- 1Department of Radiotherapy, The Royal Marsden NHS Foundation Trust, London, United Kingdom
- 2University of California, Los Angeles, Los Angeles, CA, United States
- 3Radboud University Medical Center, Nijmegen, Netherlands
- 4Department of Radiotherapy and Imaging, Institute of Cancer Research, London, United Kingdom
External beam radiotherapy remains the primary treatment modality for localized prostate cancer. The radiobiology of prostate carcinoma lends itself to hypofractionation, with recent studies showing good outcomes with shorter treatment schedules. However, the ability to accurately deliver hypofractionated treatment is limited by current image-guided techniques. Magnetic resonance imaging is the main diagnostic tool for localized prostate cancer and its use in the therapeutic setting offers anatomical information to improve organ delineation. MR-guided radiotherapy, with daily re-planning, has shown early promise in the accurate delivery of radiotherapy. In this article, we discuss the shortcomings of current image-guidance strategies and the potential benefits and limitations of MR-guided treatment for prostate cancer. We also recount present experiences of MR-linac workflow and the opportunities afforded by this technology.
Introduction
Prostate cancer has accounted for 23.2% of all male cancer diagnoses in Europe in 2020 so far (1), a large proportion of whom will be treated with external beam radiotherapy (EBRT) for localized disease. EBRT offers patients non-invasive radical treatment and the move toward hypofractionation has allowed treatment schedules to be shortened. The low estimated α/β ratio of prostate cancer hypothesizes a benefit of hypofractionation, which has subsequently been evidenced in a number of trials (2–4) and transitioned into clinical practice guidelines across Europe (5–7) and America (8). Such results have encouraged clinicians to explore the boundaries of ultra-hypofractionation (UHF), testing 5 or 7 fraction schedules with promising oncological results (9–11).
Whilst the biology of prostate cancer may lend itself to hypofractionation, multiple obstacles remain in the pursuit of accurate dose delivery. Inter- and intra-fractional variability of target organ morphology and position as well as organ-at-risk (OAR) deformation limit the safety of dose escalation and hypofractionation with current image-guided radiotherapy (IGRT) techniques. The HYPO-RT-PC trial, comparing UHF for localized prostate cancer to conventional fractionation, reported significantly higher levels of patient-reported acute bowel and urinary toxicity with UHF (11), though late-term toxicity appeared equivalent regardless of treatment arm. However, these findings were not correlated in acute toxicity findings from the PACE-B trial in which the SBRT cohort reported similar levels of acute toxicity to the standard fractionation cohort (10). These differences may be due to radiotherapy technique, underlying the importance of optimizing dose delivery. Specifically, the radiation planning technique used for 80% of patients in the HYPO-RT-PC trial was three-dimensional conformal RT, rather than the more modern intensity-modulated RT, which has been associated with lower absolute rates of toxicity (11). Additionally, more generous planning margins were placed around the prostate to mitigate uncertainties due to prostate motion. Thus, the absolute rates of toxicity in the HYPO-RT-PC trial are likely higher than would be expected with modern treatment planning and delivery. Nonetheless, toxicity remains a possibility with all techniques and this remaining toxicity is likely determined not only by intrinsic radiosensitivity but also by doses delivered to critical adjacent organs.
The use of IGRT in prostate cancer is associated with improved biochemical control and lower rates of toxicity (12–14). MR-guided radiotherapy (MRgRT) brings IGRT to a higher level with improved soft tissue contrast and online adaptive planning allowing for greater accuracy of fraction delivery. MRgRT provides the opportunity to improve cancer outcomes while reducing treatment-related toxicity. Presently, there are two commercially available systems from which current experiences are drawn: Elekta Unity (Elekta AB, Stockholm, Sweden) which uses a 1.5 Tesla MRI machine, and Viewray MRIdian MR Linacs (Viewray Inc, Oakwood, OH) which uses a 0.35 Tesla MRI (15).
In this review, we will explore the shortcomings of current IGRT methods and the potential benefit and limitations of online adaptive MRgRT in prostate cancer. We will also describe current clinician experience of MR-guided workflow and the potential opportunities for future development and trials.
Shortcomings of Current IGRT Strategies
Current IGRT techniques include the use of cone-beam CT (CBCT) and implanted fiducial markers (FM), which may be used in conjunction; however, both have their limitations. CBCT alone has poor soft tissue resolution, limiting the accuracy of prostate-prostate matching (16). The use of radiopaque fiducials allows for rigid-registration but provides little to no information about organ deformation, seminal vesicle location, or bladder or rectal distension (17). The placement of fiducial markers is also an invasive procedure. Uncertainties in current IGRT strategies require larger planning margins to account for internal margin and set-up error, which can increase toxicity. Inter-fraction volumetric changes of the prostate gland have also been observed in moderate and profound hypofractionation schedules (18–20) and, with the move toward ultra-hypofractionation, direct visualization of the prostate serves to ensure dose coverage.
Any inter-fraction displacement necessitating contour repositioning is purely based on prostate matching and does not take into account the potential for differential movement of target organs such as seminal vesicles and pelvic lymph nodes (21, 22). Peng et al. analyzed 486 daily CT scans for 20 patients and found that in around 30% of fractions translational shifts were unable to adequately mitigate anatomical changes, indicating a need for online adaptive radiotherapy (ART) (23). While dosimetric coverage of the lymph node areas may be retained if bladder and rectal filling is pristinely maintained from fraction to fraction (24, 25), changes in anatomy could lead to overdosing of adjacent organs such as the small bowel. Furthermore, there is an increasing trend to dominant intraprostatic lesion boosts (26–28), which require additional accuracy in prostate matching adjustments on traditional kV planar or CBCT imaging.
Intra-fraction movement is an additional issue, which is sub-optimally mitigated by many current IGRT strategies. The prostate itself can move between image acquisition and beam on. Furthermore, bladder filling or rectal gas movement may influence target organ position by the order of a few millimeters, sufficient to affect CTV coverage. Both CT-based and MR-based analyses have demonstrated significant rates of intra-fractional motion. Calypso four-dimensional localization systems with the use of implanted electromagnetic markers showed prostate displacement of >3 mm 13.2% of the time during treatment (29). Similarly, three-dimensional cine MRI tracking of fiducials found prostate motion >2 mm in 43% scans by 5 min of treatment (30).
Any corrections to the field may be rendered inaccurate during beam on due to the aforementioned target position diversity (31–33), or otherwise clinicians must extend the planning margin to cover the expected excursion of prostate motion (34). A small number of non-MRgRT systems have intra-fraction motion solutions such as Cyberknife, which uses KV imaging tracking of fiducial seeds. During a fraction, which may take up to 45 min, fiducial seeds are tracked and adjustments to position can be made at 30–60 s intervals (35). However, systems for managing intra-fraction motion on the basis of fiducial markers require exposure to low doses of ionizing radiation.
