- 1Department of Clinical Medicine, North Sichuan Medical College, Nanchong, China
- 2Department of Urology, Affiliated Hospital of North Sichuan Medical College, Nanchong, China
- 3Department of Urology, First Affiliated Hospital of Chongqing Medical University, Chongqing, China
- 4Center for Immunology Research, Chongqing Medical University, Chongqing, China
- 5Department of Urology, Fuling Center Hospital of Chongqing, Chongqing, China
- 6Key Laboratory of Diagnostics Medicine Designated by the Ministry of Education, Chongqing Medical University, Chongqing, China
The treatment of castration-resistant prostate cancer (CRPC) remains challenging due to the failure of androgen deprivation therapy (ADT); hence the search for other molecular therapeutic targets besides androgen receptor signaling is ongoing. This study systematically investigated the expression of SOX17 and Notch receptors in CRPC tissues and cells in vitro, showing that consistent clinical CRPC, SOX17/Notch1, and Notch4 were responsible for enzalutamide resistance in CRPC cells. The γ secretase inhibitors, BMS-708163, GSI-IX, PF-3084014, and RO4929097 abrogated the enzalutamide resistance by inhibiting Notch1 or/and Notch4 in vitro, with GSI-IX and RO4929097 being more effective than BMS-708163 and PF-3084014 in reliving bone metastasis in vivo. In conclusion, the Notch1 and Notch4 inhibitors GSI-IX and RO4929097 are promising therapeutic agents for the treatment of CRPC.
Introduction
Prostate cancer (PCa) is the second leading cause of cancer-related deaths, accounting for 33,330 deaths in the United States (1). Its mortality rate is also increasing at a rate of 5.5% per year from 2000 to 2011 in China (2). Androgen deprivation therapy (ADT), such as abiraterone, enzalutamide (mainly bicalutamide in China) is the first-line treatment strategy for advanced prostate cancer; however, this relief is temporary and castration-resistant prostate cancer (CRPC) occurs within a few years (3, 4). Chemotherapeutic agents such as docetaxel and cabazitaxel are widely used for CRPC but due to their poor therapeutic effects (5, 6) new-generation drugs, such as abiraterone and apalutamide, have been developed. However, the benefit for CRPC patients is very limited (7–10); hence, the search continues for other molecular targets to treat CRPC.
The signaling pathways Wnt/β-catenin (11–14), Hedgehog (15), mTOR/PI3K/AKT (16, 17), and the Notch signaling pathway (18–20) are thought to be involved in the occurrence and development of CRPC. The Notch signaling pathway consists of Notch receptors (Notch1–4), ligands, and downstream target genes (21), and is recognized as an oncogene in various tumors including PCa (22–24). Notch1 signaling is overexpressed in enzalutamide-resistant cells, with inhibition of Notch1 signaling restoring enzalutamide function (25). Also, the downregulation of Notch3 enhances the efficacy of ADT for PCa (20), but there has been no systematic evaluation of the role of the Notch receptors in the drug resistance of CRPC models.
SOX17 (SRY-box containing gene 17) is homologous to the sex-determining gene SRY (26) and a tumor suppressor in various cancers (27–29). On the other hand, SOX17 promotes tumor angiogenesis and destabilizes tumor vessels in Lewis lung cancer, resulting in tumor metastasis and resistance to cisplatin (30). Overexpression of SOX17 initiates and accelerates tumorigenesis (31), but little is known about the role of SOX17 in PCa including CRPC models.
This study investigated the expression of SOX17, Notch receptors 1–4 in prostate cancer and CRPC tissue samples, and enzalutamide-resistant LNCaP cells (Enza-R). Downregulation of Notch receptors was associated with the sensitivity of Enza-R cells to enzalutamide; hence we systematically evaluated the effects of Notch inhibitors on the restoration of enzalutamide sensitivity both in vitro and in vivo. Some findings of this study may provide a novel treatment approach for patients with CRPC.
Materials and Methods
Patient and Tissue Samples
Thirty benign prostatic hyperplasia (BPH) and 36 prostate samples were collected at the First Affiliated Hospital of Chongqing Medical University, Chongqing, China, from September 2018 to April 2019. Thirty-three CRPC tissues, including paraffin and frozen tissue samples, were obtained from the First Affiliated Hospital of Chongqing Medical University, Chongqing, China (25 cases) and the Fuling Central Hospital, Chongqing, China (eight cases) from May 2008 to October 2018. All PCa and CRPC tissues were confirmed by a pathologist. Informed consent was obtained from the patients or their family members and this study was approved by the Ethics Committee of Chongqing Medical University, the Ethics Committee of Fuling Central Hospital, and complied with the Helsinki Declaration.
Immunohistochemistry
All the embedded PCa and CRPC samples were cut into 5-µm-thick sections. The immunoreactivities of SOX17, Notch1, Notch2, Notch3, Notch4 were detected by an immunoperoxidase staining procedure with the primary antibodies (anti-SOX17, 1:200, Abcam, cat.no.ab192453; anti-Notch1, 1:200, Abcam, cat. no.ab8925; anti-Notch2, 1:200, Cell Signaling Technology, cat.no. D76A6; anti-Notch3, 1:200, Abcam, cat.no. ab23426; anti-Notch4, 1:200, Santa Cruz Biotechnology, cat.no. sc-377399 ). Staining scoring, according to staining intensity, was defined as 0, no staining; 1, weak staining; 2, light staining; 3, moderate staining; and 4, strong staining. Staining scores of ≤1 were defined as negative expression, while staining scores of ≥2 were defined as positive expression.
Cell Culture
RWPE-1, LNCaP, and DU145 cells were cultured in RPMI-1640 containing 10% fetal bovine serum (FBS) and 1% penicillin/streptomycin. To induce enzalutamide-resistant cells (Enza-R), LNCaP cells were treated with 10 µM enzalutamide (Selleck, USA) for at least 6 months (32), and then 1 × 105 Enza-R cells were seeded into six‐well plates. The lentivirus (Shanghai Gene Pharma Company, China) containing LV‐NC or LV‐shNotch1, LV-shNotch2, LV-shNotch3, and LV-shNotch4, was added to the culture medium for 8 h, then cells were treated with 1 μg/ml puromycin and incubated for 72 h to generate Notch1–4‐silenced stable drug resistance cells.
CCK8 Assay
The cell counting kit-8 (CCK-8) assay was used to assess cell viability. The cells (2,000 cells/well) were seeded into 96-well plates, then 10 µl CCK-8 reagent (Solarbio, Beijing, China) was added to each well, and the optical density was evaluated using a microplate reader (Bio-Rad Laboratories, CA, USA). For the half-maximal inhibitory concentration (IC50) of enzalutamide, after pretreatment with various agents, such as LV-NC and LV-shNotch1, the LNCaP and Enza-R were transplanted into 96-well plates and cultured with various concentrations of enzalutamide for 24 h using DMSO (Sigma, USA) as control.
Western Blotting
Protein samples (50 µg) were transferred to PVDF membranes. After blocking with 5% non-fat milk for 2 h at room temperature, the membranes were incubated with primary antibodies overnight at 4°C, anti-E-Cadherin (1:2,000, Cell Signaling Technology, cat.no.14472), anti-N-cadherin (1:2,000, Cell Signaling Technology, cat.no.13116s), anti-Vimentin (1:1,000, Cell Signaling Technology, cat. no.5741s), anti-Zeb-1(1:2,000, Cell Signaling Technology, cat.no.70512s), anti-AR (1:2,000, Cell Signaling Technology, cat.no.19672s), anti-SOX17 (1:2,000, Abcam), anti-Notch1(1:2000, Abcam); anti-Notch2 (1:2,000, CST); anti-Notch3 (1:1,000, Abcam); anti-Notch4 (1:1000, Santa Cruze), and anti-GAPDH (1:1,000, CST, cat. no.5174s) was used as a loading control. The intensity of the protein bands was determined using Image-Pro plus 6.0.
Immunofluorescence
After cultured with various treatments, the cells were seeded into a 12-well plate and incubated for 24 h, fixed in 4% paraformaldehyde for 20 min, and incubated with various primary antibodies for 1 h in dark room,(anti-Notch1 : 1:00, anti-Notch2: 1:100, anti-Notch3: 1:100, anti-Notch4: 1:100, anti-SOX17:1:150, anti-AR: 1:100). The cells were treated with secondary antibody (Zhongshan Golden Bridge Biotechnology, Beijing, China). DAPI (Zhongshan Golden Bridge Biotechnology) was used for nuclear staining.
Reverse transcription-quantitative PCR
The Taq™ II kit (Takara, Japan) was used for RT-qPCR on a CFX96™ Real-Time PCR Detection System (Bio-Rad, Hercules, CA, USA). The primer sequences were as follows: Notch1 sense, 5-GAACGGGGCUAACAAAGAUTT-3′, antisense, 5′-AUCUUUGUUAGCCCCGUUCTT-3′; Notch2 sense, 5′-TCAACTGCCAAGCGGATGT-3′; antisense, 5′-CTTGGCTGCTTCATAGCTCC-3′; Notch3 sense, 5′-GCTCAACGGCACTGATCCT-3′, antisense, 5′-AGCCCAGTGTAAGGCTGATT-3′; Notch4 sense, 5′-GGAGACTGC AGACCAGAAGG-3′, antisense, 5′-GACCCTCAGAGTCAGGGACA-3′, AR sense, 5′-TTCCCTCCCTATCTAACCCTC-3′, antisense: 5′-TCTAAACTTCCCGTGGCATAA-3′; SOX17 sense, 5′-ATCCTCAGACTCCTGGGTTT-3′, antisense, 5′-ACTGTTCAAGTGGCAGACAAA-3′.
