- 1Department of Intensive Care Unit, Affiliated Hangzhou First People’s Hospital, Zhejiang University School of Medicine, Hangzhou, China
- 2Department of Oncology, The First Affiliated Hospital of Chongqing Medical University, Chongqing, China
- 3Chongqing Key Laboratory of Molecular Oncology and Epigenetics, The First Affiliated Hospital of Chongqing Medical University, Chongqing, China
Background: Studies on the prognostic value of the soluble programmed death ligand 1 (sPD-L1) in cancer patients have not yielded consistent results.
Objective: This meta-analysis was performed to assess the association between sPD-L1 and the prognosis of cancer patients.
Methods: Published articles in Pubmed, EMBASE, and Cochrane clinical trial databases were searched from the inception to September 2020. Overall survival (OS), progression-free survival (PFS), recurrence-free survival (RFS), and disease-free survival (DFS) data were evaluated using a hazard ratio (HR) at 95% confidence interval (95% CI).
Results: A total 31 studies involving 17 tumors and 3,780 patients were included. The overexpression of sPD-L1 was associated with shorter OS (HR 1.85, 95% CI 1.59–2.15, I2 = 33%). High sPD-L1 had worse PFS (HR 2.40, 95% CI 1.55–3.72, I2 = 83%), and worse DFS (HR 2.92, 95% CI 2.02–4.29, I2 = 40%), without significant statistical difference in RFS (HR 2.08, 95% CI 0.99–4.40, I2 = 0%).
Conclusions: High sPD-L1 levels were associated with worse survival prognosis in cancer patients. The sPD-L1 may be a potential prognostic, non-invasive, and dynamic monitoring biomarker for cancers in the future.
Introduction
Immune checkpoint inhibitors such as anti-PD-1/PD-L1 have remarkable clinical benefits in a variety of tumors (1, 2). The expression of programmed death ligand 1 (PD-L1) is often observed in a variety of cancers. The combination of PD-L1 and its receptor PD-1 on activated T cells suppresses antitumor immunity by counteracting T cell activation signals. Antibody-based PD-1/PD-L1 inhibitors can induce durable tumor remission of various advanced cancer types.
PD-L1 exists in two forms; membrane-bound and soluble form (3). Studies have shown that soluble PD-L1 (sPD-L1) may be derived from the shedding of tumor cells or the release of immune cells in tumor tissues (3, 4). Evidence shows that the surfaces of exosomes secreted by tumor cells have biologically active PD-L1, which can suppress immune responses (5). The circulatory cell expressing PD-L1 still retains its biological activity, and it can specifically bind the PD-1 receptor of the T cells in peripheral blood, thereby activating the PD-1/PD-L1 pathway and establishing systemic immunosuppression (6, 7). Moreover, sPD-L1 is a prognostic marker of tumor treatment (8).
Studies on the relationship between sPD-L1 and the prognosis of cancer patients have yielded contrasting results. In NSCLC, studies by He et al. showed that patients with high sPD-L1 have a reduced risk of death (9). However, data from Okuma et al. showed that lung cancer patients with high sPD-L1 have an increased risk of death (10). In gastric cancer and nasopharyngeal carcinoma, there is no correlation between soluble PD-L1 levels and overall survival (4, 11). This systematic review and meta-analysis aimed at evaluating the value and clinical significance of sPD-L1 in the survival prognosis of cancer patients.
Materials and Methods
Search Strategy and Inclusion Criteria
Relevant articles published in various databases were searched since the inception of the databases to September 2020. The Pubmed, EMBASE, and Cochrane clinical trial databases were searched for the meta-analysis. The Clinicaltrial.gov, American Society of Clinical Oncology (ASCO) and ESMO databases were also searched. Search terms were composed of various combinations of “soluble PD-L1”, “sPD-L1”, “serum PD-L1”, “plasma PD-L1”, “circulatory PD-L1”, “blood PD-L1”, “programmed death ligand 1”, “B7-H1” and “cancer”, “neoplasm”, “carcinoma”, “lymphoma”, “sarcomas”. The language of the article was not restricted.
The inclusion criteria for various studies were: i. The included patients were all pathologically diagnosed tumor patients; ii. With analyzable soluble PD-L1 data; iii. With sufficient clinical features and data that could be combined to analyze soluble PD- L1 and survival prognosis; iv. Had their sPD-L1 levels detected by enzyme-linked immunosorbent assay (ELISA). The exclusion criteria were: i. Case reports, reviews, and tissue immunohistochemical (IHC) detection for PD-L1; ii. Animal experiments; iii. No direct analysis of the relationship between soluble PD-L1 and survival prognosis; iv. Incomplete data or non-original research.
