- 1Department of Neurosurgery, Zhengzhou University People’s Hospital, Henan Provincial People’s Hospital, Zhengzhou, China
- 2School of Basic Medicine, Henan University of Chinese Medicine, Zhengzhou, China
- 3Department of Neurology, The Fifth Affiliated Hospital of Zhengzhou University, Zhengzhou, China
Tumor in situ fluid (TISF) refers to the fluid at the local surgical cavity. We evaluated the feasibility of TISF-derived circulating tumor DNA (ctDNA) characterizing the genomic landscape for glioma. This retrospective study included TISF and tumor samples from 10 patients with glioma, we extracted cell-free DNA (cfDNA) from the TISF and then performed deep sequencing on that. And we compared genomic alterations between TISF and tumor tissue. Results showed that the concentration of cfDNA fragments from the patients for TISF ranged from 7.2 to 1,397 ng/ml. At least one tumor-specific mutation was identified in all 10 patients (100%). Further analysis of TISF ctDNA revealed a broad spectrum of genetic mutations, which have been reported to have clinical relevance. The analysis of concordance between TISF and tumor tissue reflected the spatiotemporal heterogeneity of glioma. Collectively, TISF ctDNA was a powerfully potential source for characterizing the genomic landscape of glioma, which provided new possibilities for precision medicine in patients with glioma.
Introduction
Glioma is a tumor with high molecular heterogeneity, its constantly evolving genetic landscape can be a huge obstacle to the clinical management of patients (1). Studies about sequential glioma biopsies have unearthed enormous divergence of the cancer genome between the initial and recurrent tumor from the same patient (2, 3). The need for precision medicine is to characterize the real-time molecular status of the tumor, which is believed to have therapeutic significance for glioma patients (4). However, serial biopsies constitute a high risk to patients and are not realistic in clinical practice.
Liquid biopsy, defined as biofluids used for molecular diagnostics (5), has brought new hope and possibilities to precision medicine. For example, blood-based circulating tumor DNA (ctDNA) detection has proved to be of great clinical significance for a variety of solid tumors (5), such as breast (6), gastric (7), and esophageal (8) cancers. However, regarding the CNS, identifying detectable ctDNA in plasma remains challenging (9). Current studies reported that cerebrospinal fluid (CSF) could be a promising reservoir of ctDNA for brain glioma (10, 11), but with the concomitant imperfection that tumor shedding DNA to the CSF is affected by tumor burden and tumor abutting CSF (11).
The body fluid for liquid biopsy should include any conceivable stable-acquired fluid collection (12). The surgical resection for glioma always creates a cavity at the tumor site, inside exists fluid that connects or does not connect with the CSF reservoir. Here, we coined the term “Tumor in situ fluid (TISF)” to describe the fluid in the local surgical cavity. It is known that most gliomas relapse at the local site (13, 14), thus tumor-shed biomarkers would congregate in TISF over the process of treatment resistance and tumor progression. Moreover, with an intraoperatively implanted reservoir, obtaining TISF postoperatively is clinically feasible and uninjurious. Based on this hypothesis, we retrospectively investigated whether ctDNA from TISF could be a new source of liquid biopsy for precision medicine for patients with glioma.
Materials and Methods
Patients and Ethics Statement
This study included tumor in situ fluid (TISF) and tumor samples from 10 patients who were diagnosed as glioma (WHO grade III or IV) and treated in Henan Provincial People’s Hospital (HPPH). All patients received surgical treatment with a reservoir implanted into the surgical cavity intraoperatively and TISF were collected after surgery. The retrospective study was approved by the institutional review board and ethics committee of HPPH (Zhengzhou, China). Written informed consent was signed by all patients and/or their legal representatives.
Sample Collection
As mentioned above, a reservoir for postoperative local chemotherapy was indwelled into the resection cavity during the surgery for every patient. After surgery, 0.5 to 1 ml TISF was collected for every patient using the indwelled reservoir (Figure 1). Unstained paraffin-embedded tumor tissues (UPETT) were collected, and tumor DNA was isolated for 9 of the 10 cases (90%). Blood samples (5 ml) for germline DNA control from each patient were also obtained.
