- 1Department of Radiation Oncology, Dongguk University College of Medicine, Gyeongju, South Korea
- 2Department of Radiation Oncology, University of Florida College of Medicine, Gainesville, FL, United States
- 3University of Florida Health Proton Therapy Institute, Jacksonville, FL, United States
- 4Department of Radiation Oncology, Orlando Health Cancer Institute, Orlando, FL, United States
- 5Department of Radiation Oncology, Ajou University School of Medicine, Suwon, South Korea
Background: Although there are some controversies regarding whole pelvic radiation therapy (WPRT) due to its gastrointestinal and hematologic toxicities, it is considered for patients with gynecological, rectal, and prostate cancer. To effectively spare organs-at-risk (OAR) doses using multi-leaf collimator (MLC)’s optimal segments, potential dosimetric benefits in volumetric modulated arc therapy (VMAT) using a half-beam technique (HF) were investigated for WPRT.
Methods: While the size of a fully opened field (FF) was decided to entirely include a planning target volume in all beam’s eye view across arc angles, the HF was designed to use half the FF from the isocenter for dose optimization. The left or the right half of the FF was alternatively opened in VMAT-HF using a pair of arcs rotating clockwise and counterclockwise. Dosimetric benefits of VMAT-HF, presented with dose conformity, homogeneity, and dose–volume parameters in terms of modulation complex score, were compared to VMAT optimized using the FF (VMAT-FF). Consequent normal tissue complication probability (NTCP) by reducing the irradiated volumes was evaluated as well as dose–volume parameters with statistical analysis for OAR. Moreover, beam-on time and MLC position precision were analyzed with log files to assess plan deliverability and clinical applicability of VMAT-HF as compared to VMAT-FF.
Results: While VMAT-HF used 60%–70% less intensity modulation complexity than VMAT-FF, it showed superior dose conformity. The small intestine and colon in VMAT-HF showed a noticeable reduction in the irradiated volumes of up to 35% and 15%, respectively, at an intermediate dose of 20–45 Gy. The small intestine showed statistically significant dose sparing at the volumes that received a dose from 15 to 45 Gy. Such a dose reduction for the small intestine and colon in VMAT-HF presented a significant NTCP reduction from that in VMAT-FF. Without sacrificing the beam delivery efficiency, VMAT-HF achieved effective OAR dose reduction in dose–volume histograms.
Conclusions: VMAT-HF led to deliver conformal doses with effective gastrointestinal-OAR dose sparing despite using less modulation complexity. The dose of VMAT-HF was delivered with the same beam-on time with VMAT-FF but precise MLC leaf motions. The VMAT-HF potentially can play a valuable role in reducing OAR toxicities associated with WPRT.
Introduction
Elective whole pelvic radiation therapy (WPRT) irradiating pelvic lymph nodes is regarded as a standard treatment regimen for intermediate- or high-risk rectal, anal, and gynecological cancers (1–3). Clinical outcome studies reported mild acute and late gastrointestinal (GI), genitourinary (GU), and hematological toxicity profiles in anal cancer patients in intensity-modulated radiation therapy (IMRT), employing simultaneously integrated boost technique and image guidance in IMRT for WPRT (3, 4). In addition, IMRT optimized to spare dose to pelvic bone marrow showed dosimetric benefits to reduce GI complications and hematological toxicities in anal and cervical cancer patients in WPRT (5, 6). Although the benefits of WPRT in patients with locally advanced prostate cancer have been controversial (7, 8), its role and gain have been reexamined considering the interaction between radiation therapy (RT) and adjuvant androgen deprivation therapy based on the duration and timing of hormone therapy and field size effect of RT (9). As per a new protocol, recent results also reported treatment gains in short-term androgen deprivation therapy plus pelvic lymph node irradiation in IMRT for prostate cancer (10–12).
However, late and acute GI toxicities have been significant concerns due to extensive fields (13–15), although WPRT can have clinical merits combined with other treatment schemes and a clever dose optimization technique for various treatment sites (16–20). When a large field size has to be used to cover target volumes plus regional lymph nodes in WPRT, organs-at-risk (OAR) exposure is unavoidable as long as the lower abdomen has to be included in the treatment fields. However, if OAR dose can be effectively spared using dose optimization and precise dose delivery using a multi-leaf collimator (MLC), the extended role of RT can be expected for a better outcome in cancer treatment. OAR dose sparing can reduce acute and late toxicities of the GI tract. Therefore, it becomes essential to provide successful treatment strategies in RT. To provide conformal dose distribution to large and complicated anatomical geometries such as WPRT (21–23), volumetric modulated arc therapy (VMAT) is an efficient dose delivery method.
Optimal segments to deliver conformal dose distributions are vital to implementing VMAT with high dose agreements successfully. Because MLC leaves move in one direction as the collimator angle is fixed, if the OAR are located between the separated target volumes along the same direction with the MLC movement, suboptimal MLC segments can be created. When each discretized gantry angle has one MLC segment, the limit of a maximum MLC traveling distance and speed between control points can restrict full utilization of the optimization engine’s capability to provide optimal MLC sequence (24–26). For example, while one side of the MLC conforms to half of the target contour, the other side cannot properly shield OAR or opens more than necessary outside of the planning target volume (PTV) to meet the prescribed dose. Thus, when the VMAT plan is optimized to cover a large and complex-shaped target, such as in WPRT, suboptimal MLC segments can be created and affect dose conformity.
In this study, VMAT optimized using a half-beam technique (VMAT-HF) was devised to provide superior OAR dose sparing, especially for the GI tract, and achieve dose conformity for the PTV in WPRT. The potential dosimetric benefits of the VMAT-HF were evaluated with dose homogeneity and conformity, dose–volume parameters, and normal tissue complication probability (NTCP) with statistical analysis. Compared to the dose distribution in VMAT optimized using a fully opened field (FF) to sufficiently cover the PTV, clinical usefulness of the VMAT-HF was suggested for dosimetric benefits and beam delivery with precise MLC leaf position accuracy.
Materials and Methods
Patient Selection
A total of 15 eligible patients diagnosed with anal, vaginal, and cervical cancer were included in this study on WPRT as per the National Comprehensive Cancer Network guidelines (27, 28). The patient and tumor characteristics are presented in Table 1 with the treatment regimen. In patients with cervical and vaginal cancer, WPRT was followed by high-dose rate brachytherapy. All patients underwent computed tomography simulation in the supine position with arms on the chest. This retrospective dosimetric study was approved by the institutional review board of the Dongguk University Medical Center (110757-201711-HR-02-01), and written informed consent was obtained from all patients. All information was anonymized prior to analysis.
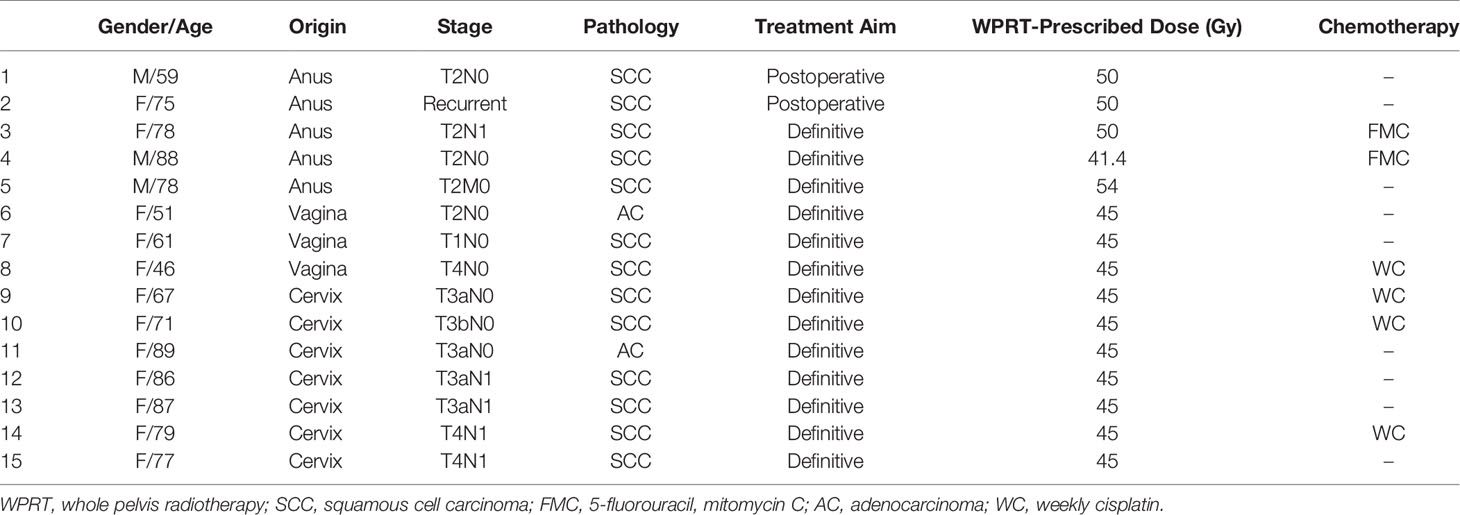
Table 1 Characteristics of patients, tumors, and treatment regimen selected for whole pelvic radiation therapy.