Data Extraction and Quality Assessment
First, the outcomes of each study were extracted, including overall survival (OS), progression-free survival (PFS), recurrence-free survival (RFS), and disease-free survival (DFS) prognosis data. Next, the baseline data of the studies were extracted and analyzed; publication years, study type, patient characteristics, treatment methods, and the cut-off value of sPD-L1, etc. Based on the purpose of our research, OS was the primary outcome of interest. All data screening and extraction processes were independently performed by two investigators.
The Newcastle-Ottawa Quality Assessment Scale (NOS) was used to assess the quality of the study. The NOS consists of three parts: selection (4 points), comparability (2 points), and outcome evaluation (3 points). Table 1 shows the NOS scores of the included studies. All processes were independently performed by two reviewers and in case of disagreements, they were discussed to reach a consensus. Studies with an NOS score ≥6 were considered high-quality.
Statistical Analysis
The 95% confidence interval (CI) of all results was calculated. All outcomes were directly extracted from the studies. Time-to-event endpoints (OS, PFS, DFS, and RFS) were pooled with the use of hazard ratio (HR). Heterogeneity was assessed using the χ2-based Q test and I2 statistics. I2 >75% indicated considerable heterogeneity (38). The random-effects model was applied for pooled analysis. The RevMan software (version 5.3) from Cochrane library was used for meta-analysis. Publication bias was evaluated by funnel plots. Sensitivity analysis was performed by the one by one elimination method of individual studies to assess the reliability of the results.
Results
Characteristics of the Included Studies
We initially identified 926 articles from the searched databases, and after excluding duplicates, 642 articles remained. After excluding 514 records, the full text of 128 articles were screened. After full-text screening, 97 studies were excluded due to the following reasons; not original studies (n = 11), irrelevant studies (n = 24), irrelevant outcomes (n = 32), incomplete data (n = 17), and the cohort overlaps (n = 13). Finally, 31 studies involving 17 tumors and a total of 3,780 patients met our inclusion criteria (Figure 1). The tumor types included in the study were; Nasopharyngeal Carcinoma (11), Cholangiocarcinoma (12), lung cancer (9, 10, 13–17), Hepatocellular carcinoma (20, 21, 39, 40), Thyroid carcinoma (22), Mesothelioma (23), Colorectal carcinoma (24, 25), NK/T cell lymphoma (26, 27), Peripheral T-cell lymphoma (28), Large B-Cell lymphoma (29, 35), Hodgkin lymphoma (31), Ovarian carcinoma (32), Soft tissue sarcomas (33), Renal cell carcinoma (7), Esophageal carcinoma (34), Gastric carcinoma (4, 35), and Pancreatic adenocarcinoma (36, 41).
The main characteristics of the 31 studies are summarized in Table 1. The NOS scores of the included studies were all greater than or equal to 6 points, except for several conference abstract articles (Table 1).
Survival Analysis
Among the included studies, 28 studies have OS as their primary outcome. OS data from these 28 studies were pooled, and the results showed that overexpression of sPD-L1 was associated with shorter OS (HR 1.85, 95% CI 1.59–2.15, I2 = 33%) (Figure 2). The funnel plot showed that there was no significant publication bias (Figure 3). The publication bias analysis of RFS was not performed due to the limited number of included studies. Sensitivity analysis showed that the results were stable and reliable.
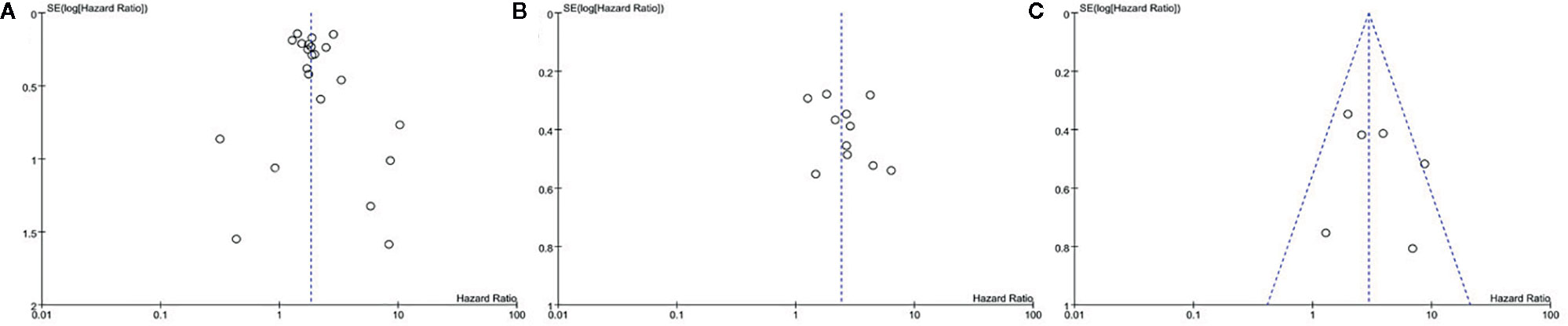
Figure 3 Funnel plot of the (A) overall survival, (B) PFS, and (C) DFS in patients of high and low sPD-L1.
