- Department of Gastroenterology, The Second Affiliated Hospital, Zhejiang University School of Medicine, Hangzhou, China
Tumor progression depends on the collaborative interactions between tumor cells and the surrounding stroma. First-line therapies direct against cancer cells may not reach a satisfactory outcome, such as gastric cancer (GC), with high risk of recurrence and metastasis. Therefore, novel treatments and drugs target the effects of stroma components are to be promising alternatives. Mesenchymal stem cells (MSC) represent the decisive components of tumor stroma that are found to strongly affect GC development and progression. MSC from bone marrow or adjacent normal tissues express homing profiles in timely response to GC-related inflammation signals and anchor into tumor bulks. Then the newly recruited “naïve” MSC would achieve phenotype and functional alternations and adopt the greater tumor-supporting potential under the reprogramming of GC cells. Conversely, both new-comers and tumor-resident MSC are able to modulate the tumor biology via aberrant activation of oncogenic signals, metabolic reprogramming and epithelial-to-mesenchymal transition. And they also engage in remodeling the stroma better suited for tumor progression through immunosuppression, pro-angiogenesis, as well as extracellular matrix reshaping. On the account of tumor tropism, MSC could be engineered to assist earlier diagnosis of GC and deliver tumor-killing agents precisely to the tumor microenvironment. Meanwhile, intercepting and abrogating vicious signals derived from MSC are of certain significance for the combat of GC. In this review, we mainly summarize current advances concerning the reciprocal metabolic interactions between MSC and GC and their underlying therapeutic implications in the future.
Introduction
Gastric cancer (GC) is one of the most refractory malignancies with high morbidity and mortality. Updated statistics indicates that GC is the fifth frequently diagnosed cancer with over 1,000,000 new cases and the third leading cause of cancer-associated morbidity, an estimated 783,000 deaths worldwide (1). Helicobacter pylori (Hp) eradication, gastroscopy, and endoscopic treatment have reduced the risk of developing GC, as well as provided better long-term health-related quality of life for patients with early GC (2, 3). Regrettably, a larger population of people is already in the advanced stage at the first diagnosis for rarely present symptoms. Although a combination of surgical resection with adjuvant chemotherapy is the preferential option for advanced GC, the survival outcome stays disappointing, dropping a median overall survival of 10-12 months (4, 5). Hence, searching for new strategies, such as immunotherapy, targeted therapy, and making clinical transformation remain urgently needed.
Of note, the occurrence and development of tumor cannot be isolated from the tumor microenvironment (TME). The surrounding microenvironment “soil” is to facilitate the survival and thriving of tumor cell “seed” via substantial reciprocal crosstalk between cell-cell or cell-non-cell components (6). TME is generally a complex network and largely composed of the stroma of tumor, covering mesenchymal stem cells (MSC), cancer-associated fibroblasts (CAF), endothelial cells (EC), pericytes, immune cells, vasculature together with the extracellular matrix (ECM) surrounding the cancerous tissue (7–9). Contrast to stroma under normal physiological conditions, which encompasses structural and supportive framework to maintain the stability of tissues and suppresses cancer proliferation, the tumor stroma is in an active state and has been documented to be firmly correlated with the aggressiveness and unfavorable clinical outcomes of a spread of malignancies including GC (10–12). A cohort study with 583 gastric adenocarcinomas demonstrated that stroma-rich patients tend to acquire a worse 5-year prognosis than stroma-poor ones, no matter in intestinal or diffuse histological phenotype (13). Other studies suggest tumor-stroma ratio a reliable prognostic indicator to optimize risk stratification in GC for the ability to quantify the effect of tumor-stroma interactions on tumor biology (14, 15). Such evidence sheds light on the important role of stroma for tumor development, which would arise novel anti-cancer strategies focusing not restrictive on cancer cells.
Among the stromal cells, MSC, a population of non-hematopoietic cells with self-renewal capacity, multi-differential potential, and immune-modulatory property, have received recent attention as a key contributor in directing tumor behavior and TME remodeling. MSC are spindle-shaped cells and capable of forming colonies when originally isolated from the hematopoietic microenvironment of bone marrow (BM), also named colony-forming unit-fibroblast (CFU-F) (16). Then the International Society for Cellular Therapy (ISCT) enacted the minimal criteria for better isolation of these cells: firstly, be plastic-adherent; secondly, highly express (>95%) surface molecules of CD73, CD90, CD105 and lack expression of (<2%) CD45, CD34, CD14, CD11b, CD79α, CD19 and HLA-DR; thirdly, hold the ability to differentiate into osteoblasts, adipocytes, and chondroblasts ex vivo (17). Over time, other surface antigens, like CD271, STRO-1, CD106 and CD146 come to be accepted and recognized for MSC identification (18, 19). MSC used to arouse excitement for regenerative medicine owing to they can quickly engraft to inflammatory cytokines or chemoattractant gradients produced by injured tissue and organs to exhibit their tissue healing functions, alone or in combination with other methods (20, 21). With the hypothesis “tumor equates wound that never heals” arises, the function of MSC in tumors has been realized to parallel the role of MSC in wound healing that actively promotes MSC from bone marrow or adjacent tissues to mobilize into the TME (22, 23). In a mouse model of hepatocellular carcinoma (HCC), MSC possess distinct homing profiles and contribute to a significant rapid depletion from circulation in cancerous condition (24). The result is consistent with findings in the patients with GC showing that an intensified peripheral trafficking of MSC in comparison to healthy individuals; and the egressed MSC are commonly aggregated in tumor bulks over adjacent normal tissues (25, 26). That throws the great interest to explore the possible roles of MSC in tumor progression and aggressiveness which previously may be neglected in GC-stroma interactions. In the following, we mainly cover recent advances in the interactions between MSC and GC, the role of MSC in rewiring the nearing cancer stroma, and potential underlying mechanisms. Furthermore, fresh boundaries regarding the potential application of MSC in GC are also within our discussion to inspire more preventive and therapeutic strategies.
The Recruitment and Reprogramming Course of Naïve MSC Into GC-MSC
The Tropism and Remodeling of Naïve MSC
GC as well as its progression niche is a reservoir of cytokines, chemokines, growth factors that specifically drive the tropism and motility of BM-derived cells including MSC. Under the co-culture system, the migratory ability of BM-MSC could be raised up to two- to threefold because of the high expression of CXC receptor 2 (CXCR2) in response to CXC ligand 1 (CXCL1), a chemokine stimulating factor released from cancer cells (26). Except for this, chemotactic signals derived from GC such as C-C chemokine ligand 19 (CCL19) and CXCL12 would also augment the migration potential of MSC to cancer in a dose-dependent manner, while the concrete mechanisms still to be further investigated (27). On the other hand, it is important to note that Hp infection would accelerate the trafficking of MSC into the stomach at the early stage of carcinogenesis. Hp-mediated chronic inflammation of gastric epithelial cells would significantly increase the secretion of tumor necrosis factor-alpha (TNF-α), an appreciable molecule in stimulating MSC migration in Nuclear Factor-kappa B (NF-κB)-dependent manner (28). Subsequently to being recruited, the naïve MSC [mainly refers to BM-MSC or MSC isolated from adjacent non-cancerous tissue (GCN-MSC)] were relentless to be educated by GC cells to become specialized ones equipped with the tumor-supporting capacity (29). Moreover, the immunomodulatory function of MSC could also be modified via the activation of the NF-κB signaling pathway, to strengthen their activation ability to immune cells (30). Intriguingly, a recent finding suggested that BM-MSC incorporated into metastatic lymph node microenvironment could be reprogrammed by cancer cells. Yes-associated protein (YAP) activation elicited by exosomal Wnt5a from lymph node-derived GC cells, was verified pivotal for their reprogramming into cancer-associated MSC (31).
Characterization of GC-MSC
Evidence incline to depict that GC-MSC and naïve MSC share equivalent spindle-shaped morphology, similar surface antigens and stem cell-related gene expression. GC-MSC are positive for CD13, CD29, CD44, CD73, CD90 and CD105, but negative for CD14, CD31, CD34, CD38, CD45, CD71, CD133 and HLA-DR, among which the expression of CD105 was strongly associated with the poor prognosis of GC patients (12, 32–34). Another study denoted that the higher co-expression of CD29 and CD90 are more commonly seen in GC-MSC than GCN-MSC, and was correlated with more advanced pathological stage, worse disease-free survival and overall survival (35). In comparison with naïve MSC, GC-MSC seem to be on a less quiescent stage, ultrastructurally, phenotypically and functionally. GC-MSC typically feature greater number of cell organelles, such as mitochondria and endocytoplasmic reticulum, and higher expression of proliferation-related genes, all of which are coincide with their greater proliferative potential (32). Besides, GC-MSC display a stronger intensity of reactive stroma cell markers including fibroblast activation protein (FAP) and α-smooth muscle actin (α-SMA) (36). In virtue of the reprogramming by cancer cells, GC-MSC exhibit a higher secretion of inflammatory cytokines than naïve MSC, e.g., interleukin-6 (IL-6), IL-8, transforming growth factor β1 (TGF-β1), ect, which in turn display superior efficiency in facilitating cancer cell growth, invasion, migration and tumorigenesis in vitro and in vivo (36, 37).
