby UL69 gene in breast cancer biopsies. A lower detection of the UL69 gene (as measured by the percentage of UL69 positive samples) was observed in breast tumor tissue compared to paired healthy breast tissue, in agreement with the previously reported block of HCMV replication in tumor tissue (55, 56). Since HCMV is detected in both tumor tissue and healthy tissue present in the vicinity of the breast tumor, the presence of HCMV in epithelial cells, tissue macrophages, or endothelial cells in normal breast tissue could fuel cellular transformation leading to breast cancer in some patients.
In conclusion, our findings highlight the potential of a clinical HCMV strain, namely the HCMV-DB isolate, to fulfill all the requirements contributing to the transformation of HMECs in vitro, causing the genesis of CTH cells. HCMV could possibly induce transformation through viral gene expression, including the UL69 gene, which has been hijacked by latent and/or lytic viral cycle toward cellular transformation. The presence of UL69 DNA in the majority of biopsies isolated from women with breast cancer indicates a potential relevance of UL69 gene in breast cancer pathophysiology. The study of the UL69 gene in CTH cells could be relevant to understand the molecular mechanisms involved in the development of breast cancer.
Data Availability Statement
The datasets presented in this study can be found in online repositories. The names of the repository/repositories and accession number(s) can be found in the article/Supplementary Material.
Ethics Statement
The studies involving human participants were reviewed and approved by the study was approved by the local ethics committees of Besançon University Hospital (Besançon, France) and the French Research Ministry (AC-2015-2496, CNIL n°1173545, NF-S-96900 n°F2015). Genomic DNA isolated from biopsies of breast cancer patients (n=30) was provided by the regional tumor bank (BB-0033-00024 Tumorothèque Régionale de Franche-Comté). The patients/participants provided their written informed consent to participate in this study.
Author Contributions
SH, FAM, RB, ZN, SP, AK, FM and CM performed experiments. SP, GH, RK, M-PA, MD and TS participated to the data analysis. GH conceived and designed the project. SH, RB, SP and GH wrote the manuscript. All authors contributed to the article and approved the submitted version.
Funding
This work was supported by grants from the University of Franche-Comté (CR3300), and the Région Franche-Comté (2020-11890). The funders had no role in the data collection, analysis, patient recruitment, or decision to publish.
Conflict of Interest
The authors declare that the research was conducted in the absence of any commercial or financial relationships that could be construed as a potential conflict of interest.
Supplementary Material
The Supplementary Material for this article can be found online at: https://www.frontiersin.org/articles/10.3389/fonc.2021.627866/full#supplementary-material
Supplementary Figure 1 | Blast analysis of UL69 nucleotide sequence. Comparison of UL69 gene sequence present in CTH cells (Query) to the wild type HCMV-DB (Subject).
Supplementary Figure 2 | Assessment of pUL69 protein expression and HCMV growth in MRC5 cells treated with UL69 siRNA and siRNA scramble. (A) pUL69 expression and (B) HCMV growth were monitored 24 hours post transfection with siRNA in HCMV-DB infected MRC5 cells by western blot and detection of IE1 in culture supernatants by qPCR assay, respectively. Results are representative of two independent experiments.
Abbreviations
A, adenine; C, cytosine; CDK19, cyclin dependent kinase 19; CTH cells, CMV Transformed HMECs; EBV, Epstein-Barr virus; G, guanine; HCMV, human cytomegalovirus; HMECs, human mammary epithelial cells; IFN, interferon; IHD, ICP27 homology domain; KSHV, Kaposi’s sarcoma-associated herpesvirus; LncRNA4.9, long non-coding RNA4.9; MMP-10, matrix metalloproteinase-10; MSA, multi-sequences alignments; NTC, no template control; PCR, polymerase chain reaction; RT, reverse transcriptase; RT-PCR, reverse transcription polymerase chain reaction; SIMs, SUMO-interacting sequences; sORFs, short open reading frames; STING, stimulator of interferon genes; SUMOs, small ubiquitin-like modifiers; T, thymine; WES, whole exome sequencing.
