- 1Nuclear Medicine Department, Henri Becquerel Cancer Center, Rouen, France
- 2QuantIF-LITIS EA4108, University of Rouen, Rouen, France
- 3Radiotherapy Department, Henri Becquerel Cancer Center, Rouen, France
Computed tomography (CT) has revolutionized external radiotherapy by making it possible to visualize and segment the tumors and the organs at risk in a three-dimensional way. However, if CT is a now a standard, it presents some limitations, notably concerning tumor characterization and delineation. Its association with functional and anatomical images, that are positron emission tomography (PET) and magnetic resonance imaging (MRI), surpasses its limits. This association can be in the form of a trimodality PET/CT/MRI. The objective of this mini-review is to describe the process of performing this PET/CT/MRI trimodality for radiotherapy and its potential clinical applications. Trimodality can be performed in two ways, either a PET/MRI fused to a planning CT (possibly with a pseudo-CT generated from the MRI for the planning), or a PET/CT fused to an MRI and then registered to a planning CT (possibly the CT of PET/CT if calibrated for radiotherapy). These examinations should be performed in the treatment position, and in the second case, a patient transfer system can be used between the PET/CT and MRI to limit movement. If trimodality requires adapted equipment, notably compatible MRI equipment with high-performance dedicated coils, it allows the advantages of the three techniques to be combined with a synergistic effect while limiting their disadvantages when carried out separately. Trimodality is already possible in clinical routine and can have a high clinical impact and good inter-observer agreement, notably for head and neck cancers, brain tumor, prostate cancer, cervical cancer.
Introduction
External radiotherapy consists of treating an internal lesion, superficial and/or external lesion with an external source of radiation. In the nineties, computed tomography (CT) has revolutionized external radiotherapy by making it possible to visualize the tumor(s), corresponding to the target volume, and the organs at risk (OARs), which are normal tissues whose sensitivity to radiation can significantly influence treatment planning and/or prescribed dose. Because the treatment beams can be afterwards individually oriented on the tumor in a three-dimensional (3D) approach, this marked the beginning of 3D conformal radiotherapy (1).
A following improvement was the control of the intensity of the treatment beams which opened in the new millennium the era of the intensity-modulated radiotherapy (IMRT) whose aim is to deliver a high dose to the target volume while sparing the adjacent tissues, notably OARs (2). With this technique, a homogeneous dose is prescribed to the planning target volume (PTV) that considers uncertainty in treatment planning by encompassing the gross tumor volume (GTV, corresponding to the delineated macroscopic and radiologically measurable tumor) and the clinical target volume (CTV, which adds a margin to the GTV to cover nearby areas at risk of hosting microscopic disease) (3). The accuracy of anatomical localization is of particular importance for stereotactic radiotherapy (SRT), corresponding to an external beam radiotherapy used to deliver a high dose of radiation very precisely, as a single dose or a small number of fraction (4).
However, if CT imaging is a now a standard for radiotherapy, it presents some limitations, notably concerning tumor delineation which can be difficult, especially for soft tissues (3) or for the characterization of the lesions. Other 3D imaging modalities have therefore emerged for radiotherapy, in particular, positron emission tomography (PET) and magnetic resonance imaging (IRM). PET and MRI can notably visualize biological processes distinct and complementary to purely anatomical imaging. This led to the concept of biological target volume (BTV) focused on a metabolic function. For example, a boost radiotherapy can be performed on hypoxic tumors more resistant to radiation, identified by 18F-fluoromisonidazole (FMISO) PET/CT (5). Finally, CT, PET, and MRI can be used to follow the patient during the radiotherapy, at the end of the treatment, or during the follow-up (6)
If these 3D imaging modalities (CT, PET, and MRI) can be considered separately, they can also be associated to form a hybrid imaging, two by two (PET/CT, CT/MRI, PET/MRI) but also as a trimodality PET/CT/MRI. Multimodality is already possible in clinical routine with a high clinical impact and good inter-observer agreement (7).