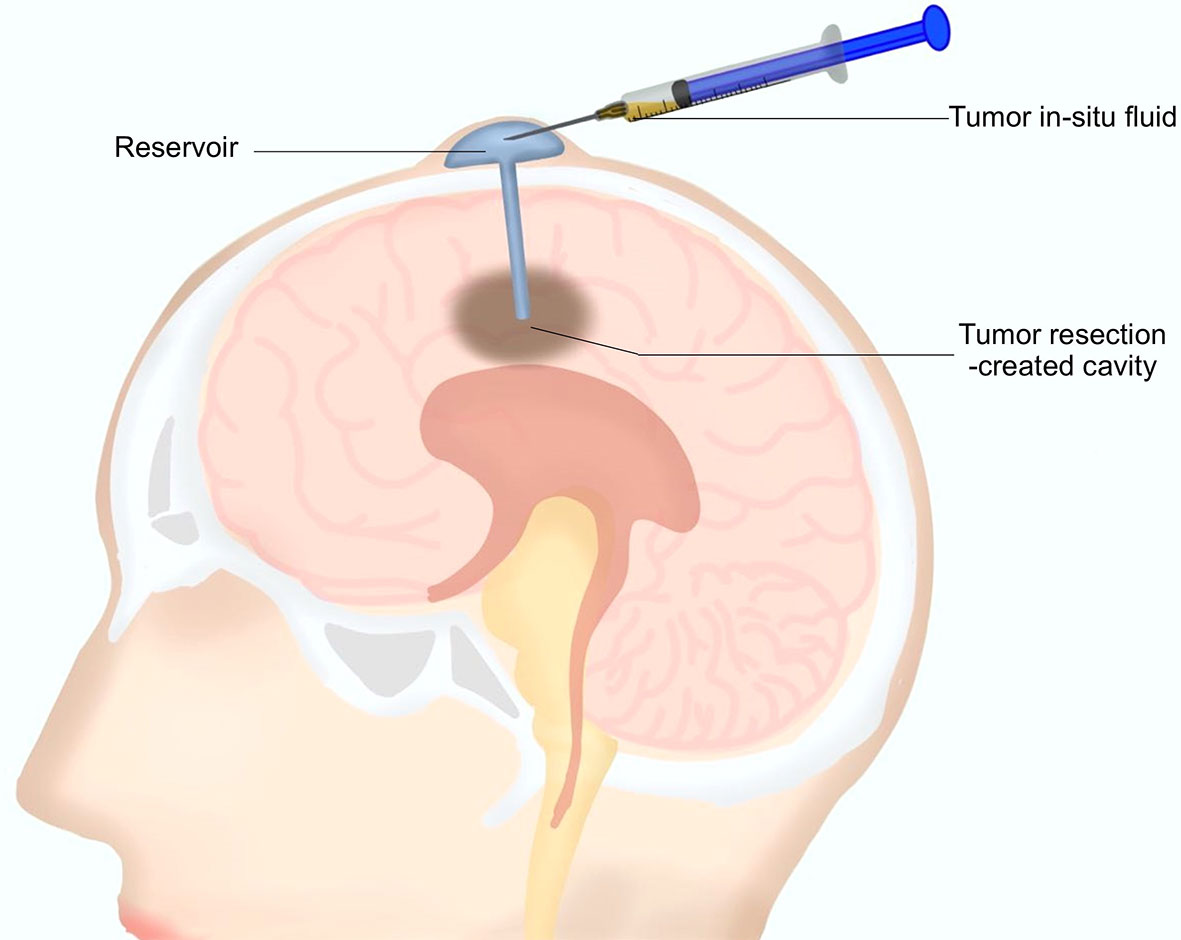
Figure 1 A diagram for the tumor in situ fluid (TISF) collection. A reservoir was implanted into the resection cavity during the resection surgery. The dome end was placed beneath the scalp attached to the catheter that was inserted within the brain leading to the tumor resection-created cavity. A small amount of fluid could be extracted with a disposable milliliter syringe from the resection cavity via this reservoir postoperatively.
Isolation of Tumor DNA and Germline DNA
As described elsewhere (10), EDTA tubes containing biofluids (TISF or CSF or blood) were centrifuged at 1,900g for 10 min, after that, the supernatants from those were additionally centrifuged at 16,000g for 10 min. And then samples were stored at −80°C before extraction. Genomic DNA was extracted from UPETT with the QIAamp DNA Tissue & Blood Kit (Qiagen; Germantown, MD, USA). Cell-free DNA (cfDNA) was extracted from biofluids using the MagMAX™ CellFree DNA Isolation Kit (ThermoFisher Scientific; Waltham, MA, USA). Finally, all segregated DNA was quantified using the Qubit 2.0 Fluorometer with the Qubit dsDNA HS Assay kit (Life Technologies; Carlsbad, CA, USA) following the advised protocol.
NGS Library and Sequencing Data Analysis
As described elsewhere, the DNA libraries were captured with a designed panel of 68 genes for brain tumors (GenetronHealth; Beijing, China) (10), those genes containing major brain tumor-related genes. The DNA sequencing was based on novaseq high-throughput sequencing platform.
Statistical Analysis
The log-rank test was used to compare the survival difference between different groups, and the nonparametric Mann Whitney test was for difference analysis between the two groups.
Result
Patient Characteristics
This cohort included seven female and three male who were diagnosed as WHO grade III or IV glioma, Specifically, six IDH wild-type glioblastoma (GBM) (60%), three IDH mutant low-grade (III) glioma and 1 IDH mutant GBM (40%) according to the 2016 WHO brain tumor classification with IDH status (15). The mean age was 52.7 years (33–66 years). Four tumors were located at the frontal lobe, three at the temporoparietal lobe, two at the temporal lobe, and the last one at the thalamus. Patients’ characteristics were summarized in Table 1. All patients received concurrent chemoradiotherapy (CRT) followed by adjuvant temozolomide (TMZ) combining with local Methotrexate chemotherapy after surgery. Clinical courses of patients were presented in Figure 2.