References
1. Dolan A, Cunningham C, Hector RD, Hassan-Walker AF, Lee L, Addison C, et al. Genetic content of wild-type human cytomegalovirus. J Gen Virol (2004) 85(5):1301–12. doi: 10.1099/vir.0.79888-0
2. Tirosh O, Cohen Y, Shitrit A, Shani O, Le-Trilling VTK, Trilling M, et al. The Transcription and Translation Landscapes during Human Cytomegalovirus Infection Reveal Novel Host-Pathogen Interactions. PloS Pathog (2015) 11(11):e1005288. doi: 10.1371/journal.ppat.1005288
3. Van Damme E, Van Loock M. Functional annotation of human cytomegalovirus gene products: an update. Front Microbiol (2014) 5:218. doi: 10.3389/fmicb.2014.00218
4. Forte E, Zhang Z, Thorp EB, Hummel M. Cytomegalovirus Latency and Reactivation: An Intricate Interplay With the Host Immune Response. Front Cell Infect Microbiol (2020) 10:130. doi: 10.3389/fcimb.2020.00130
5. Moussawi FA, Kumar A, Pasquereau S, Tripathy MK, Karam W, Diab-Assaf M, et al. The transcriptome of human mammary epithelial cells infected with the HCMV-DB strain displays oncogenic traits. Sci Rep (2018) 8(1):12574. doi: 10.1038/s41598-018-30109-1
6. Kumar A, Tripathy MK, Pasquereau S, Al Moussawi F, Abbas W, Coquard L, et al. The Human Cytomegalovirus Strain DB Activates Oncogenic Pathways in Mammary Epithelial Cells. EBioMedicine (2018) 30:167–83. doi: 10.1016/j.ebiom.2018.03.015
7. Herbein G. The Human Cytomegalovirus, from Oncomodulation to Oncogenesis. Viruses (2018) 10(8):408. doi: 10.3390/v10080408
8. Pasquereau S, Al Moussawi F, Karam W, Diab Assaf M, Kumar A, Herbein G. Cytomegalovirus, Macrophages and Breast Cancer. Open Virol J (2017) 11(1):15–27. doi: 10.2174/1874357901711010015
9. Bristol JA, Djavadian R, Albright ER, Coleman CB, Ohashi M, Hayes M, et al. A cancer-associated Epstein-Barr virus BZLF1 promoter variant enhances lytic infection. PloS Pathog (2018) 14(7):e1007179. doi: 10.1371/journal.ppat.1007179
10. Grundhoff A, Ganem D. Inefficient establishment of KSHV latency suggests an additional role for continued lytic replication in Kaposi sarcoma pathogenesis. J Clin Invest (2004) 113(1):124–36. doi: 10.1172/JCI200417803
11. Weidner-Glunde M, Kruminis-Kaszkiel E, Savanagouder M. Herpesviral Latency—Common Themes. Pathogens (2020) 9(2):125. doi: 10.3390/pathogens9020125
12. Ye L, Qian Y, Yu W, Guo G, Wang H, Xue X. Functional Profile of Human Cytomegalovirus Genes and Their Associated Diseases: A Review. Front Microbiol (2020) 11:2104. doi: 10.3389/fmicb.2020.02104
13. Khan KA, Coaquette A, Davrinche C, Herbein G. Bcl-3-Regulated Transcription from Major Immediate-Early Promoter of Human Cytomegalovirus in Monocyte-Derived Macrophages. J Immunol (2009) 182(12):7784–94. doi: 10.4049/jimmunol.0803800
14. Hall TA. BioEdit: a user-friendly biological sequence alignment editor and analysis program for Windows 95/98/NT. Nucleic Acids Symposium Ser (1999) 41:95–8.