Target Delineation and Volumetric Modulated Arc Therapy Optimization Using Different Field Sizes
Clinical target volume and pelvic lymph nodes were delineated according to the consensus guidelines (29–31). The PTV was created by adding a 5-mm margin to the clinical target volume. Obturator, presacral, and internal iliac node chains are included in the pelvic lymph nodes in all cases. Treatment volume for patients with gynecological cancer includes the tumor involving the lower third of the vagina and tumor bed, parametrium, uterosacral ligaments, and pelvic lymph nodes. The small bowel, bladder, rectum, and femur heads were contoured as OAR. The anorectum in patients with anal cancer was contoured superiorly from the rectosigmoid flexure to the inferior level, 3 cm above the anal verge. Rectum was defined as rectumwPTV or rectumwoPTV depending on whether it overlaps with PTV to avoid optimization conflicts between target dose coverage and rectal dose sparing. Each PTV received a prescribed dose of 50 or 46 Gy in 25 or 23 fractions as in Table 1 except for one case. External iliac and perirectal nodes are included in patients with gynecological and anal cancer, respectively. For effective plan optimization to achieve conformal dose distributions, three pseudo structures are used, which are created by subtracting expanded PTVs with a 4-mm, an 8-mm, and a 14-mm margin from the body limited in the calculation volume.
All VMAT plans consisted of four full arcs alternating clockwise and counterclockwise in Eclipse (version 10.0, Varian Medical Systems, Palo Alto, CA). The collimator angles < ± 40° were used for each arc to improve dose conformity and minimize the interleaf leakage and tongue-and-groove effect in VMAT (32). Meanwhile, dose distributions of the VMAT plans were optimized using different field sizes, as shown in Figure 1. An optimized VMAT-FF is created with an FF sufficient to cover an entire PTV but <15 cm with X-jaws considering the maximum leaf span of the Millennium MLC (Varian Medical Systems). The opening of the FF was adjusted in a superior–inferior direction to sufficiently include the PTV plus a margin <1 cm, as shown in Figure 1A. Appropriateness of the field size was reviewed at all beam’s eye views at the different angles composed of VMAT arcs. The VMAT-HF is optimized with half the size of the FF. The optimized dose was delivered by opening the half and the other half of the FF for two arcs rotating clockwise and counterclockwise, respectively, as shown in Figure 1B.
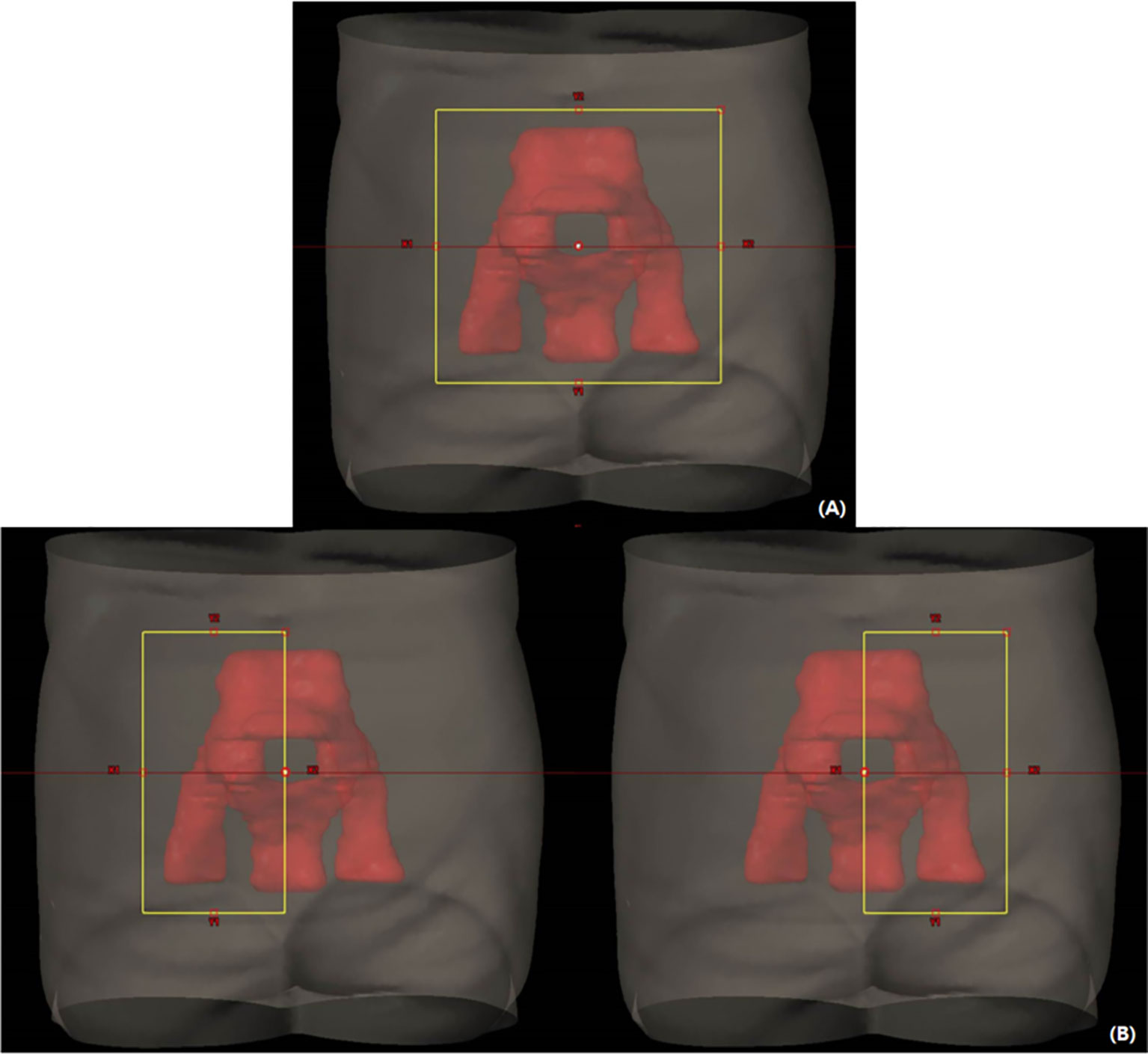
Figure 1 Volumetric modulated arc therapy (VMAT) plans optimized with different field sizes for whole pelvic radiation therapy. (A) A fully opened field size (FF) to sufficiently cover a planning target volume in a beam’s eye view across arc angles. (B) Half of the FF size for each arc rotating clockwise and counterclockwise.
The dose constraints were applied to meet dose criteria for target and OAR as per the radiation therapy oncology group protocols for each treatment site (1, 2). More specifically, the optimization objectives and their priorities for the structures were applied as shown in Table 2 with automatic normal tissue optimization with a priority of 350. The dose distributions for each treatment site were calculated in Eclipse using Analytical Anisotropic Algorithm (version 10.0, Varian Medical Systems) and progressive resolution optimizer (version 10.0, Varian Medical Systems). The dose was calculated with a grid size of 2.5 mm. Dosimetric benefits of VMAT-FF and VMAT-HF were compared when the prescribed dose covered 95% of the PTV with the same dose constraints applied for OAR.
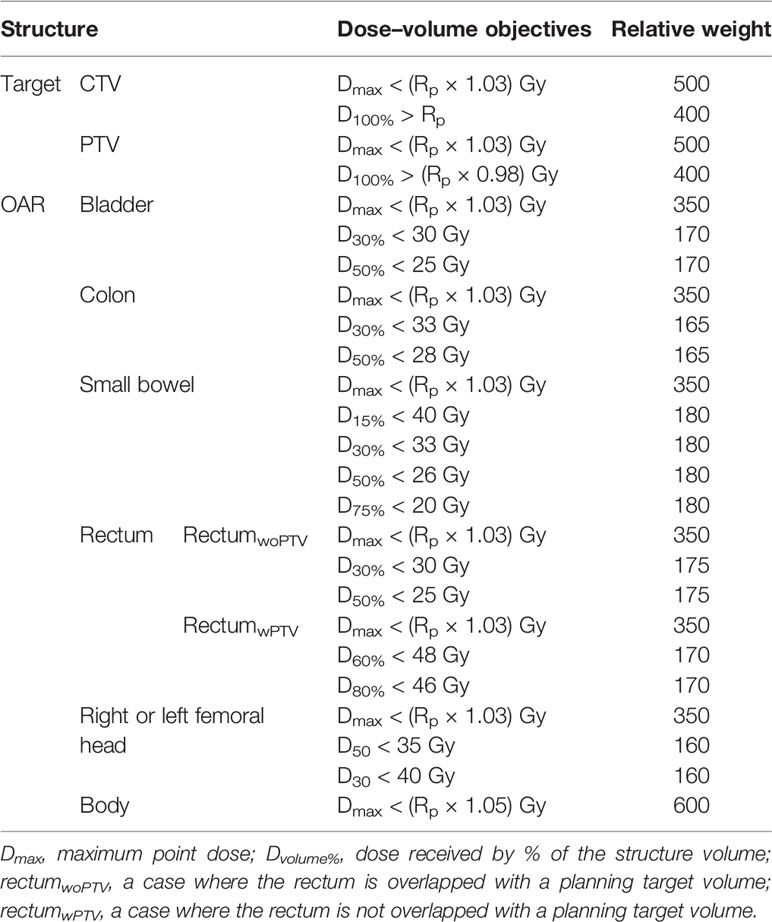
Table 2 Plan optimization objectives for target and organs at risk (OAR) using dose–volume parameters and relative weights, when 95% of the planning target volume is covered by the prescribed dose (Rp) in volumetric modulated arc therapy (VMAT) plans.