Potential for Benefit With MRgRT for Prostate Cancer
MRI guidance with or without ART has multiple potential advantages in terms of improving accurate dose delivery. First, because the prostate is much better visualized on MRI images compared to CT images, prostate CTVs generated by MRI are smaller and more precise than CT-based contours (36). Figure 1 shows an image of the prostate from Unity. Typically, radiation-therapy planning MRIs are fused to CT simulation images to aid in contouring, but the fusion itself introduces 1–2 mm of residual error. Use of an MR-only workflow will bypass these issues. Second, on-board MRI imaging will allow direct tracking of the prostate, dispensing with the need for fiducials and sparing the patient an invasive procedure. Third, as a treatment course progresses, the daily image acquisition and adaptive re-planning allows for compensation related to prostate gland swelling, shrinkage, or deformation and inter-fractional motion of target or OARs. This daily sparing of OARs has the potential to decrease toxicity in both the short and long term. The ability to provide daily online adaptation minimizes inter-fraction uncertainty. Figure 2 shows a daily adaptive prostate plan from a 0.35T MR-linac.
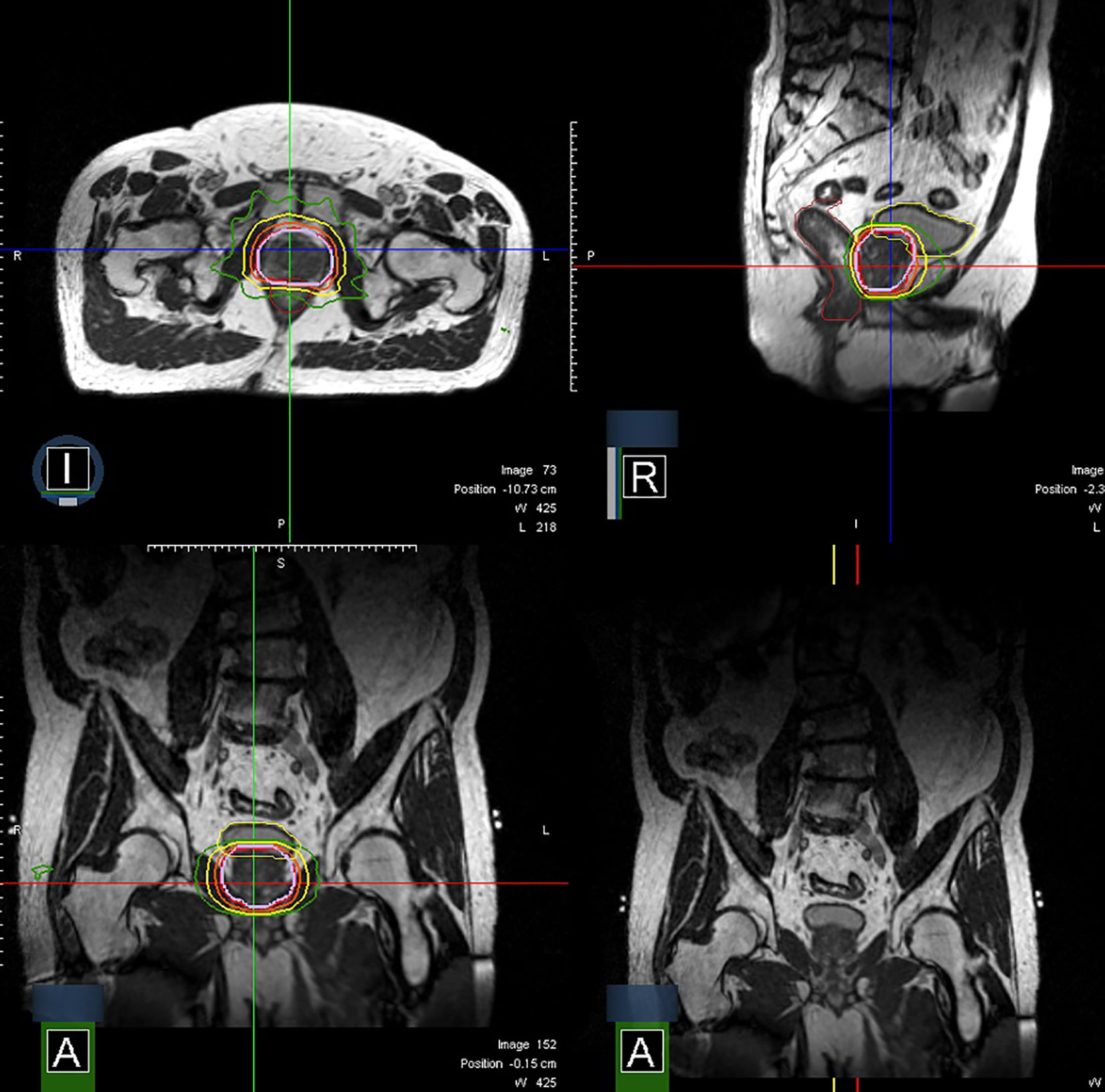
Figure 2 Axial, sagittal, and coronal images of a prostate plan on the MRIdian (isodoses: Red = 40 Gy, Orange = 36 Gy, Yellow = 24 Gy, Green = 20 Gy).
The workflow for the 1.5T MR-linac (Elekta Unity) is shown in Figure 3 and the 0.35T MR-linac (Viewray MRIdian) in Figure 4. On the 0.35T MR-linac, a high resolution (1.5 mm isotropic voxel size or better) scan will be taken utilizing the on-board MRI to establish target and OAR geometry at the time of treatment. If deemed necessary, online ART with daily re-planning can be performed. During treatment, real-time imaging is acquired using MRIs obtained in a single sagittal plane at 4 frames per second, with a gating boundary on the prostate CTV at the physician’s discretion. Tolerances for the proportion of the CTV outside of the gating boundary can be set, and 2-dimensional table shifts can be performed as per the physician’s discretion.
For the 1.5T MR-linac, the decision to perform daily re-planning rests on review of daily anatomy alone. If anatomy has changed, re-contouring precedes a full re-optimization of the plan. The acquisition of a verification image subsequent to contouring and planning allows for there to be a shift of the new plan immediately prior to beam on (called ‘Adapt-to-Position’ workflow) to account for any prostate motion, which occurs during the workflow. Typically, this is due to rectal or bladder filling.
In the future, the prospect of intra-fraction dose adaptation brings us closer to the ideal online adaptive dose delivery system (37), capable of achieving the optimal balance of target dose and OAR sparing during the entirety of beam on.
Current Experience of MRgRT in Prostate Cancer
Knowledge and experience of prostate MRgRT, on both Elekta Unity and Viewray MRIdian systems, has developed rapidly in the past few years. With MRgRT presenting a revolution in RT delivery, development of workflow and assessment of patient outcomes were initial priorities. Illustrative workflows are shown for the 1.5T MR-linac (Figure 3) and the 0.35T MR-linac systems (Figure 4). Such parameters were detailed by the Amsterdam VU team who described their experiences after 700 fractions were delivered (38).