Xenograft and Bone Metastasis Model
This study was approved by the Ethics Committee of Chongqing Medical University and performed according to the Guidelines on Animal Experimentation. Enza-R cells (3 × 108) were subcutaneously injected into the left flank or the right tibia of nude mice with surgical castration. After two weeks, the mice with xenograft tumors were treated with enzalutamide and various Notch receptor inhibitors by intraperitoneal injection twice per week. The xenograft tumors in the left flank were evaluated every 5 days. The bone metastasis was harvested after eight weeks, and the xenograft tumors were harvested after four weeks. The tumor-volume (mm3) was calculated according to the following formula: volume (mm3) = 1/2 × length × width.
Statistical Analysis
SPSS 19.0 was used for statistical analyses. All data were presented as mean ± SD and analyzed using Kaplan–Meier survival analysis, a stratified log-rank test, Multivariable analyses with the Cox proportional-hazards models, the χ2 test, one-way ANOVA, two-way ANOVA, the Student’s t-test, Spearman’s correlation analysis, and the Mann–Whitney test. A P-value <0.05 was considered statistically significant.
Results
The Expression of SOX17 and Notch Receptors Was Up-Regulated in CRPC Tissue Samples
The expression of SOX17 in CRPC tissues (64%, 21/33) was significantly higher than in prostate cancer tissues (42%, 15/36) (Figures 1A, G, Table 1). SOX17 was also detected in the tumor thrombus of CRPC suggesting that activated SOX17 may be associated with tumor metastasis (Figure 1F). Similarly, the expression of Notch1 and Notch4 was increased in CRPC tissues compared to prostate cancer tissues (Figures 1B, E, H, K), but there was no significant difference in the expression of Notch2 and Notch3 (Figures 1C, D, I, J). Furthermore, the up-regulation of SOX17 was positively correlated with the expression of Notch1 (r = 0.327, P = 0.032) and Notch2 (r = 0.448, P = 0.004), Notch4 (r = 0.328, P = 0.031) (Figures 1L–O).
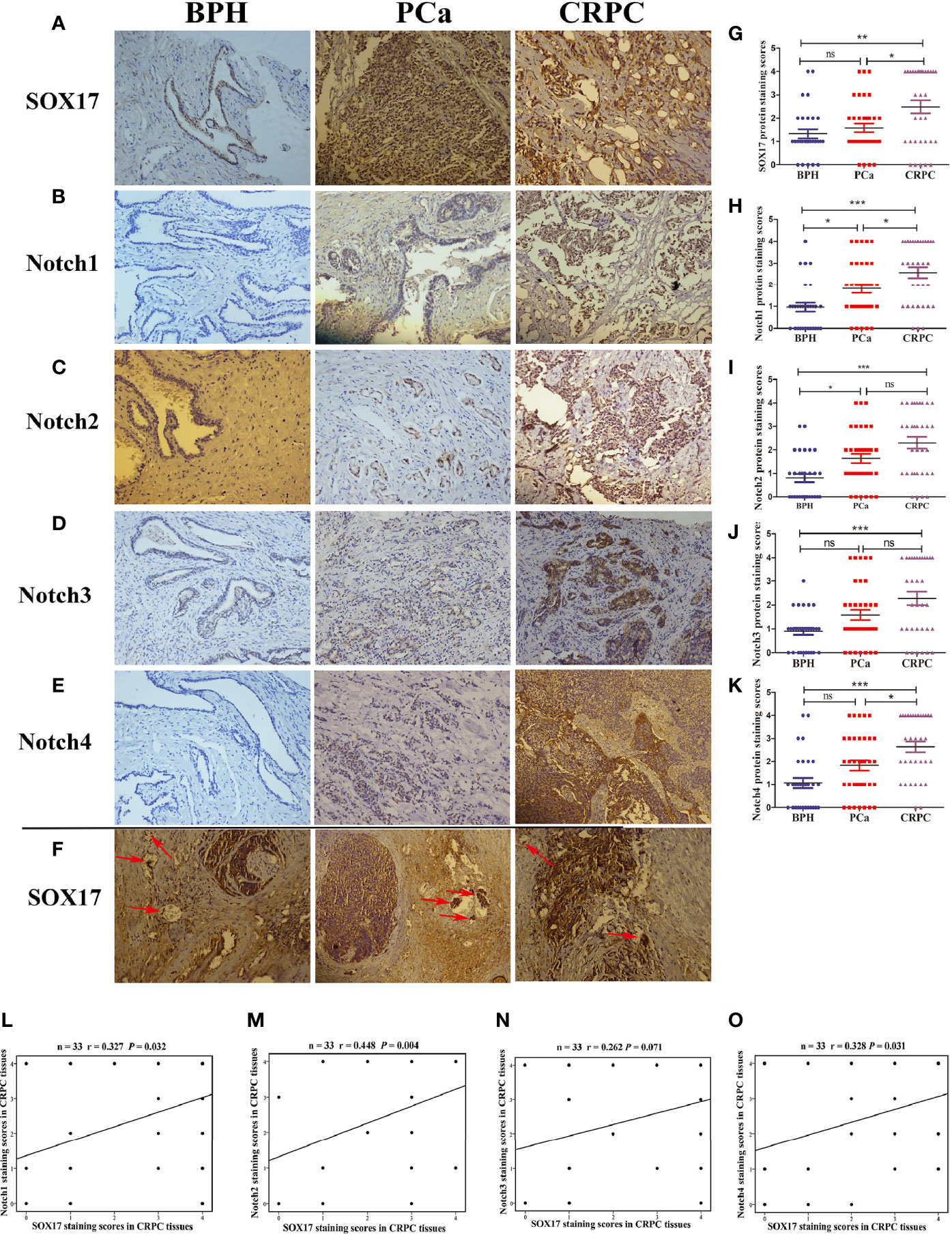
Figure 1 The expression of SOX17 and Notch receptors in samples of benign prostatic hyperplasia (BPH), prostate cancer (PCa) and castration-resistant prostate cancer (CRPC). (A–E) The expression levels of SOX17, Notch1 and Notch2, Notch3, Notch4 were detected by using immunohistochemistry (×200). (F) The positive expression of SOX17 in cancer thrombus of CRPC (red arrows) (×200). (G–K) Average staining scores for SOX17, Notch1, Notch2, Notch3, Notch4 BPH, PPC and CRPC samples. Staining scoring, according to staining intensity, was defined as 0, no staining; 1, weak staining; 2, light staining; 3, moderate staining; and 4, strong staining. Staining scores of ≤1 were defined as negative expression, while staining scores of ≥2 were defined as positive expression. (L–O) The correlation curve analysis for SOX17 staining scores versus Notch1 and Notch2, Notch3, Notch4 staining scores in CRPC tissues. Values of P < 0.05 were considered to be statistically significant. *P < 0.05, **P < 0.01, ***P < 0.001, ns, no significance.
Next, we determined the association between the expression of SOX17, Notch1, Notch2, Notch3, Notch4, and the key clinical characteristics of PCa and CRPC patients (Table 1). The expression of SOX17 and Notch 4 in PCa patients was significantly associated with a higher PSA. Furthermore, Notch1 (P = 0.01) and Notch4 (P = 0.023) positivity in PCa was associated with a higher Gleason score, while all Notch receptors positive in CRPC were associated with a higher Gleason score. SOX17 up-regulation was associated with bone metastases in both prostate cancer (P = 0.014) and CRPC (P = 0.036) tissues.
The Expression of SOX17, Notch1, and Notch2 Was Associated With a Short Progression-Free Survival
Kaplan–Meier survival analysis was performed to evaluate the relationship between PFS and SOX17, Notch1, Notch2, Notch3, Notch4 in CRPC, revealing that the median PFS was 21 months in CRPC patients positive for SOX17, 36.5 months in SOX17-negative patients (Figure 2A, P = 0.0315). Moreover, the patients positive for Notch1 had a significantly shorter PFS than Notch1 negative patients (23 vs 38 months, P = 0.0352) (Figure 2B). In addition, the median PFS in Notch2-positive CRPC patients was shorter than Notch2-negative patients (24 vs 34.5 months, P = 0.0403) (Figure 2C). However, the median PFS in patients with Notch3-positive or Notch4-positive was not significantly different with Notch3-negative or Notch4-negative patients (Figures 2D, E).
Moreover, multivariable analyses with the Cox proportional-hazards models were performed to estimate the simultaneous effects of prognostic factors on PFS of CRPC patients. As shown in Table 2, expression of SOX17 (HR = 4.94, P = 0.002), Notch1 (HR = 2.85, P = 0.044) was linked to a poor prognosis in PFS of CRPC patients (Table 2).