MSC Impact on GC Cells
Aberrantly Activate Oncogenic Cell Signaling
MSC are capable of reprogramming GC cells to orchestrate the proliferation, invasion, migration and chemoresistance, via stimulation of oncogenic signaling pathways associated with aberrantly growth or transforming (Figure 1). The enhanced secretion of IL-8 from GC-MSC has been linked to cancer progression by inducing the activation of protein kinase B (AKT) and extracellular signal-regulated protein kinase (ERK) 1/2 signaling pathway (37). Moreover, GC-MSC could robustly express hepatocyte growth factor (HGF) as ligand of c‐MET to trigger phosphorylation of its downstream signaling cascade in cancer cells, and aberrant HGF/c-MET axis has been well-established to be critical for GC progression (38). Recently, researches highlighted the crucial mediator of CXCR6 and signal transducer and activator of transcription 3 (STAT3) pathway, which suggest a prominent production of CXCL16 in MSC via the activation of Wnt5a/Ror2 signaling axis, in turn, activates its corresponding receptor CXCR6 to increase the expression of Ror1 via the activation of STAT3, eventually resulting in the promotion of proliferation and migration of GC cells (39, 40). In addition to these, emerging studies have uncovered the oncogenic potential of platelet-derived growth factor (PDGF)-DD/PDGFR-β axis (41) and YAP/β-catenin signaling (42) in the MSC-induced cancer initiation and progression, and blocking or interference of these signals has designated a certain positive significance for the treatment of GC.
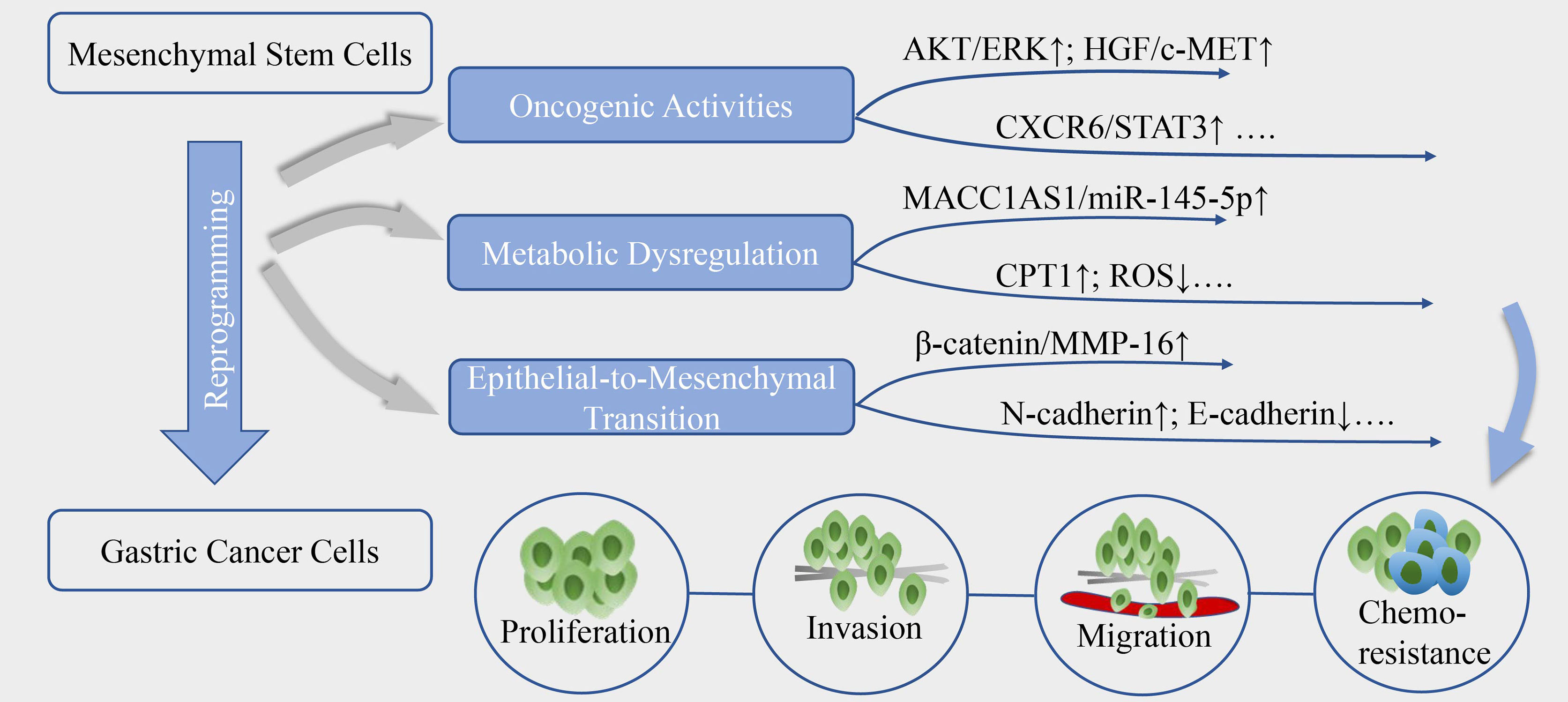
Figure 1 The reprogramming of GC by MSC. MSC could reprogram the biological activities of gastric cancer cells, mainly through aberrantly activate oncogenic signals, metabolic reprogramming and epithelial-to-mesenchymal transition, contributing to cancer cells proliferation, invasion, migration and resistance to chemotherapy.
Exosomes prove a kind of small lipid bilayer membrane vesicles delivering intercellular communication bioactive molecules, like miRNAs, long non-coding RNAs (lncRNAs), and proteins to reshape the biological behavior of adjacent cells or remote targets of the body (43, 44). As research conducted by Ji et al. (45), the exosomes from MSC can trigger the calcium-dependent protein kinases (CaM-Ks) and its downstream RAF/MEK/ERK signaling cascade, elevating the expression of multi-drug resistant proteins in cancer cells to counteract 5-fluorouracil (5-FU) induced cell apoptosis, however, the exact molecules in exosomes that mediate this effect have yet been identified. Ubiquitin-protein ligase E3 component n-recognin 2 (UBR2) is a kind of protein that accounts for ubiquitination and degradation, was found to highly aggregate in exosomes of especially p53 deficient BM-MSC, could be internalized into GC cells and induce the activation of Wnt/β-catenin pathway to promote cell proliferation, migration and stemness maintenance (46). In addition, evidence has clarified that miRNAs are deregulated in exosomes of GC-MSC, to be transferred to transcriptionally modulate cancer aggressiveness, of which miR-221 deregulation has been linked with various tumorigenic pathways (47, 48).
Dysregulate Metabolic Plasticity
Metabolic reprogramming has been proposed as a new hallmark for cancer progression. Emerging discoveries highlight that MSC could dysregulate cell metabolism to confer GC cells stemness and tolerance to drug stress (Figure 1). He et al. (35) proposed that TGF-β1 secretion by MSC co-opt TGF-β receptors in GC cells could raise the expression level of lncRNA MACC1-AS1, which have sponge interaction with miR-145-5p to boost the expression of CPT1, the fatty acid oxidation (FAO) speed-limiting enzyme, subsequently decreasing reactive oxygen species (ROS) production and cell apoptosis under 5-FU and oxaliplatin. Mechanistically, MACC1-AS1 also is found to augment the expression of MACC1, an oncogene and a poor prognosis marker in GC, to elevate glutathione and nicotinamide adenine dinucleotide phosphate (NADPH) levels and sustain a lower ROS load under metabolic stress (49). In another study, MSC-induced lncRNA histocompatibility leukocyte antigen complex P5 (HCP5) in GC cells was demonstrated to serve as a miR-3619-5p sponge to facilitate FAO via the transactivation of CPT1, thereby alleviating the cell cycle arrest effect of cancer cells caused by chemotherapeutics (50). Besides, the antagonist of CPT-1 could remarkably reverse the MSC-induced tumor growth under FOLFOX regiment treatment in vivo (35), indicating that deregulated FAO could be a key regulator of MSC-mediated chemoresistance in GC and a potential target for anti-resistance interventions.
