- 1Department of Pathology, Fudan University Shanghai Cancer Center, Shanghai, China
- 2Department of Oncology, Shanghai Medical College, Fudan University, Shanghai, China
Recent studies have demonstrated the benefits of metformin on patients with lymphomas. B-cell receptor (BCR)-PI3K-AKT pathway-dependent cholesterol synthesis may represent a positive feedback mechanism responsible for the pathogenesis of BCR-dependent diffuse large B-cell lymphomas (DLBCLs). Thus, restriction of lipid synthesis would affect the integrity of lipid-forming membranes and block the BCR signaling pathway. Our in vitro findings suggested that the blocking effect of metformin on BCR signaling pathway is possibly exerted via blocking the biosynthesis of cholesterol. A retrospective case-control study was subsequently conducted on type II diabetic patients with DLBCL who were on metformin. Metformin was identified to be associated with improved response rate and PFS in diabetic patients and appeared to be an effective therapeutic drug against DLBCL.
Introduction
Metformin is one of the most common medications for diabetic patients and can enhance insulin sensitivity by decreasing hepatic glucose production and increasing peripheral tissue glucose use (1). In addition to its hypoglycemic effect, recent clinical studies have roused interest in its anticancer role in different malignancies (2–7). There are also a few studies on the role of metformin in hematological malignancies, such as monoclonal gammopathy of undetermined significance and multiple myeloma (8, 9). However, the effects of concomitant metformin use on the clinical outcomes of lymphoma, for example, the most common subtype, diffuse large B-cell lymphoma (DLBCL) patients remain unclear. Limited retrospective or prospective studies have demonstrated inconsistent results (10, 11).
Several studies have explored the possible mechanisms underlying the anticancer effects of metformin, and adenosine 5’-monophosphate (AMP)-activated protein kinase (AMPK) seems to play an important role (12–14). As one of the serine/threonine protein kinase family members, AMPK can be activated by metformin, and is involved in the regulation of almost all metabolic processes in cells. The metabolic regulatory effects of AMPK classically target the liver, fat, and muscle, and its lipid metabolic effects involve increasing energy material by promoting catabolism and reducing material synthesis by inhibiting anabolism (15–17). AMPK downregulation might further facilitate lymphomagenesis, indicating that AMPK activation can potentially be used to treat lymphoma (18).
Recently, updated schemes of the classification of DLBCL have been proposed according to its gene alterations (19–21). Different molecular subtypes of DLBCL may correlate to different mechanisms of pathogenesis, and thus arouse oncologists’ interests of individualized targeted therapy. It is noteworthy that the survival and progression of a substantial fraction of DLBCL appear to be highly dependent on the B-cell receptor (BCR) signaling. For example, there are genetic alterations involving the BCR-dependent NF-κ B signaling pathway in the MCD and BN2 subtypes of DLBCL, and involving the PI3K pathway in the ST2 and EZB subtypes (19–21). In addition, three subgroups of DLBCL have also been proposed by Monti et al. based on their transcriptional profiles, including the BCR, oxidative phosphorylation (OxPhos), and host response (HR) subtypes (22). In those BCR-dependent DLBCL cases, the activated BCRs are mobile, aggregated, and polarized via lipid rafts on the cell membrane surface to amplify the signal transduction effect (23, 24). Lipid rafts-consist of lipids (such as cholesterol), which are essential for this BCR function by maintaining the integrity of lipid rafts (25–27).
BCR is important for the survival of DLBCL, and blocking the BCR signaling pathway may thus inhibit tumor cell proliferation. There remains a question if BCR signaling, or, the metabolism of cholesterol in BCR-dependent DLBCL cells, is related to the metformin-induced activation of AMPK? We thus explored the effects of metformin on the BCR signaling and cholesterol synthesis in DLBCL cell lines first, and then compared the outcomes of diabetic DLBCL patients on metformin with those on other hypoglycemic agents.
Materials and Methods
Cell Experiments
Three BCR-dependent (with surface Ig) DLBCL cell lines, OCI-LY1 (IMDM, GIBCO), OCI-LY8 (IMDM, GIBCO), and NU-DUL-1 (1640, GIBCO), were employed. All of the cells were maintained at 37°C in a 5% CO2 incubator. The status of surface Ig in the cell lines was provided by American Tissue Culture Collection and Deutsche Sammlung von Mikroorganismen und Zellkulturen GmbH and was validated by immunophenotyping. For BCR stimulation, or cross-linking, 3×106 cells were treated with 10 mg/mL rabbit anti-human IgM (Jackson ImmunoResearch Laboratories, West Grove, PA) or goat anti-human IgG (Jackson ImmunoResearch Laboratories) for 10 min. The expression of two BCR signaling-related molecules, phosphorylated SYK (pSYK) and phosphorylated AKT (pAKT), and a key enzyme for cholesterol biosynthesis, 3-hydroxy-3-methylglutaryl-CoA synthase1 (HMGCS1), was evaluated by immunoblotting. BCRs (Cy3-conjugated AffiniPure F(ab’)2 Fragment Rabbit Anti-Goat IgG or IgM, Jackson) and membrane cholesterol (filipin staining kit; GENMED) were further detected by an immunofluorescence assay. The cells were visualized using a Leica SP5X confocal microscope. Images were captured and analyzed using LAS AF software (Leica Microsystems CMS GmbH). And the BCR and membrane cholesterol levels were evaluated by flow cytometry (FACS Canto II, BD Biosciences) using cell suspensions (5×106 cells each). The extracellular cholesterol was quantified by gas chromatography mass spectrometry (GC/MS) using extracellular culture medium obtained by centrifugation. Cell proliferation activity was assessed using a cell counting kit (CCK-8) (Dojindo, Kumamoto, Japan) according to the manufacturer’s instructions.