In terms of secondary outcomes, PFS, RFS, and DFS were also pooled for analysis. The pooled data of 11 studies showed that high sPD-L1 is correlated with worse PFS (HR 2.40, 95% CI 1.55–3.72, I2 = 83%). Besides, six studies included DFS data. High sPD-L1 was correlated with worse DFS (HR 2.92, 95% CI 2.02–4.29, I2 = 40%). Moreover, only two studies reported RFS, and sPDL1 was insignificantly correlated with a worse RFS (HR 2.08, 95% CI 0.99–4.40, I2 = 0%) (Table 2).
Subgroup Analysis of OS
In the included 28 studies with OS data, there were different observation types, treatment methods, cut-off values of sPD-L1 and survival analysis methods. Therefore, we performed a subgroup analysis of these differences. The results showed that high sPD-L1 in both retrospective (HR 1.70, 95% CI 1.47–1.96, I2 = 21%) and prospective studies (HR 2.44, 95% CI 1.70–3.51, I2 = 32%) was correlated with worse OS. Studies were stratified according to whether surgery was performed, and the results showed that surgery did not affect the correlation between sPD-L1 and OS (surgery: HR 2.06, 95% CI 1.43–2.96, I2 = 31%; non-surgery: HR 1.91, 95% CI 1.57–2.32, I2 = 44%) (Table 3). The subgroup analysis based on immunotherapy revealed similar results.
As an important indicator, the cut-off value of sPD-L1 was divided into three levels of less than 1, 1–5, and greater than 5 ng/ml for subgroup analysis. In the three levels, high sPD-L1 was consistently correlated with worse OS (<1 ng/ml: HR 2.17, 95% CI 1.62–6.89, I2 = 45%; 1–5 ng/ml: HR 2.12, 95% CI 1.42–3.17, I2 = 44%, and >5 ng/ml: HR 1.53, 95% CI 1.17–1.99, I2 = 21%). We also performed subgroup analyses based on cut-off points (0.5 and 1 ng/ml, 1 and 10 ng/ml, and others). We found that the significant association between sPD-L1 and survival did not change with the change in cutoff points. Furthermore, sPD-L1 was associated with worse OS in both univariate (HR 2.07, 95% CI 1.40–3.04, I2 = 45%) and multivariate analysis (HR 1.83, 95% CI 1.55–2.18, I2 = 30%) (Table 3). We further performed subgroup analysis based on the time point of the detection of sPD-L1. Majority of the studies used the pre-treatment sPD-L1. They assessed the level of sPD-L1 at baseline when patients were initially diagnosed. The high level of sPD-L1 represented a worse OS in all three subgroups. Additionally, we performed subgroup analysis according to the specific cancer types. As presented in Figure 4, sPD-L1 was correlated with a worse OS in a majority of the cancers. Insignificant results were only obtained in the subgroups with less included studies (nasopharyngeal cancer, ovarian carcinoma, soft tissue sarcomas, renal cell carcinoma and esophageal carcinoma, gastric carcinoma).
Discussion
This meta-analysis summarizes the correlation between the level of sPD-L1 and the prognosis of various cancer patients. The main significance of sPD-L1 detection is to establish a minimally invasive, easy-to-clinical, and predictive immune biomarker for cancer patients.
PD-L1 can be divided into membrane-bound PD-L1 (mPD-L1) and sPD-L1. It is believed that sPD-L1 is mainly produced by the proteolysis of mPD-L1. The immunosuppressive correlation and prognostic values of mPD-L1/sPD-L1 have not been established. Currently, IHC detection is commonly used in clinical practice to detect mPD-L1. Previous studies have explored the relationships between mPD-L1 expression levels in tumor tissues and clinical prognosis. However, these studies are limited by difficulties in sampling tumor tissues, as well as by the inability to dynamically detect changes in patients during treatment. In addition, the relationships between the levels of PD-L1 as detected by tissue IHC and the prognosis of cancer patients are not clinically binding (42, 43). Uncertainty in sampling tumor tissues, IHC staining conditions and antibody complexity, evaluator standards as well as positive cut-off values may all affect the assessment of PD-L1 levels. The advantage of sPD-L1 over mPD-L1 is that sPD-L1 can be ubiquitously present in plasma and serous effusions, and its detection is relatively convenient, repeatable and objective. A study tracked and monitored the levels of PD-L1 in plasma exosomes of patients with melanoma, and showed that all patients had exosomal-PD-L1, while only 67% of tumor biopsy specimens were PD-L1 positive (44).