Elicit Epithelial-to-Mesenchymal Transition (EMT)
Phenotypic transition occurs in GC cells when close physical contact with MSC as well (Figure 1), they adopt mesenchymal phenotype including longitude ridging, ruffled membranes and finger-like extensions, concomitantly with increased level of α-SMA, N-cadherin, vimentin and Snail and repressed expression of cellular adhesion molecules especially E-cadherin (51–53). EMT is a dynamic process and the mesenchymal traits endow the malignant cells migratory and invasive capacities, and the susceptibility to cancer intravasation and metastasis. The paracrine signals of MSC also induce EMT to promote transendothelial migration, mechanistically, dependent on high expression of snail, twist, β-catenin and matrix metalloproteinase-16 (MMP-16) (52). Moreover, the activation of PI3K/AKT signaling pathway was shown to be linked with the process (51). Intriguingly, researchers found cell fusion maybe one of the underlying mechanisms in the MSC-primed EMT. Hybrids acquire the mesenchymal and stemness proteins, enhanced proliferation and migration potential during a physical fusion event with MSC (54). Notably, hybrids generated by MSC and immortalized non-tumorigenic human gastric epithelial cells also undergo EMT and are vulnerable to be malignant transformation, which makes a difference in cancer initiation (55).
MSC Remodeling the GC Stroma
Immunosuppression Potential of MSC
Not only function directly back upon tumor cells to boost the growth and progression, but MSC are also involved in the continuous updating and transformation of stroma components, for the aim of enhancing their tumor-supporting roles and accommodating the rapid metabolic process of tumors (Figure 2). The immunomodulatory property of MSCs has been well exploited to prevent and treat severe graft-verse-host diseases (56). With the reprogramming of GC, MSC incorporation engraftment the TME could modulate the differentiation, polarization or anergy of immune cells thus offering local immune-suppressive milieu. GC-MSC, mainly by extracellular cytokines secretion, such as IL-15 contained in the conditioned medium (CM) could predispose peripheral blood mononuclear cells (PBMC) to skew their differentiation into regulatory T (Treg) population (57). The balance of Treg and Th17 subsets defines a key regulator for immune homeostasis for they exert the opposite immune-modulatory functions (58). Consistently, a study conducted by Wang et al. (59) revealed that by the joint activation of GC-MSC, the enhanced differentiation of Treg subsets and the suppressed Th17 cells proliferation can reverse the tumor-inhibitory effect of PBMC to significantly improve GC growth potential and facilitate liver metastases formation in vivo. Li and colleagues (60) have shown that GC-MSC also perform an intricate crosstalk with macrophages to drive the conversion of macrophages toward alternatively activated, immunosuppressive M2 phenotype, which exhibit a higher potential in promoting GC invasion and metastasis than that of GC-MSC. Importantly, the M2 phenotype has been manifested to play extensive roles in immune tolerance, neo-angiogenesis, pre-metastatic niche formation for GC advancement (61). Mirroring macrophages, the IL-6 enriched in the GC-MSC-CM can stimulate the phosphorylation of STAT3 and ERK1/2 in neutrophils, promoting their recruitment and activation into a pro-tumor phenotype that would finely cooperate with GC-MSC to synergistically prompt cancer migration and angiogenesis (34). Intriguingly, GC-MSC could induce the anergy and silence of immune potency through the secretion of IL-8 to upregulate the expression level of PD-LI in GC cells that drive the exhaustion of CD8 T cells, resulting in immune resistance and contributing to GC progression (62). Emerging research spots that the immunophenotype of GC-MSC are also influenced by the CD4 T cells via the p-STAT3 signaling pathway to boost cancer growth rate-promoting role of GC-MSCs, highlighting that TME is a huge complicated signaling network in which tumor cells, immune cells, MSC and other components are in a multi-angle communication to facilitate cancer progression (63).
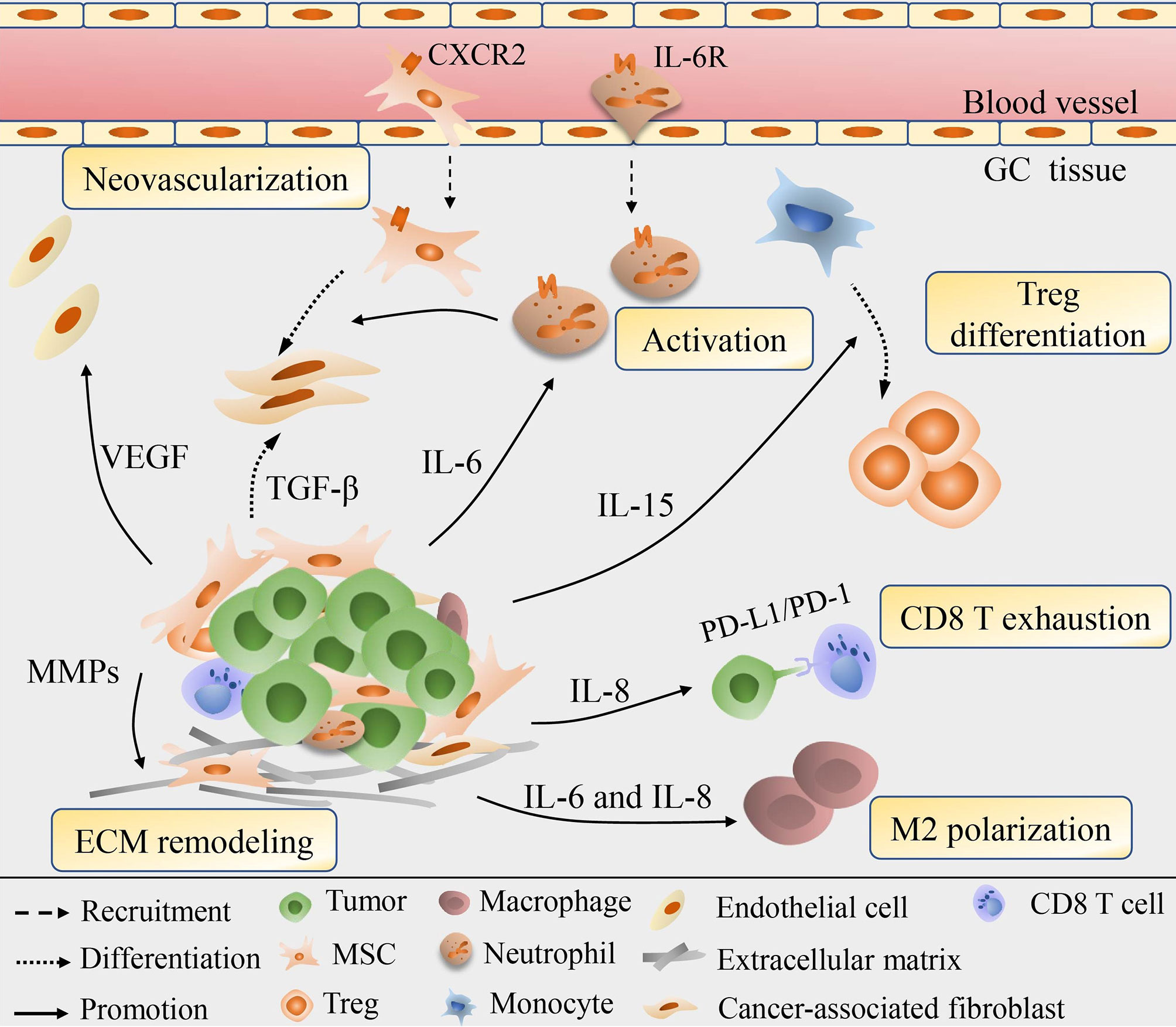
Figure 2 Schematic diagram for the role of MSC in the remodeling of GC stroma. MSC could be recruited into cancer stroma and are involved in the continuous updating and transformation of stroma components. Via secretion of various cytokines, e.g., IL-6, IL-8, IL-15, MSC modulate the Treg differentiation, macrophage polarization, neutrophil recruitment and activation, exhaustion of CD8 T cells, thus offering local immune-suppressive milieu. MSC could also secret VEGF and MMPs to induce neovascularization and ECM degrading, or directly differentiate into CAF to exhibit synergistic effect in remodeling cancer cells and stroma.