Beam Modulation Complexity
The traveling distances and segment shapes between MLC control points can affect the complexity of the VMAT intensity modulation. The modulation complexity score (MCS), using variabilities of leaf sequences (LSV) and segment area (AAV), was adopted to comprehensively present the plan complexity across all segments (33). It is formulated using equation (1) by reflecting each segment weight to the corresponding relative arc weight.
The parameters of n and N indicate the total number of control points per arc and the total number of arcs used in each VMAT plan. The LSVcp and AAVcp for each control point are calculated using equations (2) and (3), respectively, where m is the number of MLC leaves that move underneath the unblocking portion of the field defined by X and Y jaws for each control point:
The presents the i-th leaf position of the MLC at the left bank. The and indicate the farthest position of the MLC leaf from the isocenter among all MLC leaves constituting a shape of the individual segment and across all control points of an individual arc. R denotes the MLC leaves on the right bank. The LSV presents variability of the MLC leaf traveling distances sweeping each set of control points relative to the maximum lateral separation from the isocenter for each side. The AAV presents the complexity of separation of each pair of MLC leaves relative to the maximum separation created among all MLC leaves across all segments consisting of the arc.
Dose Evaluation for Target and Organs at Risk With Statistical Analysis
To compare dose distributions in two VMAT plans depending on MLC segments and sequences, dose conformity (CN) for PTV was calculated using equation (4). The TV is the target volume. The TVRI and VRI mean the target volume and the volume covered by the reference prescribed isodose, respectively (34). The ideal value of CN is 1. As it is closer to 1, the dose distribution is more conformal to the target. In addition, two different formulas were used to calculate dose homogeneity for the PTV. One is the homogeneity index (HI) proposed by ICRU-83 (35). The other is the s-index representing the standard deviation (DSD) of doses predicted to the PTV (36). The HI was calculated using doses for the 2% (D2%), 98% (D98%), and 50% (D50%) of the PTV, as shown in equation (5). The standard deviations of the dose element (Di) for each voxel volume (vi) of the PTV were calculated to the prescribed dose (DRp) for the TV (36) using equation (6). The ideal value of HI and s-index is 0. The closer the value is to 0, the better the dose distribution is homogeneous to the prescribed dose.
Dose sparing for OAR was evaluated with dose–volume histograms (DVHs) and dose–volume parameters associated with acute and late toxicities. Since the dose–volume predictors on acute or late GI toxicities can be different depending on patient surgery, concurrent therapy, prescribed dose, and treatment techniques of RT (18, 37–44), each volume receiving the doses from 5 to 45 Gy was evaluated for small bowel and colon, with a dose interval of 5 Gy. Maximum (Dmax) and mean (Dmean) dose and the dose (D2cc) delivered to the 2 cc of organ volume were also analyzed. Radiobiological effects were estimated by calculating the equivalent uniform dose (EUD) and NTCP using Emami–Burman parameters (45). To present the sensitivities and variabilities of NTCP in terms of analytic models, Lyman–Kutcher–Burman and EUD-based log-logistic models were also adopted (46, 47). The alpha–beta ratios for acute and late toxicities and required biological parameters to calculate NTCP are shown in Table 3 (43, 46–48). Because volume variations at doses can be different for rectumwPTV and rectumwoPTV, DVH was separately presented in anal cancer cases. Both rectumwPTV and rectumwoPTV were combined to evaluate dose–volume parameters and NTCP. Furthermore, to distinguish the statistically significant OAR dose sparing and the consequent effect of VMAT-HF from VMAT-FF, the Wilcoxon signed-rank test was performed using statistical analysis software (SPSS version 20, SPSS Inc., Chicago, IL). A p-value <0.05 was considered statistically significant.
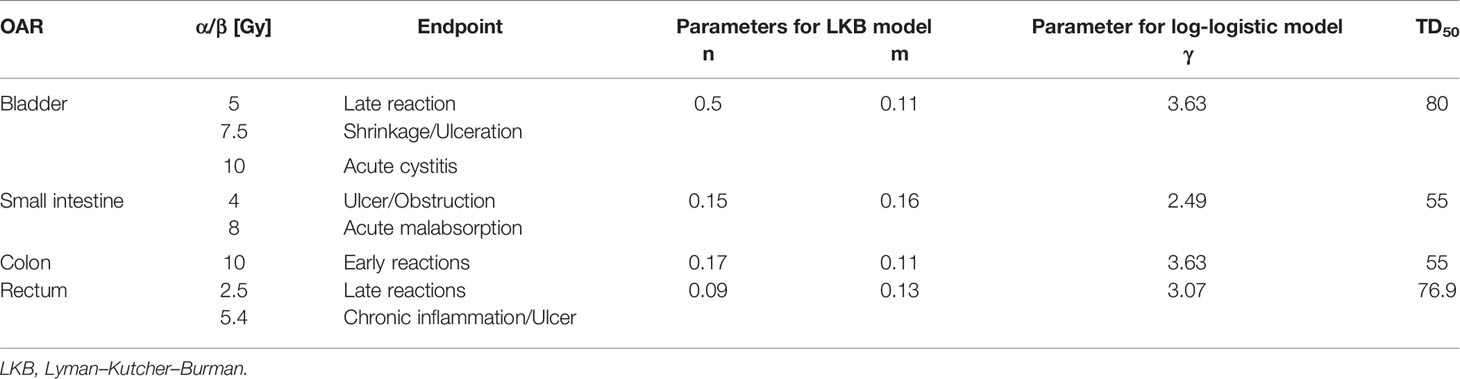
Table 3 Radiobiological parameters to calculate equivalent uniform dose and normal tissue complication probabilities for the bladder and gastrointestinal tract at different endpoints and alpha–beta ratios.
Plan Deliverability
The VMAT plan’s deliverability was evaluated by generating Dyanlog files through dry runs for each patient case (49). As the sensitivity and accuracy of the MLC position errors are significant in dose agreement in IMRT (50), MLC position errors of the VMAT-FF and VMAT-HF were investigated with the log information recorded every 50 ms. The beam-on times required to deliver different monitor units (MUs) were compared between VMAT-FF and VMAT-HF. In addition, the positions of each MLC leaf, which moves inside the field defined by the jaw, were compared with the corresponding planned MLC positions to analyze errors at individual control points. Data in the log files were analyzed in a customized code written by Matlab (version 9.0.0.96032; The MathWorks, Inc., Natick, MA).
Results
As dose distributions of each VMAT plan are compared, VMAT-HF showed that a 70%–75% isodose line compactly surrounds the target shapes. As Figure 2 shows superior high dose gradients in a representative cervix and an anal cancer case, VMAT-HF resulted in sculpted dose curvatures along the posterior bladder wall and anterior rectal wall. It shows conformal dose distribution for the target in the axial and the sagittal view (the first and the third rows in Figure 2). Such dose sparing for the bladder is also distinguishable in the coronal view (the second row in Figure 2). The VMAT-HF demonstrates the isodose distribution of less than 70% between the left and right iliac lymph nodes. The OAR dose sparing in VMAT-HF was manifested in the DVH comparisons, as shown in Figure 3. As the DVHs for the PTV were identical in two VMAT plans, the small intestine and colon showed noticeable dose reduction at the intermediate dose range from 25 to 45 Gy, as in Figure 3A. The volume reduction was up to 35% at 25–30 Gy and 15% at 35–40 Gy for the small intestine and colon. The volume reduction at the dose ranges from 30 to 50 Gy was also observed in rectumwoPTV. The VMAT-HF achieved the sharper dose fall-off for the PTV and rectumwPTV without an excessive hot spot, as shown in Figure 3B. The bladder DVH showed a noticeable dose–volume difference from 20 to 50 Gy in VMAT-HF.