Clinicopathological Studies
The studies involving human participants were reviewed and approved by the Medical Ethics Committee of Fudan University Shanghai Cancer Center. Fifty DLBCL patients with type II diabetes who received metformin throughout their chemotherapeutic treatment course were identified. We extracted important clinicopathological features, including the age, gender, stage of the disease, International Prognostic Index (IPI) score, cell-of-origin (COO) subtype of the tumor, and the outcome of treatment. DLBCL patients with type II diabetes treated with other hypoglycemic agents during the same period, but with other clinicopathological characteristics comparable, served as the control. All of these patients were treated with a regimen containing rituximab, cyclophosphamide, adriamycin, vincristine, and prednisone (R-CHOP). In order to further verify the findings by in vitro experiment, we also evaluated the expression levels of pSYK, pAKT, and HMGCS1 in selected cases of both cohorts by immunohistochemistry using formalin-fixed, paraffin-embedded tumor specimens obtained prior to the immunochemotherapy.
Statistical Analysis
Survival was determined from the time of diagnosis until the time of death (or last follow-up) or progression. Survival curves were constructed by the Kaplan–Meier method. Survival distributions were compared using the log-rank test. All of the statistical analyses were carried out using GraphPad Prism, version 7.0. Categorical variables were compared using the chi-squared test or Fisher’s exact test, and continuous variables were compared with the t test or the rank-sum test. All P-values were two sided, and a P-value ≤ 0.05 was considered to be statistically significant.
Results