MRgRT involves a multi-disciplinary team of radiographers, physicists, and clinicians. Most global experience is with daily re-contouring and re-planning. For example, the Amsterdam team reported that 97% of their delivered fractions were online ART plans (38).
The average duration of a delivered fraction is around 45 min, during, which time the patient is required to be on the treatment couch. The Amsterdam team also reported on a number of patient-reported outcomes and found that noise was the most common complaint (38, 39). Noise may be partially mitigated by the use of noise reduction headphones, which also enables communication between patients and radiation therapists during treatment (40). Our experience to date is that patients have not had any significant problems with the treatment, and patient experience is positive (41, 42). This is echoed by other practitioners including the group at VU University Medical Center (43).
Future studies about MR-linac clinical feasibility and patient toxicity outcomes are currently underway such as the Prostate Radiotherapy Integrated with Simultaneous MRI (PRISM study, NCT03658525), and the MOMENTUM study [The Multiple Outcome Evaluation of Radiotherapy Therapy Using the MR-linac Study (NCT04075305)] (44), which will help develop faster, more efficient workflows and benchmark multi-center patient outcomes. The ongoing Magnetic Resonance Imaging-Guided Stereotactic Body Radiotherapy for Prostate Cancer trial (MIRAGE trial, NCT04384770) is a phase III randomized study comparing standard CT-guided SBRT versus MRI-guided SBRT, with the primary endpoint of acute grade ≥2 genitourinary (GU) toxicity. It is designed as a superiority study, and secondary endpoints include patient-reported outcomes and late toxicity.
Published Literature on Prostate MRgRT
Outcomes for prostate radiotherapy are expected to be good for most patients, with generally low levels of side effects and high expectations of efficacy. For these patients, the benefit of MRgRT will be hard to show. However, small or marginal gains will have a high population effect due to the number of prostate cancer patients and the high likelihood of cure. There is a subset of patients with challenging anatomy where inferior dose distributions have to be accepted to preserve OAR integrity. Dosimetric improvement over a course of 20 fractions has been shown, with the number of fractions achieving all target dosimetric goals being 86% for MRgRT and 80% for simulated conventional IGRT (45). For one patient with exceptionally challenging anatomy, the prostate CTV D98% delivered was 54.5 Gy with MRgRT and would have been 49.9 Gy with conventional techniques over 20 fractions. Therefore, even though reductions in bowel and bladder toxicity will be challenging to show on a population level, this technology could meaningfully impact quality of life in those who will live for many years after cure.
Small clinical series describing experiences with MRgRT for prostate cancer have been published previously and provided detailed suggestions about the proposed benefits, challenges, and future development in this cancer type (46–48). To date, only one prospective study has published outcomes. Bruynzeel et al. (39) published early toxicity results from a phase II study on MRg-SBRT for localized prostate cancer, which reported on RTOG and CTCAE clinician-reported and patient-reported outcomes (PROMs) for 101 patients for 3 months post-treatment with 36.25 Gy in 5 fractions. Clinician-reported outcomes suggested early GI and GU toxicity peaked at the final fraction of treatment and no grade 3 or higher toxicities were reported. The rates of grade ≥2 early GU and GI toxicities at the end of the treatment were 19.8% and 3%, respectively. The maximum cumulative grade ≥2 early GU and GI toxicity (by 12 weeks) measured by any symptom at any study time point was 23.8% and 5.0%. Patient-reported outcomes correlated closely with clinician reported outcomes with urinary toxicity peaking at the end of treatment and resolving by 3 months. The most common GI symptom was bloating. As a comparison to the above study, the PACE-B trial (10) showed a cumulative (exceeding baseline) CTCAE grade ≥2 GU and GI toxicity of 27.4% and 15.3% in the 5-fraction arm.
Tetar et al. (49) recently provided an update on the VU series with toxicity information extending through one year of follow-up. No grade 3 or higher toxicities were reported. All symptoms returned to baseline by 12 months. International prostate symptom scores (IPSS) returned to baseline 6 months post-treatment. 2.2% of patients reported GI symptoms at 1 year follow-up. Follow-up is too short to evaluate oncologic efficacy.
It is too early to form robust toxicity comparisons between MRgRT and non-MRgRT SBRT trials, but outcomes encourage further prospective and long-term trials to interrogate this important point.
Limitations of MRgRT for Prostate Cancer
There are limitations to MRgRT for prostate cancer. The process of MRgRT provides a significant paradigm shift in the operation of radiotherapy departments, which necessitates updated safety training for staff, including all aspects of MR safety. Online ART requires the attention of several staff members for each treatment, often including a radiation oncologist, multiple radiographers and a physicist. Obviously, the person-hours required to deliver MRgRT are currently high when compared to traditional linac treatment, however efficiencies are likely to be forthcoming over time.
From a logistical point of view, there is limited availability of MR-linac machines and, as a result, clinician familiarity with such systems and online adaptive planning is still progressing. The predominance of radiation oncology experience until now has been centered on CT imaging and therefore the nuances and technicalities of MRI imaging are still being learned.
Maximum field size with 1.5T MR-linac machines could also lead to limitations of therapeutic capabilities and application in node-positive prostate cancer patients. With the current Unity maximum field size of 22cm in the superior-inferior (SI) plane, it is estimated that 80% of plans across cancer types would be suitable for MR-linac treatment (50) but a significant proportion of pelvic nodal irradiation fields would be too large. With the MRIdian Linac, the maximum field size is 24 cm SI, so a similar limitation applies. However, technical solutions to this limitation, and others, are being explored and current treatment possibilities do not represent the likely full capability of MR-linac machines (51). With prostate cancer predominantly affecting those over the age of 50, there is also likely to be a greater prevalence of medical contraindications to treatment on the MR-linac, thereby reducing numbers of suitable patients. From the patient perspective, the significantly longer time on the couch may deter some, and requires greater attention to patient comfort during treatment.
One element, which could reduce workload in the future of online ART, is automation of multiple components of the workflow. Auto-segmentation has been investigated and shown to decrease inter-observer variability while increasing dosimetric consistency on CT imaging (52, 53). This was replicated in MR-guided auto-delineation of pelvic organs although there has been evidence of poor concordance of auto-segmentation for targets such as seminal vesicles and the prostate (54–56). The creation of a library of contours and atlases from which an automated algorithm can learn will likely improve outcomes further. Currently, auto-generated contours are available for clinicians on both the 0.35T and 1.5T MR-linac machines and allow for a “warm-start contour” (i.e., not starting from scratch).