We found that high sPD-L1 levels can be used as a prognostic biomarkers for poor treatment outcomes. Secondly, it has been proved that the sPD-L1 still retains its biological activity. We can further postulate that sPD-L1 in peripheral blood can be able to specifically bind T cells in peripheral blood, thereby, inhibiting T cell activity and inducing systemic immune suppression (33). When PD-L1 positive exosomes were co-cultured with activated T cells, a significant decrease in CD69 on the surface of T cells could be observed (45). The sPD-L1 can not only exert an immunosuppressive effect in the local tumor microenvironment but can also act on the distal end of the body. sPD-L1 in the blood can effectively suppress the secretion of IFN-γ by T cells, and can participate in systemic anti-tumor immune regulation by targeting T lymphocytes in secondary lymphoid organs (44, 46).
Subgroup analyses were performed based on the treatment or cut-off value of the patient. In the subgroup analysis of patients receiving immunotherapy, patients with high sPD-L1 levels were correlated with a worse OS. An NSCLC study showed that the death risk in patients with high levels of sPD-L1 was 2.68 (95%CI 1.36–5.28) times the risk in patients with low sPD-L1 levels (14). Given that sPD-L1 can be derived from tumor tissue cells, the level of sPD-L1 can reflect tumor regression to a certain extent. This implies that sPD-L1 has the potential to be used as a biomarker for predicting the prognosis of immunotherapy. Due to the high variations in sPD-L1 between various tumors and the limited number of samples, the median level of the sPD-L1 and the analyzed cut-off values are quite different. We divided sPD-L1 into three intervals for subgroup analysis, and the results consistently showed that sPD-L1 was associated with poor prognosis. The sampling error of patients and the differences between multiple tumors make it difficult for us to accurately analyze the relationship between the specific level of sPD-L1 and prognosis, however, it can still reflect the prognostic differences between patients with high and low sPD-L1. More stringent cut-off values require further exploration of large sample RCT experiments. Additionally, we also found that sPD-L1 can predict prognosis in patients subjected to, and those not subjected to surgical procedures. In NSCLC patients after radical surgery, sPD-L1 with a median value of 3.84 ng/ml could still be detected (9). This suggests that there is no release of primary foci and that there is still some detectable sPD-L1 in peripheral blood. Moreover, it implies that sPD-L1 can be derived from antigen-presenting cells in the blood. This indicates that in patients with radically resected tumors, the detection of sPD-L1 can be used to monitor immune checkpoint suppression, predict the efficacy of immunotherapy, and predict survival prognosis. This is the ability that tumor tissue IHC does not have in PD-L1 detection.
sPD-L1 can be used as a good indicator for the survival of cancer patients. Several studies have evaluated the predictive role of other immune-related biomarkers (such as soluble PD-L1, Vascular Endothelial Growth Factor A, soluble CD40L, CTLA-4, and soluble CD44) in different types of cancers (47). Meyo et al. proposed a composite biomarker using sPD-L1 and other immune-related biomarkers to predict nivolumab efficacy in NSCLC patients (13). The combination of inflammatory status indicators such as neutrophil to lymphocyte ratio with sPD-L1 has also been evaluated (12). The peripheral cytokine can be combined with sPD-L1 to predict treatment benefits and prognosis (48). The prognostic values of biomarkers that play a role in the expression of PD-L1 (such as STAT3) and their combination with sPD-L1 have also been explored (49). More combinations of sPD-L1 and other plasma indicators should be evaluated for cancer patients’ prognosis. The plasma indicators might improve the predictive performance of sPD-L1.
Two systematic reviews and meta-analysis have evaluated the relationship between sPD-L1 and tumor prognosis. The review by Ding et al. in 2017 included eight articles with a total of 1,102 cancer patients (50). Wei et al. also published a review article in 2017 that included eight articles and a total of 1,040 patients with solid tumors (51). They all found worse prognostic outcomes for patients with highly expressed sPD-L1. In recent years, a studies sPD-L1 have been published, and their results are not consistent with the above two reviews. Therefore, this review, we increased the number of studies to 31 involving 17 tumors. Compared to the previous reviews, the results of this study show that sPD-L1 is associated with worse prognostic outcomes among tumor patients receiving immunotherapy. This is different from the well-known high response rate of immunotherapy in patients with positive mPD-L1 expression (52), which also shows the different functions and significance of sPD-L1 and mPD-L1 in tumors.