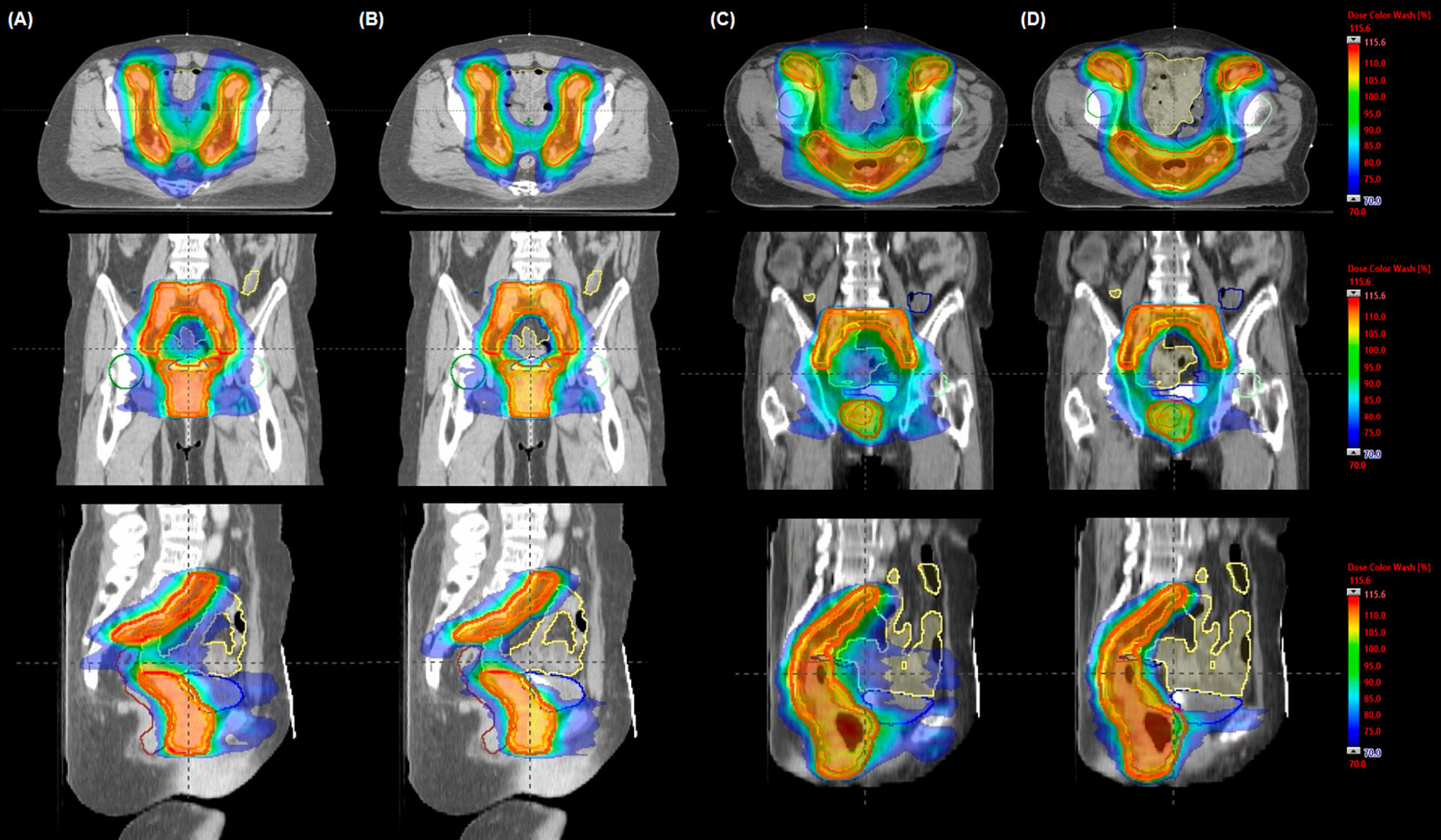
Figure 2 Comparison of representative dose distributions in volumetric modulated arc therapy (VMAT) plans for a cervix (A, B) and an anal (C, D) cancer case. The dose was optimized using (A, C) a fully opened field size (VMAT-FF) to cover the planning target volume and (B, D) a half-beam technique (VMAT-HF). Isodose and structures displayed as a color-wash overlay and delineated contours, respectively: red for planning target volume, yellow for small bowel, navy for colon, brown for anorectum, blue for bladder, green for right femur head, and cyan for left femur head.
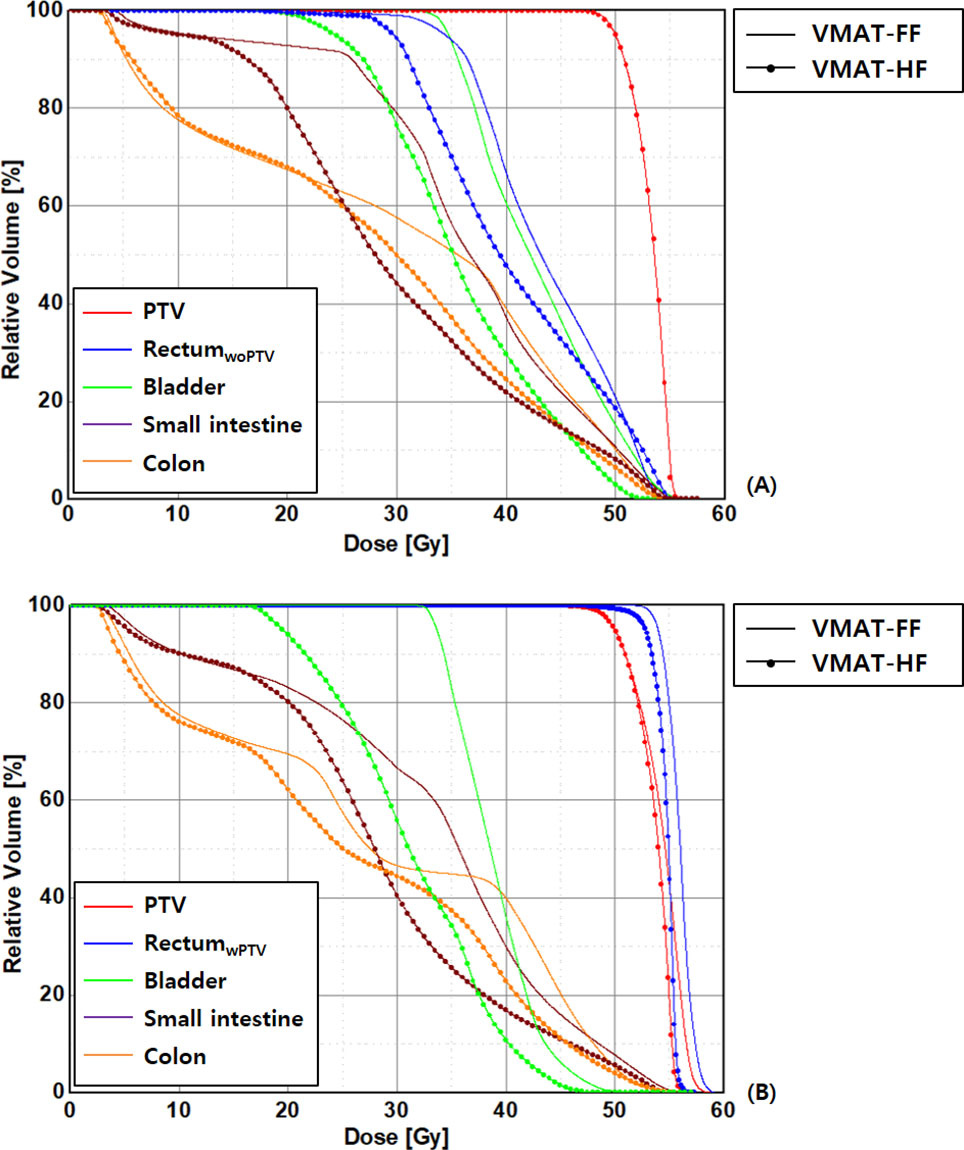
Figure 3 Comparison of dose–volume histograms in patients with anal cancer in the volumetric modulated arc therapy (VMAT) plans optimized using a fully opened field size (FF) and a half-beam technique (HF), as rectal volume is (A) overlapped (rectumwPTV) and (B) not overlapped (rectumwoPTV) with planning target volumes.
The differences of OAR dose sparing were more presented explicitly in Table 4 with major dose–volume parameters, which showed statistical significance. The reduction of intermediate or high dose to OAR in VMAT-HF led to reducing Dmean. The VMAT-HF showed volume reduction at the dose range from 20 to 45 Gy for small bowel, colon, and bladder. The small bowel and bladder showed significant volume reduction at 15 Gy as well. The rectum also showed dose–volume sparing at the dose range from 30 to 45 Gy. Furthermore, the VMAT-HF resulted in significantly lower EUD and NTCP in LKB and logistic models for the small bowel and colon, as in Table 5.
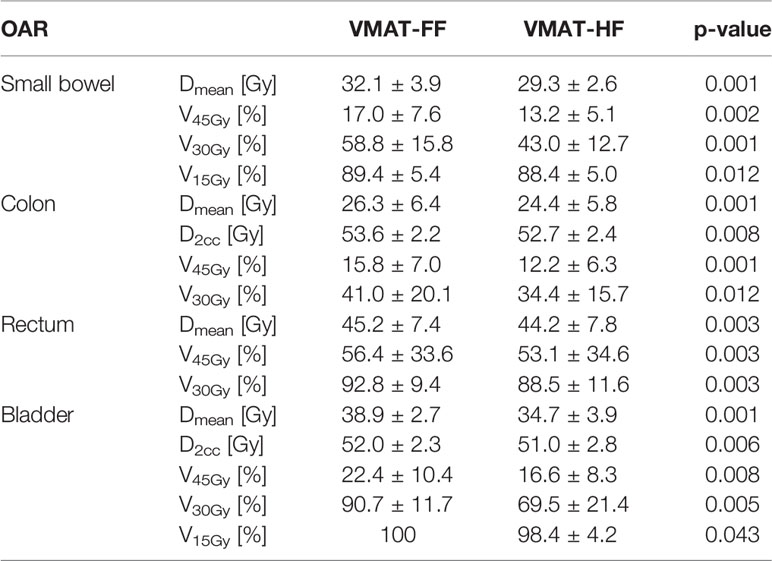
Table 4 Comparison of dose–volume parameters for the small bowel, colon, rectum, and bladder in the volumetric modulated arc therapy (VMAT) plans optimized using a fully opened field (FF) and a half-beam technique (HF) with statistical analysis using Wilcoxon signed-rank test.