Cell Experiments: Metformin May Inhibit the Growth of DLBCL by Blocking the BCR Signaling and the Biosynthesis of Cholesterol
In vitro, we observed metformin-induced growth inhibition of the tumor cells in all three DLBCL cell lines (Figure 1A). The BCR stimulation led to an increased expression of pSYK and pAKT. These activating effects, however, were remarkably ablated by a metformin pretreatment (Figure 1B). BCR stimulation also correlated with an increased expression of HMGCS1, which could be ablated by metformin pretreatment, too (Figure 2A). The GC/MS detection demonstrated that a pretreatment with metformin could reduce the extracellular cholesterol concentration (Figure 2B). By immunofluorescence, we noticed the phenomenon of BCR capping, which was caused by BCR stimulation, and represented polymers formed by BCR cross-linking. The colocalization of the cholesterol and BCR signals was observed throughout the experiment, that is, colocalized cholesterol and BCR capping signals appeared at BCR stimulation, whereas the capping signals of both decreased or disappeared following metformin treatment (Figure 3A). In addition, the BCR and membrane cholesterol levels, revealed by flow cytometry assay, were also decreased by the pretreatment with metformin after induction by BCR stimulation (Figures 3B, C).
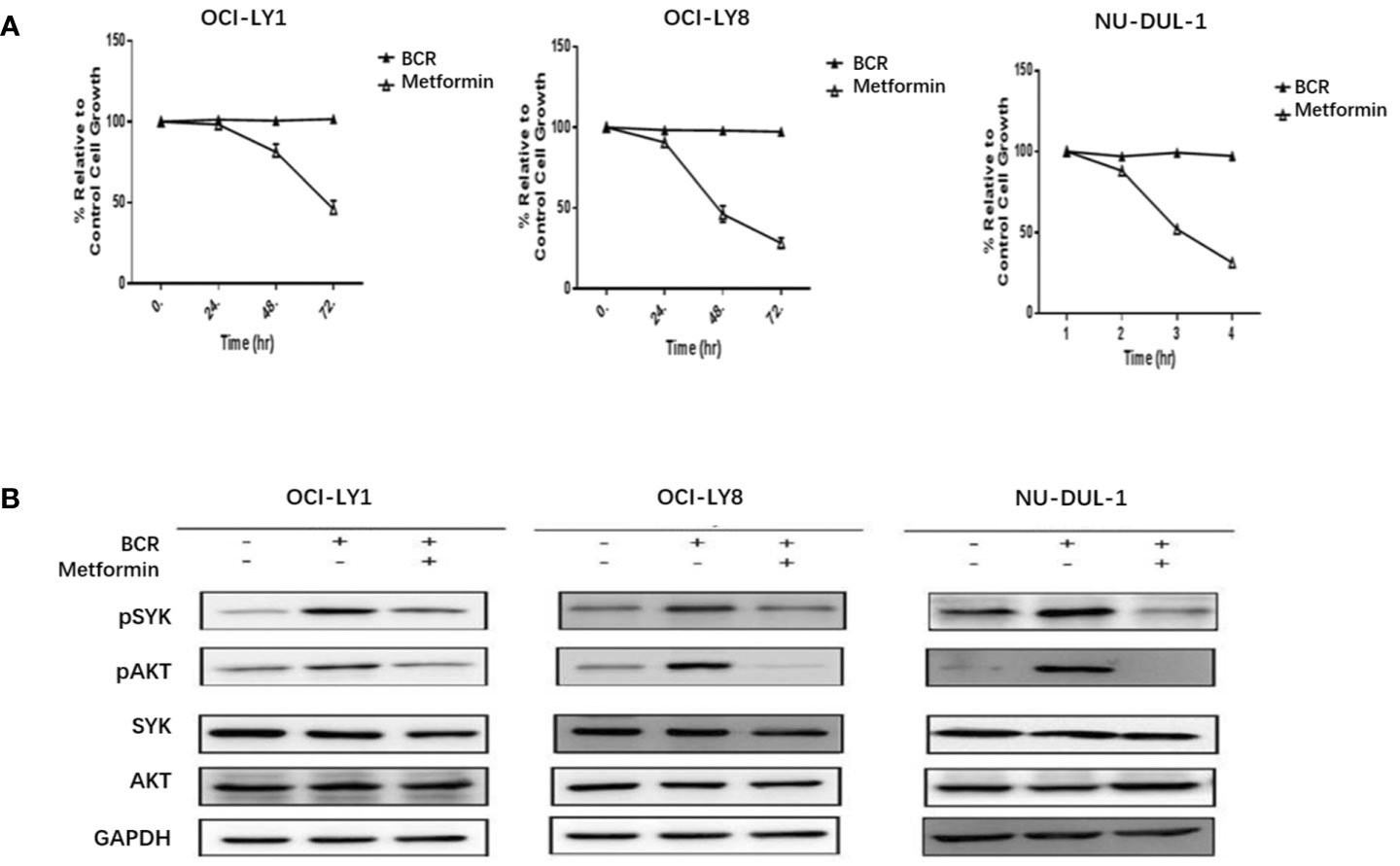
Figure 1 Metformin inhibits the growth rates of three DLBCL cell lines (A). BCR stimulation upregulates the expression of pSYK and pAKT, which is ablated by a pretreatment with metformin (B).
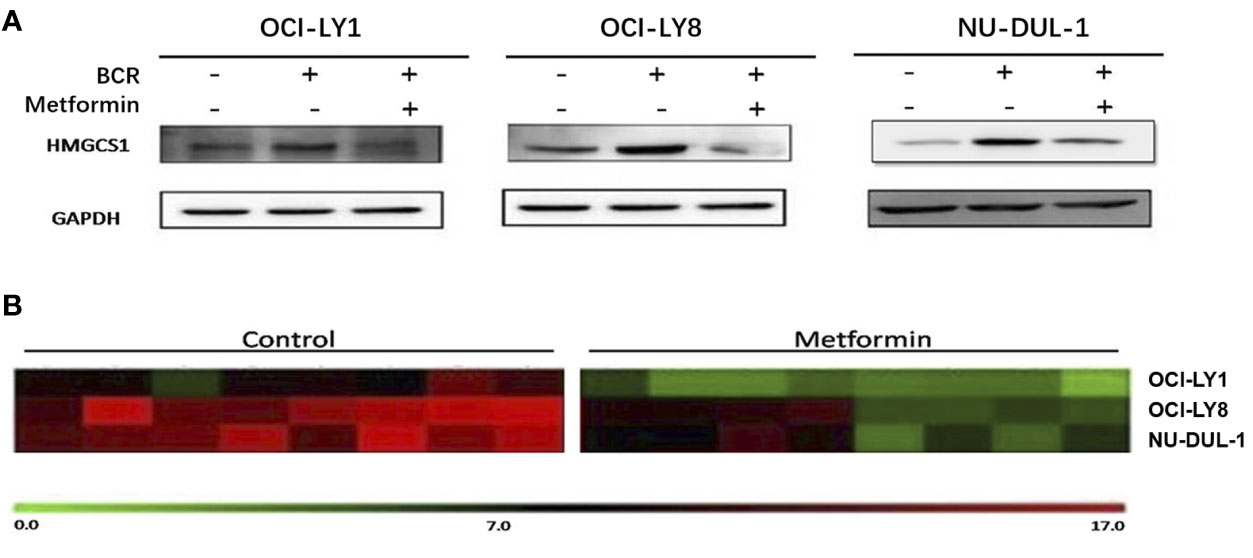
Figure 2 BCR stimulation induces an increased expression of HMGCS1, which is ablated by a pretreatment with metformin (A). The GC/MS detection demonstrates that metformin treatment can reduce extracellular cholesterol concentration (B).