This study has certain limitations. First, the studies included in this meta-analysis were observational studies, due to the lack of relevant randomized controlled trials (RCTs). Higher quality RCTs are needed to further evaluate the prognostic value of sPD-L1. Second, the number of patients with each tumor included in this study was small, and a larger sample population is needed for verification. Third, different tumor types have different molecular signatures including immune checkpoint regulators, therefore, combining them leads to inaccurate inferences and misleading clinical applications. Finally, the cut-off values of sPD-L1 in different studies were significantly different, leading to limitations in clinical applications. In future, more studies should aim at accurately establishing the correlation between sPD-L1 expression level and prognosis. However, this study shows the prognostic value of sPD-L1 in a variety of tumors and immunotherapy, indicating that sPD-L1 can potentially serve as an innovative biomarker for predicting the prognosis of cancer patients.
In conclusion, this meta-analysis revealed that high sPD-L1 levels were associated with worse survival outcomes (including OS, DFS, and PFS) in cancer patients. And among patients who had received immunotherapy, patients with high sPD-L1 levels had worse OS. The sPD-L1 may be a potential prognostic, non-invasive, and dynamic monitoring biomarker for cancers.
Data Availability Statement
The original contributions presented in the study are included in the article/supplementary material. Further inquiries can be directed to the corresponding authors.
Author Contributions
PH, HL: protocol/project development. PH, HL, YW: data collection and management. HL, WH: data analysis. HL, WH, YZ: manuscript writing/editing. All authors contributed to the article and approved the submitted version.
Conflict of Interest
The authors declare that the research was conducted in the absence of any commercial or financial relationships that could be construed as a potential conflict of interest.
References
1. Carlisle JW, Steuer CE, Owonikoko TK, Saba NF. An update on the immune landscape in lung and head and neck cancers. CA Cancer J Clin (2020). doi: 10.3322/caac.21630
2. Galluzzi L, Humeau J, Buque A, Zitvogel L, Kroemer G. Immunostimulation with chemotherapy in the era of immune checkpoint inhibitors. Nat Rev Clin Oncol (2020). doi: 10.1038/s41571-020-0413-z
3. Abu Hejleh T, Furqan M, Ballas Z, Clamon G. The clinical significance of soluble PD-1 and PD-L1 in lung cancer. Crit Rev Oncol Hematol (2019) 143:148–52. doi: 10.1016/j.critrevonc.2019.08.009
4. Takahashi N, Iwasa S, Sasaki Y, Shoji H, Honma Y, Takashima A, et al. Serum levels of soluble programmed cell death ligand 1 as a prognostic factor on the first-line treatment of metastatic or recurrent gastric cancer. J Cancer Res Clin Oncol (2016) 142(8):1727–38. doi: 10.1007/s00432-016-2184-6
5. Xie F, Xu M, Lu J, Mao L, Wang S. The role of exosomal PD-L1 in tumor progression and immunotherapy. Mol Cancer (2019) 18(1):146. doi: 10.1186/s12943-019-1074-3
6. Chen Y, Wang Q, Shi B, Xu P, Hu Z, Bai L, et al. Development of a sandwich ELISA for evaluating soluble PD-L1 (CD274) in human sera of different ages as well as supernatants of PD-L1+ cell lines. Cytokine (2011) 56(2):231–8. doi: 10.1016/j.cyto.2011.06.004
7. Frigola X, Inman BA, Lohse CM, Krco CJ, Cheville JC, Thompson RH, et al. Identification of a soluble form of B7-H1 that retains immunosuppressive activity and is associated with aggressive renal cell carcinoma. Clin Cancer Res (2011) 17(7):1915–23. doi: 10.1158/1078-0432.CCR-10-0250
8. Jia Y, Li X, Zhao C, Ren S, Su C, Gao G, et al. Soluble PD-L1 as a Predictor of the Response to EGFR-TKIs in Non-small Cell Lung Cancer Patients With EGFR Mutations. Front Oncol (2020) 10:1455. doi: 10.3389/fonc.2020.01455
9. He J, Pan Y, Guo Y, Li B, Tang Y. Study on the Expression Levels and Clinical Significance of PD-1 and PD-L1 in Plasma of NSCLC Patients. J Immunother (Hagerstown Md 1997) (2020) 43(5):156–64. doi: 10.1097/CJI.0000000000000315