The aim of this mini-review is to present the concept of PET/CT/MRI trimodality, its rationale for radiotherapy, and its potential interest in characterizing tumor, performing treatment planning, and doing ART.
Technical Parts
CT, MRI, and PET
CT is the reference imaging used by radiation oncologists for target volumes and organs at risk delineation for radiotherapy treatment planning. It is a high spatial resolution imaging modality that provides anatomical information with good spatial accuracy which is unaffected by geometric distortions. CT also provides a mapping of tissue electron density necessary for dosimetric calculations in radiotherapy. However, CT is an irradiating imaging modality with certain disadvantages such as lack of contrast in soft tissue and artifacts due to the presence of metal (8).
MRI is an anatomical and functional imaging modality that provides very good soft tissue contrast with millimetric spatial resolution. Although it has the advantage of being non-irradiating, the acquisition process is time consuming and this technique presents many contraindications (9). The possibility of using only MRI for radiotherapy treatment planning is however limited by the absence of information on tissue electronic density, a non-constant intensity of the images, and the presence of geometric distortions that deform images, including the volumes of interest.
CT and MRI are often associated with PET, a functional imaging modality. It provides a very good tumor/node contrast and the possibility to acquire large field of view. However, it is an irradiating examination with poor spatial resolution. In addition, the presence of partial volume artifacts creates blurred edges making more difficult the segmentation of volumes of interest.
Therefore, trimodality appears to be a technique of choice in the treatment of cancer in radiotherapy. It provides anatomical and functional information of high spatial resolution and allows improving the definition of target volumes in radiotherapy (10–12). A summary of the advantages and disadvantages of CT, MRI, and PET separately and combined in PET/CT/MRI is presented in Table 1.
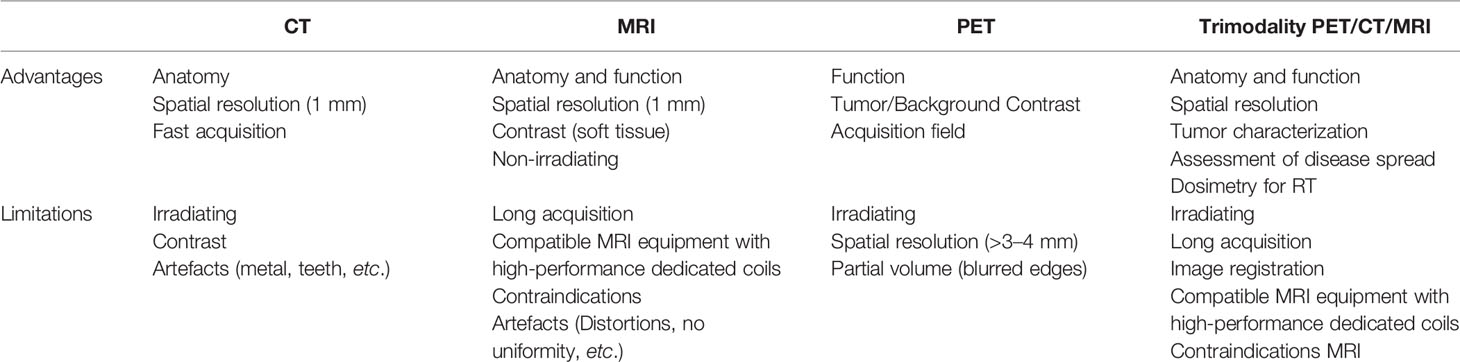
Table 1 summary of the advantages and disadvantages of CT, MRI and PET separately and combined in PET/CT/MRI.