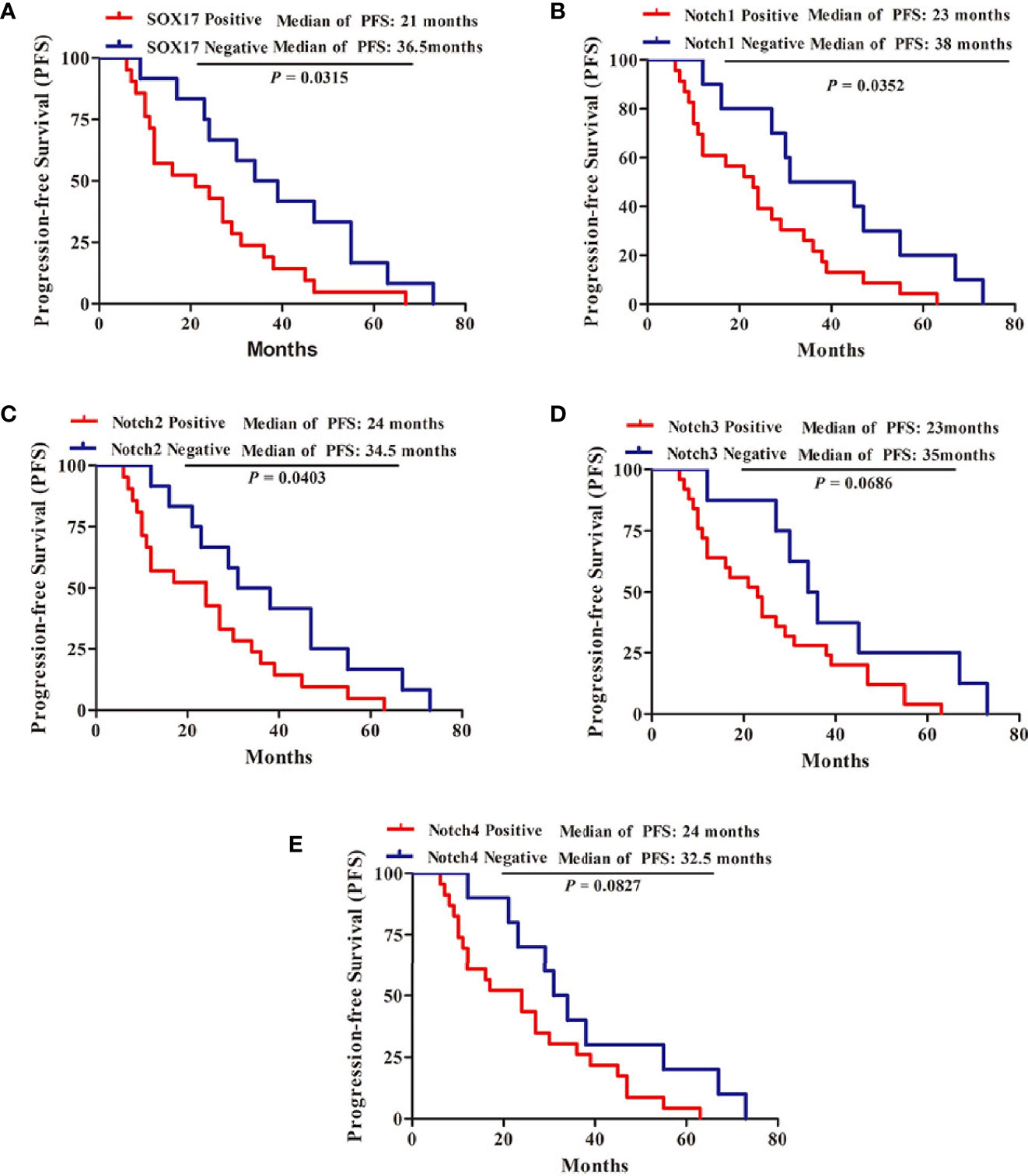
Figure 2 Kaplan-Meier survival analysis for the progression-free survival (PFS) of 33 patients with CRPC. (A) 21 patients with SOX17-positive, 12 patients with SOX17-negative, (B) 23 patients with Notch1-positive, 10 patients with Notch1-negative, (C) 21 patients with Notch2-positive, 12 patients with Notch2-negative, (D) 25 patients with Notch3-positive, eight patients with Notch3-negative, (E) 23 patients with Notch4-positive, 10 patients with Notch4-negative. P < 0.05 was considered statistically significant.
The Expression of SOX17 and Notch Receptors Was Up-Regulated in Enza-R Cells, and Knockdown Decreased Enza-R Cell Viability
To determine the possible role of SOX17 and Notch receptors in CRPC models, enzalutamide-resistant LNCaP (Enza-R) cells were constructed by continuously treating LNCaP with enzalutamide for at least 6 months. As shown in Figure 3A, the enzalutamide resistance of Enza-R cells increased 100-fold compared with LNCaP cells. Also, as expected, the mRNA and protein expression of SOX17, Notch1, Notch3, and Notch4 was up-regulated in Enza-R cells compared with the parental cells (Figures 3B–D). To further explore the role of SOX17 and Notch receptors in Enza-R cells, their expression was knocked down using lentivirus, resulting in the significant suppression of cell proliferation (Figures 3E–I). Surprisingly, knockdown of SOX17 reversed enzalutamide resistance by nearly six-fold, suggesting that dysregulation of SOX17 is responsible for enzalutamide resistance (Figure 3J).
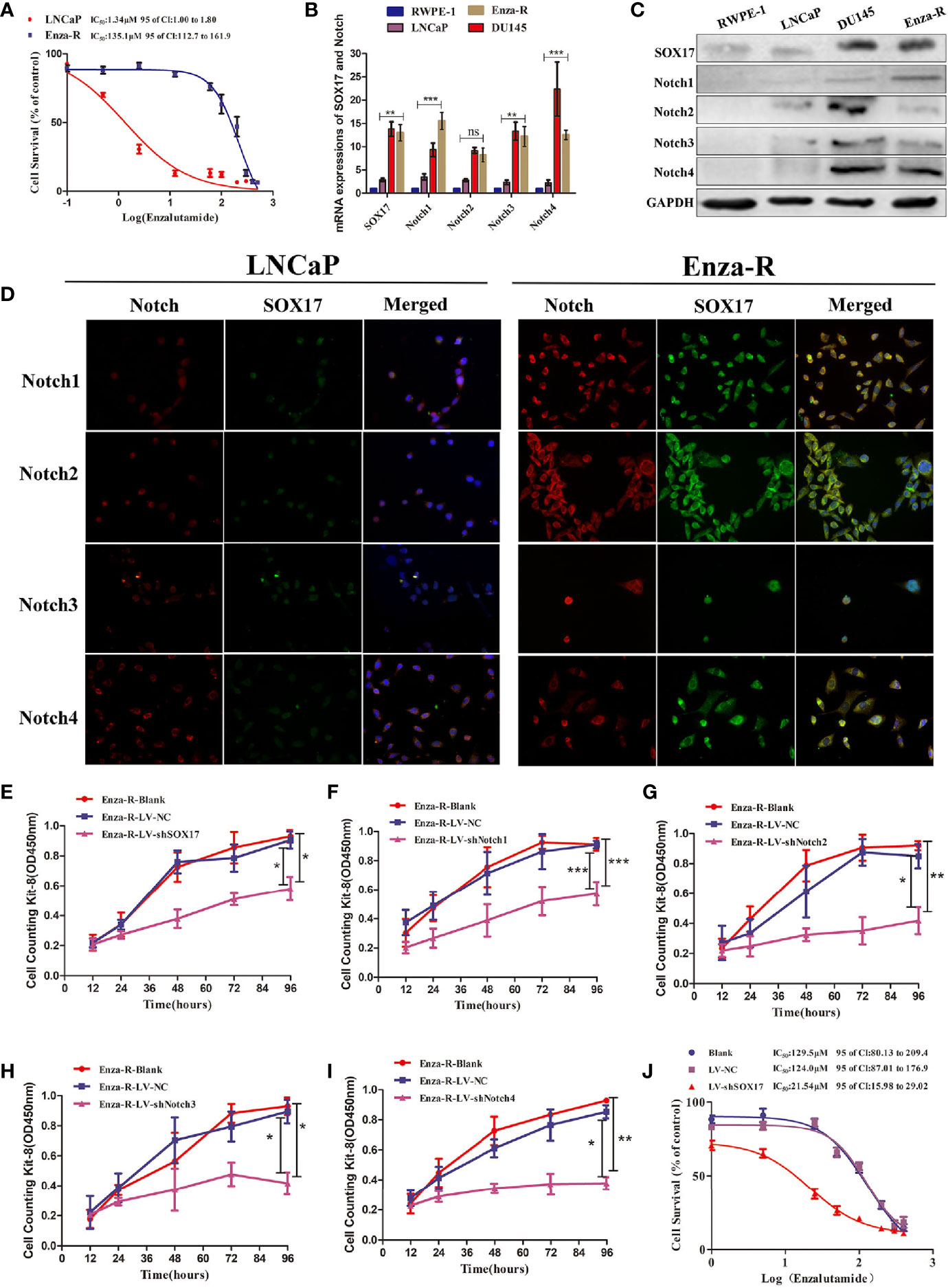
Figure 3 Expression of SOX17 and Notch receptors was detected in Enza-R cells and knocking down them inhibited the viability of Enza-R cells. (A) Identification for CRPC cells, both LNCaP and Enza-R cells were treated with increasing concentrations of enzalutamide for 24 h and IC50 was detected by cell counting kit-8 (CCK-8) assay. (B–D) Expression of SOX17, Notch receptors in both mRNA and protein level was detected by RT-PCR, Western blot and Immunofluorescence assay (magnification, ×200). (E–I) After incubated for 96 h, the viability of Enza-R cells, treated with shSOX17, shNotch1, shNotch2, shNotch3, and shNotch4 respectively, was evaluated by CCK-8 assay. (J) Enza-R cells, infected with LV-NC or LV-shSOX17, were treated with increasing concentrations of enzalutamide for 24 h, and the half maximal inhibitory concentration (IC50) was determined by CCK-8 assay. Enza-R: enzalutamide-resistant LNCaP cells. *P < 0.05, **P < 0.01, ***P < 0.001, ns, no significance.