10. Okuma Y, Hosomi Y, Nakahara Y, Watanabe K, Sagawa Y, Homma S. High plasma levels of soluble programmed cell death ligand 1 are prognostic for reduced survival in advanced lung cancer. Lung Cancer (Amsterdam Netherlands) (2017) 104:1–6. doi: 10.1016/j.lungcan.2016.11.023
11. Lu T, Chen Y, Li J, Guo Q, Lin W, Zheng Y, et al. High Soluble Programmed Death-Ligand 1 Predicts Poor Prognosis in Patients with Nasopharyngeal Carcinoma. Onco Targets Ther (2020) 13:1757–65. doi: 10.2147/OTT.S242517
12. Ha H, Nam AR, Bang JH, Park J-E, Kim T-Y, Lee K-H, et al. Soluble programmed death-ligand 1 (sPDL1) and neutrophil-to-lymphocyte ratio (NLR) predicts survival in advanced biliary tract cancer patients treated with palliative chemotherapy. Oncotarget (2016) 7(47):76604–12. doi: 10.18632/oncotarget.12810
13. Tiako Meyo M, Jouinot A, Giroux-Leprieur E, Fabre E, Wislez M, Alifano M, et al. Predictive Value of Soluble PD-1, PD-L1, VEGFA, CD40 Ligand and CD44 for Nivolumab Therapy in Advanced Non-Small Cell Lung Cancer: A Case-Control Study. Cancers (2020) 12(2):473. doi: 10.3390/cancers12020473
14. Jin J, Si J, Liu Y, Wang H, Ni R, Wang J. Elevated serum soluble programmed cell death ligand 1 concentration as a potential marker for poor prognosis in small cell lung cancer patients with chemotherapy. Respir Res (2018) 19(1):197. doi: 10.1186/s12931-018-0885-x
15. Zhao J, Zhang P, Wang J, Xi Q, Zhao X, Ji M, et al. Plasma levels of soluble programmed death ligand-1 may be associated with overall survival in nonsmall cell lung cancer patients receiving thoracic radiotherapy. Med (Baltimore) (2017) 96(7):e6102. doi: 10.1097/MD.0000000000006102
16. Okuma Y, Wakui H, Utsumi H, Sagawa Y, Hosomi Y, Kuwano K, et al. Soluble Programmed Cell Death Ligand 1 as a Novel Biomarker for Nivolumab Therapy for Non-Small-cell Lung Cancer. Clin Lung Cancer (2018) 19(5):410–17.e411. doi: 10.1016/j.cllc.2018.04.014
17. Bai G, Xu Y, Wang M. Relationship of the level of soluble PD-L1 and its relevant proteins in peripheral blood with the prognosis of patients with NSCLC. Ann Oncol (2018) 29:ix143–4. doi: 10.1093/annonc/mdy446.002
18. Lee HW, Cho KJ, Shin SY, Kim BK, Kim SU, Park JY, et al. PD-1 and PD-L1 levels do not predict prognosis in patients with hepatocellular carcinoma. Liver Cancer (2018) 7Supplement 1 (121).
19. Ma X, Yang W, Wang H, Wang B, Shen M, Zhou Y, et al. Soluble programmed death-ligand 1 indicate poor prognosis in hepatocellular carcinoma patients undergoing transcatheter arterial chemoembolization. Annals Oncology (2019) 30Supplement 5 (v283–v284).
20. Chang B, Huang T, Wei H, Shen L, Zhu D, He W, et al. The correlation and prognostic value of serum levels of soluble programmed death protein 1 (sPD-1) and soluble programmed death-ligand 1 (sPD-L1) in patients with hepatocellular carcinoma. Cancer Immunol Immunother (2019) 68(3):353–63. doi: 10.1007/s00262-018-2271-4
21. Finkelmeier F, Canli Ö, Tal A, Pleli T, Trojan J, Schmidt M, et al. High levels of the soluble programmed death-ligand (sPD-L1) identify hepatocellular carcinoma patients with a poor prognosis. Eur J Cancer (2016) 59:152–9. doi: 10.1016/j.ejca.2016.03.002
22. Aghajani MJ, Roberts TL, Yang T, McCafferty CE, Caixeiro NJ, DeSouza P, et al. Elevated levels of soluble PD-L1 are associated with reduced recurrence in papillary thyroid cancer. Endocr Connect (2019) 8(7):1040–51. doi: 10.1530/EC-19-0210
23. Chiarucci C, Cannito S, Daffinà MG, Amato G, Giacobini G, Cutaia O, et al. Circulating Levels of PD-L1 in Mesothelioma Patients from the NIBIT-MESO-1 Study: Correlation with Survival. Cancers (Basel) (2020) 12(2):361. doi: 10.3390/cancers12020361
24. Omura Y, Toiyama Y, Okugawa Y, Yin C, Shigemori T, Kusunoki K, et al. Prognostic impacts of tumoral expression and serum levels of PD-L1 and CTLA-4 in colorectal cancer patients. Cancer Immunol Immunother (2020) 69:2533–46. doi: 10.1007/s00262-020-02645-1