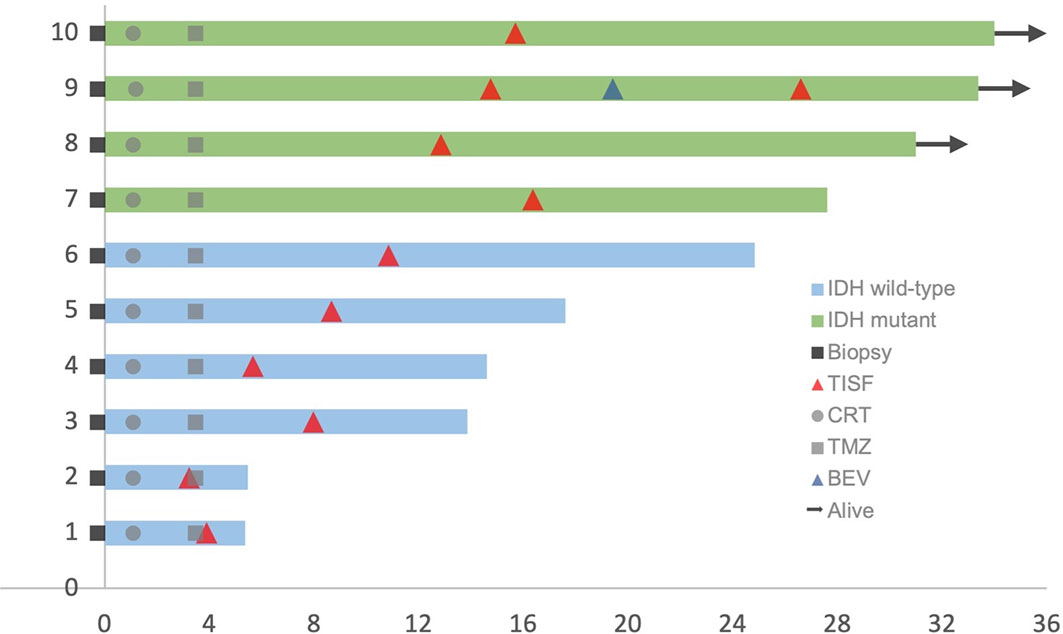
Figure 2 Clinical courses of included patients. Each patient began from the primary surgery (Biopsy). TISF, tumor in situ fluid; CRT, concurrent chemoradiotherapy; TMZ, adjuvant temozolomide chemotherapy; BEV, bevacizumab.
TISF ctDNA Represented the Genetic Landscape of Glioma
The concentration of cfDNA from TISF ranged between 7.2 and 1,397 ng/ml (Figure 3C), which was high enough to minimize analytic errors in subsequent analysis. In total, 83 alterations of 37 different genes were detected. The average number of mutations detected in the TISF-derived ctDNA of respective individuals was 7.55 ± 6.79 (range, 1 to 22). The most frequently altered genes were TP53 (50%), SETD2 (40%), NF1 (30%), PTEN (30%), RELA (30%), DAXX (30%), EGFR (30%), and FAT1 (30%). The most common category of mutation was missense mutation (65.1%), followed by frame-shifting mutation (18.1%) and nonsense mutation (13.3%) (Figure 3A).