The duration of fraction delivery could also be aided by auto-segmentation. As mentioned, average duration of a single fraction for prostate cancer is around 45 min and this inevitably leads to greater bladder filling and variability of rectal distension, which have been shown to affect volume and position of the prostate and seminal vesicles to independent degrees (57). Current experiences are that intra-fraction OAR variation has not resulted in a significant number of adaptations required during beam on, although further published literature is required to confirm this. The role of auto-segmentation could reduce fraction duration and thereby minimize possible compromises to target organ dose delivery.
Another limitation of MRgRT is the risk of over-intervention with MRI imaging. The session MR image acquired at the beginning of each day’s treatment is a snapshot in time and one may devise a new plan based on that particular image with compromise of PTV coverage due to proximity of an OAR (e.g., bowel). OARs may move intra-fractionally (e.g., bowel peristalsis) and therefore may have unnecessarily compromised target coverage for that day.
Opportunities for Future Development of MRgRT in Prostate Cancer
While MRgRT provides hope for safe and effective dose delivery in prostate cancer treatment, further clinical studies are required to demonstrate a benefit.
Development of an MR-only, online workflow, without pre-treatment planning, would help to decrease radiotherapy pathway duration. Dispensing of the requirement for pre-treatment procedures, such as planning scans, would allow departments to condense pathways to benefit both clinicians and patients although acquisition of pre-treatment reference plans remains the standard in MR-only workflows currently (58). Removing the requirement to fuse planning CT to planning MRI would remove a potential source of error and uncertainty in the pathway. Although CT-based electron density calculations are considered to be the gold standard for radiotherapy planning, there are commercial MR-only solutions currently available, which may become more widely used (59).
Presently, operation of MRgRT requires a significant number of person-hours. Further streamlining of session times would be likely to result from incorporating auto-segmentation, as re-contouring is the most time-consuming component of the daily workflow. It remains to be seen if the accuracy of auto-delineation ever meets the standard set forth by radiation oncologists.
Amalgamation of roles within the inter-professional team may also reduce person-hours for treatment delivery. Inter-observer variation of MR contouring has shown good concordance (60) and is sure to lead to an evolution of roles within the MR-linac team starting with high volume, low complexity cases, which may become radiographer-led.
The predominant areas of opportunity lie within extreme hypofractionation in the online ART setting. Within the field of primary treatment of localized prostate cancer, ultra-hypofractionated SBRT schedules have been shown to be non-inferior to conventionally fractionated schedules (11). The increased levels of acute toxicity in the HYPO-RT-PC trial (11), and the lack of this in the PACE B trial (10), underline the importance of technical iteration to improve patient outcomes. Further studies to compare SBRT on traditional linac compared to MR-linac are under way, including the aforementioned phase III MIRAGE trial.
Many studies are undergoing to investigate possible superiority of dominant intraprostatic lesion (DIL) boosts (27). Doses of over 90 Gy equivalency have been shown to be safe (61, 62) but, as discussed above, our current IGRT strategies are imperfect for adapting to daily anatomical changes. Online ART using a 1.5T MR-linac would allow direct visualization of DILs during treatment. This is achievable on 1.5 T MR-linacs with diffusion scanning capabilities but, at present, 0.35T machines do not provide sufficient resolution to visualize DILs. Therefore rigid propagation is one option for this technique on a 0.35T MR-linac but alternative techniques to improve primary tumor visibility may be required; these have been employed in the diagnostic MR setting (63) but not as of yet in the therapeutic field. The feasibility of DIL visualization is also decreased with concomitant androgen deprivation therapy (62).
Other opportunities, beyond the scope of this review, include the use of MRgRT for post-operative prostatic bed irradiation or re-irradiation for radio-recurrent disease. The ability to provide more accurate dose-escalated treatment with direct visualization of tumor bulk has implications for post-prostatectomy relapses. The RADICALS-RT trial recently reported its 5 year results, which showed non-inferiority of salvage radiotherapy compared to adjuvant treatment (64). Currently, standard of practice is to treat the prostate bed empirically upon biochemical failure. The use of multi-parametric MRI (mpMRI) has been shown to be of use in detection of locally recurrent disease (65, 66); those with macroscopic disease on MRI could be triaged to treatment on the MR-linac with the possibility of macroscopic lesion boost (67, 68). In addition, as larger margins and a formulaic derivation of the target volume is currently used for prostate bed treatments, there is the prospect of reducing toxicity with MRgRT—one current phase II study promises to shed light on the efficacy and toxicity of MR-guided SBRT and CT-based SBRT delivered in the post-prostatectomy setting (NCT03541850). There are also few salvage treatments for locoregional recurrent disease after radical prostate EBRT. Early toxicity results of re-irradiation salvage SBRT are favourable (68–70) and further research into MR-guided salvage re-irradiation may be useful.
Qualitative and quantitative inter-fraction assessment of tumor response with functional MRI has implications for future treatment (71). The ability to directly visualize biological response to radiotherapy during a treatment course would allow the opportunity to tailor dose delivery. Online daily ART to target areas of persistent areas of restricted diffusion, for example, could possibly improve outcomes although implementation of functional imaging on MR-linac poses a number of challenges (72). For instance, there is a decrease in signal intensity of healthy prostate tissue on T2-weighted imaging during the course of treatment, which reduces visibility of the dominant intraprostatic lesion (73).
Thanks to the persistent and focused efforts of many prostate radiotherapy researchers over the last decade, significant GI and GU side effects of radiotherapy are becoming rarer. Effects of radiotherapy on sexual function are now the most prevalent long term side effect experienced by patients. The structures, which require dosimetric sparing in order to preserve sexual function, are not well elucidated, but it is thought that this is vascularly-mediated. Excellent outcomes have been seen after sparing the internal pudendal artery (74) using standard image-guidance strategies. As the vascular structures can be clearly seen on the MR-linac, it may be possible to preserve sexual function by sparing visualized vessels. Further study is planned.
As we progressively hypofractionate in prostate cancer, optimising image-guidance becomes ever-more important. Research is currently planned to investigate reducing the number of fractions below 5, to explore the limits of hypofractionation. The ONE SHOT trial aims to assess the efficacy of a 19 Gy fraction with 17Gy urethral sparing with a 2 mm margin. No grade 3 or higher GU and no grade 2 or higher GI toxicities were observed (75), although current HDR brachytherapy data suggests that a single fraction may be sub-optimal (76). Two-fraction HDR appears to have excellent outcomes and the MR-linac would be the perfect EBRT platform to test this in prostate cancer.
Conclusions
MRgRT presents a new paradigm shift in the delivery of prostate radiotherapy. Increasing accuracy of delivery and promising early experience will further encourage larger investigations of the benefit of MRgRT. The use of MRgRT could abolish the requirement for pre-planning and lead to shorter pathways, potentially with improved outcomes. Cohort randomized trials are needed and these will require collaboration between industry and academic partners to provide robust evidence for practice.