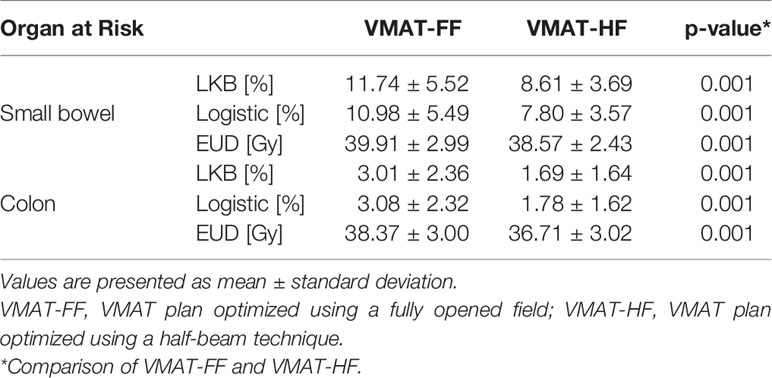
Table 5 Comparison of normal tissue complication probabilities (NTCP) using Lyman–Kutcher–Burman (LKB) and logistic models and equivalent uniform dose (EUD) with statistical analysis using Wilcoxon signed-rank test in volumetric modulated arc therapy (VMAT) plans.
When the dosimetric benefits were analyzed in terms of MCS, the VMAT-HF showed that it used 17% less modulation complexity as in Figure 4A but achieved superior dose conformity (0.89 vs. 0.85 in average) as in Figure 4B. The VMAT-HF as compared to VMAT-FF showed a significant difference in AAV (0.29 ± 0.04 vs. 0.35 ± 0.04, p-value: 0.001) and LSV (0.71 ± 0.02 vs. 0.79 ± 0.01, p-value: 0.001). Both plans showed comparable dose homogeneity with HI and s-index in Figures 4C, D. In addition, as shown in Figure 4E, while VMAT-HF used two times higher MU than VMAT-FF, beam-on time was identical for both plans because VMAT can adjust dose-rate in beam delivery. MLC leaf position average errors were also comparable between VMAT-HF and VMAT-FF (0.36 ± 0.40 mm vs. 0.39 ± 0.36 mm, p-value: 0.013).
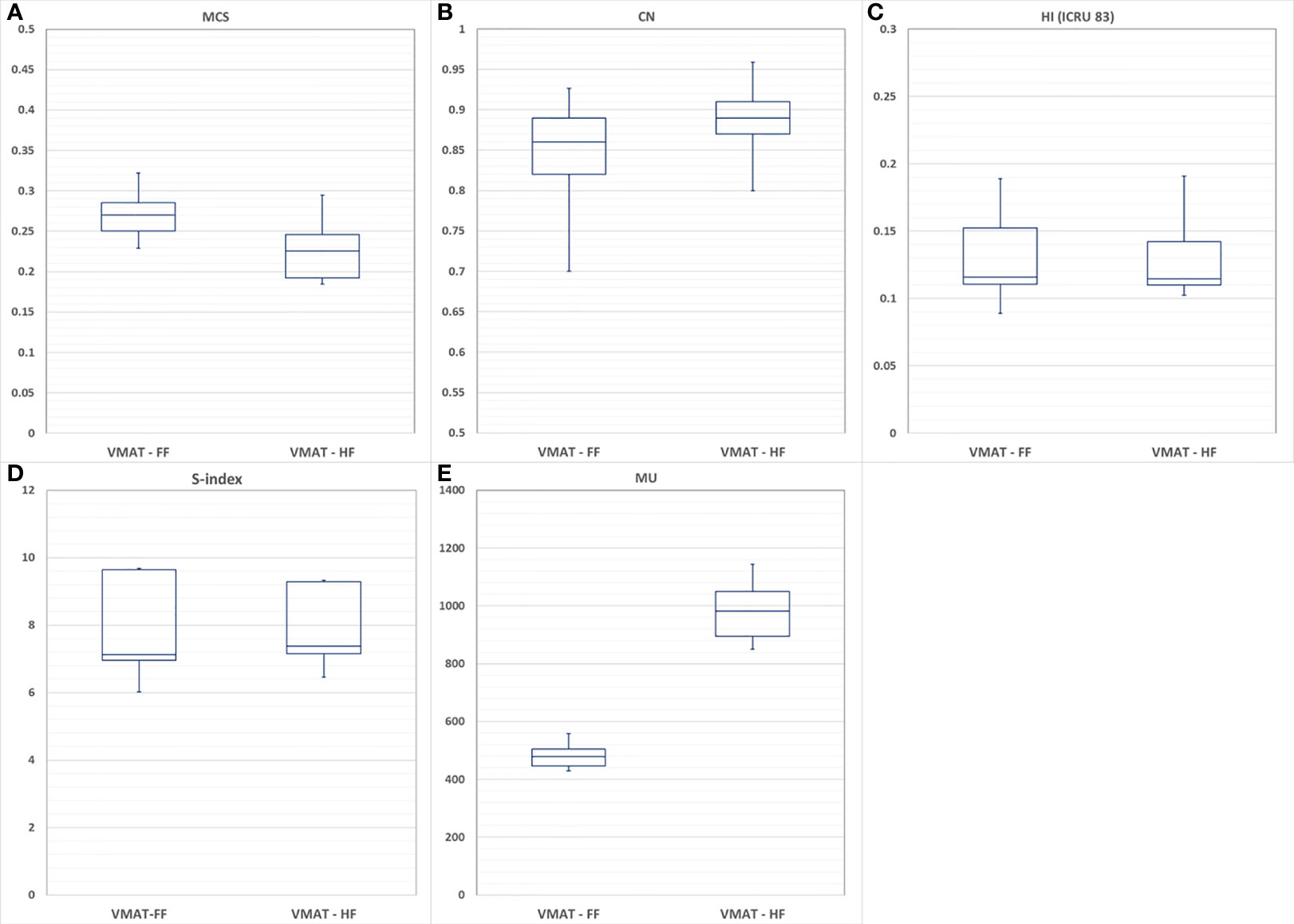
Figure 4 Comparison of volumetric modulated arc therapy (VMAT) optimized with using a fully opened field (VMAT-FF) and a half-beam technique (VMAT-HF) with (A) modulation complexity score (MCS), (B) conformity number (CN), (C) homogeneity index (HI), (D) s-index, and (E) monitor units (MU).
Discussion
The HF has been used in the field matching for head and neck cancer and breast cancer when supraclavicular lymph nodes are included in the treatment volume (51, 52). A mono-isocentric technique using the HF facilitated a more reliable patient setup and simple beam matching using a non-divergent beam edge. In addition, the HF at the matching line could bring out dose reduction of the lung in breast treatment. Maintaining such dosimetric benefit and more effectively reducing doses to OAR, studies were expanded to employ intensity modulation using optimal segments from HF (26, 53, 54). Consequently, VMAT-HF showed reducing volume receiving a dose of less than 10 Gy for left-sided breast cancer, which is more challenging than the right-sided case due to heart dose sparing. Furthermore, the VMAT using a fixed-jaw (opening of 15 cm in the X direction), considering the limitation of maximum leaf span of the MLC, also showed parotid dose sparing (26).
However, the HF has not been used for WPRT because the whole pelvic region is not usually regarded as a site that needs beam matching or can be covered with the half size of the FF for a large PTV. If we can make the most use of the capability of an optimization engine to generate optimal fluence and leaf sequencing, dose optimization using the HF and multiple arcs can effectively meet plan objectives in VMAT. In light of using optimal MLC segments, the VMAT-HF could demonstrate dosimetric benefits for WPRT with superior dose conformity and effective OAR sparing while using less beam modulation complexity.
To cover the large PTV with the prescribed dose, especially when the fixed field size is required before starting dose optimization in treatment planning, opening a sufficient field size covering the PTV plus a margin is typically considered. However, when the FF is used to treat complex-shaped PTV, particularly having some separated subvolumes as shown in Figure 5A, suboptimal segments or non-blocking areas can be created. Although the optimization engine tries to spare doses to the normal tissues between the subvolumes, it can conflict with PTV dose coverage. However, MLC is limited to move along one direction, and only one MLC segment is allowed at one discretized gantry angle in VMAT. Then, the MLC segment may have to adopt unnecessary dose delivery to normal tissues. Consequently, it can deteriorate dose conformity. Even if more stringent OAR constraints are applied to improve the quality of dose optimization in VMAT-FF, it could be challenging to improve dose conformity without increasing hot spots unless the MLC segments are improved. However, HF can effectively guide the optimization engine to utilize its ability for a limited area. As the HF is integrated with VMAT, which uses arc beams going through different gantry angles, VMAT-HF can successfully induce optimal and deliverable MLC segments, as shown in Figure 5B.