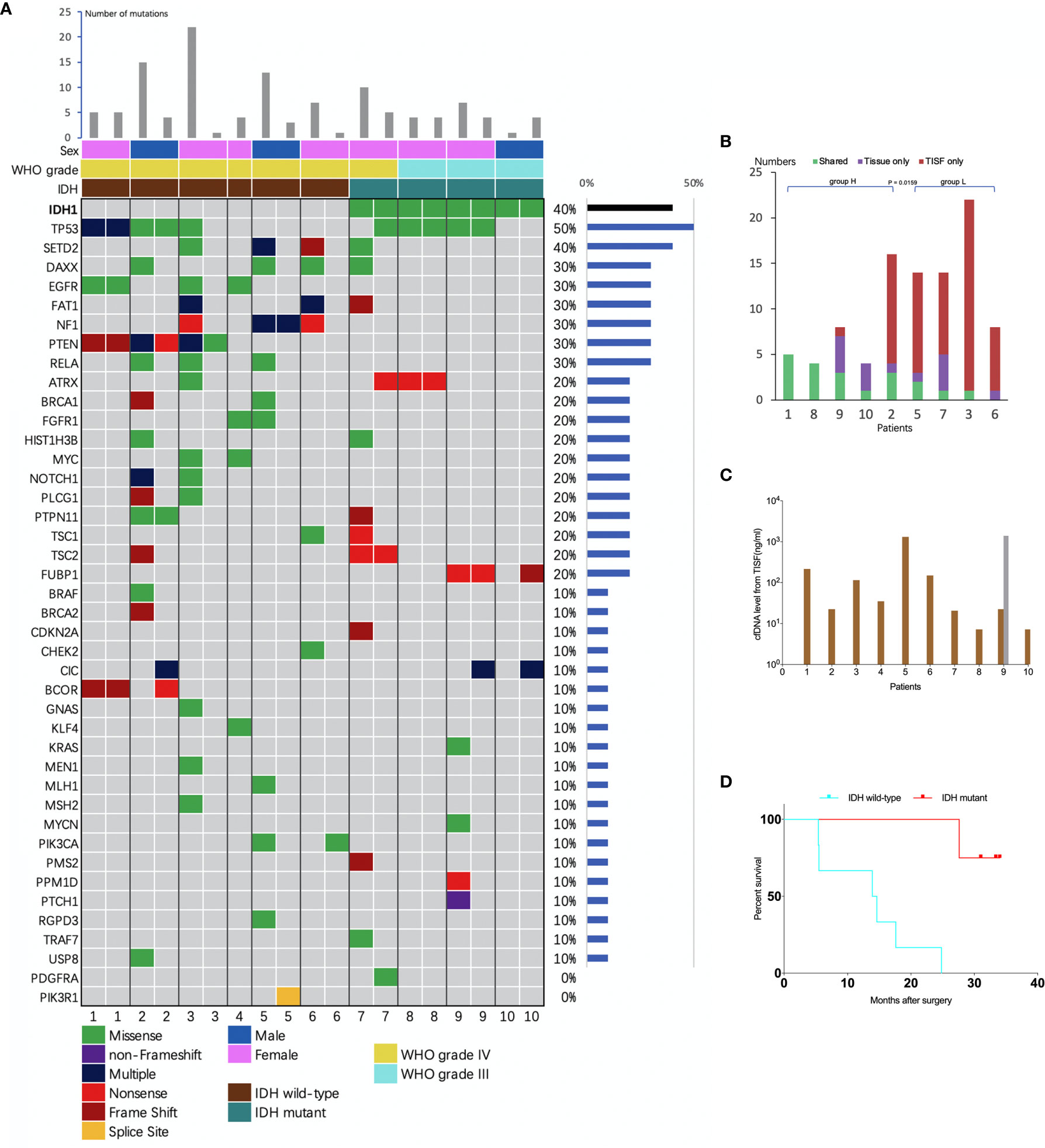
Figure 3 Analysis of tumor DNA from TISF and tumor tissue. (A) the mutational landscape for both TISF and tumor tissue. For each patient, the left column represented TISF-derived ctDNA and the right column represented tumor tissue, patient 4 just had one column for the TISF. (B) Concordance of tumor DNA between TISF and tumor tissue. The percentage of shared mutations varied from 0% to 100% (median 30.0%). (C) Cell-free DNA (cfDNA) levels from TISF. The concentration of cfDNA from TISF ranged between 7.2 and 1397 ng/ml. For patient 9, the second time TISF cfDNA experienced a significant increase. (D) Survival curve for patients with different IDH status. In all 10 patients (including 6 IDH wild type and 4 IDH mutant gliomas), the IDH status was accordant between the TISF and tumor tissue (100%). Median overall survival after the primary surgery was 14.2 and 32.2 months (P = 0.0038) for TISF wild-type IDH and TISF mutant IDH glioma, respectively.
To identify whether TISF ctDNA could reflect the genome alterations of glioma, we analyzed the sequencing results in depth. Firstly, IDH status was included to determining the diagnosis for glioma in the WHO 2016 classification (15). We examined whether this genetic alteration could be detected in the TISF and matched that of the tumor tissue. In all 10 patients (including six IDH wild type and four IDH mutant gliomas), the IDH status was accordant between the TISF and tumor tissue (100%). Median overall survival after the primary surgery was 14.2 and 32.2 months for wild-type IDH and mutant IDH glioma (P = 0.0038), respectively (Figure 3D).
Further analysis of TISF ctDNA revealed a broad spectrum of genetic mutations which have been reported to have clinical relevance. For example, mutations of PTEN, TSC2, TSC1, NF1, and PIK3CA have been demonstrated activating PI3K/AKT/mTOR pathways, which will then boost the growth and progression of the tumor (16); FAT1 alteration is reported closely related to Wnt signaling pathway (17); modification on tumor suppressor genes, including TP53, BRCA1, BRCA2, CHEK2, and PTCH1 and on cell cycle genes, such as CDKN2A (18, 19). Many of these mutations are promising targets of precision therapeutic regimens (Table 2). Additionally, TMZ as an alkylating agent can induce the mutations of mis-match repair (MMR) genes, which is related to treatment resistance (20). We identified MMR gene mutations (MSH2, MLH1, and PMS2) in three patients (patients 3, 5, and 7), including two IDH wild-type GBM and one IDH-mutant GBM, all of them received systematic TMZ treatment previously, and we did not detect this signature in their tumor tissues.