Trimodality PET/CT/MRI
As trimodality allows obtaining additional information on disease and tumors; images of each modality must be performed in radiotherapy treatment position (13). Each machine is equipped with a rigid table that is positioned on the device table. Each of these rigid tables has markers and an indexing system that allows fixing radiotherapy immobilization solutions. In order to have exactly the same position for all acquisitions, it is essential that the equipment used is compatible with all the installations and in particular non-magnetic equipment for MR. Patient repositioning on each device is done using markers on the skin (i.e. the positioning referential) made during the planning CT and external positioning lasers.
Currently, no medical device allows simultaneous acquisition of all three imaging modalities. The solution is to use two separate imaging devices, a bimodal hybrid machine and an independent machine. Two trimodality systems are possible: a PET/MRI coupled with a CT or a PET/CT coupled with an MRI (14). For each of them, image registration will be indispensable to delineate volumes of interest and to perform radiotherapy treatment planning (15).
For the solution with PET/MR, precautions must be taken for data acquisition and processing. The patient is positioned on the device with MR coils compatible with radiotherapy immobilization fixations (16). To perform attenuation correction on the PET image, an attenuation mapping of MR coils must be performed before (17). The PET/MR images and the planning CT are then registered before volume delineation and dosimetric planning. An alternative to this solution is to replace the planning CT by a synthetic CT, commonly referred as pseudo-CT (18, 19). With the emergence of artificial intelligence, new robust algorithms such as GANs (Generative Adversial Networks) (20, 21) allow the creation of attenuation maps, synthetic CT, from the different MR images. Treatment planning can then be performed without proceeding to the image registration step.