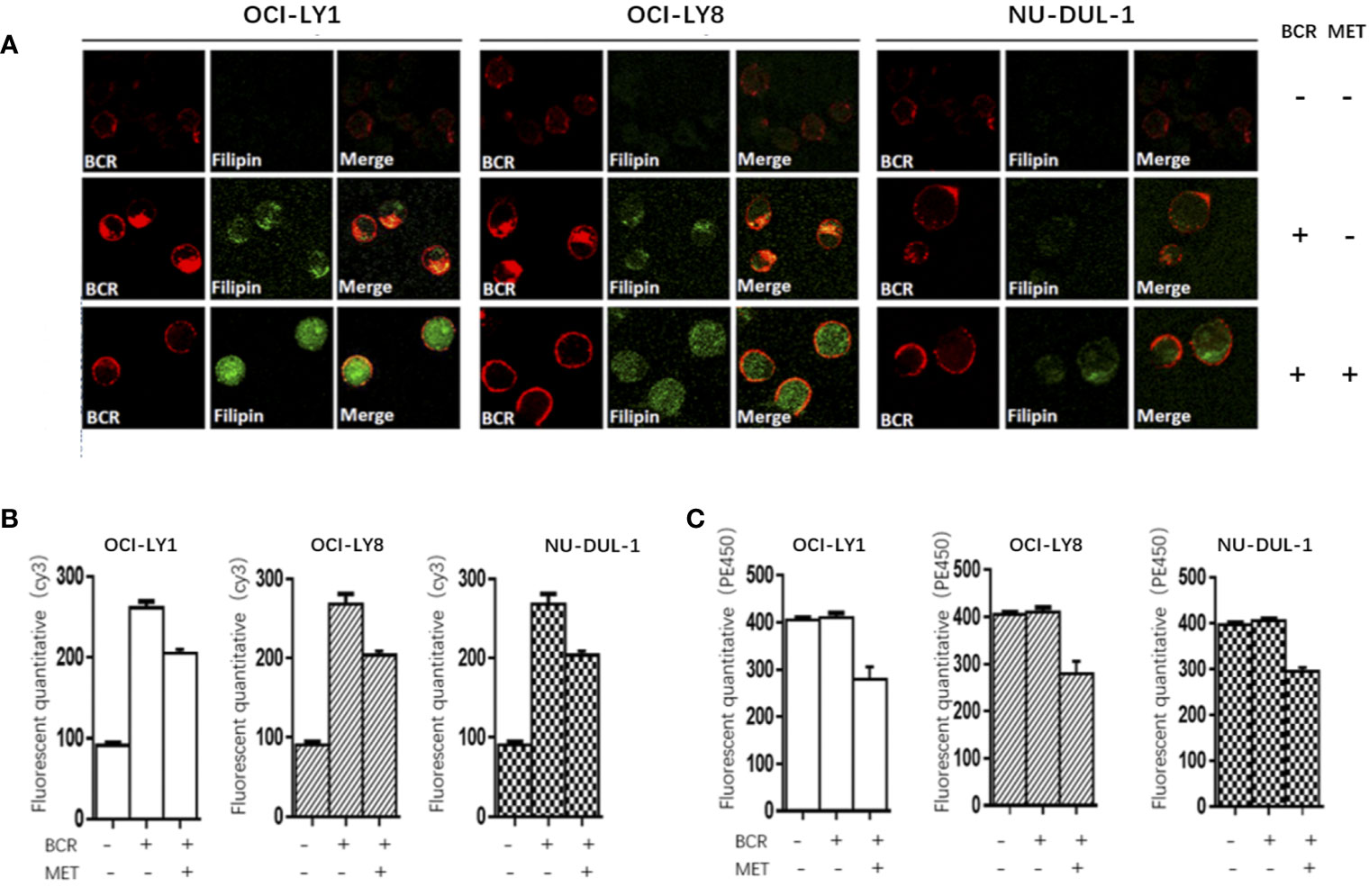
Figure 3 At BCR stimulation, the BCR capping signals (red) and cholesterol capping signals (green by Filipin) appear in three DLBCL lines, and the merged images show the colocalization of these signals. These capping signals decrease or disappear following a pretreatment of metformin (A). The Cy3 (BCR) levels increase after a BCR stimulation, and decrease following a pretreatment with metformin (B). The metformin treatment results in reduced PE450 (membrane cholesterol) levels (C).
Clinicopathological Findings: Metformin Improves the Response Rates and Survival of Diabetic DLBCL Patients
The clinicopathological characteristics of the DLBCL patients on metformin treatment and the control group were summarized in Table 1. In general, there were no significant differences with regard to the distribution of age, gender, stage of the disease, IPI score, and COO subtype of the tumor between the metformin and the control cohort. Of note, patients on metformin showed significantly higher response rates to the immunochemotherapy compared with the control group, reflected by a complete response (CR) rate of 84% and an objective response rate (ORR) of 88% in the former, in contrast to the CR rate of 48% (P = 0.0003) and the ORR of 68% (P = 0.0283) in the control cohort. The metformin group (with a medium follow-up period of 33 months) also featured a better progression-free survival (PFS) compared with the control group (with a medium follow-up period of 35 months) (P = 0.0298). Furthermore, there was a trend of improved overall survival (OS) present in the metformin group, although the difference was not statistically significant (Figure 4). Immunohistochemically, the expression levels of pSYK, pAKT and HMGCS1 in the metformin group were significantly lower than those in the control group (Figure 5).