Vascularization and ECM Remodeling
Neovascularization provides favorable conditions for tumor invasion and metastasis and is therefore considered a marker of poor prognosis. Under the pretreatment of GC-CM in vitro, MSC would elevate the expression of vascular endothelial growth factor (VEGF), macrophage inflammatory protein-2 (MIP-2), TGF-β1, IL-6, and IL-8, among them VEGF being highly angiogenic works prominently in vascular development (37). Consistent with the prior finding, Feng et al. (64) proposed that VEGF production, in collaboration with NF-κB signaling in GC-MSC, could induce angiogenesis through driving the human umbilical vein endothelial cells (HUVEC) tube formation, suggesting the active involvement of GC-MSC in tumor neovascularization, while the inherent regulation mechanisms in VEGF/NF-κB signaling remains unquestioned. MSC are observed to trans-differentiation into EC with the induction of basic fibroblast growth factor (bFGF) or VEGF, and RNA chip result indicates the intrinsic epigenetic modifications of MSC affect their differentiation into multiple cell lineages including EC that reshape the surrounding pathological GC stroma, hence MSC probably directly give rise to EC to contribute to GC vascular network (65–67). Additionally, experimental evidence shows that MSC are able to give rise to CAF with the activation of TGF-β derived from GC-exosomes (68). CAF are well-demonstrated stromal cells and are often coordinated and overlapping with GC-MSC in reprogramming tumor itself and the stroma vicinity to contribute to cancer invasion and migration, acquired chemoresistance and EMT (69–71). Hp infection in GC cells would therefore enhance the expression of hepatoma-derived growth factor (HDGF) which also accelerates the transition of MSC into CAF and amplify their synergistic effects (72). Former studies stressed the role of CAF in ECM remodeling that control the aggressiveness and metastasis of cancer cells (73, 74). However, newly data indicate that secretion of MMP-2, MMP-7, MMP-9 and MMP-14, matrix metalloproteinases needed for ECM degradation are also increased in MSC to destruct external barriers to facilitate GC invasion and migration (75).
The Prospects of MSC in Targeted Therapy
MSC Act as Drug Delivery Vehicles
With their inherent advantages, like tumor and metastases tropism, easy isolation, low immunogenicity, MSC are ideal vehicles for tumor-directed therapy to raise efficacy (Table 1). Recently, a novel polymer AHP-OA-FA has been employed to infuse with MSC that enhance the tropism into GC with increased drug concentration and aggregation in local tumor lesions than pure MSC, and the polymer exerts no impact on the surface marker, proliferative capacity and motility of MSC, thus could be serving as more potent carriers for targeted therapy (84). Prerequisites for application of MSC are that the therapeutic agents they transported can be released after reaching the tumor site and exhibit strong toxicity only to tumor tissues. Cai and coworkers (76) established immuneapoptotin-armored MSC which continually secrete immuneapoptotin e23sFv-Fdt-tBid and exhibit significantly killing effect to GC cells expressing HER2 in vitro study. Importantly, after being intravenously administered in HER2 reconstituted syngeneic mouse models, these primed MSC exhibit persistent localization at tumor areas, display markedly stronger immunoapoptotin staining and better anti-tumor effect in comparison with direct delivery of the purified immunoapoptotin or delivery by Jurkat cells, indicating that MSC mobility can be well extended for the specific killing of HER-2 overexpressed GC. Besides, MSC expressing a transgene encoding NK4, the antagonist of HGF receptors, were observed to migrate and accumulate in tumor tissue, and effectively inhibit GC growth via suppressing tumor angiogenesis as well as triggering tumor cell apoptosis (77). In much the same way, umbilical cord blood (UCB)-MSC being infected with lentivirus vectors carrying LIGHT (TNF receptor superfamily) genes are also reported to induce severer apoptosis of GC cells (78). These trails illustrate that MSC as vectors possess a large scope of complexity, including apoptosis-inducing genes, oncolytic viruses, cytotoxic agents and anti-angiogenic agents. To our interest, a recent study demonstrated that MSC could also be modified to carry hemoglobin genes and supply oxygen to GC cells to reverse the hypoxic microenvironment and reduce the resistance to cisplatin and 5-FU (79). However, there are still lacking in evidence to ensure that the engineered MSC do not cause tumor progression or recurrence after long periods of infiltrating in cancer stroma. The first prospective, uncontrolled, single-arm phase I/II study on MSC-based therapy using autologous genetically modified MSCs in advanced gastrointestinal adenocarcinoma (TREAT-ME1) has been finished and suggested that MSC_apceth_101 cells (a total dose of 3.0×106 cells/kg) expressing herpes simplex virus tyrosine kinase (HSV-TK) combined with ganciclovir was safe and could be well tolerated (85, 86). While it is a small study with 10 patients and no GC cases included, thus future multicenter investigations with larger samples are warranted to realize the safe and effective transformation of MSC-based therapy into GC settings.
Target the Recruitment Course of MSC and the Downstream Vicious Signals
In most circumstances, the active recruitment of MSC commonly occurs ahead of GC initiation, especially in Hp-related carcinogenesis. Hp induced inflammation milieu is abundant in functional molecules such as TNF-α, TGF-β, CXCL12 and interferon γ that conducive to MSC recruitment for their tissue healing functions (28, 87–89). Corresponding to this point, Ruan et al. (27) labeled MSC with amino-modified FMNP that keep stable fluorescent signal and magnetic properties with 14 days to display out the early gastric cancer (EGC) area, not only being with the potential of imaging EGC, these MSC also could inhibit tumor growth markedly under alternating magnetic field irradiation. MSC aggregating at inflamed stomach further prompts gastric carcinogenesis mainly through EMT once they fail to repair (55, 90). Hence earlier Hp eradication or intercepting MSC recruitment would make a difference to suspend carcinogenesis and progression (Table 1). Prior study has manifested that the AMD3100, an inhibitor of the CXCL12/CXCR4 signaling axis, could block the transformation of MSC into α-SMA+ myofibroblasts and the recruitment of MSC, inhibiting tumor growth and the development of gastric dysplasia (80). Similarly, the administration of antagonists of the CXCL1/CXCR2 axis was found to block the MSC recruitment in the GC mice model, decreasing the size of tumors as well as the number of lymph node metastases (26). On the other hand, directly disrupt inter-communication between MSC and GC also offer novel insights for GC treatment (Table 1). Such as resveratrol, which could suppress and revert the pro-metastatic effect of GC-MSC via counteracting GC-MSC-mediated Wnt/β-catenin signaling of GC cells (81). Curcumin is a bioactive compound and found to abrogate the NF-κB signaling and VEGF production to attenuate the GC-MSC-triggered tumor angiogenesis (64). In addition to these, several researchers have successfully adopted specific neutralizing antibodies of IL-8, IL-6, and CCL-5 to inhibit MSC-mediated GC invasive motility, and 17β- estradiol also impair the functions of IL-8, IL-6, and CCL-5 under the same context via ceasing the activation of their downstream Src/Cas/Paxillin signaling pathway, thus hormonal therapy might be anticipated based on MSC activity (82, 83, 91).
Conclusions
MSC, with their multi-lineage differentiation and immune privilege nature, have shared great popularity in regenerative medicine and allogeneic transplantation. As the stromal progenitor cells, their role in tumor progression and TME is being put under the spotlight of tumor researches. Most studies have confirmed the tumor-contributing role of MSC, while the anti-tumor effect of MSC gradually unveils in several cancer types such as melanoma; glioma; HCC (92–94). Also, there are opposite results clarifying that MSC from human adipose tissue and the umbilical cord could inhibit GC progression and induce apoptosis of cancer cells (95, 96). One convincing explanation of the discrepancy attaches importance on the process of reprogramming of tumor cells that convert naïve MSC, which often exert a divergent effect on tumor progression, into pro-tumorigenic educated tumor-associated MSC. However, other factors, like the differences among tumor models, the heterogeneity of MSC, the timing and dose of the MSC injected are also accepted to influence the process of MSC-cancer interactions and lead to inconsistent results [reviewed in (97, 98)].
This review stresses the reciprocal crosstalk of malignant cells and MSC in the progression of GC, which can partly account for the complexity and heterogeneity of tumor-stroma connections. GC and the secret mediators in the niche would induce MSC recruitment and educate them into cancer-associated MSC with stronger tumor-promoting potential. In response, through cell physical contact or secretomes, MSC could aberrantly motivate oncogenic signals, deregulate metabolic plasticity and elicit EMT in GC cells to promote proliferation, invasion, migration and chemoresistance. Indirectly, reprogrammed MSC can deliver their signals horizontally to non-tumor cells in the TME to boost their pro-tumor functions with the repression of local immune response, stimulation of tumor angiogenesis and ECM remodeling. Given that MSC homing occurs early in the precancerous stage, they can be used for the detection of EGC. And they are also ideal carriers to deliver anti-cancer agents to tumor lesions with their low immunogenicity and well-accommodation, an increased concentration and lethality of drugs in target tissues would be expected. While further researches are warranted to identify whether the tumor-promoting role of MSC would override the inhibiting effect from drugs they delivered. In addition, the reciprocal reprogramming of MSC and GC as well as their domino effect spread to the TME prove beneficial for tumor growth and progression, strategies intercepting these vicious signaling connections represent a hopeful prospect in GC treatment. Taken together, the reciprocal reprogramming of GC and MSC triggers more active tumor-supporting signals that sustain tumor progression and remodel the surrounding pathological stroma. The tumor tropism nature of MSC and their extensive roles in GC deserve more in-depth investigation as they earn promising targets for cutting-edge cancer treatments.