Collectively, these results indicated that ctDNA from TISF was capable to characterize the genomic landscape of glioma during its progression process.
Concordance Between TISF and Tumor Tissue
We compared the sequencing results of tumor DNA from TISF and UPETT for nine patients (Figure 4). The median interval between biopsy (surgery) and the first TISF collection was 10.8 months (3.2–16.4 months). The percentage of shared mutations varied from 0% to 100% (median, 31.6%) (Figure 3B). We further divided the 9 patients into 2 groups: group H (n=5, patients 1, 2, 8, 9, 10) included patients with low-grade glioma ((WHO grade III)) or underwent a short interval between TISF collection and tumor tissue biopsy (less than 4 months); group L included the remaining four patients (patients 3, 5, 6, 7). Subsequently, the median for the percentages of shared mutations of group H came at 54.5% (31.6%–100%), and the median for group L became 8.3% (0–25%), there was a significant difference between the two groups (P = 0.0159) (Figure 3B).
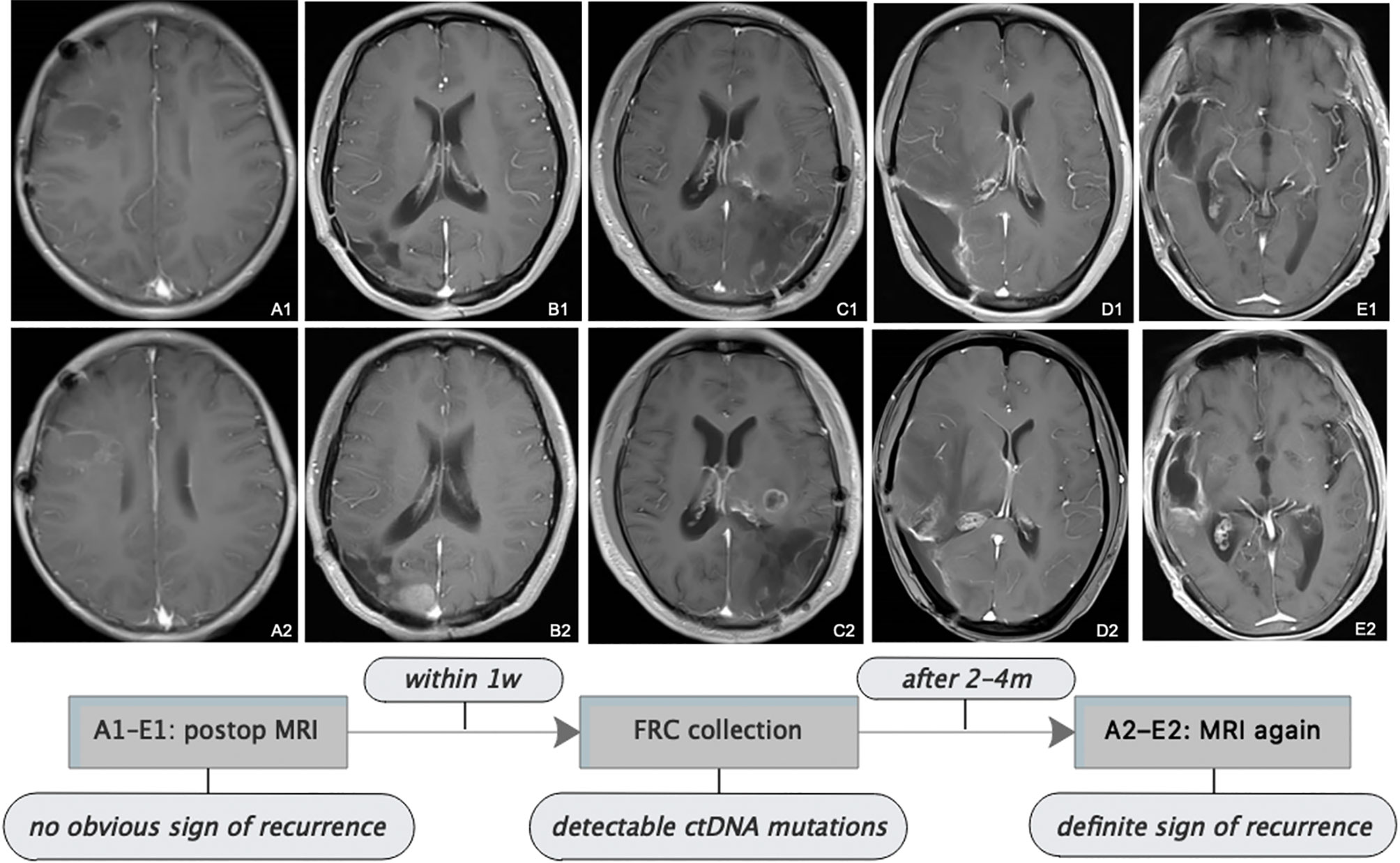
Figure 4 Follow-up MRI showed tumor progression. All patients received serial MRI as the standard of care. As presented in the images and the illustrative flowchart, A1–E1 stand for a postoperative representative timepoint, at which we could not notice an obvious sign of tumor progression on the MRI scans, but at which we suspected tumor progression according to the comprehensive conditions of patients, then TISF was collected, in which tumor-specific ctDNA mutations were detected. 2–4 months later, as shown in the images A2–E2, definite radiologic sign of tumor recurrence appeared. This is interesting but we still presume that the simple signature of ctDNA-positive in TISF could not be a biomarker for early predicting the recurrence of glioma, because of the special location of TISF, which is at the local site of the tumor. The tumor progression should be determined by a specific genetic analysis.