25. Tominaga T, Akiyoshi T, Yamamoto N, Taguchi S, Mori S, Nagasaki T. Clinical significance of soluble programmed cell death-1 and soluble programmed cell death-ligand 1 in patients with locally advanced rectal cancer treated with neoadjuvant chemoradiotherapy. PLoS One (2019) 14(2):e0212978. doi: 10.1371/journal.pone.0212978
26. Wang H, Wang L, Liu W-J, Xia Z-J, Huang H-Q, Jiang W-Q, et al. High post-treatment serum levels of soluble programmed cell death ligand 1 predict early relapse and poor prognosis in extranodal NK/T cell lymphoma patients. Oncotarget (2016) 7(22):33035–45. doi: 10.18632/oncotarget.8847
27. Nagato T, Ohkuri T, Ohara K, Hirata Y, Kishibe K, Komabayashi Y, et al. Programmed death-ligand 1 and its soluble form are highly expressed in nasal natural killer/T-cell lymphoma: a potential rationale for immunotherapy. Cancer Immunol Immunother (2017) 66(7):877–90. doi: 10.1007/s00262-017-1987-x
28. Shen H, Ji Y, Zhou D, Zhang Y, Wang W, Sun J, et al. Soluble programmed death-ligand 1 are highly expressed in peripheral T-cell lymphoma: a biomarker for prognosis. Hematology (2019) 24(1):392–8. doi: 10.1080/16078454.2019.1590965
29. Rossille D, Gressier M, Damotte D, Maucort-Boulch D, Pangault C, Semana G, et al. High level of soluble programmed cell death ligand 1 in blood impacts overall survival in aggressive diffuse large B-Cell lymphoma: results from a French multicenter clinical trial. Leukemia (2014) 28(12):2367–75. doi: 10.1038/leu.2014.137
30. Rossille D, Azzaoui I, Feldman AL, Maurer MJ, Labouré G, Parrens C, et al. Soluble programmed death-ligand 1 as a prognostic biomarker for overall survival in patients with diffuse large B-cell lymphoma: a replication study and combined analysis of 508 patients. Leukemia (2017) 31(4):988–91. doi: 10.1038/leu.2016.385
31. Guo X, Wang J, Jin J, Chen H, Zhen Z, Jiang W, et al. High Serum Level of Soluble Programmed Death Ligand 1 is Associated With a Poor Prognosis in Hodgkin Lymphoma. Transl Oncol (2018) 11(3):779–85. doi: 10.1016/j.tranon.2018.03.012
32. Buderath P, Schwich E, Jensen C, Horn PA, Kimmig R, Kasimir-Bauer S, et al. Soluble Programmed Death Receptor Ligands sPD-L1 and sPD-L2 as Liquid Biopsy Markers for Prognosis and Platinum Response in Epithelial Ovarian Cancer. Front Oncol (2019) 9:1015. doi: 10.3389/fonc.2019.01015
33. Asanuma K, Nakamura T, Hayashi A, Okamoto T, Iino T, Asanuma Y, et al. Soluble programmed death-ligand 1 rather than PD-L1 on tumor cells effectively predicts metastasis and prognosis in soft tissue sarcomas. Sci Rep (2020) 10(1):9077. doi: 10.1038/s41598-020-65895-0
34. Cheng JCH, Hsu FM, Lee JM, Huang PM, Guo JC, Hsu CH. A Prospective Study on Serum PD-L1, TGF-β1, and VEGF-A as Immune-integrated Biomarkers for Locally Advanced Esophageal Squamous Cell Carcinoma. Int J Radiat Oncol Biology Physics (2019) 105(1):S132. doi: 10.1016/j.ijrobp.2019.06.118
35. Shigemori T, Toiyama Y, Okugawa Y, Yamamoto A, Yin C, Narumi A, et al. Soluble PD-L1 Expression in Circulation as a Predictive Marker for Recurrence and Prognosis in Gastric Cancer: Direct Comparison of the Clinical Burden Between Tissue and Serum PD-L1 Expression. Ann Surg Oncol (2019) 26(3):876–83. doi: 10.1245/s10434-018-07112-x
36. Bian B, Fanale D, Dusetti N, Roque J, Pastor S, Chretien A-S, et al. Prognostic significance of circulating PD-1, PD-L1, pan-BTN3As, BTN3A1 and BTLA in patients with pancreatic adenocarcinoma. Oncoimmunology (2019) 8(4):e1561120. doi: 10.1080/2162402X.2018.1561120
37. Park H, Bang J-H, Nam A-R, Park JE, Jin MH, Bang Y-J, et al. Prognostic implications of soluble programmed death-ligand 1 and its dynamics during chemotherapy in unresectable pancreatic cancer. Sci Rep (2019) 9(1):11131.
38. DerSimonian R, Laird N. Meta-analysis in clinical trials revisited. Contemp Clin Trials (2015) 45(Pt A):139–45. doi: 10.1016/j.cct.2015.09.002
39. Han SH. PD-1 and PD-L1 levels do not predict prognosis in patients with hepatocellular carcinoma. Liver Cancer (2018) 7(Supplement 1):121.