Knockdown of SOX17 Decreased the Expression of AR and Notch Receptors
SOX17 was expressed in the tumor thrombus of CRPC (Figure 1F); thus, it was hypothesized that decreasing SOX17 expression inhibits metastasis. The analysis of the expression of epithelial-mesenchymal transition (EMT) proteins in Enza-R cells revealed that the knockdown of SOX17 was associated with the up-regulation of E-cadherin and the downregulation of N-cadherin, Vimentin, and Zeb-1, indicating that the expression of SOX17 may promote metastasis of CRPC models (Figure 4A).
As mentioned above, knockdown of SOX17 restores enzalutamide sensitivity in Enza-R cells. Moreover, it is well known that amplification of AR plays a key role in CRPC; hence, it was hypothesized that SOX17knockdown reverses the resistance by down-regulating AR activity. The mRNA and protein expression of AR decreased in Enza-R and DU145 when SOX17 was knocked down (Figures 4B–D). Also, the high expression of SOX17 was positively correlated with Notch1, Notch2, and Notch4 in CRPC tissues (Figures 1L–O), so all Notch receptors were detected in SOX17 knockdown Enza-R cells. Reducing SOX17 expression also significantly down-regulated the mRNA expression of Notch1 and Notch4, as well as the protein expression of all Notch receptors, suggesting that SOX17 positively regulates the Notch signaling pathway (Figures 4E–G).
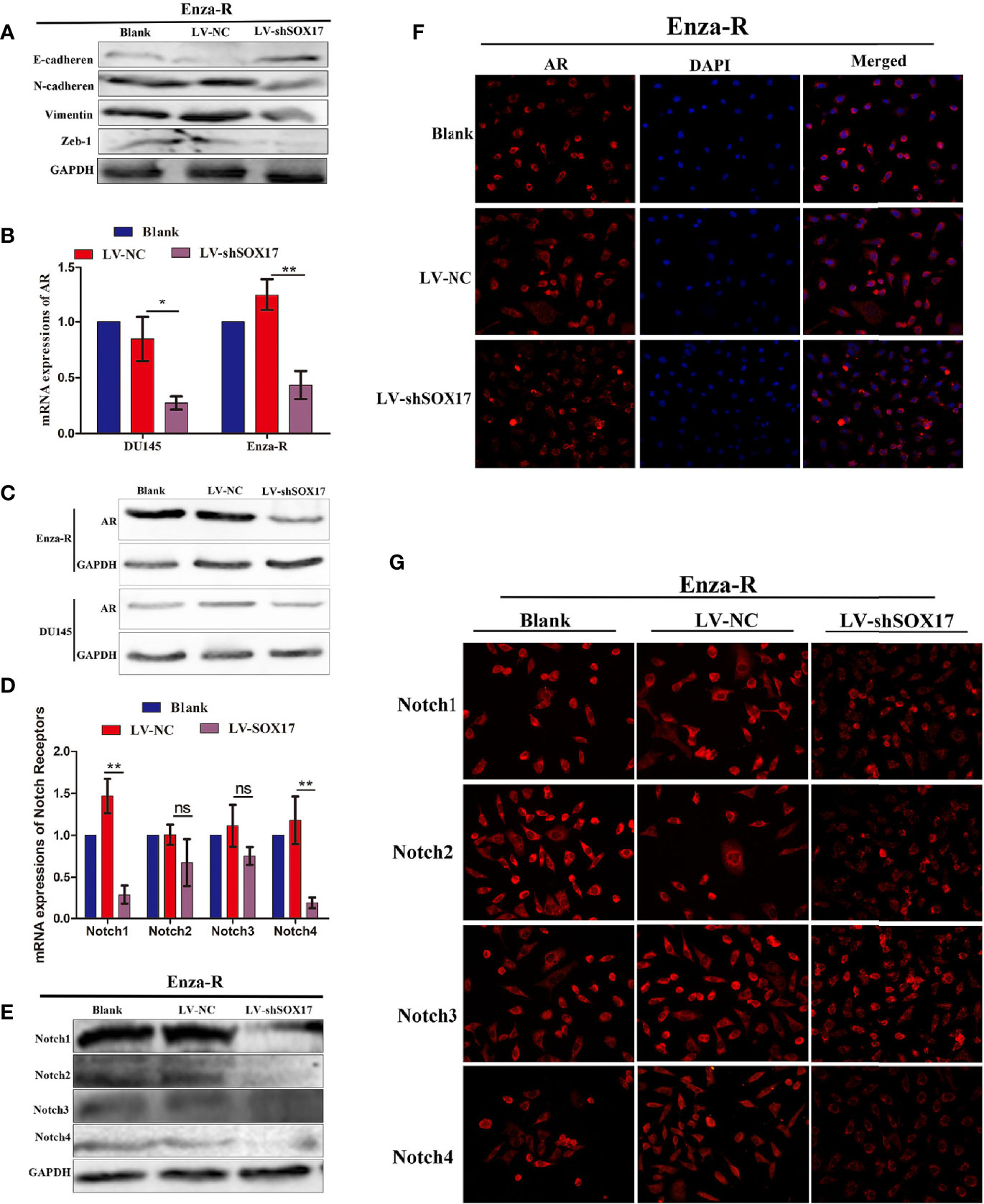
Figure 4 Knocking down SOX17 reducing the expression of EMT related proteins, AR, Notch receptor members. (A) Expression of E-cadherin, N-cadherin, Vimentin and Zeb-1 in Enza-R cells, infected with LV-NC or LV-shSOX17, was examined by Western blot assay. GAPDH served as a loading control. (B–D) The expression of AR in Enza-R or/and DU145 cells, infected with LV-NC or LV-shSOX17, was evaluated by using RT-PCR, Western blot and Immunofluorescence (magnification, ×200) assay. GAPDH served as a loading control. (E–G) Expression of Notch1, Notch2, Notch3, Notch4 were investigated by using RT-PCR, Western blot and Immunofluorescence (magnification, ×200) assay in Enza-R cells treated with LV‐NC or LV‐shSOX17, *P < 0.05, **P < 0.01, ns, no significance; AR, androgen receptor; Enza-R, enzalutamide-resistant LNCaP cells.
The Knockdown of Notch1 and Notch 4 Partially Restored the Sensitivity of Enza-R Cells to Enzalutamide
The down-regulation of SOX17 decreased the expression of AR, reversed enzalutamide resistance in Enza-R cells (Figures 3J, 4B–D), and inhibited the expression of Notch receptors (Figures 4E–G); thus, it was hypothesized that the down-regulation of Notch receptors also reverses enzalutamide resistance by decreasing AR activity. As shown in Figures 5A–C, knockdown of Notch1 and Notch4 significantly inhibited AR expression, however, the inhibition did not be detected in Enza-R cells knocking down Notch2, Notch3. Next, we determined the IC50 values of enzalutamide for Enza-R cells knocking down all Notch receptors respectively. Down-regulation of Notch1 and Notch4 increased the sensitivity of the Enza-Rcells to enzalutamide by 3.3-fold, 4.7-fold, respectively (Figures 5D, G). These results indicate that dysregulation of Notch1 and Notch4 but not Notch2 and Notch3 is responsible for enzalutamide resistance in Enza-R cells.