Despite those considerable divergences between TISF and tumor tissue, for some key genes, on the other hand, high concordance was observed between TISF and tumor tissue, one is the above-mentioned IDH1, another example is the PTEN mutation (three patients, 100%) which can activate the PI3K/AKT/mTOR signaling pathways (18). Furthermore, for patient 9 (WHO grade III anaplastic oligodendroglioma), we collected another TISF for ctDNA analysis at 26.6 months after surgery. We observed more shared mutations with tumor tissue than the first TISF (3 vs. 0) and higher accordance (60% vs. 0).
TISF ctDNA Was Before Imaging Progression
Postoperatively, all patients had serial follow-up MRI. We noticed that TISF collection was performed at time points when apparent signs could not be spotted on the MRI scans for five patients. And it was until 2 to 4 months after TISF collection when doubtless recurrence signs could be observed on the images (Figure 4). However, we presumed that shedding DNA to TISF was a universal property of glioma because of the special location of TISF, thus ctDNA-positive in TISF could not be a biomarker for predicting the recurrence of glioma, and tumor progression should be determined by the specific genetic results as described above.
Discussion
Dynamically characterizing the heterogeneity of glioma with liquid-based biomarkers is a powerful tool for precision medicine, which has been increasing the likelihood of curing malignancies (5). Here we originally demonstrated the feasibility of TISF as a steady source of liquid biopsy for glioma, providing new insight to precision medicine for brain tumors.
We identified abundant alterations derived from glioma in TISF, in-depth analysis proved that those mutations could reflect the genomic landscape of glioma during the treatment process. The landscape included PI3K/AKT/mTOR pathways-related genes, tumor suppressor genes, cell cycle genes, etc. This evidenced that multiple mechanisms contribute to the genesis and development of glioma, many of them are potential targets of precision therapies (Table 2).
In a previous CSF-based liquid biopsy study, Miller et al. observed a considerable divergence of shared mutations between CSF samples and tumor tissues (0–100%) across patients. Meantime, they found that samples collected at a closer interval (<3 weeks) had a higher percentage of shared mutations (79%) than those collected at a longer interval (>1,000 days) (29%) (11). Here we observed a comparable phenomenon in this pilot study. All samples were collected at a long interval (>3 months) and the percentage of shared mutations varied considerably from 0% to 100% (median, 31.6%). Interestingly, when we divided patients into two groups, i.e., group H containing patients with low-grade glioma or with a shorter interval (<4 months) and group L including the remaining patients, the fact came that group H had a higher percentage of shared mutations (median 54.5%) than group L did (median 8.3%). Several scenarios might account for these divergences. One is the spatial heterogeneity of glioma, i.e., the observation that tumor cells can show distinct genetic characteristics and one tumor biopsy usually only represents a part of tumor heterogeneity. Two is the temporal heterogeneity of glioma, which refers to that tumor can develop clonal and subclonal evolution with time, as it did in patient 9, who received two-time TISF ctDNA collection, we observed higher concordance with tumor tissue and higher cfDNA level than of the first TISF. This dynamic variation was expected correlated with the decrease in tumor burden attributed to the surgical resection and then the gradually clonal together with subclonal progression of the tumor. Another factor is due to the therapeutic pressure, which typically affects the genetic evolution of a tumor (21, 22). For example, it has been elucidated that TMZ application can induce the MMR deficit with the hypermutation ensuring in the glioma genome, which then gives contributions to the TMZ treatment resistance (20). We observed MMR gene mutations in three patients in our cohort, which was attributed to TMZ treatment. Despite those divergences and dynamics, we also noted that certain mutations were present in both tumor tissue and TISF, such as IDH1 and PTEN, which support the perspective that certain mutations or pathways occurred at the early stage of tumorigenesis and contribute to the tumor growth and progression throughout the life of the tumor (22, 23). In a word, these phenomenon and features of the tumor greatly evidenced the urgent need of precision characterization of genomic status for glioma in real time. Future frequent TISF-based liquid biopsies would be able to depict the genomic dynamics under therapeutic pressure and based on that to guide the modification of treatment combinations.