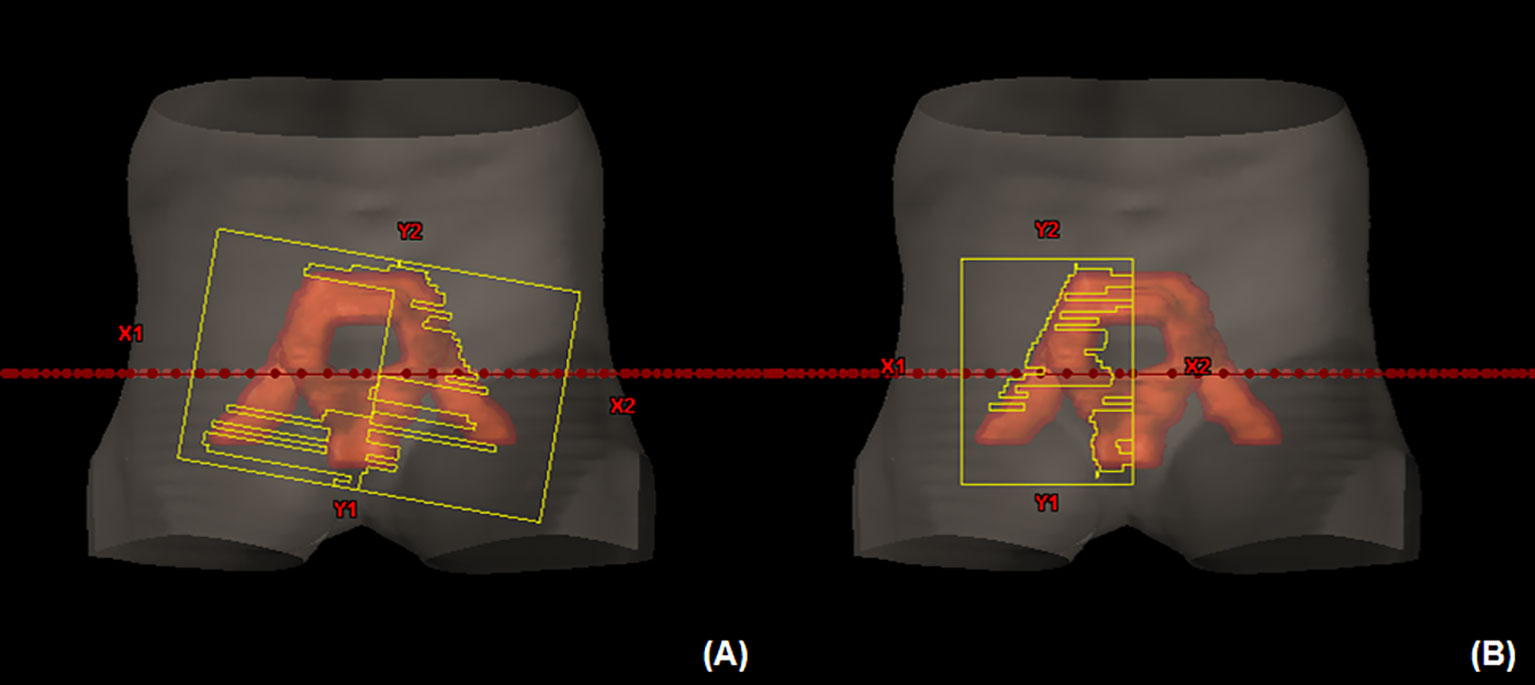
Figure 5 (A) A suboptimal multi-leaf collimator (MLC) segment in volumetric modulated arc therapy (VMAT) optimized using a fully opened field compared to (B) an MLC segment for the planning target volume in VMAT optimized using a half-beam technique at the beam’s eye view.
Such an optimal MLC segment in VMAT-HF led to achieving comparable or superior conformal dose distributions without excessive beam modulation presented with MCS. The modulation index can be a useful tool to comprehensively evaluate beam modulation complexities based on MLC segments (55). However, the lower modulation complexity and indices would not always result in precise dose delivery showing better agreement between predicted and delivered doses (56, 57). In this study, without sacrificing beam delivery efficiency and accuracy in terms of beam-on time and MLC position accuracy, VMAT-HF could achieve effective OAR dose sparing.
Different dose–volume parameters were used to find the best predictor to reduce the incidence of acute and late GI toxicities. The GI tract showed radiation dose–volume effects and maximum dose effect to a small volume in estimating acute and late toxicity. Clinical studies showed significant dose–volume predictors on acute and late GI toxicities according to treatment modalities before and after RT, prescribed doses, and dose fractionations (58, 59). In WPRT using IMRT, while the volume receiving high-dose >45 Gy showed a strong correlation with a higher incidence of acute small bowel’s toxicity for gynecologic cancers (14), less acute GI toxicity was observed by a reduction of volume receiving the intermediate dose of 30–35 Gy among prostate patients receiving a whole pelvic dose of 54 Gy (40). Three-dimensional conformal RT for rectal cancer showed a significant correlation of acute toxicity with the volume exposed to a lower dose, particularly at or less than 15 Gy, for small bowel during chemoradiotherapy (44). When the maximum dose in the small bowel or colon has to be compromised for target dose coverage, volume reduction to high and intermediate dose could reduce GI tract toxicities (38, 40). As VMAT-HF showed statistically significant GI and GU dose sparing to intermediate-dose >15 Gy and lower NTCP compared to VMAT-FF, potentially less acute and late GI toxicity can be likely expected in WPRT using VMAT-HF.
Conclusions
This dosimetric study was conducted to effectively save OAR doses for WPRT via dose optimization using the HF. The VMAT-HF achieved noticeable physical dose sparing for GI tract and bladder and significantly lower NTCP even using less beam modulation complexity. The VMAT-HF showed conformal dose distribution using optimal MLC segments without non-blocking phenomena. The VMAT-HF showed potential dosimetric benefits compared to VMAT-FF without sacrificing beam delivery efficiency and MLC leaf position precision.
Data Availability Statement
The original contributions presented in the study are included in the article/supplementary material. Further inquiries can be directed to the corresponding authors.
Ethics Statement
This retrospective dosimetric study was approved by the Institutional Review Board of the Dongguk University Medical Center (110757-201711-HR-02-01), and written informed consent was obtained from all patients.
Author Contributions
HJ, JP, MA, and H-JP conceived this study. HJ collected clinical cases and delineated structures for VMAT. JP, MA, YZ, and H-JP performed treatment planning. JP, MA, and YZ created computation modules to analyze results. JR analyzed the data and conducted the statistical analysis. SH, PJ, M-HK, MC, Y-TO, and ON provided clinical expertise and reviewed the VMAT plans and data. All authors contributed to the article and approved the submitted version.
Conflict of Interest
The authors declare that the research was conducted in the absence of any commercial or financial relationships that could be construed as a potential conflict of interest.
Publisher’s Note
All claims expressed in this article are solely those of the authors and do not necessarily represent those of their affiliated organizations, or those of the publisher, the editors and the reviewers. Any product that may be evaluated in this article, or claim that may be made by its manufacturer, is not guaranteed or endorsed by the publisher.
Acknowledgments
We thank our colleagues at the Department of Radiation Oncology, Ajou University of School of Medicine, and the University of Florida Health Proton Therapy Institute, who assisted in the research.
References
1. Hong TS, Moughan J, Garofalo MC, Bendell J, Berger AC, Oldenburg NB, et al. NRG Oncology Radiation Therapy Oncology Group 0822: A Phase 2 Study of Preoperative Chemoradiation Therapy Using Intensity Modulated Radiation Therapy in Combination With Capecitabine and Oxaliplatin for Patients With Locally Advanced Rectal Cancer. Int J Radiat Oncol Biol Phys (2015) 93(1):29–36. doi: 10.1016/j.ijrobp.2015.05.005
2. Jhingran A, Winter K, Portelance L, Miller B, Salehpour M, Gaur R, et al. A Phase II Study of Intensity Modulated Radiation Therapy to the Pelvis for Postoperative Patients With Endometrial Carcinoma: Radiation Therapy Oncology Group Trial 0418. Int J Radiat Oncol Biol Phys (2012) 84(1):e23–28. doi: 10.1016/j.ijrobp.2012.02.044
3. Arcadipane F, Franco P, Ceccarelli M, Furfaro G, Rondi N, Trino E, et al. Image-Guided IMRT With Simultaneous Integrated Boost as Per RTOG 0529 for the Treatment of Anal Cancer. Asia Pac J Clin Oncol (2017) 14(3):217–23. doi: 10.1111/ajco.12768
4. Tomasoa NB, Meulendijks D, Nijkamp J, Cats A, Dewit L. Clinical Outcome in Patients Treated With Simultaneous Integrated Boost – Intensity Modulated Radiation Therapy (SIB-IMRT) With and Without Concurrent Chemotherapy for Squamous Cell Carcinoma of the Anal Canal. Acta Oncol (2016) 55(6):760–6. doi: 10.3109/0284186X.2015.1124141
5. Franco P, Ragona M, Arcadipane F, Mistrangelo M, Cassoni P, Rondi N, et al. Dosimetric Predictors of Acute Hematologic Toxicity During Concurrent Intensity-Modulated Radiotherapy and Chemotherapy for Anal Cancer. Clin Transl Oncol (2017) 19(1):67–75. doi: 10.1007/s12094-016-1504-2
6. Huang J, Gu F, Ji T, Zhao J, Li G. Pelvic Bone Marrow Sparing Intensity Modulated Radiotherapy Reduces the Incidence of the Hematologic Toxicity of Patients With Cervical Cancer Receiving Concurrent Chemoradiotherapy: A Single-Center Prospective Randomized Controlled Trial. Radiat Oncol (2020) 15(1):180. doi: 10.1186/s13014-020-01606-3
7. Dirix P, Haustermans K, Junius S, Withers R, Oyen R, Van Poppel H. The Role of Whole Pelvic Radiotherapy in Locally Advanced Prostate Cancer. Radiother Oncol (2006) 79(1):1–14. doi: 10.1016/j.radonc.2006.03.011
8. Mangar SA. The Re-Emerging Role of Elective Pelvic Nodal Irradiation in High-Risk Node Negative Prostate Cancer. J Cancer Ther (2017) 8:1135–48. doi: 10.4236/jct.2017.813097
9. Lawton CA, DeSilvio M, Roach M 3rd, Uhl V, Kirsch R, Seider M, et al. An Update of the Phase III Trial Comparing Whole Pelvic to Prostate Only Radiotherapy and Neoadjuvant to Adjuvant Total Androgen Suppression: Updated Analysis of RTOG 94-13, With Emphasis on Unexpected Hormone/Radiation Interactions. Int J Radiat Oncol Biol Phys (2007) 69(3):646–55. doi: 10.1016/j.ijrobp.2007.04.003
10. Roach M, Moughan J, Lawton CAF, Dicker AP, Zeitzer KL, Gore EM, et al. Sequence of Hormonal Therapy and Radiotherapy Field Size in Unfavourable, Localised Prostate Cancer (NRG/RTOG 9413): Long-Term Results of a Randomised, Phase 3 Trial. Lancet Oncol (2018) 19(11):1504–15. doi: 10.1016/S1470-2045(18)30528-X
11. Pollack A, Low D, Watkins-Bruner D, Speight JC, Gomella LG, Aminm MB, et al. Radiation Therapy Oncology Group 0534: A Phase III Trial of Short Term Androgen Deprivation With Pelvic Lymph Node or Prostate Bed Only Radiotherapy (SPPORT) in Prostate Cancer Patients With a Rising PSA After Radical Prostatectomy. (2010). Available online at: https://clinicaltrials.gov/ProvidedDocs/80/NCT00567580/Prot_SAP_000.pdf.