Author Contributions
BT composed the original draft and was responsible for incorporating alterations and produced a figure. AK, TM, and AT contributed figures and critically appraised the article. LK critically appraised the article. All authors contributed to the article and approved the submitted version.
Funding
This work was supported by Cancer Research UK grant C33589/A28284.
Conflict of Interest
AT receives institutional research funding from Elekta, Varian, and Accuray and has received honoraria/travel grants from Elekta. AK received research funding and honoraria from ViewRay, Inc., as well as honoraria and consulting fees from Varian Medical Systems, Inc. and consulting fees from Intelligent Automation, Inc.
The remaining authors declare that the research was conducted in the absence of any commercial or financial relationships that could be construed as a potential conflict of interest.
Acknowledgments
This project represents independent research supported by the National Institute for Health Research (NIHR) Biomedical Research Centre at The Royal Marsden Hospital and the Institute of Cancer Research, London. The views expressed are those of the authors (BT and AT) and not necessarily those of the NIHR or the Department of Health and Social Care. AK gratefully acknowledges support from the American Society for Radiation Oncology and the Prostate Cancer Foundation. We would like to thank Minsong Cao, PhD, for assistance with Figure 2. AT gratefully acknowledges the support of the Medical Research Council and the Rosetrees Trust.
References
1. European Comission. Estimates of cancer incidence and mortality in 2020, for all countries. Eur Com (2020). Available at: https://ecis.jrc.ec.europa.eu/explorer.php?$0-1$1-All$2-All$4-1,2$3-0$6-0,85$5-2004,2010$7-2$CRatesByRegistry$X0_10-ASR_EU_NEW
2. Dearnaley DP, Griffin C, Syndikus I, Khoo V, Birtle AJ, Choudhury A, et al. Eight-year outcomes of a phase III randomized trial of conventional versus hypofractionated high-dose intensity modulated radiotherapy for prostate cancer (CRUK/06/016): Update from the CHHiP Trial. J Clin Oncol (2020) 38:325. doi: 10.1200/JCO.2020.38.6_suppl.325
3. Lee WR, Dignam JJ, Amin MB, Bruner DW, Low D, Swanson GP, et al. Randomized Phase III Noninferiority Study Comparing Two Radiotherapy Fractionation Schedules in Patients With Low-Risk Prostate Cancer. J Clin Oncol (2016) 34:2325–32. doi: 10.1200/JCO.2016.67.0448
4. Catton CN, Lukka H, Gu CS, Martin JM, Supiot S, Chung PWM, et al. Randomized trial of a hypofractionated radiation regimen for the treatment of localized prostate cancer. J Clin Oncol (2017) 35:1884–90. doi: 10.1200/JCO.2016.71.7397
5. Mohler JL, Antonarakis ES, Armstrong AJ, D’Amico AV, Davis BJ, Dorff T, et al. Prostate Cancer, Version 2.2019, NCCN Clinical Practice Guidelines in Oncology. J Natl Compr Canc Netw (2019) 17:479–505. doi: 10.6004/jnccn.2019.0023
6. Mottet N, Bellmunt J, Bolla M, Briers E, Cumberbatch MG, De Santis M, et al. EAU-ESTRO-SIOG Guidelines on Prostate Cancer. Part 1: Screening, Diagnosis, and Local Treatment with Curative Intent. Eur Urol (2017) 71:618–29. doi: 10.1016/j.eururo.2016.08.003
7. Cornford P, Bellmunt J, Bolla M, Briers E, De Santis M, Gross T, et al. EAU-ESTRO-SIOG Guidelines on Prostate Cancer. Part II: Treatment of Relapsing, Metastatic, and Castration-Resistant Prostate Cancer. Eur Urol (2017) 71:630–42. doi: 10.1016/j.eururo.2016.08.002
8. Morgan SC, Hoffman K, Loblaw DA, Buyyounouski MK, Patton C, Barocas D, et al. Hypofractionated Radiation Therapy for Localized Prostate Cancer: Executive Summary of an ASTRO, ASCO, and AUA Evidence-Based Guideline. Pract Radiat Oncol (2018) 8:354–60. doi: 10.1016/j.prro.2018.08.002
9. Kishan AU, Dang A, Katz AJ, Mantz CA, Collins SP, Aghdam N, et al. Long-term Outcomes of Stereotactic Body Radiotherapy for Low-Risk and Intermediate-Risk Prostate Cancer. JAMA Netw Open (2019) 2:e188006. doi: 10.1001/jamanetworkopen.2018.8006
10. Brand DH, Tree AC, Ostler P, van der Voet H, Loblaw A, Chu W, et al. Intensity-modulated fractionated radiotherapy versus stereotactic body radiotherapy for prostate cancer (PACE-B): acute toxicity findings from an international, randomised, open-label, phase 3, non-inferiority trial. Lancet Oncol (2019) 20:1531–43. doi: 10.1016/S1470-2045(19)30569-8
11. Widmark A, Gunnlaugsson A, Beckman L, Thellenberg-Karlsson C, Hoyer M, Lagerlund M, et al. Ultra-hypofractionated versus conventionally fractionated radiotherapy for prostate cancer: 5-year outcomes of the HYPO-RT-PC randomised, non-inferiority, phase 3 trial. Lancet (2019) 394:385–95. doi: 10.1016/S0140-6736(19)31131-6
12. Singh J, Greer PB, White MA, Parker J, Patterson J, Tang CI, et al. Treatment-Related Morbidity in Prostate Cancer: A Comparison of 3-Dimensional Conformal Radiation Therapy With and Without Image Guidance Using Implanted Fiducial Markers. Int J Radiat Oncol Biol Phys (2013) 85:1018–23. doi: 10.1016/j.ijrobp.2012.07.2376