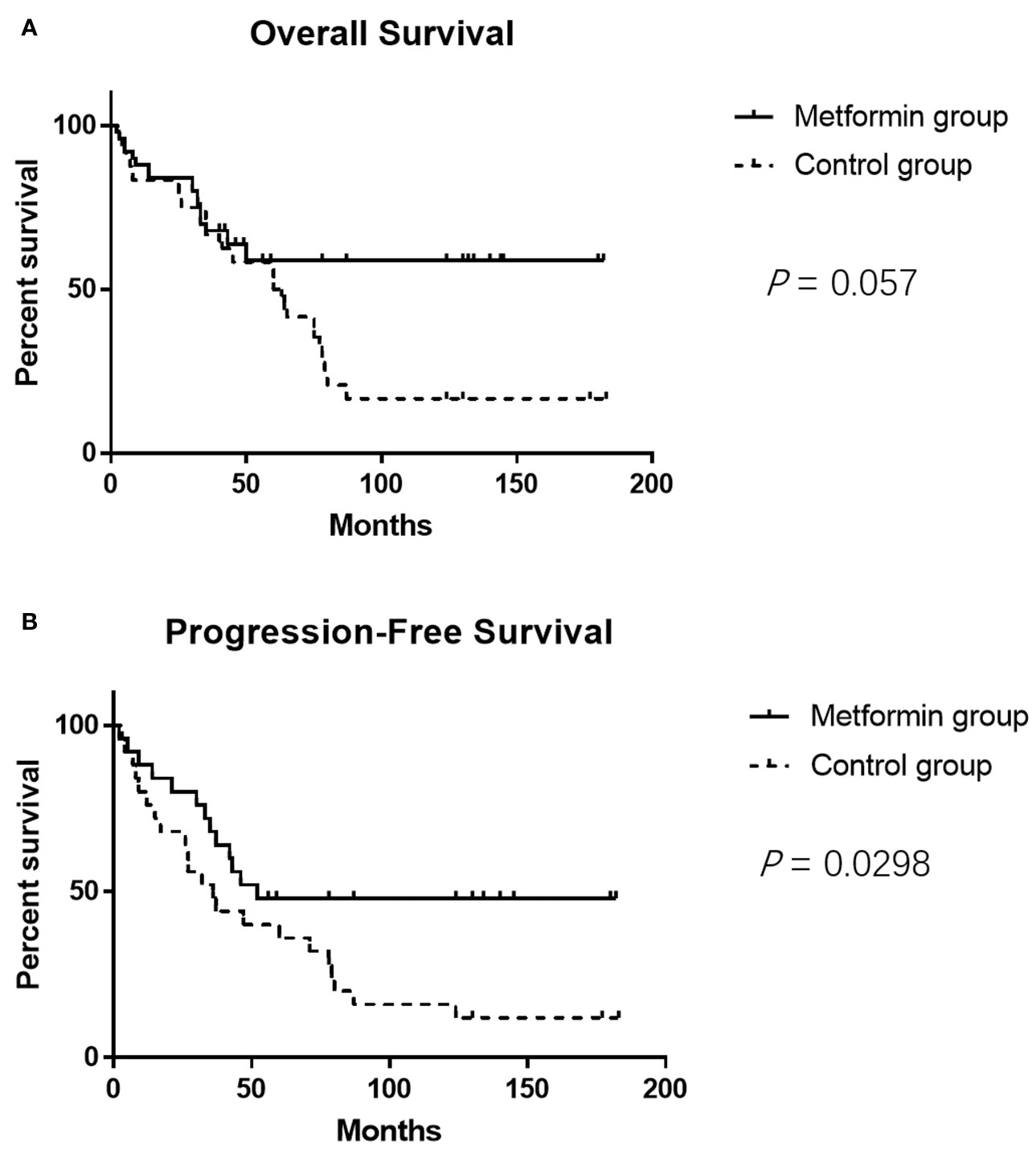
Figure 4 The metformin group shows better PFS compared with the control group (P = 0.0298) (A). It seems that the metformin group also features a trend of better OS, but the difference is not statistically significant (P = 0.057) (B).
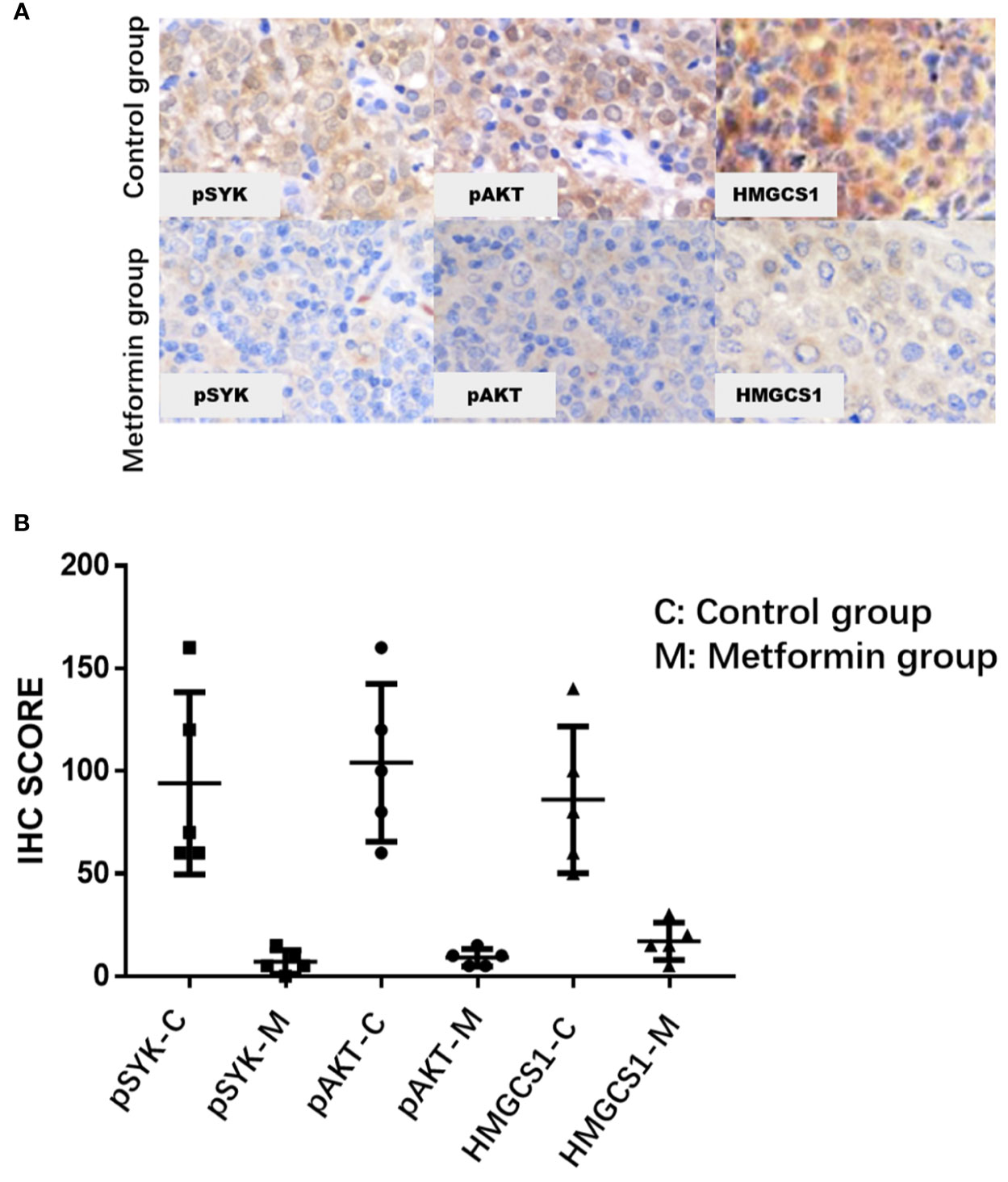
Figure 5 The immunohistochemical expression levels of pSYK, pAKT, and HMGCS1 of DLBCL patients from metformin group are significantly lower than that of control group (A, B).