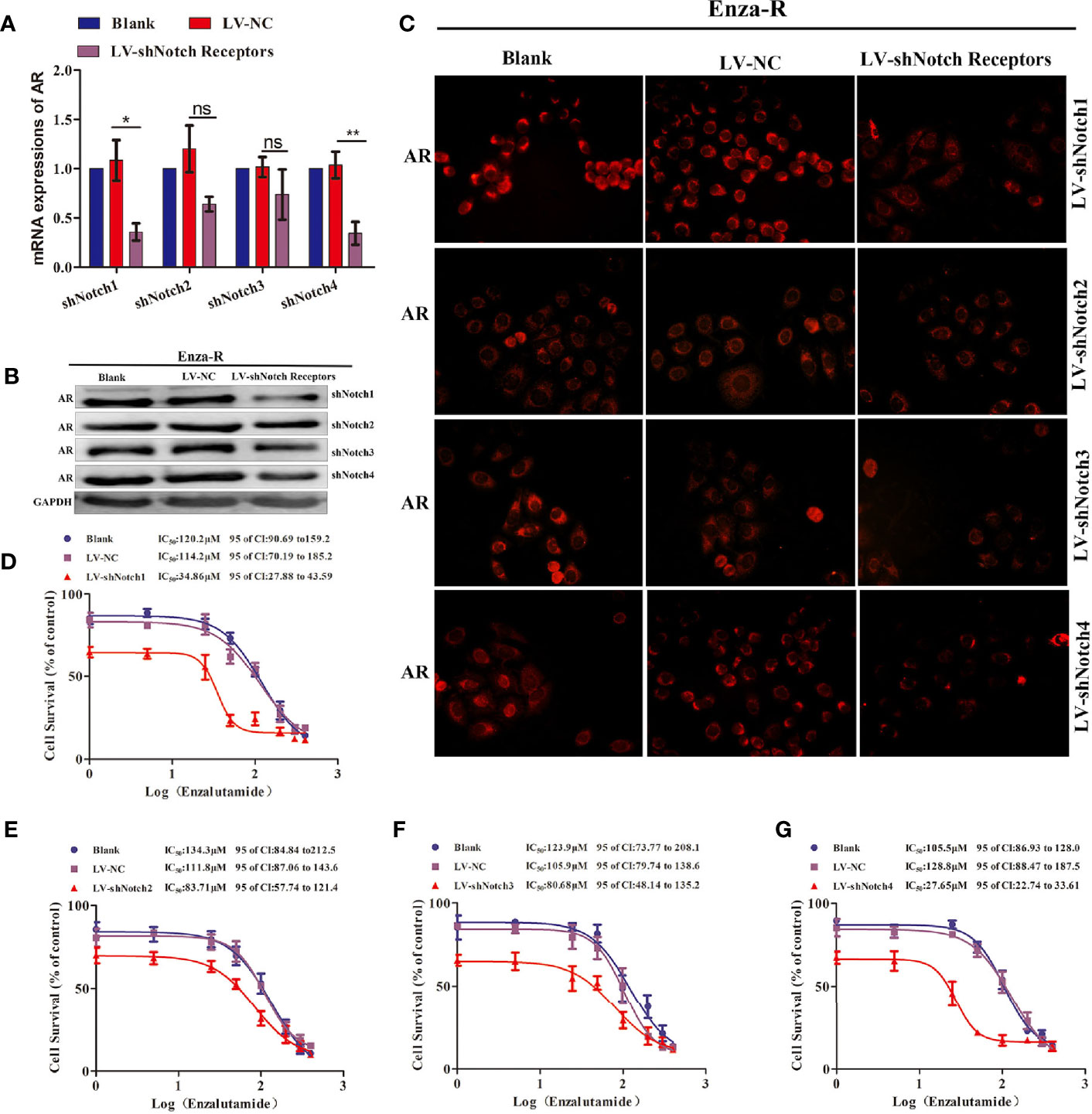
Figure 5 Knocking down Notch receptors inhibited the expression of AR and reversed the enzalutamide resistance in Enza-R cells. (A–C) Expression of AR in Enza-R cells infected with LV-shNotch1, LV-shNotch2, LV-shNotch3, LV-shNotch4 were respectively determined by using RT-PCR, Western blot and Immunofluorescence (magnification, ×200) assay. *P < 0.05, **P < 0.01, ns, no significance. (B–G) The half maximal inhibitory concentration (IC50) was determined by CCK-8 assay after the cells were treated with increasing concentrations of enzalutamide for 24 h. Enza-R cells were infected with LV-NC or LV-shNotch1, LV-shNotch2, LV-shNotch3, LV-shNotch4 respectively. AR, androgen receptor; Enza-R, enzalutamide-resistant LNCaP cells.
γ-Secretase Inhibitors Reversed Enzalutamide Resistance by Decreasing Notch1 and NOTCH4 activity
The γ-secretase complex cleaves Notch receptors into the Notch extracellular domain (NECD) and Notch intracellular domain (NICD), which are transported from the cell membrane to the nucleus and known as “activated Notch” (33–35). It has been reported that γ-secretase inhibitors, such as DAPT and PF-3085014, inhibit malignant biological behavior and reverse ADT-resistance in PCa including CRPC models (18–20, 25, 32). There are many γ-secretase inhibitors on the market, some of which have shown good curative effects on various tumors (36–38); however, their effects on PCa, including CRPC models are still unclear. Moreover, a comprehensive comparison of the effectiveness of these inhibitors on the drug resistance for CRPC models has yet been performed. Thus, seven γ-secretase inhibitors were used to decrease Notch signaling (37) in Enza-R cells. Our data revealed that BMS-708163, GSI-IX, PF-3084014, and RO4929097 restored the sensitivity of Enza-R cells to enzalutamide 7.0-fold, 3.8-fold, 3.3-fold, 5.8-fold. However, we failed to find any effects of LY3039478, LY450139, YO01027 on reversing the drug resistance. (Figures 6A–C).
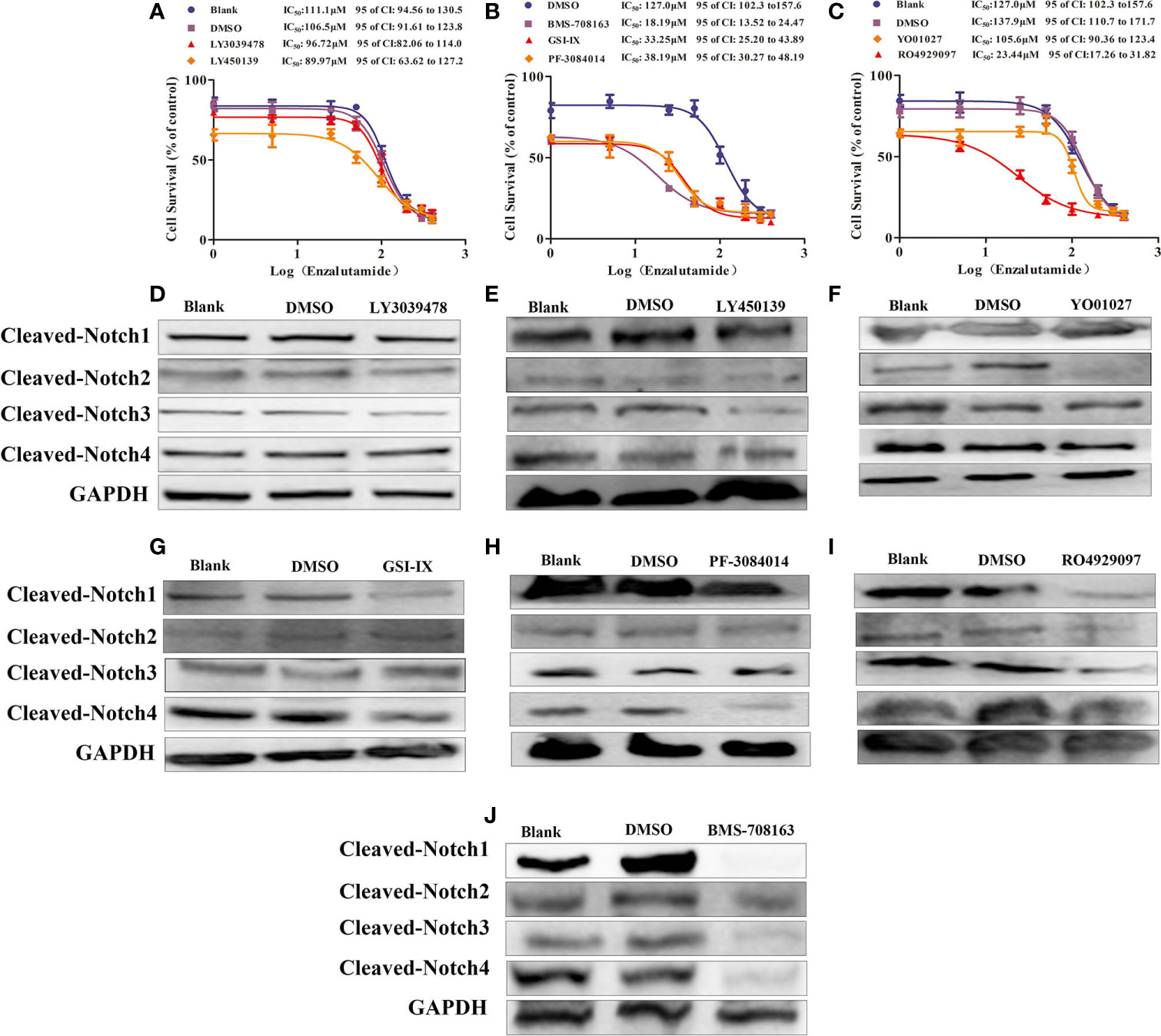
Figure 6 γ-secretase inhibitors reversed the resistance by decreasing activities of Notch receptors. (A–C) The half maximal inhibitory concentration (IC50) was determined by CCK-8 assay after the Enza-R cells were treated with enzalutamide and γ-secretase inhibitors, LY3039478, BMS-708163, LY-450139, GSI-IX, PF-3084014, RO4929097, LY01027 respectively for 24 h. (D–J) Activities of each Notch receptor member were determined in Enza-R cells after treated them with various γ-secretase inhibitors respectively. The cleaved Notch 1–4 means the intracellular domain part of Notch receptor members spliced by γ-secretase, which realizes the function of notch receptor members. GAPDH served as a loading control.
PF-3084014 and DAPT enhance the anti-tumor effects of ADT in PCa reversing enzalutamide resistance in CRPC models by decreasing Notch1 activity (18, 25). However, it is still unknown whether these inhibitors reverse the drug resistance by decreasing other Notch receptors besides Notch1. Our data revealed that knockdown of Notch1 and Notch4 but not Notch2 and Notch3 sensitized Enza-R cells to enzalutamide. Also, the BMS-708163, GSI-IX, PF-3084014, and RO4929097 inhibitors affected drug resistance, while others, such as LY3039478 and LY450139 had no effect (Figures 6A–C). Thus, we speculated that each γ-secretase inhibitor down-regulates one activated Notch receptors, some of which are responsible for the resistance. Western blotting was performed to detect the expression of the activated Notch receptors after the treatment of Enza-R cells with various γ-secretase inhibitors. LY3039478, which did not reverse enzalutamide resistance, was unable to decrease any activated Notch receptors in Enza-R cells (Figure 6D, Table 3). LY450139 down-regulated activated Notch3 but not Notch1, 2, or 4, and failed to reverse enzalutamide resistance (Figure 6E, Table 3). Although YO01027 inhibited the expression of activated Notch2, it had no therapeutic potential for drug resistance (Figure 6F, Table 3). Importantly, BMS-708163, by down-regulating activated Notch1, Notch2, Notch3, and Notch4, increased the sensitivity of Enza-R cells to enzalutamide (7.0-fold), GSI-IX, by down-regulating activated Notch1 and Notch4, increased the sensitivity by 3.8-fold, PF-3084014 decreased activated Notch1 and Notch4 restoring the sensitivity of Enza-R by 3.3-fold and RO4929097 inhibited activated Notch1, Notch2, and Notch3 enhancing the sensitivity by 5.8-fold. Taken together, the knockdown of Notch1 or/and Notch4 by lentivirus (Figures 5E, F) and down-regulation of activated Notch1 or/and Notch4 by γ-secretase inhibitors (Figures 6G–J, Table 3) restored the sensitivity of Enza-R cells to enzalutamide, further suggesting that over-activated Notch1 and Notch4 are responsible for enzalutamide resistance in Enza-R cells.