The second PET/CT + MRI solution is performed following the same process; the patient has these two examinations one after the other in the radiotherapy treatment position. Two techniques can be used for this PET/CT + MRI workflow. The first is to use a transfer system compatible with both imaging devices (22). This consists of an air cushion bed with low attenuation and a non-magnetic stretcher that allows the bed to be moved from one device to the other without moving the patient. The air-cushioned bed is placed on the rigid tabletop of the first imaging device, and the patient is positioned in a position in agreement with the positioning referential realized for planning CT. At the end of the acquisition, the patient on the air-cushioned bed is moved to the stretcher with the help of a suction system and is then transferred to the second imaging unit from the stretcher to the examination table using the same suction system. In the end, the system allows the realization of multi-modal acquisitions while keeping the patient in the same position.
For the second technique, the PET/CT and MRI images are also performed in radiotherapy planning conditions, but the patient stands up between the two acquisitions. The patient is positioned on the first imager in radiotherapy treatment conditions using markers determined during planning CT acquisition and external lasers. He is then positioned in the same conditions to the second device. A summary of the methods of achieving PET/CT/MRI trimodality for radiotherapy is presented in Figure 1.
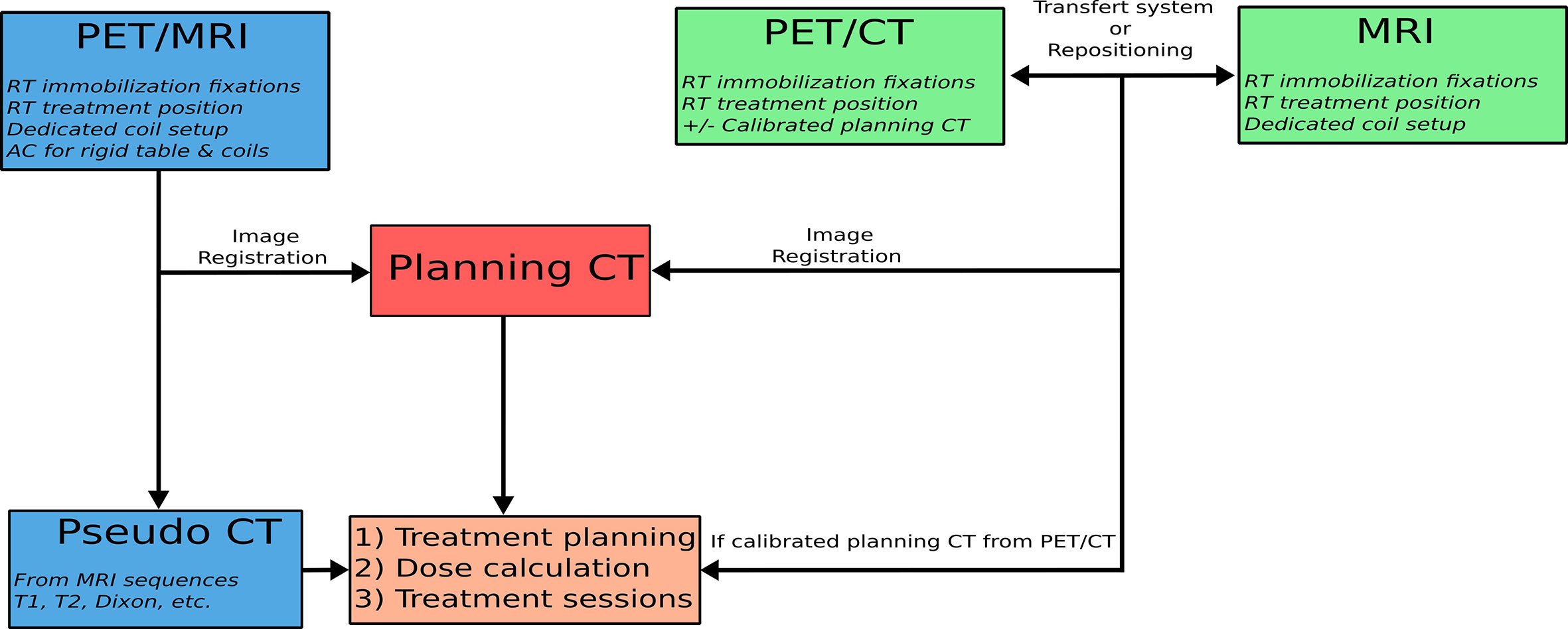
Figure 1 Diagram of the process for performing the PET/CT/MRI trimodality in the radiotherapy treatment position.