12. Pollack A, Karrison TG, Balogh TG Jr, Low D, Bruner DW, Wefel JS, et al. Short Term Androgen Deprivation Therapy Without or With Pelvic Lymph Node Treatment Added to Prostate Bed Only Salvage Radiotherapy: The NRG Oncology/RTOG 0534 SPPORT Trial. 2018 ASTRO Annual Meeting; 2018 Oct 21-24; San Antonio, Texas, USA. Int J Radiat Oncol Biol Phys (2018) 102(5):1605. doi: 10.1016/j.ijrobp.2018.08.052
13. Roach M, DeSilvio M, Valicenti R, Grignon D, Asbell SO, Lawton C, et al. Whole-Pelvis, “Mini-Pelvis,” or Prostate-Only External Beam Radiotherapy After Neoadjuvant and Concurrent Hormonal Therapy in Patients Treated in the Radiation Therapy Oncology Group 9413 Trial. Int J Radiat Oncol Biol Phys (2006) 66(3):647–53. doi: 10.1016/j.ijrobp.2006.05.074
14. Roeske JC, Bonta D, Mell LK, Lujan AE, Mundt AJ. A Dosimetric Analysis of Acute Gastrointestinal Toxicity in Women Receiving Intensity-Modulated Whole-Pelvic Radiation Therapy. Radiother Oncol (2003) 69(2):201–7. doi: 10.1016/j.radonc.2003.05.001
15. Yang TJ, Oh JH, Son CH, Apte A, Deasy JO, Wu A, et al. Predictors of Acute Gastrointestinal Toxicity During Pelvic Chemoradiotherapy in Patients With Rectal Cancer. Gastrointest Cancer Res (2013) 6(5-6):129–36.
16. Mabuchi S, Isohashi F, Yokoi T, Takemura M, Yoshino K, Shiki Y, et al. A Phase II Study of Postoperative Concurrent Carboplatin and Paclitaxel Combined With Intensity-Modulated Pelvic Radiotherapy Followed by Consolidation Chemotherapy in Surgically Treated Cervical Cancer Patients With Positive Pelvic Lymph Nodes. Gynecol Oncol (2016) 141(2):240–6. doi: 10.1016/j.ygyno.2016.02.011
17. Holliday EB, Lester SC, Harmsen WS, Eng C, Haddock MG, Krishnan S, et al. Extended-Field Chemoradiation Therapy for Definitive Treatment of Anal Canal Squamous Cell Carcinoma Involving the Para-Aortic Lymph Nodes. Int J Radiat Oncol Biol Phys (2018) 102(1):102–8. doi: 10.1016/j.ijrobp.2018.04.076
18. Lestrade L, Zilli T, Kountouri M, Jumeau R, Matzinger O, Bourhis J, et al. Early-Stage Favourable Anal Cancer: A Retrospective Analysis of Clinical Outcomes of a Moderately Low Dose Elective Nodal Intensity-Modulated Radiotherapy Schedule. Clin Oncol (R Coll Radiol) (2017) 29(7):e105–9. doi: 10.1016/j.clon.2017.01.044
19. Magli A, Moretti E, Tullio A, Giannarini G, Tonetto F, Urpis M, et al. Hypofractionated Simultaneous Integrated Boost (IMRT-SIB) With Pelvic Nodal Irradiation and Concurrent Androgen Deprivation Therapy for High-Risk Prostate Cancer: Results of a Prospective Phase II Trial. Prostate Cancer Prostatic Dis (2018) 21(2):269–76. doi: 10.1038/s41391-018-0034-0
20. Lin YW, Lin LC, Lin KL. The Early Result of Whole Pelvic Radiotherapy and Stereotactic Body Radiotherapy Boost for High-Risk Localized Prostate Cancer. Front Oncol (2014) 4:278. doi: 10.3389/fonc.2014.00278
21. Ishii K, Ogino R, Hosokawa Y, Fujioka C, Okada W, Nakahara R, et al. Whole-Pelvic Volumetric-Modulated Arc Therapy for High-Risk Prostate Cancer: Treatment Planning and Acute Toxicity. J Radiat Res (2015) 56(1):141–50. doi: 10.1093/jrr/rru086
22. Wong E, D’Souza DP, Chen JZ, Lock M, Rodrigues G, Coad T, et al. Intensity-Modulated Arc Therapy for Treatment of High-Risk Endometrial Malignancies. Int J Radiat Oncol Biol Phys (2005) 61(3):830–41. doi: 10.1016/j.ijrobp.2004.06.253
23. Ishii K, Ogino R, Hosokawa Y, Fujioka C, Okada W, Nakahara R, et al. Comparison of Dosimetric Parameters and Acute Toxicity After Whole-Pelvic vs Prostate-Only Volumetric-Modulated Arc Therapy With Daily Image Guidance for Prostate Cancer. Br J Radiol (2016) 89(1062):20150930. doi: 10.1259/bjr.20150930
24. Huang B, Fang Z, Huang Y, Lin P, Chen Z. A Dosimetric Analysis of Volumetric-Modulated Arc Radiotherapy With Jaw Width Restriction vs 7 Field Intensity-Modulated Radiotherapy for Definitive Treatment of Cervical Cancer. Br J Radiol (2014) 87(1039):20140183. doi: 10.1259/bjr.20140183
25. Rossi M, Boman E, Skytta T, Kapanen M. A Novel Arc Geometry Setting for Pelvic Radiotherapy With Extensive Nodal Involvement. J Appl Clin Med Phys (2016) 17(4):73–85. doi: 10.1120/jacmp.v17i4.6028
26. Zhang WZ, Lu JY, Chen JZ, Zhai TT, Huang BT, Li DR, et al. A Dosimetric Study of Using Fixed-Jaw Volumetric Modulated Arc Therapy for the Treatment of Nasopharyngeal Carcinoma With Cervical Lymph Node Metastasis. PLoS One (2016) 11(5):e0156675. doi: 10.1371/journal.pone.0156675
27. Benson AB, Venook AP, Al-Hawary MM, Cederquist L, Chen YJ, Ciombor KK, et al. Anal Carcinoma, Version 2.2018, NCCN Clinical Practice Guidelines in Oncology. J Natl Compr Canc Netw (2018) 16(7):852–71. doi: 10.6004/jnccn.2018.0060
28. Koh WJ, Abu-Rustum NR, Bean S, Bradley K, Campos SM, Cho KR, et al. Cervical Cancer, Version 3.2019, NCCN Clinical Practice Guidelines in Oncology. J Natl Compr Canc Netw (2019) 17(1):64–84. doi: 10.6004/jnccn.2019.0001
29. Small W Jr., Mell LK, Anderson P, Creutzberg C, De Los Santos J, Gaffney D, et al. Consensus Guidelines for Delineation of Clinical Target Volume for Intensity-Modulated Pelvic Radiotherapy in Postoperative Treatment of Endometrial and Cervical Cancer. Int J Radiat Oncol Biol Phys (2008) 71(2):428–34. doi: 10.1016/j.ijrobp.2007.09.042
30. Gay HA, Barthold HJ, O’Meara E, Bosch WR, El Naqa I, Al-Lozi R, et al. Pelvic Normal Tissue Contouring Guidelines for Radiation Therapy: A Radiation Therapy Oncology Group Consensus Panel Atlas. Int J Radiat Oncol Biol Phys (2012) 83(3):e353–362. doi: 10.1016/j.ijrobp.2012.01.023
31. Myerson RJ, Garofalo MC, El Naqa I, Abrams RA, Apte A, Bosch WR, et al. Elective Clinical Target Volumes for Conformal Therapy in Anorectal Cancer: A Radiation Therapy Oncology Group Consensus Panel Contouring Atlas. Int J Radiat Oncol Biol Phys (2009) 74(3):824–30. doi: 10.1016/j.ijrobp.2008.08.070
32. Ahamad A, D’Souza W, Salehpour M, Iyer R, Tucker SL, Jhingran A, et al. Intensity-Modulated Radiation Therapy After Hysterectomy: Comparison With Conventional Treatment and Sensitivity of the Normal-Tissue-Sparing Effect to Margin Size. Int J Radiat Oncol Biol Phys (2005) 62(4):1117–24. doi: 10.1016/j.ijrobp.2004.12.029
33. McNiven AL, Sharpe MB, Purdie TG. A New Metric for Assessing IMRT Modulation Complexity and Plan Deliverability. Med Phys (2010) 37(2):505–15. doi: 10.1118/1.3276775
34. Van’t Riet A, Mak AC, Moerland MA, Elders LH, van der Zee W. A Conformation Number to Quantify the Degree of Conformality in Brachytherapy and External Beam Irradiation: Application to the Prostate. Int J Radiat Oncol Biol Phys (1997) 37(3):731–6. doi: 10.1016/s0360-3016(96)00601-3
35. International Commission on Radiation Units and Measurements. The ICRU Report 83: Prescribing, Recording and Reporting Photon Beam Intensity-Modulated Radiation Therapy (IMRT). J ICRU 10(1). doi: 10.1093/jicru/10.1.Report83
36. Yoon M, Park SY, Shin D, Lee SB, Pyo HR, Kim DY, et al. A New Homogeneity Index Based on Statistical Analysis of the Dose-Volume Histogram. J Appl Clin Med Phys (2007) 8(2):9–17. doi: 10.1120/jacmp.v8i2.2390
37. Kamran SC, Mamon HJ, Wo JY. Rectal and Colon Cancer: Radiation Therapy Planning. In: Hong T, Das P, editors. Radiation Therapy for Gastrointestinal Cancers. Cham, Switerland: Springer (2017). p. 171–80.