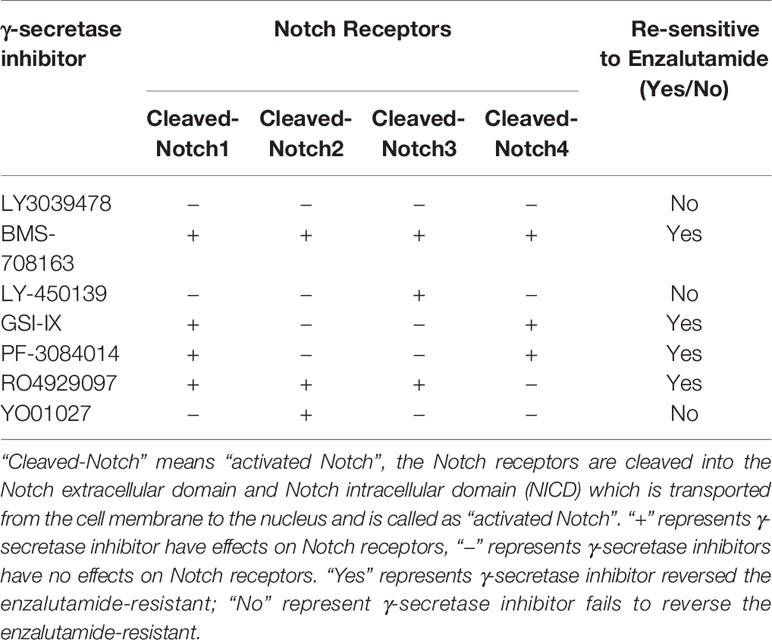
Table 3 The effectiveness of γ-secretase inhibitors on reversing enzalutamide resistance by inhibiting activities of Notch receptors.
Combination Therapy of Enzalutamide and γ-Secretase Inhibitors Blocks the Growth and Bone Metastasis of Enza-R Cells In Vivo
The therapeutic potential of γ-secretase inhibitors, BMS-708163, GSI-IX, PF-3084014, and RO4929097, was evaluated in vivo. A xenograft tumor was constructed by treating castrated nude mice with a combination of enzalutamide and γ-secretase inhibitors. As expected, compared to the control group, BMS-708163, GSI-IX, PF-3084014, and RO4929097 significantly inhibited tumor growth (Figures 7A–D). Next, we subcutaneously injected Enza-R cells into the right tibia to investigate the therapeutic potential of the four γ-secretase inhibitors for bone metastasis. The mice were treated with a combination of enzalutamide and each γ-secretase inhibitor by injection into the tail vein, with X-ray and H&E assays performed to evaluate bone destruction. GSI-IX, RO4929097, BMS-708163, a+nd PF-3084014 relieved the bone damage caused by Enza-R cells (Figure 7E). Importantly, our data showed that GSI-IX and RO4929097 were more effective than BMS-708163 and PF-3084014 in reducing bone metastasis (Figures 7F–G). This systematic investigation of γ-secretase inhibitors indicates that GSI-IX and RO4929097 have the potential for the synergistic treatment of CRPC models with enzalutamide.
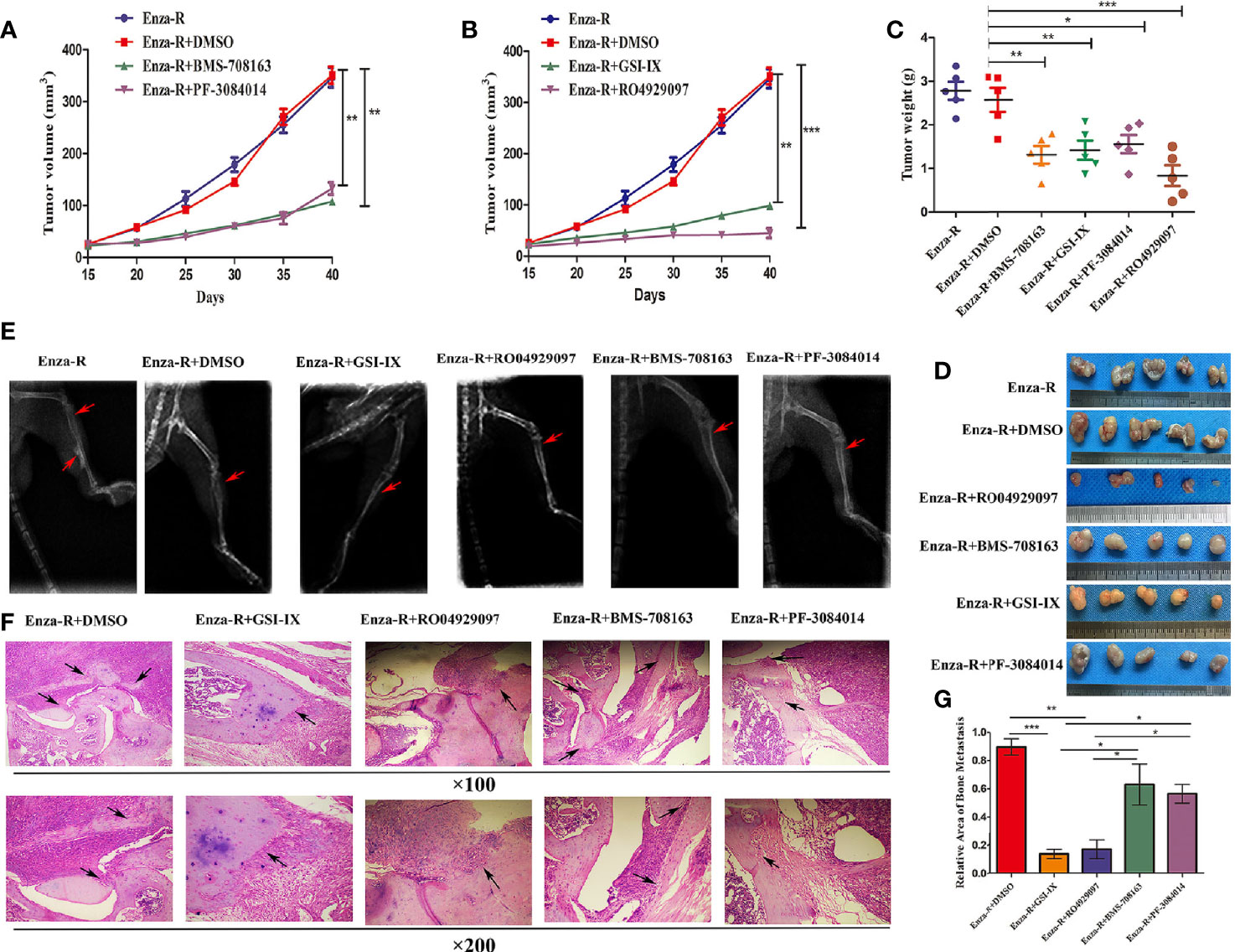
Figure 7 The combination of γ-secretase inhibitors and enzalutamide suppressed growth and bone metastasis of Enza-R cells in vivo. The nude mice with subcutaneous or bone xenograft were respectively treated 10 mg/kg enzalutamide and 50 mg/kg various γ-secretase inhibitors. (A, B) Tumor growth curve (C) Weight of tumors (D) Images of the recovered tumors (E) X-ray for bone metastasis (F) H&E staining for bone metastasis (upper×100, lower ×200). (G) The quantitative results of bone metastasis based on bone metastasis. *P < 0.05, **P < 0.01, ***P < 0.001.
Discussion
SOX17 is a tumor suppressor in various cancer types (28, 39, 40). Of note, recently, some studies revealed that SOX17 may promote rather than suppress tumorigenesis (41, 42). The present study demonstrated that SOX17 is overexpressed in both CRPC tissues and Enza-R cells and associated with a poorer prognosis in CRPC patients. Down-regulation of SOX17 significantly restored enzalutamide sensitivity in Enza-R cells by decreasing AR activity. Taken together, SOX17 is an oncogene in CRPC models, hence may be a potential target for CRPC therapy.
Dysregulation of the Notch signaling pathway is associated with PCa, including CRPC (15, 18–20, 25), with the down-regulation of Notch signaling reducing the growth and invasion of prostate cancer (43–45). The present study showed that knockdown of Notch1 and Notch4 but not Notch2 and Notch3 partly restored the sensitivity to enzalutamide, suggesting that Notch1 and Notch4 are responsible for the drug resistance by inhibiting AR activity. This is in contrast to a study by Yin Wang et al (46) which reported that reducing Notch signaling by down-regulating DLL1 fails to inhibit AR signaling in LNCaP, PC3 cells. In our opinion, unlike in PCa cells, the biological behavior and phenotype of signaling pathways are changed in CRPC models, which may result in an association between Notch signaling and the AR signaling pathway.