15. Lepiller Q, Abbas W, Kumar A, Tripathy MK, Herbein G. HCMV Activates the IL-6-JAK-STAT3 Axis in HepG2 Cells and Primary Human Hepatocytes. PloS One (2013) 8(3):e59591. doi: 10.1371/journal.pone.0059591
16. Lischka P, Toth Z, Thomas M, Mueller R, Stamminger T. The UL69 Transactivator Protein of Human Cytomegalovirus Interacts with DEXD/H-Box RNA Helicase UAP56 To Promote Cytoplasmic Accumulation of Unspliced RNA. Mol Cell Biol (2006) 26(5):1631–43. doi: 10.1128/MCB.26.5.1631-1643.2006
17. Thomas M, Sonntag E, Müller R, Schmidt S, Zielke B, Fossen T, et al. pUL69 of Human Cytomegalovirus Recruits the Cellular Protein Arginine Methyltransferase 6 via a Domain That Is Crucial for mRNA Export and Efficient Viral Replication. J Virol (2015) 89(18):9601–15. doi: 10.1128/JVI.01399-15
18. Bikandi J, Millan RS, Rementeria A, Garaizar J. In silico analysis of complete bacterial genomes: PCR, AFLP-PCR and endonuclease restriction. Bioinformatics (2004) 20(5):798–9. doi: 10.1093/bioinformatics/btg491
19. Elston CW, Ellis IO. pathological prognostic factors in breast cancer. I. The value of histological grade in breast cancer: experience from a large study with long-term follow-up. Histopathology (1991) 19(5):403–10. doi: 10.1111/j.1365-2559.1991.tb00229.x
20. Antona D, Lepoutre A, Fonteneau L, Baudon C, Halftermeyer-Zhou F, Le Strat Y, et al. Seroprevalence of cytomegalovirus infection in France in 2010. Epidemiol Infect (2017) 145(7):1471–8. doi: 10.1017/S0950268817000103
21. Depledge DP, Palser AL, Watson SJ, Lai IY-C, Gray ER, Grant P, et al. Specific Capture and Whole-Genome Sequencing of Viruses from Clinical Samples. PloS One (2011) 6(11):e27805. doi: 10.1371/journal.pone.0027805
22. Kalejta RF. Functions of Human Cytomegalovirus Tegument Proteins Prior to Immediate Early Gene Expression. In: Shenk TE, Stinski MF, editors. Human Cytomegalovirus. Berlin, Heidelberg: Springer Berlin Heidelberg (2008). p. 101–15.
23. Winkler M, Rice SA, Stamminger T. UL69 of human cytomegalovirus, an open reading frame with homology to ICP27 of herpes simplex virus, encodes a transactivator of gene expression. J Virol (1994) 68(6):3943–54. doi: 10.1128/JVI.68.6.3943-3954.1994
24. Tunnicliffe RB, Collins RF, Ruiz Nivia HD, Sandri-Goldin RM, Golovanov AP. The ICP27 Homology Domain of the Human Cytomegalovirus Protein UL69 Adopts a Dimer-of-Dimers Structure. mBio (2018) 9(3):e01112–18. doi: 10.1128/mBio.01112-18
25. Herbein G, Nehme Z. Polyploid Giant Cancer Cells, a Hallmark of Oncoviruses and a New Therapeutic Challenge. Front Oncol (2020) 10:567116. doi: 10.3389/fonc.2020.567116
26. Winkler M, aus dem Siepen T, Stamminger T. Functional Interaction between Pleiotropic Transactivator pUL69 of Human Cytomegalovirus and the Human Homolog of Yeast Chromatin Regulatory Protein SPT6. J Virol (2000) 74(17):8053–64. doi: 10.1128/JVI.74.17.8053-8064.2000
27. Cygnar D, Hagemeier S, Kronemann D, Bresnahan WA. The Cellular Protein SPT6 Is Required for Efficient Replication of Human Cytomegalovirus. J Virol (2012) 86(4):2011–20. doi: 10.1128/JVI.06776-11
28. Thomas M, Müller R, Horn G, Bogdanow B, Imami K, Milbradt J, et al. Phosphosite Analysis of the Cytomegaloviral mRNA Export Factor pUL69 Reveals Serines with Critical Importance for Recruitment of Cellular Proteins Pin1 and UAP56/URH49. J Virol (2020) 94(8):e02151–19. doi: 10.1128/JVI.02151-19
29. Zielke B, Thomas M, Giede-Jeppe A, Muller R, Stamminger T. Characterization of the Betaherpesviral pUL69 Protein Family Reveals Binding of the Cellular mRNA Export Factor UAP56 as a Prerequisite for Stimulation of Nuclear mRNA Export and for Efficient Viral Replication. J Virol (2011) 85(4):1804–19. doi: 10.1128/JVI.01347-10