38. Kavanagh BD, Pan CC, Dawson LA, Das SK, Li XA, Ten Haken RK, et al. Radiation Dose–Volume Effects in the Stomach and Small Bowel. Int J Radiat Oncol Biol Phys (2010) 76(3):S101–7. doi: 10.1016/j.ijrobp.2009.05.071
39. Valdagni R, Rancati T, Fiorino C. Predictive Models of Toxicity With External Radiotherapy for Prostate Cancer: Clinical Issues. Cancer (2009) 115(13 Suppl):3141–9. doi: 10.1002/cncr.24356
40. Cozzarini C, Fiorino C, Di Muzio N, Alongi F, Broggi S, Cattaneo M, et al. Significant Reduction of Acute Toxicity Following Pelvic Irradiation With Helical Tomotherapy in Patients With Localized Prostate Cancer. Radiother Oncol (2007) 84(2):164–70. doi: 10.1016/j.radonc.2007.07.013
41. Fokdal L, Honoré H, Høyer M, Vone Der Masse H. Dose-Volume Histograms Associated to Long-Term Colorectal Functions in Patients Receiving Pelvic Radiotherapy. Radiother Oncol (2005) 74(2):203–10. doi: 10.1016/j.radonc.2004.11.001
42. Huang EY, Sung CC, Ko SF, Wang CJ, Yang KD. The Different Volume Effects of Small-Bowel Toxicity During Pelvic Irradiation Between Gynecologic Patients With and Without Abdominal Surgery: A Prospective Study With Computed Tomography-Based Dosimetry. Int J Radiat Oncol Biol Phys (2007) 69(3):732–9. doi: 10.1016/j.ijrobp.2007.03.060
43. Michalski JM, Gay H, Jackson A, Tucker SL, Deasy JO. Radiation Dose-Volume Effects in Radiation-Induced Rectal Injury. Int J Radiat Oncol Biol Phys (2010) 76(3 Suppl):S123–9. doi: 10.1016/j.ijrobp.2009.03.078
44. Robertson JM, Lockman D, Yan D, Wallace M. The Dose-Volume Relationship of Small Bowel Irradiation and Acute Grade 3 Diarrhea During Chemoradiotherapy for Rectal Cancer. Int J Radiat Oncol Biol Phys (2002) 70(2):413–8. doi: 10.1016/j.ijrobp.2007.06.066
45. Burman C, Kutcher GJ, Emami B, Goitein M. Fitting of Normal Tissue Tolerance Data to an Analytic Function. Int J Radiat Oncol Biol Phys (1991) 21(1):123–35. doi: 10.1016/0360-3016(91)90172-z
46. Luxton G, Keall PJ, King CR. A New Formula for Normal Tissue Complication Probability (NTCP) as a Function of Equivalent Uniform Dose (EUD). Phys Med Biol (2008) 53(1):23–36. doi: 10.1088/0031-9155/53/1/002
47. Moiseenko V, Song WY, Mell LK, Bhandare N. A Comparison of Dose-Response Characteristics of Four NTCP Models Using Outcomes of Radiation-Induced Optic Neuropathy and Retinopathy. Radiat Oncol (2011) 6:61. doi: 10.1186/1748-717X-6-61
48. Dasu A, Toma-Dasu I, Olofsson J, Karlsson M. The Use of Risk Estimation Models for the Induction of Secondary Cancers Following Radiotherapy. Acta Oncol (2005) 44(4):339–47. doi: 10.1080/02841860510029833
49. Varian Medical System. Dynalog File Viewer Reference Guide (Document ID: 100013698-08). Palo Alto, CA, USA: Varian Medical System, Inc (2015).
50. Yan G, Liu C, Simon TA, Peng LC, Fox C, Li JG. On the Sensitivity of Patient-Specific IMRT QA to MLC Positioning Errors. J Appl Clin Med Phys (2008) 10(1):120–8. doi: 10.1120/jacmp.v10i1.2915
51. Kagkiouzis J, Platoni K, Kantzou I, Dilvoi M, Patatoukas G, Kypraiou E, et al. Review of the Three-Field Techniques in Breast Cancer Radiotherapy. J BUON (2017) 22(3):599–605.
52. Abdel-Hakim K, Nishimura T, Takai M, Skahara H. Review of Monoisocentric Split-Field Technique for Conventional and IMRT Treatment in Head and Neck Cancers: Technical Limitations and Approaches for Optimization. Technol Cancer Res Treat (2005) 4(1):107–13. doi: 10.1177/153303460500400114
53. Lai Y, Chen Y, Wu S, Shi L, Fu L, Ha H, et al. Modified Volumetric Modulated Arc Therapy in Left Sided Breast Cancer After Radical Mastectomy With Flattening Filter Free Versus Flattened Beams. Med (Baltimore) (2016) 95(14):e3295. doi: 10.1097/MD.0000000000003295
54. Casey KE, Wong PF, Tung SS. Effect of Interfractional Shoulder Motion on Low Neck Nodal Targets for Patients Treated Using Volumetric-Modulated Arc Therapy (VMAT). J Appl Clin Med Phys (2015) 16(4):40–51. doi: 10.1120/jacmp.v16i4.5206
55. McGarry CK, Agnew CE, Hussein M, Tsang Y, McWilliam A, Hounsell AR, et al. The Role of Complexity Metrics in a Multi-Institutional Dosimetry Audit of VMAT. Br J Radiol (2016) 89(1057):20150445. doi: 10.1259/bjr.20150445
56. Crowe SB, Kairn T, Middlebrook N, Sutherland B, Hill B, Kenny J, et al. Examination of the Properties of IMRT and VMAT Beams and Evaluation Against Pre-Treatment Quality Assurance Results. Phys Med Biol (2015) 60(6):2587–601. doi: 10.1088/0031-9155/60/6/2587
57. Glenn MC, Hernandez V, Saez J, Followill DS, Howell RM, Pollard-Larkin JM, et al. Treatment Plan Complexity Does Not Predict IROC Houston Anthropomorphic Head and Neck Phantom Performance. Phys Med Biol (2018) 63(20):205015. doi: 10.1088/1361-6560/aae29e
58. Fowler JF. The Radiobiology of Prostate Cancer Including New Aspects of Fractionated Radiotherapy. Acta Oncol (2005) 44(3):265–76. doi: 10.1080/02841860410002824
Keywords: volumetric modulated arc therapy, half beams, whole pelvic conformal radiotherapy, normal tissue complication probability (NTCP), modulation complexity score, dose conformity
Citation: Jang H, Park J, Artz M, Zhang Y, Ricci JC, Huh S, Johnson PB, Kim M-H, Chun M, Oh Y-T, Noh OK and Park H-J (2021) Effective Organs-at-Risk Dose Sparing in Volumetric Modulated Arc Therapy Using a Half-Beam Technique in Whole Pelvic Irradiation. Front. Oncol. 11:611469. doi: 10.3389/fonc.2021.611469
Received: 29 September 2020; Accepted: 19 July 2021;
Published: 18 August 2021.
Edited by:
Tiziana Rancati, Istituto Nazionale dei Tumori (IRCCS), ItalyReviewed by:
Alan McWilliam, The University of Manchester, United KingdomPierfrancesco Franco, University of Eastern Piedmont, Italy
Stefania Naccarato, Advanced Radiation Oncology IRCCS Ospedale Sacro Don Calabria, Italy
Lidia Strigari, IRCCS Azienda Universitaria di Bologna, Italy
Copyright © 2021 Jang, Park, Artz, Zhang, Ricci, Huh, Johnson, Kim, Chun, Oh, Noh and Park. This is an open-access article distributed under the terms of the Creative Commons Attribution License (CC BY). The use, distribution or reproduction in other forums is permitted, provided the original author(s) and the copyright owner(s) are credited and that the original publication in this journal is cited, in accordance with accepted academic practice. No use, distribution or reproduction is permitted which does not comply with these terms.
*Correspondence: Hae-Jin Park, Haejin.Park.physicist@gmail.com; Young-Taek Oh, ohyoung@ajou.ac.kr
†These authors have contributed equally to this work and share first authorship