Several γ-secretase inhibitors have been reported to have anti-tumor effects in various cancer types, for example, clinical trials of PF-03084014, LY900009, and DAPT have shown promising anti-tumor effects in advanced cancer types (47–49). Down-regulation of Notch activities by γ-secretase inhibitors, such as DAPT, MK-0752, and PF-3084014, re-sensitizes enzalutamide-resistant prostate cancer cells to enzalutamide (18–20, 25, 32, 45); hence γ-secretase inhibitors are promising agents for the treatment of advanced PCa, including CRPC models. In the present study, we found that γ-secretase inhibitors significantly re-sensitize Enza-R cells to enzalutamide, with GSI-IX and RO4929097 being more effective than BMS-708163 and PF-3084014 in reliving bone metastasis, suggesting that both GSI-IX and RO4929097 have more potential for the treatment of CRPC by reversing enzalutamide resistance.
Taken together, our findings provide evidence that activity of SOX17/Notch1 or the Notch4 axis is responsible for enzalutamide resistance in CRPC models, and the pharmacological inhibition of the Notch signaling pathway by the γ-secretase inhibitors GSI-IX and RO4929097 is a promising therapeutic strategy for CRPC.
Data Availability Statement
The original contributions presented in the study are included in the article/supplementary material. Further inquiries can be directed to the corresponding authors.
Ethics Statement
The studies involving human participants were reviewed and approved by the Ethics Committee of Chongqing Medical University. The patients/participants provided their written informed consent to participate in this study. The animal study was reviewed and approved by the Ethics Committee of Chongqing Medical University. Written informed consent was obtained from the individual(s) for the publication of any potentially identifiable images or data included in this article.
Author Contributions
Conception and design: ZD, LL, CL, XDY, and XW. Development of methodology: ZD, LL, WS, PZ, SC, and XSY. Acquisition of data: ZD, LL, WS, and PZ. Analysis and interpretation of data: ZD, LL, WS, XDY, and XW. Writing, review, and/or revision of the manuscript: ZD, LL, XDY, and XW. All authors contributed to the article and approved the submitted version.
Funding
This study was supported by the Natural Science Foundation of China (no 81901629), Health and Family Planning Commission of Sichuan Province (no 20PJ154), and Nanchong Municipal Science, Technology and Intellectual Property Office (no. 180180).
Conflict of Interest
The authors declare that the research was conducted in the absence of any commercial or financial relationships that could be construed as a potential conflict of interest.
Acknowledgments
The authors would like to thank Dr. Xuemei Xie, Department of Pathology, Affiliated Hospital of North Sichuan Medical College for her technical assistance with immunohistochemistry and immunofluorescence.
References
1. Siegel RL, Miller KD, Jemal A. Cancer statistics, 2019. CA Cancer J Clin (2019) 69(1):7–34. doi: 10.3322/caac.21551
2. Chen W, Zheng R, Baade PD, Zhang S, Zeng H, Bray F, et al. Cancer statistics in China, 2015. CA Cancer J Clin (2016) 66:115–32. doi: 10.3322/caac.21338
3. Davis ID, Martin AJ, Stockler MR, Begbie S, Chi KN, Chowdhury S, et al. Enzalutamide with standard first-line therapy in metastatic prostate cancer. N Engl J Med (2019) 381(2):121–31. doi: 10.1056/NEJMoa1903835
4. Fizazi K, Tran N, Fein L, Matsubara N, Rodriguez-Antolin A, Alekseev BY, et al. Abiraterone plus prednisone in metastatic, castration-sensitive prostate cancer. N Engl J Med (2017) 377(4):352–60. doi: 10.1056/NEJMoa1704174
5. Oudard S, Fizazi K, Sengeløv L, Daugaard G, Saad F, Hansen S, et al. Cabazitaxel Versus Docetaxel As First-Line Therapy for Patients With Metastatic Castration-Resistant Prostate Cancer: A Randomized Phase III Trial-FIRSTANA. J Clin Oncol (2017) 35(28):3189–97. doi: 10.1200/JCO.2016.72.1068
6. de Bono JS, Oudard S, Ozguroglu M, Hansen S, Machiels JP, Kocak I, et al. Prednisone plus cabazitaxel or mitoxantrone for metastatic castration-resistant prostate cancer progressing after docetaxel treatment: a randomised open-label trial. Lancet (2010) 376(9747):1147–54. doi: 10.1016/S0140-6736(10)61389-X
7. Karim F, Howard IS, Arturo M, Christopher JL, Kim NC, Robert JJ, et al. Abiraterone acetate for treatment of metastatic castration-resistant prostate cancer: final overall survival analysis of the COU-AA-301 randomised, double-blind,placebo-controlled phase 3 study. Lancet Oncol (2012) 13:983–92. doi: 10.1016/S1470-2045(12)70379-0
8. Ryan CJ, Smith MR, Fizazi K, Saad F, Mulders PF, Sternberg CN, et al. Abiraterone acetate plus prednisone versus placebo plus prednisone in chemotherapy-naïve men with metastatic castration-resistant prostate Cancer (COU-AA-302): final overall survival analysis of a randomised, double-blind, placebo-controlled phase 3 study. Lancet Oncol (2015) 16(2):152–60. doi: 10.1016/S1470-2045(14)71205-7
9. Christopher JDW, Thenappan C, Hanan G, Laurence K, Neil F, Raj S, et al. Advanced Androgen Blockage in Nonmetastatic Castration-resistant Prostate Cancer: An Indirect Comparison of Apalutamide and Enzalutamide. Eur Urol Oncol (2018) 1(3):238–41. doi: 10.1016/j.euo.2018.04.004
10. Joaquin M, Karim F, Silke G, Axel H, Perez-Lopez R, Wim JGO, et al. Managing Nonmetastatic Castration-resistant Prostate Cancer. Eur Urol (2019) 75(2):285–93. doi: 10.1016/j.eururo.2018.07.035
11. Zhang ZZ, Cheng LJ, Li J, Farah E, Nadia MA, Pete EP, et al. Inhibition of the Wnt/β-Catenin Pathway Overcomes Resistance to Enzalutamide in Castration-Resistant Prostate Cancer. Cancer Res (2018) 78(12):3147–62. doi: 10.1158/0008-5472.CAN-17-3006
12. Pak S, Park S, Kim Y, Park JH, Park CH, Lee KJ, et al. The small molecule WNT/β-catenin inhibitor CWP232291 blocks the growth of castration-resistant prostate cancer by activating the endoplasmic reticulum stress pathway. J Exp Clin Cancer Res (2019) 38(1):342. doi: 10.1186/s13046-019-1342-5
13. Prabhakar R, Ian MS, Eugenia MV, Ernest M, Janis F, Mark D, et al. Next-generation sequencing of advanced prostate cancer treated with a1ndrogen-deprivation therapy. Eur Urol (2014) 66(1):32–9. doi: 10.1016/j.eururo.2013.08.011
14. Li L, Du ZB, Gao YY, Tang Y, Fan YR, Sun W, et al. PLCϵ knockdown overcomes drug resistance to androgen receptor antagonist in castration-resistant prostate cancer by suppressing the wnt3a/β-catenin pathway. J Cell Physiol (2019) 234(9):15472–86. doi: 10.1002/jcp.28195
15. Guo YJ, Zhan K, Cheng CP, Ji ZZ, Wang X, Wang ML, et al. Numb-/low enriches a castration resistant prostate cancer cell subpopulation associated with enhanced Notch and Hedgehog signaling. Clin Cancer Res (2017) 23(21):6744–56. doi: 10.1158/1078-0432.CCR-17-0913
16. Minoru K, Carmen AB, Yusuke I, Jacky KL, Daniel PC, Wang J, et al. Co-targeting androgen receptor splice variants and mTOR signaling pathway for the treatment of castration-resistant prostate cancer. Clin Cancer Res (2016) 22(11):2744–54. doi: 10.1158/1078-0432.CCR-15-2119
17. Étienne A, Catherine RD, Tracey Y, Fatima ZZ, Ming Y, Georges K, et al. Nuclear mTOR acts as a transcriptional integrator of the androgen signaling pathway in prostate cancer. Genes Dev (2017) 31(12):1228–42. doi: 10.1101/gad.299958.117
18. Stoyanova T, Riedinger M, Lin S, Faltermeier CM, Smith BA, Zhang KX, et al. Activation of Notch1 synergizes with multiple pathways in promoting castration-resistant prostate cancer. Proc Natl Acad Sci (2016) 113(42):E6457–66. doi: 10.1073/pnas.1614529113
19. Ajinkya R, Maria LP, Alberto T, Abdullah A, Chen JJ, Hermeto G, et al. Inhibition of Notch pathway arrests PTEN-deficient advanced prostate cancer by triggering p27-driven cellular senescence. Nat Commun (2016) 7:13719. doi: 10.1038/ncomms13719