13. Gill S, Thomas J, Fox C, Kron T, Rolfo A, Leahy M, et al. Acute toxicity in prostate cancer patients treated with and without image-guided radiotherapy. Radiat Oncol (2011) 6:145. doi: 10.1186/1748-717X-6-145
14. Zelefsky MJ, Kollmeier M, Cox B, Fidaleo A, Sperling D, Pei X, et al. Improved Clinical Outcomes With High-Dose Image Guided Radiotherapy Compared With Non-IGRT for the Treatment of Clinically Localized Prostate Cancer. Int J Radiat Oncol (2012) 84:125–9. doi: 10.1016/j.ijrobp.2011.11.047
15. Ménard C, van der Heide U. Introduction: Systems for Magnetic Resonance Image Guided Radiation Therapy. Semin Radiat Oncol (2014) 24:192. doi: 10.1016/j.semradonc.2014.02.010
16. Morrow NV, Lawton CA, Qi XS, Li XA. Impact of computed tomography image quality on image-guided radiation therapy based on soft tissue registration. Int J Radiat Oncol Biol Phys (2012) 82:e733–8. doi: 10.1016/j.ijrobp.2011.11.043
17. Deegan T, Owen R, Holt T, Fielding A, Biggs J, Parfitt M, et al. Assessment of cone beam CT registration for prostate radiation therapy: Fiducial marker and soft tissue methods. J Med Imaging Radiat Oncol (2015) 59:91–98. doi: 10.1111/1754-9485.12197
18. Sanguineti G, Marcenaro M, Franzone P, Foppiano F, Vitale V. Neoadjuvant androgen deprivation and prostate gland shrinkage during conformal radiotherapy. Radiother Oncol (2003) 66:151–7. doi: 10.1016/S0167-8140(03)00031-8
19. Nichol AM, Brock KK, Lockwood GA, Moseley DJ, Rosewall T, Warde PR, et al. A magnetic resonance imaging study of prostate deformation relative to implanted gold fiducial markers. Int J Radiat Oncol Biol Phys (2007) 67:48–56. doi: 10.1016/j.ijrobp.2006.08.021
20. Gunnlaugsson A, Kjellén E, Hagberg O, Thellenberg-Karlsson C, Widmark A, Nilsson P. Change in prostate volume during extreme hypo-fractionation analysed with MRI. Radiat Oncol (2014) 9:22. doi: 10.1186/1748-717X-9-22
21. Gill S, Dang K, Fox C, Bressel M, Kron T, Bergen N, et al. Seminal vesicle intrafraction motion analysed with cinematic magnetic resonance imaging. Radiat Oncol (2014) 9:174. doi: 10.1186/1748-717X-9-174
22. Graf R, Boehmer D, Budach V, Wust P. Interfraction rotation of the prostate as evaluated by kilovoltage X-ray fiducial marker imaging in intensity-modulated radiotherapy of localized prostate cancer. Med Dosim (2012) 37:396–400. doi: 10.1016/j.meddos.2012.02.006
23. Peng C, Ahunbay E, Chen G, Anderson S, Lawton C, Li XA. Characterizing interfraction variations and their dosimetric effects in prostate cancer radiotherapy. Int J Radiat Oncol Biol Phys (2011) 79:909–14. doi: 10.1016/j.ijrobp.2010.05.008
24. Kishan AU, Lamb JM, Jani SS, Kang JJ, Steinberg ML, King CR. Pelvic nodal dosing with registration to the prostate: Implications for high-risk prostate cancer patients receiving stereotactic body radiation therapy. Int J Radiat Oncol Biol Phys (2015) 91:832–9. doi: 10.1016/j.ijrobp.2014.11.035
25. Kishan AU, Tyran M, Weng J, Upadhyaya S, Lamb J, Steinberg M, et al. Stereotactic body radiotherapy to the prostate and pelvic lymph nodes: A detailed dosimetric analysis of a phase II prospective trial. Br J Radiol (2019) 92:20181001. doi: 10.1259/bjr.20181001
26. Draulans C, van der Heide UA, Haustermans K, Pos FJ, van der Voort van Zyp J, De Boer H, et al. Primary endpoint analysis of the multicentre phase II hypo-FLAME trial for intermediate and high risk prostate cancer. Radiother Oncol (2020) 147:92–98. doi: 10.1016/j.radonc.2020.03.015
27. Monninkhof EM, van Loon JWL, van Vulpen M, Kerkmeijer LGW, Pos FJ, Haustermans K, et al. Standard whole prostate gland radiotherapy with and without lesion boost in prostate cancer: Toxicity in the FLAME randomized controlled trial. Radiother Oncol (2018) 127:74–80. doi: 10.1016/j.radonc.2017.12.022
28. Murray JR, Tree AC, Alexander EJ, Sohaib A, Hazell S, Thomas K, et al. Standard and Hypofractionated Dose Escalation to Intraprostatic Tumor Nodules in Localized Prostate Cancer: Efficacy and Toxicity in the DELINEATE Trial. Int J Radiat Oncol Biol Phys (2020) 106:715–24. doi: 10.1016/j.ijrobp.2019.11.402
29. Langen KM, Willoughby TR, Meeks SL, Santhanam A, Cunningham A, Levine L, et al. Observations on Real-Time Prostate Gland Motion Using Electromagnetic Tracking. Int J Radiat Oncol Biol Phys (2008) 71:1084–90. doi: 10.1016/j.ijrobp.2007.11.054
30. De Muinck Keizer DM, Pathmanathan AU, Andreychenko A, Kerkmeijer LGW, Van Der Voort Van Zyp JRN, Tree AC, et al. Fiducial marker based intra-fraction motion assessment on cine-MR for MR-linac treatment of prostate cancer. Phys Med Biol (2019) 64:07NT02. doi: 10.1088/1361-6560/ab09a6
31. Budiharto T, Slagmolen P, Haustermans K, Maes F, Junius S, Verstraete J, et al. Intrafractional prostate motion during online image guided intensity-modulated radiotherapy for prostate cancer. Radiother Oncol (2011) 98:181–6. doi: 10.1016/j.radonc.2010.12.019
32. Badakhshi H, Wust P, Budach V, Graf R. Image-guided radiotherapy with implanted markers and kilovoltage imaging and 6-dimensional position corrections for intrafractional motion of the prostate. Anticancer Res (2013) 33:4117–21.