Imaging-based assessment of tumor progression for glioma cannot overcome the hysteresis and cannot reflect the molecular status of the tumor, which makes it impossible for clinicians to monitor the microscope progression of glioma from the early stage of postoperative management. In this study, we noted that detectable mutations of ctDNA from TISF were prior to clear evidence of radiologic recurrence. It was interesting but we still presumed that the simple signature of ctDNA-positive in TISF could not be a biomarker for early predicting the recurrence of glioma, because of the special location of TISF, which is at the local site of the tumor. It could be a universal property of glioma to shed tumor DNA into TISF at any stage. And we hypothesized that potential biomarkers would appear locally in high concentrations in TISF and might then be found diluted in the CSF and eventually plasma, the latter two signatures might be the adverse prognostic factors. The tumor progression should be determined by a specific genetic analysis.
In conclusion, we proved the feasibility of TISF ctDNA for characterizing the genomic landscape of glioma, this original insight provided new perspectives and possibilities for precision medicine in patients with glioma.
Data Availability Statement
The data sets presented in this study can be found in online repositories. The names of the repository/repositories and accession number(s) can be found below: https://figshare.com/articles/dataset/original_gene_analysis_reports_zip/12672692.
Ethics Statement
The studies involving human participants were reviewed and approved by the Institutional Review Board (IRB) and Ethics Committee of Henan Provincial People’s Hospital (Zhengzhou, China). All methods were carried out in accordance with the approved guidelines. Written informed consent was obtained from all patients. Written informed consent was obtained from the minor(s)’ legal guardian/next of kin for the publication of any potentially identifiable images or data included in this article.
Author Contributions
XB and ZS conceived and designed the study. JY, NL, KD, and ZS collected the clinical data and samples. ZS and XB performed the data analysis. ZS wrote the manuscript. SL produced the figures. JH, HA-B, and AZ reviewed the data and revised the manuscript. YS, ZC, and ZY gave the conceptual advice. All authors contributed to the article and approved the submitted version.
Funding
This work was supported by the Science and Technology Tackle Program of Henan Province (grant 192102310126).
Conflict of Interest
The authors declare that the research was conducted in the absence of any commercial or financial relationships that could be construed as a potential conflict of interest.
Acknowledgments
We thank Juntao Li and Xiang Sun for their help in collecting and shipping samples. We thank Guangqi An, who assisted in the illustration for Figure 1.
Abbreviations
TISF, tumor in situ fluid; ctDNA, circulating tumor DNA.
References
1. Nicholson JG, Fine HA. Diffuse Glioma Heterogeneity and Its Therapeutic Implications. Cancer Discovery (2021) 11(3):575–90. doi: 10.1158/2159-8290.CD-20-1474
2. Lee JK, Wang J, Sa JK, Ladewig E, Lee HO, Lee IH, et al. Spatiotemporal genomic architecture informs precision oncology in glioblastoma. Nat Genet (2017) 49(4):594–9. doi: 10.1038/ng.3806
3. Georgescu MM, Olar A. Genetic and histologic spatiotemporal evolution of recurrent, multifocal, multicentric and metastatic glioblastoma. Acta Neuropathol Commun (2020) 8(1):10. doi: 10.1186/s40478-020-0889-x
4. Tan AC, Ashley DM, López GY, Malinzak M, Friedman HS, Khasraw M. Management of glioblastoma: State of the art and future directions. CA Cancer J Clin (2020) 70:299–312. doi: 10.3322/caac.21613
5. Pantel K, Alix-Panabières C. Liquid biopsy and minimal residual disease — latest advances and implications for cure. Nat Rev Clin Oncol (2019) 16(7):409–24. doi: 10.1038/s41571-019-0187-3
6. Chen YH, Hancock BA, Solzak JP, Brinza D, Scafe C, Miller KD, et al. Next-generation sequencing of circulating tumor DNA to predict recurrence in triple-negative breast cancer patients with residual disease after neoadjuvant chemotherapy. NPJ Breast Cancer (2017) 3:24. doi: 10.1038/s41523-017-0028-4
7. Leal A, van Grieken NCT, Palsgrove DN, Phallen J, Medina JE, Hruban C, et al. White blood cell and cell-free DNA analyses for detection of residual disease in gastric cancer. Nat Commun (2020) 1(1):525. doi: 10.1038/s41467-020-14310-3