Image registration is the last step in the trimodality process. By placing the acquisitions in a common coordinate system, image registration allows correlating the information of each modality and thus improving clinical interpretation (see Figure 2). The image registration can be considered by two complementary approaches. The first one is material-based and consists in carrying out all the acquisitions under exactly the same conditions. First of all, the patient keeps the same position for each acquisition with the same radiotherapy immobilization fixations (23). The acquisition parameters will also allow obtaining the best possible alignment of the images by keeping the table height and choosing the same slice thickness, the same acquisition plane or 3D acquisitions with a large field of view (24).
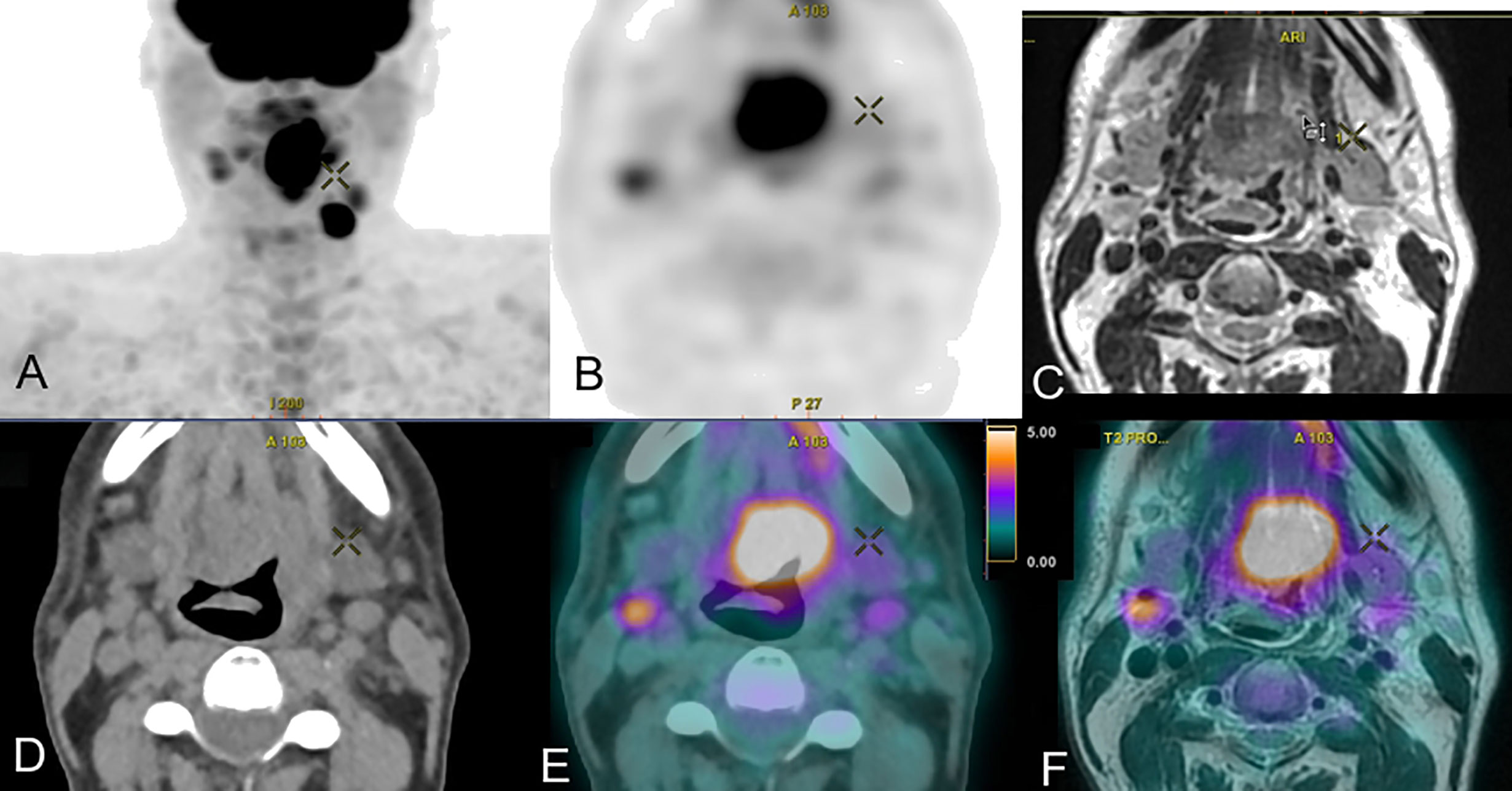
Figure 2 Trimodal acquisition of a cancer of the base of the tongue with in (A) the frontal maximum intensity projection PET image, in (B) the axial PET FDG acquisition, in (C) the axial T2 MRI acquisition, in (D) the axial CT acquisition, in (E) the axial PET/CT fusion and in (F) the axial PET/MRI fusion.
This first approach facilitates the second software-based approach. The image registration is done manually by a physician or with the help of an automatic registration algorithm. In this second case, it is necessary to first evaluate the accuracy of the algorithm used. Several studies have evaluated CT-MRI or trimodality registration algorithms, either on phantom or from patient data (25–28). The average errors obtained are between 0.4 and 2 mm. Eventually, a dedicated trimodality image fusion method can be used for better target delineation (29). A visual validation remains essential before proceeding with the planning of radiotherapy treatment, since humans are capable of detecting transformations of at least 1 mm and 1° (30). Image fusion is the last step in this process of trimodality; it allows correlating the information of each modality and improving the clinical interpretation (see Figure 2).