30. Aoyagi M, Gaspar M, Shenk TE. Human cytomegalovirus UL69 protein facilitates translation by associating with the mRNA cap-binding complex and excluding 4EBP1. Proc Natl Acad Sci (2010) 107(6):2640–5. doi: 10.1073/pnas.0914856107
31. Ogura T, Tanaka J, Kamiya S, Sato H, Ogura H, Hatano M. Human Cytomegalovirus Persistent Infection in a Human Central Nervous System Cell Line: Production of a Variant Virus with Different Growth Characteristics. J Gen Virol (1986) 67:2605–16. doi: 10.1099/0022-1317-67-12-2605
32. Cinatl J, Vogel J-U, Cinatl J, Weber B, Rabenau H, Novak M, et al. Long-Term Productive Human Cytomegalovirus Infection of a Human Neuroblastoma Cell Line. Int J Cancer (1996) 65:90–6. doi: 10.1002/(SICI)1097-0215(19960103)65:1<90::AID-IJC16>3.0.CO;2-M
33. Furukawa T. A variant of human cytomegalovirus derived from a persistently infected culture. Virology (1984) 137(1):191–4. doi: 10.1016/0042-6822(84)90023-0
34. Lu M, Shenk T. Human Cytomegalovirus UL69 Protein Induces Cells To Accumulate in G1 Phase of the Cell Cycle. J Virol (1999) 73(1):676–83. doi: 10.1128/JVI.73.1.676-683.1999
35. Hayashi ML, Blankenship C, Shenk T. Human cytomegalovirus UL69 protein is required for efficient accumulation of infected cells in the G1 phase of the cell cycle. Proc Natl Acad Sci (2000) 97(6):2692–6. doi: 10.1073/pnas.050587597
36. Cinatl J, Vogel J-U, Kotchetkov R, Wilhelm Doerr H. Oncomodulatory signals by regulatory proteins encoded by human cytomegalovirus: a novel role for viral infection in tumor progression. FEMS Microbiol Rev (2004) 28(1):59–77. doi: 10.1016/j.femsre.2003.07.005
37. Luo MH, Fortunato EA. Long-Term Infection and Shedding of Human Cytomegalovirus in T98G Glioblastoma Cells. J Virol (2007) 81(19):10424–36. doi: 10.1128/JVI.00866-07
38. Michaelis M, Doerr HW, Cinatl J. The Story of Human Cytomegalovirus and Cancer: Increasing Evidence and Open Questions. Neoplasia (2009) 11(1):1–9. doi: 10.1593/neo.81178
39. Eifler K, Vertegaal ACO. SUMOylation-Mediated Regulation of Cell Cycle Progression and Cancer. Trends Biochem Sci (2015) 40(12):779–93. doi: 10.1016/j.tibs.2015.09.006
40. Flotho A, Melchior F. Sumoylation: A Regulatory Protein Modification in Health and Disease. Annu Rev Biochem (2013) 82(1):357–85. doi: 10.1146/annurev-biochem-061909-093311
41. Hoellein A, Fallahi M, Schoeffmann S, Steidle S, Schaub FX, Rudelius M, et al. Myc-induced SUMOylation is a therapeutic vulnerability for B-cell lymphoma. Blood (2014) 124(13):2081–90. doi: 10.1182/blood-2014-06-584524
42. Rabellino A, Andreani C, Scaglioni PP. The Role of PIAS SUMO E3-Ligases in Cancer. Cancer Res (2017) 77(7):1542–7. doi: 10.1158/0008-5472.CAN-16-2958
43. Seeler J-S, Dejean A. SUMO and the robustness of cancer. Nat Rev Cancer (2017) 17(3):184–97. doi: 10.1038/nrc.2016.143
44. Bawa-Khalfe T, Yeh ETH. SUMO Losing Balance: SUMO Proteases Disrupt SUMO Homeostasis to Facilitate Cancer Development and Progression. Genes Cancer (2010) 1(7):748–52. doi: 10.1177/1947601910382555
45. Kessler JD, Kahle KT, Sun T, Meerbrey KL, Schlabach MR, Schmitt EM, et al. A SUMOylation-Dependent Transcriptional Subprogram Is Required for Myc-Driven Tumorigenesis. Science (2012) 335(6066):348–53. doi: 10.1126/science.1212728
46. De La Cruz-Herrera CF, Shire K, Siddiqi UZ, Frappier L. A genome-wide screen of Epstein-Barr virus proteins that modulate host SUMOylation identifies a SUMO E3 ligase conserved in herpesviruses. PloS Pathog (2018) 14(7):e1007176. doi: 10.1371/journal.ppat.1007176