8. Azad TD, Chaudhuri AA, Fang P, Qiao Y, Esfahani MS, Chabon JJ, et al. Circulating Tumor DNA Analysis for Detection of Minimal Residual Disease After Chemoradiotherapy for Localized Esophageal Cancer. Gastroenterology (2020) 158(3):494–505.e6. doi: 10.1053/j.gastro.2019.10.039
9. Sareen H, Garrett C, Lynch D, Powter B, Brungs D, Cooper A, et al. The role of liquid biopsies in detecting molecular tumor biomarkers in brain cancer patients. Cancers (Basel) (2020) 12(7):1831. doi: 10.3390/cancers12071831
10. Pan C, Diplas BH, Chen X, Wu Y, Xiao X, Jiang L, et al. Molecular profiling of tumors of the brainstem by sequencing of CSF-derived circulating tumor DNA. Acta Neuropathol (2019) 137:297–306. doi: 10.1007/s00401-018-1936-6
11. Miller AM, Shah RH, Pentsova EI, Pourmaleki M, Briggs S, Distefano N, et al. Tracking tumour evolution in glioma through liquid biopsies of cerebrospinal fluid. Nature (2019) 565:654–8. doi: 10.1038/s41586-019-0882-3
12. Le Rhun E, Seoane J, Salzet M, Soffietti R, Weller M. Liquid biopsies for diagnosing and monitoring primary tumors of the central nervous system. Cancer Lett (2020) 480:24–8. doi: 10.1016/j.canlet.2020.03.021
13. Fukuya Y, Ikuta S, Maruyama T, Nitta M, Saito T, Tsuzuki S, et al. Tumor recurrence patterns after surgical resection of intracranial low-grade gliomas. J Neurooncol (2019) 144:519–28. doi: 10.1007/s11060-019-03250-8
14. Spiteri I, Caravagna G, Cresswell GD, Vatsiou A, Nichol D, Acar A, et al. Evolutionary dynamics of residual disease in human glioblastoma. Ann Oncol (2019) 30(3):456–63. doi: 10.1093/annonc/mdy506
15. Louis DN, Perry A, Reifenberger G, von Deimling A, Figarella-Branger D, Cavenee WK, et al. The 2016 World Health Organization Classification of Tumors of the Central Nervous System: a summary. Acta Neuropathol (2016) 131(6):803–20. doi: 10.1007/s00401-016-1545-1
16. Esposito A, Viale G, Curigliano G. Safety, Tolerability, and Management of Toxic Effects of Phosphatidylinositol 3-Kinase Inhibitor Treatment in Patients with Cancer: A Review. JAMA Oncol (2019) 5(9):1347–54. doi: 10.1001/jamaoncol.2019.0034
17. Bugter JM, Fenderico N, Maurice MM. Mutations and mechanisms of WNT pathway tumour suppressors in cancer. Nat Rev Cancer (2021) 21(1):5–21. doi: 10.1038/s41568-020-00307-z
18. Chen L, Liu S, Tao Y. Regulating tumor suppressor genes: post-translational modifications. Signal Transduct Target Ther (2020) 5(1):90. doi: 10.1038/s41392-020-0196-9
19. Hafner A, Bulyk ML, Jambhekar A, Lahav G. The multiple mechanisms that regulate p53 activity and cell fate. Nat Rev Mol Cell Biol (2019) 20(4):199–210. doi: 10.1038/s41580-019-0110-x
20. Touat M, Li YY, Boynton AN, Spurr LF, Iorgulescu JB, Bohrson CL, et al. Mechanisms and therapeutic implications of hypermutation in gliomas. Nature (2020) 580:517–23. doi: 10.1038/s41586-020-2209-9
21. Johnson BE, Mazor T, Hong C, Barnes M, Aihara K, McLean CY, et al. Mutational analysis reveals the origin and therapy-driven evolution of recurrent glioma. Sci (80- ) (2014) 343(6167):189–93. doi: 10.1126/science.1239947
22. Barthel FP, Johnson KC, Varn FS, Moskalik AD, Tanner G, Kocakavuk E, et al. Longitudinal molecular trajectories of diffuse glioma in adults. Nature (2019) 576(7785):112–20. doi: 10.1038/s41586-019-1775-1
Keywords: tumor in situ fluid, circulating tumor DNA, glioma, precision medicine, liquid biopsy
Citation: Sheng Z, Yu J, Deng K, Andrade-Barazarte H, Zemmar A, Li S, Li N, Yan Z, Chen Z, Sun Y, Hernesniemi J and Bu X (2021) Characterizing the Genomic Landscape of Brain Glioma With Circulating Tumor DNA From Tumor In Situ Fluid. Front. Oncol. 11:584988. doi: 10.3389/fonc.2021.584988
Received: 24 July 2020; Accepted: 08 March 2021;
Published: 31 March 2021.
Edited by:
George Calin, University of Texas MD Anderson Cancer Center, United StatesReviewed by:
Zuheir Alshehabi, Tishreen University, SyriaJuliano Andreoli Miyake, Federal University of Santa Catarina, Brazil
Copyright © 2021 Sheng, Yu, Deng, Andrade-Barazarte, Zemmar, Li, Li, Yan, Chen, Sun, Hernesniemi and Bu. This is an open-access article distributed under the terms of the Creative Commons Attribution License (CC BY). The use, distribution or reproduction in other forums is permitted, provided the original author(s) and the copyright owner(s) are credited and that the original publication in this journal is cited, in accordance with accepted academic practice. No use, distribution or reproduction is permitted which does not comply with these terms.
*Correspondence: Xingyao Bu, YnV4aW5neWFvQDEyNi5jb20=