The registration can however be altered by the presence of artifacts, notably dental artifacts for CT (31, 32). Concerning MRI, the presence of geometric distortions, related to the system or the patient (33), can alter the registration. Algorithms can be used to correct distortions, but the presence of residual distortions has been mentioned (34). These distortions will affect the registration as well as the resulting treatment planning, in particular the target volume coverage in the case of stereotactic treatment (35, 36). In the context of multimodality for radiotherapy, the optimization of acquisition parameters is a crucial step to facilitate image registration.
Clinical Applications
Characterization
Combining PET/CT/MRI could provide complementary information at baseline to assess the disease spread but also to guide biopsy and characterize the tumor to help decision making.
For glioma, many MRI sequences, notably perfusion and multiparametric and PET radiotracers, notably radiolabeled amino-acid like 18F-FDOPA (FDOPA) and 18F-FET (FET), are available (37). By their combination, it has been found that metabolic (FDOPA PET/CT) and anatomic (MRI) could aid in choosing the target to be biopsied under stereotactic conditions in tumors without MR enhancement (38). In glioblastoma, another study including multiparametric imaging with FET PET/CT and FDG PET/MRI (including diffusion and dynamic contrast enhanced perfusion) has shown that combining parameters in a multivariate model enabled patient-specific maps of recurrence probability, where FET was the most important parameter (39). Comparable results were observed in a study showing that combination of apparent diffusion coefficient (ADC) and FET was more accurate to detect glioma infiltration than standard MRI in enhancing gliomas (38); such an approach could allow risk-adapted radiotherapy planning (39). Finally, for brain metastasis, FDG PET associated with MRI could be interesting for lesion targeting of stereotactic radiation therapy in case of a previously irradiated recurrent tumor (40); place of FDOPA in this indication has yet to be evaluated.
For cervical cancer, low ADC value in MRI and high FDG SUV of the primary tumor are also predictive factors for identifying high-risk patients (41). More complex parameters with a radiomics analysis combining PET and MRI parameters have also been found interesting to improve the prognostic determination in locally advanced cervical cancer treated with chemoradiotherapy (42).
Finally, for lung cancers, combination of MRI and PET can help to determine the prognostic with poor issues when low ADC value in MRI and high FDG SUVmax in PET are associated before stereotactic body radiotherapy (43) or when parameters derived from DCE and FDG PET parameters are associated (44).
Planning
For Head and Neck Cancers, it has been known for a long time that image fusion between FDG PET and MRI/CT is useful with regard to the determination of GTV and CTV for 3D conformal radiotherapy with preservation of normal tissues for the majority of the cases (45), with an interest to do them in treatment position, notably in case of intra-cranial tumor extension, heavy metal dental work, or contraindication of contrast enhanced CT (46). A special irradiation setup including thermoplastic mask, flat table, and head support can be used to allow a precise image co-registration of trimodality PET/CT MRI (47). As, according to pathological correlative series, no imaging modality completely encompasses the tumor, it can be useful to create a composite target volume derived from multi-modal imaging (48–51), notably for dose painting, consisting of delivering a heterogeneous irradiation within the tumor volume, as FDG PET and multiparametric MRI, in particular DWI, contain correlated but also independent information (47, 52–54). Concerning PET, other radiotracers than FDG can be used, such as FDOPA for skull base paraganglioma (55).
At the brain level, for gliomas treated with 3D conformal radiotherapy, or even by stereotactic radiotherapy by gamma-knife (9), the integration of both MRI and amino-acid PET/CT may help to improve GTV coverage by avoiding larger discrepancies between physical and biological imaging techniques (56–60), notably for the area of suspected non-enhancing tumor (61). Moreover for meningioma, 68Ga-DOTATOC PET, exploring the expression of SST2 receptors, can be usefully associated with CT and MRI for the treatment planning (62), notably to detect and assess the extent of infracranial meningioma invasion (63) and with an impact on sparing normal tissues (64), although all locations do not benefit from this trimodality (65).