Discussion
DLBCL is a group of biologically heterogeneous diseases, comprising a variety of clinicopathologically distinctive entities, which may need different therapeutic strategies. As aforementioned, Monti et al. demonstrated that DLBCL can be divided into three subgroups, the BCR, OxPhos, and HR, based on their different gene expression profiles (22). Nevertheless, the biological characteristics of these molecular subtypes were poorly understood for a long time, until Caro et al. verified the Monti’s discovery by using two-dimensional electrophoresis at the protein level. They found that mitochondrial oxidative phosphorylation-related proteins are expressed at low levels in BCR-dependent DLBCL (28), which suggests that the energy supply of BCR-dependent DLBCL may depend on anaerobic metabolism. In vitro experiments have shown the following metabolic features of BCR-dependent DLBCL: 1) great glucose demand but inefficient utilization; 2) enhanced glycolysis in the cytoplasm and creation of the small molecule “carbon” instead of ATP; 3) low oxygen consumption and highly acidic substance production; and 4) reversal of these phenomena and significant inhibition of cell proliferation upon BCR inhibition (16, 29). Moreover, cholesterol synthesis is remarkably upregulated in BCR-dependent DLBCL, and the raw material for cholesterol synthesis is just the small molecule “carbon” produced by glycolysis (24).
The lipid rafts, composed mainly of cholesterol and glycosphingolipid, are activated to achieve aggregation and polar distribution, which is essential for the signal transduction effect (27). Thus, restriction of cholesterol synthesis will further affect the integrity of cell membranes and influence the biological function of BCRs, resulting in the blockage of BCR signaling pathway. We found that metformin can block cholesterol synthesis by inhibiting the key cholesterol synthesis-related enzyme, HMGCS1, which results in a reduced production of the cholesterol contents, especially that of cell membrane. The decrease of membrane cholesterol leads to an inhibition of cholesterol-dependent BCR and its downstream signaling, that is, BCR signal transduction is blocked at the cell membrane level. However, the mechanism underlying the inhibitory effect of metformin on HMGCS1 in lymphoma cells remains largely unknown. One possibility lies in the roles played by sterol-regulatory element binding proteins (SREBPs), as it has been suggested that SREBPs can be phosphorylated and inhibited by AMPK in liver cells (16, 17, 30).
Few studies have translated the potential benefit of metformin use in DLBCL into clinical applications. A retrospective study showed that diabetic DLBCL patients treated with metformin during first-line immunochemotherapy had significantly improved PFS and OS compared to those of nondiabetic or diabetic DLBCL patients treated with other glucose-lowering agents (11). Another study on the effects of combined metformin and R-CHOP treatment on DLBCL patients, however, did not find any impacts on the response rate, event-free survival, or overall survival (10). Of note, the second study did not balance the clinicopathological parameters, such as the age, stage of disease, IPI score, and COO subtype, which may lead to potential bias, in the study and control groups. To avoid that, we designed the current case-control study to look into the effects of metformin on type II diabetic DLBCL patients by enrolling two cohorts of patients with matched, comparable clinicopathological parameters. The results demonstrated that metformin use indeed contributes to a significantly improved CR rate and ORR, as well as the PFS. Moreover, a trend of better OS was also observed in the metformin group, although the difference did not reach a statistically significant level, possibly due to the relatively short follow-up time. To further verify the effects of metformin on cholesterol-dependent BCR signaling, we also conducted a preliminary in vivo study, and obtained the results consistent with that of in vitro experiments. Since we employed patients of both cohorts with matched clinicopathological characteristics for the study, and the patients were all under hypoglycemic treatment before receiving biopsy and immunochemotherapy, it’s reasonable to speculate that the more favorable outcome observed in the metformin group could be attributed to the continuous administration of metformin. However, other factors that may lead to potential bias cannot be entirely ruled out. A prospective randomized controlled study is required to further confirm the benefit of metformin on patients with DLBCL.