47. Chang P-C, Izumiya Y, Wu C-Y, Fitzgerald LD, Campbell M, Ellison TJ, et al. Kaposi’s Sarcoma-associated Herpesvirus (KSHV) Encodes a SUMO E3 ligase That Is SIM-dependent and SUMO-2/3-specific. J Biol Chem (2010) 285(8):5266–73. doi: 10.1074/jbc.M109.088088
48. Poole EL, Kew VG, Lau JCH, Murray MJ, Stamminger T, Sinclair JH, et al. A Virally Encoded DeSUMOylase Activity Is Required for Cytomegalovirus Reactivation from Latency. Cell Rep (2018) 24(3):594–606. doi: 10.1016/j.celrep.2018.06.048
49. Almeida-Rios D, Graça I, Vieira FQ, Ramalho-Carvalho J, Pereira-Silva E, Martins AT, et al. Histone methyltransferase PRMT6 plays an oncogenic role of in prostate cancer. Oncotarget (2016) 7(33):53018–28. doi: 10.18632/oncotarget.10061
50. Christensen MH, Jensen SB, Miettinen JJ, Luecke S, Prabakaran T, Reinert LS, et al. HSV -1 ICP 27 targets the TBK 1-activated STING signalsome to inhibit virus-induced type I IFN expression. EMBO J (2016) 35(13):1385–99. doi: 10.15252/embj.201593458
51. Banete A, Seaver K, Bakshi D, Gee K, Basta S. On taking the STING out of immune activation. J Leukoc Biol (2018) 103(6):1189–95. doi: 10.1002/JLB.2MIR0917-383R
52. Valle Oseguera CA, Spencer JV. Human cytomegalovirus interleukin-10 enhances matrigel invasion of MDA-MB-231 breast cancer cells. Cancer Cell Int (2017) 17(1):24. doi: 10.1186/s12935-017-0399-5
53. Bishop RK, Valle Oseguera CA, Spencer JV. Human Cytomegalovirus interleukin-10 promotes proliferation and migration of MCF-7 breast cancer cells. Cancer Cell Microenviron (2015) 2(1):e678. doi: 10.14800/ccm.678
54. Rossetto CC, Tarrant-Elorza M, Pari GS. Cis and Trans Acting Factors Involved in Human Cytomegalovirus Experimental and Natural Latent Infection of CD14 (+) Monocytes and CD34 (+) Cells. PloS Pathog (2013) 9(5):e1003366. doi: 10.1371/journal.ppat.1003366
55. Xu S, Schafer X, Munger J. Expression of Oncogenic Alleles Induces Multiple Blocks to Human Cytomegalovirus Infection. J Virol (2016) 90(9):4346–56. doi: 10.1128/JVI.00179-16
Keywords: cytomegalovirus - HCMV, UL69, CTH cells, oncogenesis, latency
Citation: Haidar Ahmad S, Al Moussawi F, El Baba R, Nehme Z, Pasquereau S, Kumar A, Molimard C, Monnien F, Algros M-P, Karaky R, Stamminger T, Diab Assaf M and Herbein G (2021) Identification of UL69 Gene and Protein in Cytomegalovirus-Transformed Human Mammary Epithelial Cells. Front. Oncol. 11:627866. doi: 10.3389/fonc.2021.627866
Received: 10 November 2020; Accepted: 26 March 2021;
Published: 16 April 2021.
Edited by:
Victoria Virador, Virador and Associates, United StatesReviewed by:
Benjamin Anthony Krishna, Cleveland Clinic, United StatesCyprian Rossetto, University of Nevada, United States
Copyright © 2021 Haidar Ahmad, Al Moussawi, El Baba, Nehme, Pasquereau, Kumar, Molimard, Monnien, Algros, Karaky, Stamminger, Diab Assaf and Herbein. This is an open-access article distributed under the terms of the Creative Commons Attribution License (CC BY). The use, distribution or reproduction in other forums is permitted, provided the original author(s) and the copyright owner(s) are credited and that the original publication in this journal is cited, in accordance with accepted academic practice. No use, distribution or reproduction is permitted which does not comply with these terms.
*Correspondence: Georges Herbein, Z2Vvcmdlcy5oZXJiZWluQHVuaXYtZmNvbXRlLmZy
†These authors have contributed equally to this work