33. Tong X, Chen X, Li J, Xu Q, Lin MH, Chen L, et al. Intrafractional prostate motion during external beam radiotherapy monitored by a real-time target localization system. J Appl Clin Med Phys (2015) 16:51–61. doi: 10.1120/jacmp.v16i2.5013
34. Levin-Epstein R, Qiao-Guan G, Juarez JE, Shen Z, Steinberg ML, Ruan D, et al. Clinical Assessment of Prostate Displacement and Planning Target Volume Margins for Stereotactic Body Radiotherapy of Prostate Cancer. Front Oncol (2020) 10:539. doi: 10.3389/fonc.2020.00539
35. Katz AJ, Kang J. Stereotactic Body Radiotherapy as Treatment for Organ Confined Low- and Intermediate-Risk Prostate Carcinoma, a 7-Year Study. Front Oncol (2014) 4:240. doi: 10.3389/fonc.2014.00240
36. Pathmanathan AU, Schmidt MA, Brand DH, Kousi E, van As NJ, Tree AC. Improving fiducial and prostate capsule visualization for radiotherapy planning using MRI. J Appl Clin Med Phys (2019) 20:27–36. doi: 10.1002/acm2.12529
37. Kontaxis C, Bol GH, Lagendijk JJW, Raaymakers BW. A new methodology for inter- and intrafraction plan adaptation for the MR-linac. Phys Med Biol (2015) 60:7485–97. doi: 10.1088/0031-9155/60/19/7485
38. Tetar SU, Bruynzeel AME, Lagerwaard FJ, Slotman BJ, Bohoudi O, Palacios MA. Clinical implementation of magnetic resonance imaging guided adaptive radiotherapy for localized prostate cancer. Phys Imaging Radiat Oncol (2019) 9:69–76. doi: 10.1016/j.phro.2019.02.002
39. Bruynzeel AME, Tetar SU, Oei SS, Senan S, Haasbeek CJA, Spoelstra FOB, et al. A Prospective Single-Arm Phase 2 Study of Stereotactic Magnetic Resonance Guided Adaptive Radiation Therapy for Prostate Cancer: Early Toxicity Results. Int J Radiat Oncol Biol Phys (2019) 105:1086–94. doi: 10.1016/j.ijrobp.2019.08.007
40. Botman R, Tetar SU, Palacios MA, Slotman BJ, Lagerwaard FJ, Bruynzeel AME. The clinical introduction of MR-guided radiation therapy from a RTT perspective. Clin Transl Radiat Oncol (2019) 18:140–5. doi: 10.1016/j.ctro.2019.04.019
41. Alongi F, Rigo M, Figlia V, Cuccia F, Giaj-Levra N, Nicosia L, et al. 1.5 T MR-guided and daily adapted SBRT for prostate cancer: feasibility, preliminary clinical tolerability, quality of life and patient-reported outcomes during treatment. Radiat Oncol (2020) 15:69. doi: 10.1186/s13014-020-01510-w
42. Bertelsen AS, Schytte T, Møller PK, Mahmood F, Riis HL, Gottlieb KL, et al. First clinical experiences with a high field 1.5 T MR linac. Acta Oncol (Madr) (2019) 58:1352–7. doi: 10.1080/0284186X.2019.1627417
43. Tetar S, Bruynzeel A, Bakker R, Jeulink M, Slotman BJ, Oei S, et al. Patient-reported Outcome Measurements on the Tolerance of Magnetic Resonance Imaging-guided Radiation Therapy. Cureus (2018) 10:e2236–6. doi: 10.7759/cureus.2236
44. de Mol van Otterloo SR, Christodouleas JP, Blezer ELA, Akhiat H, Brown K, Choudhury A, et al. The MOMENTUM Study: An International Registry for the Evidence-Based Introduction of MR-Guided Adaptive Therapy. Front Oncol (2020) 10:1328. doi: 10.3389/fonc.2020.01328
45. Dunlop A, Mitchell A, Tree A, Barnes H, Bower L, Chick J, et al. Daily adaptive radiotherapy for patients with prostate cancer using a high field MR-linac: Initial clinical experiences and assessment of delivered doses compared to a C-arm linac. Clin Transl Radiat Oncol (2020) 23:35–42. doi: 10.1016/j.ctro.2020.04.011
46. Pathmanathan AU, van As NJ, Kerkmeijer LGW, Christodouleas J, Lawton CAF, Vesprini D, et al. Magnetic Resonance Imaging-Guided Adaptive Radiation Therapy: A “Game Changer” for Prostate Treatment? Int J Radiat Oncol Biol Phys (2018) 100:361–73. doi: 10.1016/j.ijrobp.2017.10.020
47. Murray J, Tree AC. Prostate cancer – Advantages and disadvantages of MR-guided RT. Clin Transl Radiat Oncol (2019) 18:68–73. doi: 10.1016/j.ctro.2019.03.006
48. McPartlin AJ, Li XA, Kershaw LE, Heide U, Kerkmeijer L, Lawton C, et al. MRI-guided prostate adaptive radiotherapy – A systematic review. Radiother Oncol (2016) 119:371–80. doi: 10.1016/j.radonc.2016.04.014
49. Tetar SU, Bruynzeel AME, Oei SS, Senan S, Fraikin T, Slotman BJ, et al. Magnetic Resonance-guided Stereotactic Radiotherapy for Localized Prostate Cancer: Final Results on Patient-reported Outcomes of a Prospective Phase 2 Study. Eur Urol Oncol (2020). doi: 10.1016/j.euo.2020.05.007
50. Chuter RW, Whitehurst P, Choudhury A, Van Herk M, McWilliam A. Technical Note: Investigating the impact of field size on patient selection for the 1.5T MR-Linac. Med Phys (2017) 44:5667–71. doi: 10.1002/mp.12557
51. Kerkmeijer LGW, Fuller CD, Verkooijen HM, Verheij M, Choudhury A, Harrington KJ, et al. The MRI-linear accelerator consortium: Evidence-based clinical introduction of an innovation in radiation oncology connecting researchers, methodology, data collection, quality assurance, and technical development. Front Oncol (2016) 6. doi: 10.3389/fonc.2016.00215
52. Hwee J, Louie AV, Gaede S, Bauman G, D’Souza D, Sexton T, et al. Technology assessment of automated atlas based segmentation in prostate bed contouring. Radiat Oncol (2011) 6:110. doi: 10.1186/1748-717X-6-110
53. Tao CJ, Yi JL, Chen NY, Ren W, Cheng J, Tung S, et al. Multi-subject atlas-based auto-segmentation reduces interobserver variation and improves dosimetric parameter consistency for organs at risk in nasopharyngeal carcinoma: A multi-institution clinical study. Radiother Oncol (2015) 115:407–11. doi: 10.1016/j.radonc.2015.05.012
54. Greenham S, Dean J, Fu CKK, Goman J, Mulligan J, Tune D, et al. Evaluation of atlas-based auto-segmentation software in prostate cancer patients. J Med Radiat Sci (2014) 61:151–8. doi: 10.1002/jmrs.64
55. Pasquier D, Lacornerie T, Vermandel M, Rousseau J, Lartigau E, Betrouni N. Automatic Segmentation of Pelvic Structures From Magnetic Resonance Images for Prostate Cancer Radiotherapy. Int J Radiat Oncol Biol Phys (2007) 68:592–600. doi: 10.1016/j.ijrobp.2007.02.005
56. Klein S, Van Der Heide UA, Lips IM, Van Vulpen M, Staring M, Pluim JPW. Automatic segmentation of the prostate in 3D MR images by atlas matching using localized mutual information. Med Phys (2008) 35:1407–17. doi: 10.1118/1.2842076