Conclusions
We find that metformin may block the BCR signaling by inhibiting the biosynthesis of cholesterol. The agent appears to be an effective therapeutic drug against DLBCL, especially in those BCR-dependent cases. Our preliminary work may provide a novel therapeutic strategy for the care of patients with DLBCL. Clinical trials are essential to evaluate the safety, efficacy, and optimal dosing schedule of metformin combined with immunochemotherapy, especially in nondiabetic DLBCL patients.
Data Availability Statement
The raw data supporting the conclusions of this article will be made available by the authors, without undue reservation.
Ethics Statement
The studies involving human participants were reviewed and approved by the Medical ethics committee of Fudan University Shanghai Cancer Center.
Author Contributions
Formal analysis: X-NJ and W-GW, investigation: X-NJ and YZ, methodology: W-GW and YZ, project administration: X-QL, resources: X-QL, software: X-NJ, writing, review and editing: X-NJ and X-QL. All authors contributed to the article and approved the submitted version.
Conflict of Interest
The authors declare that the research was conducted in the absence of any commercial or financial relationships that could be construed as a potential conflict of interest.
References
1. Ikhlas S, Ahmad M. Metformin: Insights Into its Anticancer Potential With Special Reference to AMPK Dependent and Independent Pathways. Life Sci (2017) 185:53–62. doi: 10.1016/j.lfs.2017.07.029
2. Decensi A, Puntoni M, Goodwin P, Cazzaniga M, Gennari A, Bonanni B, et al. Metformin and Cancer Risk in Diabetic Patients: A Systematic Review and Meta-Analysis. Cancer Prev Res (Phila) (2010) 3:1451–61. doi: 10.1158/1940-6207
3. Landman GW, Kleefstra N, van Hateren KJ, Groenier KH, Gans R, Bilo H, et al. Metformin Associated With Lower Cancer Mortality in Type 2 Diabetes: ZODIAC-16. Diabetes Care (2010) 33:322–6. doi: 10.2337/dc09-1380
4. Aljofan M, Riethmacher D. Anticancer Activity of Metformin: A Systematic Review of the Literature. Future Sci OA (2019) 5:FSO410. doi: 10.2144/fsoa-2019-0053
5. Zhao B, Luo J, Yu T, Zhou L, Lv H, Shang P. Anticancer Mechanisms of Metformin: A Review of the Current Evidence. Life Sci (2020) 254:117717. doi: 10.1016/j.lfs.2020.117717
6. Libby G, Donnelly LA, Donnan PT, Alessi DR, Morris AD, Evans JM, et al. New Users of Metformin are at Low Risk of Incident Cancer: A Cohort Study Among People With Type 2 Diabetes. Diabetes Care (2009) 32:1620–5. doi: 10.2337/dc08-2175
7. Chu D, Wu J, Wang K, Zhao M, Wang C, Li L, et al. Effect of Metformin Use on the Risk and Prognosis of Endometrial Cancer: A Systematic Review and Meta-Analysis. BMC Cancer (2018) 18:438. doi: 10.1186/s12885-018-4334-5
8. Chang SH, Luo S, O’Brian KK, Thomas TS, Colditz GA, Carlsson NP, et al. Association Between Metformin Use and Progression of Monoclonal Gammopathy of Undetermined Significance to Multiple Myeloma in US Veterans With Diabetes Mellitus: A Population-Based Retrospective Cohort Study. Lancet Haematol (2015) 2:e30–6. doi: 10.1016/S2352-3026(14)00037-4
9. Mishra AK, Dingli D. Metformin Inhibits IL-6 Signaling by Decreasing IL-6R Expression on Multiple Myeloma Cells. Leukemia (2019) 33:2695–709. doi: 10.1038/s41375-019-0470-4
10. Koo YX, Tan DS, Tan IB, Tai DW, Ha T, Ong WS, et al. Effect of Concomitant Statin, Metformin, or Aspirin on Rituximab Treatment for Diffuse Large B-cell Lymphoma. Leuk Lymphoma (2011) 52:1509–16. doi: 10.3109/10428194.2011.574752
11. Singh AR, Gu JJ, Zhang Q, Torka P, Sundaram S, Mavis C, et al. Metformin Sensitizes Therapeutic Agents and Improves Outcome in Pre-Clinical and Clinical Diffuse Large B-cell Lymphoma. Cancer Metab (2020) 8:10. doi: 10.1186/s40170-020-00213-w
12. Li M, Li X, Zhang H, Lu Y. Molecular Mechanisms of Metformin for Diabetes and Cancer Treatment. Front Physiol (2018a) 9:1039. doi: 10.3389/fphys.2018.01039
13. Mallik R, Chowdhury TA. Metformin in Cancer. Diabetes Res Clin Pract (2018) 143:409–19. doi: 10.1016/j.diabres.2018.05.023
14. Vancura A, Bu P, Bhagwat M, Zeng J, Vancurova I. Metformin as an Anticancer Agent. Trends Pharmacol Sci (2018) 39:867–78. doi: 10.1016/j.tips.2018.07.006
15. Luo Z, Zang M, Guo W. AMPK as a Metabolic Tumor Suppressor: Control of Metabolism and Cell Growth. Future Oncol (2010) 6:457–70. doi: 10.2217/fon.09.174