Author Contributions
YL wrote the review. XZ and YZ were responsible for figure and legend and final editing. XL contributed to conceiving the concept, analyzing the article and preparing for submission. All authors contributed to the article and approved the submitted version.
Funding
This study was supported by research grants from Zhejiang Provincial National Science Foundation of China (Grant no.LY16H160031).
Conflict of Interest
The authors declare that the research was conducted in the absence of any commercial or financial relationships that could be construed as a potential conflict of interest.
References
1. Bray F, Ferlay J, Soerjomataram I, Siegel RL, Torre LA, Jemal A. Global Cancer Statistics 2018: GLOBOCAN Estimates of Incidence and Mortality Worldwide for 36 Cancers in 185 Countries. Ca-a Cancer J Clin (2018) 68(6):394–424. doi: 10.3322/caac.21492
2. Digklia A, Wagner AD. Advanced Gastric Cancer: Current Treatment Landscape and Future Perspectives. World J Gastroenterol (2016) 22(8):2403–14. doi: 10.3748/wjg.v22.i8.2403
3. Kim YI, Kim YA, Kim CG, Ryu KW, Kim YW, Sim JA, et al. Serial Intermediate-Term Quality of Life Comparison After Endoscopic Submucosal Dissection Versus Surgery in Early Gastric Cancer Patients. Surg Endoscopy (2018) 32(4):2114–22. doi: 10.1007/s00464-017-5909-y
4. Malfertheiner P, Megraud F, O’Morain CA, Gisbert JP, Kuipers EJ, Axon AT, et al. Management of Helicobacter Pylori Infection-the Maastricht V/Florence Consensus Report. Gut (2017) 66(1):6–30. doi: 10.1136/gutjnl-2016-312288
5. Nelen SD, van Putten M, Lemmens V, Bosscha K, de Wilt JHW, Verhoeven RHA. Effect of Age on Rates of Palliative Surgery and Chemotherapy Use in Patients With Locally Advanced or Metastatic Gastric Cancer. Br J Surg (2017) 104(13):1837–46. doi: 10.1002/bjs.10621
6. Fidler IJ. The Pathogenesis of Cancer Metastasis: The ‘Seed and Soil’ Hypothesis Revisited. Nat Rev Cancer (2003) 3(6):453–8. doi: 10.1038/nrc1098
7. Atiya H, Frisbie L, Pressimone C, Coffman L. Mesenchymal Stem Cells in the Tumor Microenvironment. Adv Exp Med Biol (2020) 1234:31–42. doi: 10.1007/978-3-030-37184-5_3
8. Valkenburg KC, de Groot AE, Pienta KJ. Targeting the Tumour Stroma to Improve Cancer Therapy. Nat Rev Clin Oncol (2018) 15(6):366–81. doi: 10.1038/s41571-018-0007-1
9. Kucerova L, Skolekova S. Tumor Microenvironment and the Role of Mesenchymal Stromal Cells. Neoplasma (2013) 60(1):1–10. doi: 10.4149/neo_2013_001
10. Li J, Pu T, Yin L, Li Q, Liao CP, Wu BJ. MAOA-Mediated Reprogramming of Stromal Fibroblasts Promotes Prostate Tumorigenesis and Cancer Stemness. Oncogene (2020) 39(16):3305–21. doi: 10.1038/s41388-020-1217-4
11. Dourado MR, Miwa KYM, Hamada GB, Paranaíba LMR, Sawazaki-Calone Í, Domingueti CB, et al. Prognostication for Oral Squamous Cell Carcinoma Patients Based on the Tumour-Stroma Ratio and Tumour Budding. Histopathology (2020) 76(6):906–18. doi: 10.1111/his.14070
12. Numakura S, Uozaki H, Kikuchi Y, Watabe S, Togashi A, Watanabe M. Mesenchymal Stem Cell Marker Expression in Gastric Cancer Stroma. Anticancer Res (2019) 39(1):387–93. doi: 10.21873/anticanres.13124
13. Kemi N, Eskuri M, Herva A, Leppänen J, Huhta H, Helminen O, et al. Tumour-Stroma Ratio and Prognosis in Gastric Adenocarcinoma. Br J Cancer (2018) 119(4):435–9. doi: 10.1038/s41416-018-0202-y
14. Kemi N, Eskuri M, Kauppila JH. Tumour-Stroma Ratio and 5-Year Mortality in Gastric Adenocarcinoma: A Systematic Review and Meta-Analysis. Sci Rep (2019) 9(1):16018. doi: 10.1038/s41598-019-52606-7
15. Peng C, Liu J, Yang G, Li Y. The Tumor-Stromal Ratio as a Strong Prognosticator for Advanced Gastric Cancer Patients: Proposal of a New TSNM Staging System. J Gastroenterol (2018) 53(5):606–17. doi: 10.1007/s00535-017-1379-1
16. Friedenstein AJ, Chailakhjan RK, Lalykina KS. The Development of Fibroblast Colonies in Monolayer Cultures of Guinea-Pig Bone Marrow and Spleen Cells. Cell Tissue Kinetics (1970) 3(4):393–403. doi: 10.1111/j.1365-2184.1970.tb00347.x
17. Dominici M, Le Blanc K, Mueller I, Slaper-Cortenbach I, Marini F, Krause D, et al. Minimal Criteria for Defining Multipotent Mesenchymal Stromal Cells. The International Society for Cellular Therapy Position Statement. Cytotherapy (2006) 8(4):315–7. doi: 10.1080/14653240600855905
18. Álvarez-Viejo M, Menéndez-Menéndez Y, Otero-Hernández J. CD271 as a Marker to Identify Mesenchymal Stem Cells From Diverse Sources Before Culture. World J Stem Cells (2015) 7(2):470–6. doi: 10.4252/wjsc.v7.i2.470
19. Lv FJ, Tuan RS, Cheung KM, Leung VY. Concise Review: The Surface Markers and Identity of Human Mesenchymal Stem Cells. Stem Cells (Dayton Ohio) (2014) 32(6):1408–19. doi: 10.1002/stem.1681
20. Sarveazad A, Babahajian A, Yari A, Rayner CK, Mokhtare M, Babaei-Ghazani A, et al. Combination of Laser and Human Adipose-Derived Stem Cells in Repair of Rabbit Anal Sphincter Injury: A New Therapeutic Approach. Stem Cell Res Ther (2019) 10(1):367. doi: 10.1186/s13287-019-1477-5
21. Chetty SS, Praneetha S, Govarthanan K, Verma RS, Vadivel Murugan A. Noninvasive Tracking and Regenerative Capabilities of Transplanted Human Umbilical Cord-Derived Mesenchymal Stem Cells Labeled With I-III-IV Semiconducting Nanocrystals in Liver-Injured Living Mice. ACS Appl Mater Interfaces (2019) 11(9):8763–78. doi: 10.1021/acsami.8b19953
22. Kidd S, Spaeth E, Dembinski JL, Dietrich M, Watson K, Klopp A, et al. Direct Evidence of Mesenchymal Stem Cell Tropism for Tumor and Wounding Microenvironments Using. Vivo Biolumin Imaging Stem Cells (Dayton Ohio) (2009) 27(10):2614–23. doi: 10.1002/stem.187
23. Dvorak HF. Tumors: Wounds That do Not Heal. Similarities Between Tumor Stroma Generation and Wound Healing. New Engl J Med (1986) 315(26):1650–9. doi: 10.1056/nejm198612253152606
24. Xie C, Yang Z, Suo Y, Chen Q, Wei D, Weng X, et al. Systemically Infused Mesenchymal Stem Cells Show Different Homing Profiles in Healthy and Tumor Mouse Models. Stem Cells Trans Med (2017) 6(4):1120–31. doi: 10.1002/sctm.16-0204
25. Błogowski W, Zuba-Surma E, Sałata D, Budkowska M, Dołęgowska B, Starzyńska T. Peripheral Trafficking of Bone-Marrow-Derived Stem Cells in Patients With Different Types of Gastric Neoplasms. Oncoimmunology (2016) 5(4):e1099798. doi: 10.1080/2162402x.2015.1099798