57. Sevak PR, Nejad-Davarani S, Kim J, Weiss S, Elshaikh MA, Glide-Hurst CK. Intrafractional Motion of Target Volumes and Organs at Risk Due to Bladder Filling: Implications for MR-Only Prostate Radiation Therapy. Int J Radiat Oncol Biol Phys (2017) 99:E720–1. doi: 10.1016/j.ijrobp.2017.06.2336
58. Tyagi N, Fontenla S, Zelefsky M, Chong-Ton M, Ostergren K, Shah N, et al. Clinical workflow for MR-only simulation and planning in prostate. Radiat Oncol (2017) 12:119. doi: 10.1186/s13014-017-0854-4
59. Schmidt MA, Payne GS. Radiotherapy planning using MRI. Phys Med Biol (2015) 60:R323–61. doi: 10.1088/0031-9155/60/22/r323
60. Pathmanathan AU, McNair HA, Schmidt MA, Brand DH, Delacroix L, Eccles CL, et al. Comparison of prostate delineation on multimodality imaging for MR-guided radiotherapy. Br J Radiol (2019) 92:20180948. doi: 10.1259/bjr.20180948
61. Von Eyben FE, Kiljunen T, Kangasmaki A, Kairemo K, Von Eyben R, Joensuu T. Radiotherapy Boost for the Dominant Intraprostatic Cancer Lesion - A Systematic Review and Meta-Analysis. Clin Genitourin Cancer (2016) 14:189–97. doi: 10.1016/j.clgc.2015.12.005
62. Hötker AM, Mazaheri Y, Zheng J, Moskowitz CS, Berkowitz J, Lantos JE, et al. Prostate Cancer: assessing the effects of androgen-deprivation therapy using quantitative diffusion-weighted and dynamic contrast-enhanced MRI. Eur Radiol (2015) 25:2665–72. doi: 10.1007/s00330-015-3688-1
63. Daniel M, Kuess P, Andrzejewski P, Nyholm T, Helbich T, Polanec S, et al. Impact of androgen deprivation therapy on apparent diffusion coefficient and T2w MRI for histogram and texture analysis with respect to focal radiotherapy of prostate cancer. Strahlentherapie und Onkol (2019) 195:402–11. doi: 10.1007/s00066-018-1402-3
64. Parker CC, Clarke NW, Cook AD, Kynaston HG, Petersen PM, Catton C, et al. Timing of radiotherapy after radical prostatectomy (RADICALS-RT): a randomised, controlled phase 3 trial. Lancet (2020) 396:1413–21. doi: 10.1016/S0140-6736(20)31553-1
65. Barchetti F, Panebianco V. Multiparametric MRI for recurrent prostate cancer post radical prostatectomy and postradiation therapy. BioMed Res Int (2014) 2014:1–23. doi: 10.1155/2014/316272
66. Panebianco V, Barchetti F, Sciarra A, Musio D, Forte V, Gentile V, et al. Prostate cancer recurrence after radical prostatectomy: The role of 3-T diffusion imaging in multi-parametric magnetic resonance imaging. Eur Radiol (2013) 23:1745–52. doi: 10.1007/s00330-013-2768-3
67. Olivier J, Basson L, Puech P, Lacornerie T, Villers A, Wallet J, et al. Stereotactic re-irradiation for local recurrence in the prostatic bed after prostatectomy: Preliminary results. Front Oncol (2019) 9. doi: 10.3389/fonc.2019.00071
68. Detti B, Bonomo P, Masi L, Doro R, Cipressi S, Iermano C, et al. CyberKnife stereotactic radiotherapy for isolated recurrence in the prostatic bed. World J Urol (2016) 34:311–7. doi: 10.1007/s00345-015-1613-5
69. Arcangeli S, Agolli L, Donato V. Retreatment for prostate cancer with stereotactic body radiation therapy (SBRT): Feasible or foolhardy? Rep Pract Oncol Radiother (2015) 20:425–9. doi: 10.1016/j.rpor.2014.08.001
70. Pasquier D, Martinage G, Janoray G, Rojas DP, Zerini D, Goupy F, et al. Salvage Stereotactic Body Radiation Therapy for Local Prostate Cancer Recurrence After Radiation Therapy: A Retrospective Multicenter Study of the GETUG. Int J Radiat Oncol Biol Phys (2019) 105:727–34. doi: 10.1016/j.ijrobp.2019.07.012
71. Datta A, Aznar MC, Dubec M, Parker GJM, O’Connor JPB. Delivering Functional Imaging on the MRI-Linac: Current Challenges and Potential Solutions. Clin Oncol (2018) 30:702–10. doi: 10.1016/j.clon.2018.08.005
72. Guimaraes MD, Schuch A, Hochhegger B, Gross JL, Chojniak R, Marchiori E, et al. Functional magnetic resonance imaging in oncology: state of the art. Radiol Bras (2014) 47:101–11. doi: 10.1590/S0100-39842014000200013
73. van Schie MA, van Houdt PJ, Ghobadi G, Pos FJ, Walraven I, de Boer HCJ, et al. Quantitative MRI Changes During Weekly Ultra-Hypofractionated Prostate Cancer Radiotherapy With Integrated Boost. Front Oncol (2019) 9. doi: 10.3389/fonc.2019.01264
74. Spratt DE, Lee JY, Dess RT, Narayana V, Evans C, Liss A, et al. Vessel-sparing Radiotherapy for Localized Prostate Cancer to Preserve Erectile Function: A Single-arm Phase 2 Trial. Eur Urol (2017) 72:617–24. doi: 10.1016/j.eururo.2017.02.007
75. Zilli T, Franzese C, Bottero M, Giaj-Levra N, Förster R, Zwahlen D, et al. Single fraction urethra-sparing prostate cancer SBRT: Phase I results of the ONE SHOT trial. Radiother Oncol (2019) 139:83–6. doi: 10.1016/j.radonc.2019.07.018
76. Morton G, McGuffin M, Chung HT, Tseng C-L, Helou J, Ravi A, et al. Prostate high dose-rate brachytherapy as monotherapy for low and intermediate risk prostate cancer: Efficacy results from a randomized phase II clinical trial of one fraction of 19 Gy or two fractions of 13.5 Gy. Radiother Oncol (2020) 146:90–6. doi: 10.1016/j.radonc.2020.02.009
Keywords: prostate cancer, MR-linac, image-guided radiotherapy, online adaptive radiotherapy, MR-guided radiotherapy
Citation: Tocco BR, Kishan AU, Ma TM, Kerkmeijer LGW and Tree AC (2020) MR-Guided Radiotherapy for Prostate Cancer. Front. Oncol. 10:616291. doi: 10.3389/fonc.2020.616291
Received: 11 October 2020; Accepted: 09 November 2020;
Published: 09 December 2020.
Edited by:
Alessio Bruni, University Hospital of Modena, ItalyReviewed by:
Andrea Vavassori, European Institute of Oncology (IEO), ItalyNiccolo Giaj Levra, Sacro Cuore Don Calabria Hospital, Italy
Copyright © 2020 Tocco, Kishan, Ma, Kerkmeijer and Tree. This is an open-access article distributed under the terms of the Creative Commons Attribution License (CC BY). The use, distribution or reproduction in other forums is permitted, provided the original author(s) and the copyright owner(s) are credited and that the original publication in this journal is cited, in accordance with accepted academic practice. No use, distribution or reproduction is permitted which does not comply with these terms.
*Correspondence: Boris R. Tocco, Yi50b2Njb0BuaHMubmV0