16. Faubert B, Boily G, Izreig S, Griss T, Samborska B, Dong Z, et al. AMPK is a Negative Regulator of the Warburg Effect and Suppresses Tumor Growth in Vivo. Cell Metab (2013) 17:113–24. doi: 10.1016/j.cmet.2012.12.001
17. Shi WY, Xiao D, Wang L, Dong LH, Yan ZX, Shen ZX, et al. Therapeutic Metformin/AMPK Activation Blocked Lymphoma Cell Growth Via Inhibition of mTOR Pathway and Induction of Autophagy. Cell Death Dis (2012) 3:e275. doi: 10.1038/cddis.2012.13
18. Hardie DG. AMP-Activated Protein Kinase: An Energy Sensor That Regulates All Aspects of Cell Function. Genes Dev (2011) 25:1895–908. doi: 10.1101/gad.17420111
19. Wright GW, Huang DW, Phelan JD, Coulibaly ZA, Roulland S, Young RM, et al. A Probabilistic Classification Tool for Genetic Subtypes of Diffuse Large B Cell Lymphoma With Therapeutic Implications. Cancer Cell (2020) 37:551–68. doi: 10.1016/j.ccell.2020.03.015
20. Schmitz R, Wright GW, Huang DW, Johnson CA, Phelan JD, Wang JQ, et al. Genetics and Pathogenesis of Diffuse Large B-Cell Lymphoma. N Engl J Med (2018) 378:1396–407. doi: 10.1056/NEJMoa1801445
21. Chapuy B, Stewart C, Dunford AJ, Kim J, Kamburov A, Redd RA, et al. Molecular Subtypes of Diffuse Large B Cell Lymphoma Are Associated With Distinct Pathogenic Mechanisms and Outcomes. Nat Med (2018) 24:679–90. doi: 10.1038/s41591-018-0016-8
22. Monti S, Savage KJ, Kutok JL, Feuerhake F, Kurtin P, Mihm M, et al. Molecular Profiling of Diffuse Large B-Cell Lymphoma Identifies Robust Subtypes Including One Characterized by Host Inflammatory Response. Blood (2005) 105:1851–61. doi: 10.1182/blood-2004-07-2947
23. Rickert RC. New Insights Into pre-BCR and BCR Signalling With Relevance to B Cell Malignancies. Nat Rev Immunol (2013) 13:578–91. doi: 10.1038/nri3487
24. Chen L, Monti S, Juszczynski P, Ouyang J, Chapuy B, Neuberg D, et al. SYK Inhibition Modulates Distinct PI3K/AKT- Dependent Survival Pathways and Cholesterol Biosynthesis in Diffuse Large B Cell Lymphomas. Cancer Cell (2013) 23:826–38. doi: 10.1016/j.ccr.2013.05.002
25. Dykstra M, Cherukuri A, Sohn HW, Tzeng SJ, Pierce SK. Location Is Everything: Lipid Rafts and Immune Cell Signaling. Annu Rev Immunol (2003) 21:457–81. doi: 10.1146/annurev.immunol.21.120601.141021
26. Karnell FG, Brezski RJ, King LB, Silverman MA, Monroe JG. Membrane Cholesterol Content Accounts for Developmental Differences in Surface B Cell Receptor Compartmentalization and Signaling. J Biol Chem (2005) 280:25621–8. doi: 10.1074/jbc.M503162200
27. Gupta N, DeFranco AL. Lipid Rafts and B Cell Signaling. Semin Cell Dev Biol (2007) 18:616–26. doi: 10.1016/j.semcdb.2007.07.009
28. Caro P, Kishan AU, Norberg E, Stanley IA, Chapuy B, Ficarro SB, et al. Metabolic Signatures Uncover Distinct Targets in Molecular Subsets of Diffuse Large B Cell Lymphoma. Cancer Cell (2012) 22:547–60. doi: 10.1016/j.ccr.2012.08.014
29. Coutinho R, Clear AJ, Owen A, Wilson A, Matthews J, Lee A, et al. Poor Concordance Among Nine Immunohistochemistry Classifiers of Cell-of-Origin for Diffuse Large B-Cell Lymphoma: Implications for Therapeutic Strategies. Clin Cancer Res (2013) 19:6686–95. doi: 10.1158/1078-0432.CCR-13-1482
Keywords: metformin, cholesterol, diffuse large B-cell lymphoma, B-cell receptor, type II diabetes
Citation: Jiang X-N, Zhang Y, Wang W-G, Sheng D, Zhou X-Y and Li X-Q (2021) Alteration of Cholesterol Metabolism by Metformin Is Associated With Improved Outcome in Type II Diabetic Patients With Diffuse Large B-Cell Lymphoma. Front. Oncol. 11:608238. doi: 10.3389/fonc.2021.608238
Received: 19 September 2020; Accepted: 20 April 2021;
Published: 14 June 2021.
Edited by:
Shimin Hu, University of Texas MD Anderson Cancer Center, United StatesReviewed by:
Habibe Kurt, Brown University, United StatesRichard Eric Davis, University of Texas MD Anderson Cancer Center, United States
Zhihong Hu, University of Texas Health Science Center at Houston, United States
Copyright © 2021 Jiang, Zhang, Wang, Sheng, Zhou and Li. This is an open-access article distributed under the terms of the Creative Commons Attribution License (CC BY). The use, distribution or reproduction in other forums is permitted, provided the original author(s) and the copyright owner(s) are credited and that the original publication in this journal is cited, in accordance with accepted academic practice. No use, distribution or reproduction is permitted which does not comply with these terms.
*Correspondence: Xiao-Qiu Li, bGVleGlhb3FpdUBob3RtYWlsLmNvbQ==
†These authors have contributed equally to this work