20. Cui J, Wang YQ, Dong BJ, Qin LX, Wang C, Zhou PJ, et al. Pharmacological inhibition of the Notch pathway enhances the efficacy of androgen deprivation therapy for prostate cancer. Int J Cancer (2018) 143(3):645–56. doi: 10.1002/ijc.31346
21. Artavanis-Tsakonas S, Rand MD, Lake RJ. Notch signaling: cell fate control and signal integration in development. Science (1999) 284:770–76. doi: 10.1126/science.284.5415.770
22. Wang XD, Leow CC, Zha J, Tang Z, Modrusan Z, Radtke F, et al. Notch signaling is required for normal prostatic epithelial cell proliferation and differentiation. Dev Biol (2006) 290(1):66–80. doi: 10.1016/j.ydbio.2005.11.009
23. Oh-Joon K, Joseph MV, Zhan L, Zhang BY, Wei X, Su QT, et al. Increased Notch signalling inhibits anoikis and stimulates proliferation of prostate luminal epithelial cells. Nat Commun (2014) 5:4416. doi: 10.1038/ncomms5416
24. Joseph MV, Zhang L, Su QT, Olga D, Zhang YQ, Payam S, et al. Notch and TGFβ form a reciprocal positive regulatory loop that suppresses murine prostate basal stem/progenitor cell activity. Cell Stem Cell (2012) 11(5):676–88. doi: 10.1016/j.stem.2012.07.003
25. Elia F, Li CH, Cheng LJ, Kong YF, Nadia AL, Pete P, et al. NOTCH signaling is activated in and contributes to resistance in enzalutamide-resistant prostate cancer cells. J Biol Chem (2019) 294(21):8543–54. doi: 10.1074/jbc.RA118.006983
26. Gubbay J, Collignon J, Koopman P, Capel B, Economou A, Münsterberg A, et al. A gene mapping to the sex-determining region of the mouse Y chromosome is a member of a novel family of embryonically expressed genes. Nature (1990) 346:245–50. doi: 10.1038/346245a0
27. Elisa L, Maitane A, Laura PS, Jesus MB, Oscar B, Jose JGM, et al. MRP3-mediated chemoresistance in cholangiocarcinoma: Target for chemosensitization through restoring SOX17 expression. Hepatology (2019) 72(3):949–64. doi: 10.1002/hep.3108
28. Maite MA, Elisa L, María JP, Aitor EB, Oihane E, Álvaro SL, et al. SOX17 regulates cholangiocyte differentiation and acts as a tumor suppressor in cholangiocarcinoma. J Hepatol (2017) 67(1):72–83. doi: 10.1016/j.jhep.2017.02.017
29. Ye YW, Wu JH, Wang CM, Zhou Y, Du CY, Zheng BQ, et al. Sox17 regulates proliferation and cell cycle during gastric cancer progression. Cancer Lett (2011) 307(2):124–31. doi: 10.1016/j.canlet.2011.03.024
30. Yang HS, Lee SS, Lee S, Kim KS, Yang Y, Kim JH, et al. Sox17 promotes tumor angiogenesis and destabilizes tumor vessels in mice. J Clin Invest (2013) 123(1):418–31. doi: 10.1172/JCI64547
31. Kathleen ED, Jason CH, Kenneth KT, Fong CP, Christopher JH, Washington MK, et al. Identification and manipulation of biliary metaplasia in pancreatic tumors. Gastroenterology (2014) 146:233–44. doi: 10.1053/j.gastro.2013.08.053
32. Du ZB, Li L, Sun W, Wang X, Zhang Y, Chen ZX, et al. HepaCAM inhibits the malignant behavior of castration-resistant PCa cells by downregulating Notch signaling and PF-3084014 (a γ-secretase inhibitor) partly reverses the resistance of refractory PCa to docetaxeland enzalutamide in vitro. Int J Oncol (2018) 53(1):99–112. doi: 10.3892/ijo.2018.4370
33. Kopan R, Ilagan MX. The canonical Notch signaling pathway: Unfolding the activation mechanism. Cell (2009) 137(2):216–33. doi: 10.1016/j.cell.2009.03.045
34. Schroeter EH, Kisslinger JA, Kopan R. Notch-1 signalling requires ligand-induced proteolytic release of intracellular domain. Nature (1998) 393(6683):382–6. doi: 10.1038/30756
35. Panagiotis N, Lim JS, Sage J, Iannis A. From fly wings to targeted cancer therapies: A centennial for notch signaling. Cancer Cell (2014) 25(3):318–24. doi: 10.1016/j.ccr.2014.02.018
36. Yun J, Pannuti A, Espinoza I, Zhu H, Hicks C, Zhu X, et al. Crosstalk between PKCα and Notch-4 in endocrine-resistant breast cancer cells. Oncogenesis (2013) 2:e60. doi: 10.1038/oncsis.2013.26
37. Ran Y, Hossain F, Pannuti A, Lessard CB, Ladd GZ, Jung JI, et al. γ-secretase inhibitors in cancer clinical trials are pharmacologically and functionally distinct. EMBO Mol Med (2017) 9(7):950–66. doi: 10.15252/emmm.201607265
38. Margot JP, Tyler H, Gabriel OC, Joe JA, Jessica K, Alexander IS, et al. γ-Secretase inhibition increases efficacy of BCMA-specific chimeric antigen receptor T cells in multiple myeloma. Blood (2019) 134(19):1585–97. doi: 10.1182/blood.2019000050
39. Du YC, Hiroko O, Keisuke O, Takanori K, Hiraku I, Takashi F, et al. Induction and down-regulation of Sox17 and its possible roles during the course of gastrointestinal tumorigenesis. Gastroenterology (2009) 137:1346–57. doi: 10.1053/j.gastro.2009.06.041
40. Zhang W, Sabine CG, Guo MZ, Emi OM, David HW, Hariharan E, et al. Epigenetic inactivation of the canonical Wnt antagonist SRY-box containing gene 17 in colorectal cancer. Cancer Res (2008) 68:2764–72. doi: 10.1158/0008-5472.CAN-07-6349
41. He SH, Kim I, Megan SL, Sean JM. SOX17 expression confers self-renewal potential and fetal stem cell characteristics upon adult hematopoietic progenitors. Genes Dev (2011) 25:1613–27. doi: 10.1101/gad.2052911
42. Cheng L, Albers P, Berney DM, Feldman DR, Daugaard G, Gilligan T, et al. Testicular cancer. Nat Rev Dis Primers (2018) 4(1):29. doi: 10.1038/s41572-018-0029-0
43. Kron KJ, Murison A, Zhou S, Huang V, Yamaguchi TN, Shiah YJ, et al. TMPRSS2-ERG fusion co-opts master transcription factors and activates NOTCH signaling in primary prostate cancer. Nat Genet (2017) 49(9):1336–45. doi: 10.1038/ng.3930
44. Ahmed AM, Shyh-Han T, Charles PX, Shilpa K, Huang W, Lakshmi R, et al. Synergistic activity with NOTCH inhibition and androgen ablation in ERG-positive prostate cancer cells. Mol Cancer Res (2017) 15(10):1308–17. doi: 10.1158/1541-7786.MCR-17-0058
45. Zhang JW, Kuang Y, Wang Y, Xu QQ, Ren QH. Notch-4 silencing inhibits prostate cancer growth and EMT via the NF-κB pathway. Apoptosis (2017) 22(6):877–84. doi: 10.1007/s10495-017-1368-0
46. Wang Y, Wu X, Ou L, Yang X, Wang X, Tang M, et al. PLCϵ knockdown inhibits prostate cancer cell proliferation via suppression of Notch signalling and nuclear translocation of the androgen receptor. Cancer Lett (2015) 362(1):61–9. doi: 10.1016/j.canlet.2015.03.018
47. Wells AM, Geoffrey IS, James MC, Antonio J, Arvind D, Bo H, et al. A phase I dose-finding study in patients with advanced solid malignancies of the oralγ-secretase inhibitor PF-03084014. Clin Cancer Res (2015) 21:60–7. doi: 10.1158/1078-0432.CCR-14-0607
48. Shubham P, Suzanne FJ, Carla DK, Jeffrey RI, Kathleen NM, Howard AB, et al. A firstin-human phase i study of the oral Notch inhibitor, LY900009, in patients with advanced cancer. Eur J Cancer (2016) 56:1–9. doi: 10.1016/j.ejca.2015.11.021
Keywords: castration-resistant prostate cancer, SOX17, Notch receptor family members, γ secretase inhibitors, enzalutamide
Citation: Du Z, Li L, Sun W, Zhu P, Cheng S, Yang X, Luo C, Yu X and Wu X (2021) Systematic Evaluation for the Influences of the SOX17/Notch Receptor Family Members on Reversing Enzalutamide Resistance in Castration-Resistant Prostate Cancer Cells. Front. Oncol. 11:607291. doi: 10.3389/fonc.2021.607291
Received: 16 September 2020; Accepted: 05 January 2021;
Published: 10 March 2021.
Edited by:
Massimo Broggini, Istituto di Ricerche Farmacologiche Mario Negri (IRCCS), ItalyReviewed by:
Alfredo Berruti, University of Brescia, ItalySergio Bracarda, Azienda Ospedaliera S.Maria, Italy
Copyright © 2021 Du, Li, Sun, Zhu, Cheng, Yang, Luo, Yu and Wu. This is an open-access article distributed under the terms of the Creative Commons Attribution License (CC BY). The use, distribution or reproduction in other forums is permitted, provided the original author(s) and the copyright owner(s) are credited and that the original publication in this journal is cited, in accordance with accepted academic practice. No use, distribution or reproduction is permitted which does not comply with these terms.
*Correspondence: Xiaodong Yu, yuxiaodong1975@yeah.net; Xiaohou Wu, 112751265@qq.com
†These authors have contributed equally to this work