40. Hu B, Gong Z, Zhang X, Pan B, Zhou J, Fan J, et al. Soluble programmed death-ligand 1 indicate poor prognosis in hepatocellular carcinoma patients undergoing transcatheter arterial chemoembolization. Ann Oncol (2019) 30(Supplement 5):v283–4. doi: 10.1093/annonc/mdz247.065
41. Chu HH, Kim JH, Kim PN, Kim SY, Lim Y-S, Park SH, et al. Surgical resection versus radiofrequency ablation very early-stage HCC (≤2 cm Single HCC): A propensity score analysis. Liver Int (2019) 39(12):2397–407. doi: 10.1111/liv.14258
42. Stovgaard ES, Dyhl-Polk A, Roslind A, Balslev E, Nielsen D. PD-L1 expression in breast cancer: expression in subtypes and prognostic significance: a systematic review. Breast Cancer Res Treat (2019) 174(3):571–84. doi: 10.1007/s10549-019-05130-1
43. Tsao M-S, Le Teuff G, Shepherd FA, Landais C, Hainaut P, Filipits M, et al. PD-L1 protein expression assessed by immunohistochemistry is neither prognostic nor predictive of benefit from adjuvant chemotherapy in resected non-small cell lung cancer. Ann Oncol (2017) 28(4):882–9. doi: 10.1093/annonc/mdx003
44. Cordonnier M, Nardin C, Chanteloup G, Derangere G, Algros M-P, Arnould L, et al. Tracking the evolution of circulating exosomal-PD-L1 to monitor melanoma patients. J Extracellular Vesicles (2020) 9(1):1710899. doi: 10.1080/20013078.2019.1710899
45. Theodoraki M-N, Yerneni SS, Hoffmann TK, Gooding WE, Whiteside TL. Clinical Significance of PD-L1(+) Exosomes in Plasma of Head and Neck Cancer Patients. Clin Cancer Res (2018) 24(4):896–905. doi: 10.1158/1078-0432.CCR-17-2664
46. Gu D, Ao X, Yang Y, Chen Z, Xu X. Soluble immune checkpoints in cancer: production, function and biological significance. J ImmunoTherapy Cancer (2018) 6(1):132. doi: 10.1186/s40425-018-0449-0
47. Omura Y, Toiyama Y, Okugawa Y, Yin C, Shigemori T, Kusunoki K, et al. Prognostic impacts of tumoral expression and serum levels of PD-L1 and CTLA-4 in colorectal cancer patients. Cancer Immunol Immunother CII (2020) 69(12):2533–46. doi: 10.1007/s00262-020-02645-1
48. Ji S, Chen H, Yang K, Zhang G, Mao B, Hu Y, et al. Peripheral cytokine levels as predictive biomarkers of benefit from immune checkpoint inhibitors in cancer therapy. Biomed Pharmacother = Biomed Pharmacother (2020) 129:110457. doi: 10.2139/ssrn.3578741
49. Fei Y, Yu J, Li Y, Li L, Zhou S, Zhang T, et al. Plasma soluble PD-L1 and STAT3 predict the prognosis in diffuse large B cell lymphoma patients. J Cancer (2020) 11(23):7001–8. doi: 10.7150/jca.47816
50. Ding Y, Sun C, Li J, Hu L, Li M, Lui J, et al. The Prognostic Significance of Soluble Programmed Death Ligand 1 Expression in Cancers: A Systematic Review and Meta-analysis. Scand J Immunol (2017) 86(5):361–7. doi: 10.1111/sji.12596
51. Wei W, Xu B, Wang Y, Wu C, Jiang J, Wu C. Prognostic significance of circulating soluble programmed death ligand-1 in patients with solid tumors: A meta-analysis. Med (Baltimore) (2018) 97(3):e9617. doi: 10.1097/MD.0000000000009617
Keywords: soluble programmed death ligand 1, cancers, prognosis, survival, meta-analysis
Citation: Huang P, Hu W, Zhu Y, Wu Y and Lin H (2021) The Prognostic Value of Circulating Soluble Programmed Death Ligand-1 in Cancers: A Meta-Analysis. Front. Oncol. 10:626932. doi: 10.3389/fonc.2020.626932
Received: 07 November 2020; Accepted: 30 December 2020;
Published: 25 February 2021.
Edited by:
Andrew Zloza, Rush University Medical Center, United StatesReviewed by:
Mingzhi Han, University of Bergen, NorwayChenkui Miao, Brigham and Women’s Hospital, United States
Copyright © 2021 Huang, Hu, Zhu, Wu and Lin. This is an open-access article distributed under the terms of the Creative Commons Attribution License (CC BY). The use, distribution or reproduction in other forums is permitted, provided the original author(s) and the copyright owner(s) are credited and that the original publication in this journal is cited, in accordance with accepted academic practice. No use, distribution or reproduction is permitted which does not comply with these terms.
*Correspondence: Huapeng Lin, linhuapeng11@163.com; Yushen Wu, arenasall@163.com