26. Kasashima H, Yashiro M, Nakamae H, Kitayama K, Masuda G, Kinoshita H, et al. Cxcl1-Chemokine (C-X-C Motif) Receptor 2 Signaling Stimulates the Recruitment of Bone Marrow-Derived Mesenchymal Cells Into Diffuse-Type Gastric Cancer Stroma. Am J Pathol (2016) 186(11):3028–39. doi: 10.1016/j.ajpath.2016.07.024
27. Ruan J, Ji J, Song H, Qian Q, Wang K, Wang C, et al. Fluorescent Magnetic Nanoparticle-Labeled Mesenchymal Stem Cells for Targeted Imaging and Hyperthermia Therapy of. Vivo Gastric Cancer Nanoscale Res Lett (2012) 7(1):309. doi: 10.1186/1556-276x-7-309
28. Ferrand J, Lehours P, Schmid-Alliana A, Mégraud F, Varon C. Helicobacter Pylori Infection of Gastrointestinal Epithelial Cells in vitro induces mesenchymal stem cell migration through an NF-κB-dependent pathway. PloS One (2011) 6(12):e29007. doi: 10.1371/journal.pone.0029007
29. Shamai Y, Alperovich DC, Yakhini Z, Skorecki K, Tzukerman M. Reciprocal Reprogramming of Cancer Cells and Associated Mesenchymal Stem Cells in Gastric Cancer. Stem Cells (Dayton Ohio) (2019) 37(2):176–89. doi: 10.1002/stem.2942
30. Shen Y, Xue C, Li X, Ba L, Gu J, Sun Z, et al. Effects of Gastric Cancer Cell-Derived Exosomes on the Immune Regulation of Mesenchymal Stem Cells by the NF-kB Signaling Pathway. Stem Cells Dev (2019) 28(7):464–76. doi: 10.1089/scd.2018.0125
31. Wang M, Zhao X, Qiu R, Gong Z, Huang F, Yu W, et al. Lymph Node Metastasis-Derived Gastric Cancer Cells Educate Bone Marrow-Derived Mesenchymal Stem Cells via YAP signaling activation by exosomal Wnt5a. Oncogene (2021) 40(12):2296–308. doi: 10.1038/s41388-021-01722-8
32. Cao H, Xu W, Qian H, Zhu W, Yan Y, Zhou H, et al. Mesenchymal Stem Cell-Like Cells Derived From Human Gastric Cancer Tissues. Cancer Lett (2009) 274(1):61–71. doi: 10.1016/j.canlet.2008.08.036
33. Xu X, Zhang X, Wang S, Qian H, Zhu W, Cao H, et al. Isolation and Comparison of Mesenchymal Stem-Like Cells From Human Gastric Cancer and Adjacent non-Cancerous Tissues. J Cancer Res Clin Oncol (2011) 137(3):495–504. doi: 10.1007/s00432-010-0908-6
34. Zhu Q, Zhang X, Zhang L, Li W, Wu H, Yuan X, et al. The IL-6-STAT3 Axis Mediates a Reciprocal Crosstalk Between Cancer-Derived Mesenchymal Stem Cells and Neutrophils to Synergistically Prompt Gastric Cancer Progression. Cell Death Dis (2014) 5(6):e1295. doi: 10.1038/cddis.2014.263
35. He W, Liang B, Wang C, Li S, Zhao Y, Huang Q, et al. MSC-Regulated Lncrna MACC1-AS1 Promotes Stemness and Chemoresistance Through Fatty Acid Oxidation in Gastric Cancer. Oncogene (2019) 38(23):4637–54. doi: 10.1038/s41388-019-0747-0
36. Zhu M, Wang M, Yang F, Tian Y, Cai J, Yang H, et al. miR-155-5p Inhibition Promotes the Transition of Bone Marrow Mesenchymal Stem Cells to Gastric Cancer Tissue Derived MSC-like Cells via NF-κB p65 activation. Oncotarget (2016) 7(13):16567–80. doi: 10.18632/oncotarget.7767
37. Li W, Zhou Y, Yang J, Zhang X, Zhang H, Zhang T, et al. Gastric Cancer-Derived Mesenchymal Stem Cells Prompt Gastric Cancer Progression Through Secretion of Interleukin-8. J Exp Clin Cancer Res: CR (2015) 34(1):52. doi: 10.1186/s13046-015-0172-3
38. Berger L, Shamai Y, Skorecki KL, Tzukerman M. Tumor Specific Recruitment and Reprogramming of Mesenchymal Stem Cells in Tumorigenesis. Stem Cells (Dayton Ohio) (2016) 34(4):1011–26. doi: 10.1002/stem.2269
39. Takiguchi G, Nishita M, Kurita K, Kakeji Y, Minami Y. Wnt5a-Ror2 Signaling in Mesenchymal Stem Cells Promotes Proliferation of Gastric Cancer Cells by Activating CXCL16-CXCR6 Axis. Cancer Sci (2016) 107(3):290–7. doi: 10.1111/cas.12871
40. Ikeda T, Nishita M, Hoshi K, Honda T, Kakeji Y, Minami Y. Mesenchymal Stem Cell-Derived CXCL16 Promotes Progression of Gastric Cancer Cells by STAT3-mediated Expression of Ror1. Cancer Sci (2020) 111(4):1254–65. doi: 10.1111/cas.14339
41. Pan Z, Tian Y, Zhang B, Zhang X, Shi H, Liang Z, et al. YAP Signaling in Gastric Cancer-Derived Mesenchymal Stem Cells is Critical for its Promoting Role in Cancer Progression. Int J Oncol (2017) 51(4):1055–66. doi: 10.3892/ijo.2017.4101
42. Huang F, Wang M, Yang T, Cai J, Zhang Q, Sun Z, et al. Gastric Cancer-Derived MSC-secreted Pdgf-DD Promotes Gastric Cancer Progression. J Cancer Res Clin Oncol (2014) 140(11):1835–48. doi: 10.1007/s00432-014-1723-2
43. Zhang X, Yuan X, Shi H, Wu L, Qian H, Xu W. Exosomes in Cancer: Small Particle, Big Player. J Hematol Oncol (2015) 8:83. doi: 10.1186/s13045-015-0181-x
44. Lou G, Chen Z, Zheng M, Liu Y. Mesenchymal Stem Cell-Derived Exosomes as a New Therapeutic Strategy for Liver Diseases. Exp Mol Med (2017) 49(6):e346. doi: 10.1038/emm.2017.63
45. Ji R, Zhang B, Zhang X, Xue J, Yuan X, Yan Y, et al. Exosomes Derived From Human Mesenchymal Stem Cells Confer Drug Resistance in Gastric Cancer. Cell Cycle (Georgetown Tex) (2015) 14(15):2473–83. doi: 10.1080/15384101.2015.1005530
46. Mao J, Liang Z, Zhang B, Yang H, Li X, Fu H, et al. Ubr2 Enriched in P53 Deficient Mouse Bone Marrow Mesenchymal Stem Cell-Exosome Promoted Gastric Cancer Progression via Wnt/β-Catenin Pathway. Stem Cells (Dayton Ohio) (2017) 35(11):2267–79. doi: 10.1002/stem.2702
47. Wang M, Zhao C, Shi H, Zhang B, Zhang L, Zhang X, et al. Deregulated microRNAs in Gastric Cancer Tissue-Derived Mesenchymal Stem Cells: Novel Biomarkers and a Mechanism for Gastric Cancer. Br J Cancer (2014) 110(5):1199–210. doi: 10.1038/bjc.2014.14
48. Ma M, Chen S, Liu Z, Xie H, Deng H, Shang S, et al. miRNA-221 of Exosomes Originating From Bone Marrow Mesenchymal Stem Cells Promotes Oncogenic Activity in Gastric Cancer. OncoTargets Ther (2017) 10:4161–71. doi: 10.2147/ott.S143315
49. Zhao Y, Liu Y, Lin L, Huang Q, He W, Zhang S, et al. The Lncrna MACC1-AS1 Promotes Gastric Cancer Cell Metabolic Plasticity via AMPK/Lin28 mediated mRNA stability of MACC1. Mol Cancer (2018) 17(1):69. doi: 10.1186/s12943-018-0820-2
50. Wu H, Liu B, Chen Z, Li G, Zhang Z. MSC-Induced Lncrna HCP5 Drove Fatty Acid Oxidation Through Mir-3619-5p/AMPK/PGC1α/CEBPB Axis to Promote Stemness and Chemo-Resistance of Gastric Cancer. Cell Death Dis (2020) 11(4):233. doi: 10.1038/s41419-020-2426-z
51. Gu H, Ji R, Zhang X, Wang M, Zhu W, Qian H, et al. Exosomes Derived From Human Mesenchymal Stem Cells Promote Gastric Cancer Cell Growth and Migration via the activation of the Akt pathway. Mol Med Rep (2016) 14(4):3452–8. doi: 10.3892/mmr.2016.5625
52. Xue Z, Wu X, Chen X, Liu Y, Wang X, Wu K, et al. Mesenchymal Stem Cells Promote Epithelial to Mesenchymal Transition and Metastasis in Gastric Cancer Though Paracrine Cues and Close Physical Contact. J Cell Biochem (2015) 116(4):618–27. doi: 10.1002/jcb.25013
53. Nishimura K, Semba S, Aoyagi K, Sasaki H, Yokozaki H. Mesenchymal Stem Cells Provide an Advantageous Tumor Microenvironment for the Restoration of Cancer Stem Cells. Pathobiol: J Immunopathol Mol Cell Biol (2012) 79(6):290–306. doi: 10.1159/000337296
54. Xue J, Zhu Y, Sun Z, Ji R, Zhang X, Xu W, et al. Tumorigenic Hybrids Between Mesenchymal Stem Cells and Gastric Cancer Cells Enhanced Cancer Proliferation, Migration and Stemness. BMC Cancer (2015) 15:793. doi: 10.1186/s12885-015-1780-1
55. He X, Li B, Shao Y, Zhao N, Hsu Y, Zhang Z, et al. Cell Fusion Between Gastric Epithelial Cells and Mesenchymal Stem Cells Results in Epithelial-to-Mesenchymal Transition and Malignant Transformation. BMC Cancer (2015) 15:24. doi: 10.1186/s12885-015-1027-1
56. Bonig H, Kuçi Z, Kuçi S, Bakhtiar S, Basu O, Bug G, et al. Children and Adults With Refractory Acute Graft-versus-Host Disease Respond to Treatment With the Mesenchymal Stromal Cell Preparation “Msc-Ffm”-Outcome Report of 92 Patients. Cells (2019) 8(12):1577. doi: 10.3390/cells8121577
57. Sun L, Wang Q, Chen B, Zhao Y, Shen B, Wang X, et al. Human Gastric Cancer Mesenchymal Stem Cell-Derived Il15 Contributes to Tumor Cell Epithelial-Mesenchymal Transitionvia Upregulation Tregs Ratio and PD-1 Expression in CD4(+)T Cell. Stem Cells Dev (2018) 27(17):1203–14. doi: 10.1089/scd.2018.0043
58. Knochelmann HM, Dwyer CJ, Bailey SR, Amaya SM, Elston DM, Mazza-McCrann JM, et al. When Worlds Collide: Th17 and Treg Cells in Cancer and Autoimmunity. Cell Mol Immunol (2018) 15(5):458–69. doi: 10.1038/s41423-018-0004-4
59. Wang M, Chen B, Sun XX, Zhao XD, Zhao YY, Sun L, et al. Gastric Cancer Tissue-Derived Mesenchymal Stem Cells Impact Peripheral Blood Mononuclear Cells via disruption of Treg/Th17 balance to promote gastric cancer progression. Exp Cell Res (2017) 361(1):19–29. doi: 10.1016/j.yexcr.2017.09.036
60. Li W, Zhang X, Wu F, Zhou Y, Bao Z, Li H, et al. Gastric Cancer-Derived Mesenchymal Stromal Cells Trigger M2 Macrophage Polarization That Promotes Metastasis and EMT in Gastric Cancer. Cell Death Dis (2019) 10(12):918. doi: 10.1038/s41419-019-2131-y
61. Gambardella V, Castillo J, Tarazona N, Gimeno-Valiente F, Martínez-Ciarpaglini C, Cabeza-Segura M, et al. The Role of Tumor-Associated Macrophages in Gastric Cancer Development and Their Potential as a Therapeutic Target. Cancer Treat Rev (2020) 86:102015. doi: 10.1016/j.ctrv.2020.102015
62. Sun L, Wang Q, Chen B, Zhao Y, Shen B, Wang H, et al. Gastric Cancer Mesenchymal Stem Cells Derived IL-8 Induces PD-L1 Expression in Gastric Cancer Cells via STAT3/mTOR-c-Myc signal axis. Cell Death Dis (2018) 9(9):928. doi: 10.1038/s41419-018-0988-9
63. Xu R, Zhao X, Zhao Y, Chen B, Sun L, Xu C, et al. Enhanced Gastric Cancer Growth Potential of Mesenchymal Stem Cells Derived From Gastric Cancer Tissues Educated by CD4(+) T Cells. Cell Prolif (2018) 51(2):e12399. doi: 10.1111/cpr.12399
64. Huang F, Yao Y, Wu J, Liu Q, Zhang J, Pu X, et al. Curcumin Inhibits Gastric Cancer-Derived Mesenchymal Stem Cells Mediated Angiogenesis by Regulating NF-κb/VEGF Signaling. Am J Trans Res (2017) 9(12):5538–47.
65. Xu Y, Fu M, Li Z, Fan Z, Li X, Liu Y, et al. A Prosurvival and Proangiogenic Stem Cell Delivery System to Promote Ischemic Limb Regeneration. Acta Biomater (2016) 31:99–113. doi: 10.1016/j.actbio.2015.12.021
66. Tancharoen W, Aungsuchawan S, Pothacharoen P, Markmee R, Narakornsak S, Kieodee J, et al. Differentiation of Mesenchymal Stem Cells From Human Amniotic Fluid to Vascular Endothelial Cells. Acta Histochem (2017) 119(2):113–21. doi: 10.1016/j.acthis.2016.11.009
67. Kalamohan K, Periasamy J, Bhaskar Rao D, Barnabas GD, Ponnaiyan S, Ganesan K. Transcriptional Coexpression Network Reveals the Involvement of Varying Stem Cell Features With Different Dysregulations in Different Gastric Cancer Subtypes. Mol Oncol (2014) 8(7):1306–25. doi: 10.1016/j.molonc.2014.04.005
68. Gu J, Qian H, Shen L, Zhang X, Zhu W, Huang L, et al. Gastric Cancer Exosomes Trigger Differentiation of Umbilical Cord Derived Mesenchymal Stem Cells to Carcinoma-Associated Fibroblasts Through TGF-β/Smad Pathway. PloS One (2012) 7(12):e52465. doi: 10.1371/journal.pone.0052465
69. Zhang H, Deng T, Liu R, Ning T, Yang H, Liu D, et al. CAF Secreted miR-522 Suppresses Ferroptosis and Promotes Acquired Chemo-Resistance in Gastric Cancer. Mol Cancer (2020) 19(1):43. doi: 10.1186/s12943-020-01168-8
70. Chong Y, Tang D, Xiong Q, Jiang X, Xu C, Huang Y, et al. Galectin-1 From Cancer-Associated Fibroblasts Induces Epithelial-Mesenchymal Transition Through β1 Integrin-Mediated Upregulation of Gli1 in Gastric Cancer. J Exp Clin Cancer Res: CR (2016) 35(1):175. doi: 10.1186/s13046-016-0449-1
71. Kurashige J, Mima K, Sawada G, Takahashi Y, Eguchi H, Sugimachi K, et al. Epigenetic Modulation and Repression of miR-200b by Cancer-Associated Fibroblasts Contribute to Cancer Invasion and Peritoneal Dissemination in Gastric Cancer. Carcinogenesis (2015) 36(1):133–41. doi: 10.1093/carcin/bgu232
72. Liu CJ, Wang YK, Kuo FC, Hsu WH, Yu FJ, Hsieh S, et al. Helicobacter Pylori Infection-Induced Hepatoma-Derived Growth Factor Regulates the Differentiation of Human Mesenchymal Stem Cells to Myofibroblast-Like Cells. Cancers (2018) 10(12):479. doi: 10.3390/cancers10120479
73. Kaukonen R, Mai A, Georgiadou M, Saari M, De Franceschi N, Betz T, et al. Normal Stroma Suppresses Cancer Cell Proliferation via mechanosensitive regulation of JMJD1a-mediated transcription. Nat Commun (2016) 7:12237. doi: 10.1038/ncomms12237
74. Jang M, Koh I, Lee JE, Lim JY, Cheong JH, Kim P. Increased Extracellular Matrix Density Disrupts E-Cadherin/β-Catenin Complex in Gastric Cancer Cells. Biomater Sci (2018) 6(10):2704–13. doi: 10.1039/c8bm00843d
75. Song L, Zhou X, Jia HJ, Du M, Zhang JL, Li L. Effect of hGC-MSCs From Human Gastric Cancer Tissue on Cell Proliferation, Invasion and Epithelial-Mesenchymal Transition in Tumor Tissue of Gastric Cancer Tumor-Bearing Mice. Asian Pacific J Trop Med (2016) 9(8):796–800. doi: 10.1016/j.apjtm.2016.06.004
76. Cai Y, Xi Y, Cao Z, Xiang G, Ni Q, Zhang R, et al. Dual Targeting and Enhanced Cytotoxicity to HER2-overexpressing Tumors by Immunoapoptotin-Armored Mesenchymal Stem Cells. Cancer Lett (2016) 381(1):104–12. doi: 10.1016/j.canlet.2016.07.027
77. Zhu Y, Cheng M, Yang Z, Zeng CY, Chen J, Xie Y, et al. Mesenchymal Stem Cell-Based NK4 Gene Therapy in Nude Mice Bearing Gastric Cancer Xenografts. Drug Des Dev Ther (2014) 8:2449–62. doi: 10.2147/dddt.S71466
78. Zhu X, Su D, Xuan S, Ma G, Dai Z, Liu T, et al. Gene Therapy of Gastric Cancer Using LIGHT-secreting Human Umbilical Cord Blood-Derived Mesenchymal Stem Cells. Gastric Cancer (2013) 16(2):155–66. doi: 10.1007/s10120-012-0166-1
79. Zhou YL, Li YM, He WT. Oxygen-Laden Mesenchymal Stem Cells Enhance the Effect of Gastric Cancer Chemotherapy. Vitro Oncol Lett (2019) 17(1):1245–52. doi: 10.3892/ol.2018.9670
80. Quante M, Tu SP, Tomita H, Gonda T, Wang SS, Takashi S, et al. Bone Marrow-Derived Myofibroblasts Contribute to the Mesenchymal Stem Cell Niche and Promote Tumor Growth. Cancer Cell (2011) 19(2):257–72. doi: 10.1016/j.ccr.2011.01.020
81. Yin L, Zhang R, Hu Y, Li W, Wang M, Liang Z, et al. Gastric-Cancer-Derived Mesenchymal Stem Cells: A Promising Target for Resveratrol in the Suppression of Gastric Cancer Metastasis. Hum Cell (2020) 33(3):652–62. doi: 10.1007/s13577-020-00339-5
82. Liu CJ, Kuo FC, Wang CL, Kuo CH, Wang SS, Chen CY, et al. Suppression of IL-8-Src Signalling Axis by 17β-Estradiol Inhibits Human Mesenchymal Stem Cells-Mediated Gastric Cancer Invasion. J Cell Mol Med (2016) 20(5):962–72. doi: 10.1111/jcmm.12786
83. Kuo CH, Liu CJ, Lu CY, Hu HM, Kuo FC, Liou YS, et al. 17β-Estradiol Inhibits Mesenchymal Stem Cells-Induced Human AGS Gastric Cancer Cell Mobility via suppression of CCL5- Src/Cas/Paxillin signaling pathway. Int J Med Sci (2014) 11(1):7–16. doi: 10.7150/ijms.6851
84. Zhang S, Liu Y, Derakhshanfar S, He W, Huang Q, Dong S, et al. Polymer Self-Assembled Bmscs With Cancer Tropism and Programmed Homing. Adv Healthcare Mater (2018) 7(23):e1800118. doi: 10.1002/adhm.201800118
85. Niess H, von Einem JC, Thomas MN, Michl M, Angele MK, Huss R, et al. Treatment of Advanced Gastrointestinal Tumors With Genetically Modified Autologous Mesenchymal Stromal Cells (TREAT-ME1): Study Protocol of a Phase I/II Clinical Trial. BMC Cancer (2015) 15:237. doi: 10.1186/s12885-015-1241-x
86. von Einem JC, Guenther C, Volk HD, Grütz G, Hirsch D, Salat C, et al. Treatment of Advanced Gastrointestinal Cancer With Genetically Modified Autologous Mesenchymal Stem Cells: Results From the Phase 1/2 TREAT-ME-1 Trial. Int J Cancer (2019) 145(6):1538–46. doi: 10.1002/ijc.32230
87. Fakhari S, Kalantar E, Nikzaban M, Hakhamneshi MS, Fathi F, Nikkhoo B, et al. Effect of Helicobacter Pylori Infection on Stromal-Derived factor-1/CXCR4 Axis in Bone Marrow-Derived Mesenchymal Stem Cells. Adv Biomed Res (2014) 3:19. doi: 10.4103/2277-9175.124650
88. Donnelly JM, Engevik AC, Engevik M, Schumacher MA, Xiao C, Yang L, et al. Gastritis Promotes an Activated Bone Marrow-Derived Mesenchymal Stem Cell With a Phenotype Reminiscent of a Cancer-Promoting Cell. Dig Dis Sci (2014) 59(3):569–82. doi: 10.1007/s10620-013-2927-z
89. Donnelly JM, Chawla A, Houghton J, Zavros Y. Sonic Hedgehog Mediates the Proliferation and Recruitment of Transformed Mesenchymal Stem Cells to the Stomach. PloS One (2013) 8(9):e75225. doi: 10.1371/journal.pone.0075225
90. Choi YJ, Kim N, Chang H, Lee HS, Park SM, Park JH, et al. Helicobacter Pylori-Induced Epithelial-Mesenchymal Transition, a Potential Role of Gastric Cancer Initiation and an Emergence of Stem Cells. Carcinogenesis (2015) 36(5):553–63. doi: 10.1093/carcin/bgv022
91. Liu CJ, Kuo FC, Hu HM, Chen CY, Huang YB, Cheng KH, et al. 17β-Estradiol Inhibition of IL-6-Src and Cas and Paxillin Pathway Suppresses Human Mesenchymal Stem Cells-Mediated Gastric Cancer Cell Motility. Trans Res: J Lab Clin Med (2014) 164(3):232–43. doi: 10.1016/j.trsl.2014.04.009
92. Wu Z, Liu W, Wang Z, Zeng B, Peng G, Niu H, et al. Mesenchymal Stem Cells Derived From iPSCs Expressing interleukin-24 Inhibit the Growth of Melanoma in the Tumor-Bearing Mouse Model. Cancer Cell Int (2020) 20:33. doi: 10.1186/s12935-020-1112-7
93. Lu L, Chen G, Yang J, Ma Z, Yang Y, Hu Y, et al. Bone Marrow Mesenchymal Stem Cells Suppress Growth and Promote the Apoptosis of Glioma U251 Cells Through Downregulation of the PI3K/AKT Signaling Pathway. Biomed Pharmacother = Biomed Pharmacother (2019) 112:108625. doi: 10.1016/j.biopha.2019.108625
94. Serhal R, Saliba N, Hilal G, Moussa M, Hassan GS, El Atat O, et al. Effect of Adipose-Derived Mesenchymal Stem Cells on Hepatocellular Carcinoma: In Vitro Inhibition of Carcinogenesis. World J Gastroenterol (2019) 25(5):567–83. doi: 10.3748/wjg.v25.i5.567
95. Zhao J, Zhang Z, Cui Q, Zhao L, Hu Y, Zhao S. Human Adipose-Derived Mesenchymal Stem Cells Inhibit Proliferation and Induce Apoptosis of Human Gastric Cancer HGC-27 Cells. 3 Biotech (2020) 10(3):129. doi: 10.1007/s13205-020-2090-0
96. Meng MY, Li L, Wang WJ, Liu FF, Song J, Yang SL, et al. Assessment of Tumor Promoting Effects of Amniotic and Umbilical Cord Mesenchymal Stem Cells in vitro and in vivo. J Cancer Res Clin Oncol (2019) 145(5):1133–46. doi: 10.1007/s00432-019-02859-6
97. Rahmatizadeh F, Gholizadeh-Ghaleh Aziz S, Khodadadi K, Lale Ataei M, Ebrahimie E, Soleimani Rad J, et al. Bidirectional and Opposite Effects of Naïve Mesenchymal Stem Cells on Tumor Growth and Progression. Adv Pharm Bull (2019) 9(4):539–58. doi: 10.15171/apb.2019.063
Keywords: gastric cancer, mesenchymal stem cells, tumor tropism, reprogramming, tumor stroma, drug delivery, targeted therapy
Citation: Li Y, Zhong X, Zhang Y and Lu X (2021) Mesenchymal Stem Cells in Gastric Cancer: Vicious but Hopeful. Front. Oncol. 11:617677. doi: 10.3389/fonc.2021.617677
Received: 15 October 2020; Accepted: 22 April 2021;
Published: 11 May 2021.
Edited by:
Rui Liao, First Affiliated Hospital of Chongqing Medical University, ChinaCopyright © 2021 Li, Zhong, Zhang and Lu. This is an open-access article distributed under the terms of the Creative Commons Attribution License (CC BY). The use, distribution or reproduction in other forums is permitted, provided the original author(s) and the copyright owner(s) are credited and that the original publication in this journal is cited, in accordance with accepted academic practice. No use, distribution or reproduction is permitted which does not comply with these terms.
*Correspondence: Xinliang Lu, bHV4QHpqdS5lZHUuY24=