For lung cancer, improvements in radiotherapy techniques (IMRT) make it important to manage the definition of volume and its mobility (66). The delineation of tumor lesions in lung cancer patients based on PET/CT is advisable in radiotherapy treatment planning and for locally advanced non-small cell lung cancer treated with IMRT or SRT, PET/CT being regarded as an indispensable staging procedure. Respiratory gating techniques (4D PET/CT) optimize radiotherapy of lung cancer to reduce toxicities especially the pulmonary and cardiac late toxicities (67, 68). The place of trimodality PET/CT/MRI has however to be defined in this indication.
For cervical cancer, a study on a cohort of 134 patients has shown that dose delivered to the primary cervical tumor by the combination of MRI-guided high-dose-rate PET/CT-guided IMRT brachytherapy was highly correlated with local tumor control (69). However, the delineation stays difficult as tumor volume discrepancies are observed between MRI and PET/CT GTV (70) even if it could decrease inter-observer variability (71). If FDG PET/CT appears superior as a functional imaging modality when compared with DW MRI in tumor contouring (72), a threshold around 30% of the FDG SUVmax appears to provide the best segmentation for this cancer (73).
Concerning prostatic cancer, multimodality, in particular multiparametric MRI and 68Ga-PSMA PET/CT, offers now large possibilities whatever the risk of the disease. In low-risk patients, selection of patients for active surveillance or treatment is improved; for intermediate-risk patients, it can help to select patients for supplemental brachytherapy; for high-risk patients it can help to guarantee adapted tumor volume segmentation, and finally, for recurrent or metastatic disease, it offers opportunities for more accurate assessment of tumor burden and treatment response (74). Therefore, it was found in a prospective study that combination of PSMA PET and multiparametric MRI provided a reliable TNM staging in patients with prostate cancer with a change in the therapeutic management for almost one third of the patients (75), PSMA PET being particularly interesting to delineate lymph node metastases (76). Moreover, for focal dose escalation to the dominant intraprostatic lesions, 68Ga-PSMA PET/CT and multiparametric MRI provide concordant results for delineation in nearly 50% of the lesions, with a PET GTV significantly larger than MRI GTV and which could have a role in treatment planning with intraprostatic dose escalation (77), notably because dose distribution within dominant intraprostatic lesions defined by multiparametric MRI and/or PSMA PET imaging is an independent risk factor for biochemical failure after primary external beam radiation therapy (78). If further studies are needed to confirm the optimal imaging techniques (79), it is already possible to combine multiparametric MRI and PSMA PET with higher tumor control probability with minimal to no increase of normal tissue complication probability compared to dose escalation on GTV defined on only one imaging modality (80, 81). Moreover trimodality with PSMA PET/CT/MRI can be used to orient the therapy in case of biochemical relapse after treatment (82).
For rectal cancer, trimodality PET/CT/MRI has been known to be possible for a relatively long time, notably to allow dose escalation on primary tumor (83). As a mobile organ, non-rigid registration between PET/CT and MRI shows good results, but this must be considered for the treatment planning (83). FDG PET/CT adds therefore information to MRI, with potentially a larger GTV in total when using the union of MRI and PET, and new or differently evaluated lesions in as many as 15% of the patients, potentially changing the treatment (84); further studies are necessary to well define the place of FDG PET in this indication (85, 86).
Adaptative Radiotherapy
Functional and anatomical data can be used not only prior to treatment, but also during and after treatment to guide ART, by improving the tumor targeting while better sparing the OARs, as well as determine tumor response (87). If the ART approach has been based first historically on per-treatment CT and/or CBCT images, it is now possible with MRI-linear accelerator (MRI-linac), combining an MRI and a linear accelerator, allowing an MRI acquisition before each treatment delivery (88). PET-linac is also emerging even if it remains less mature than MRI-linac (89). While extremely promising, the utilization of functional adaptation in radiation therapy is only beginning and needs more prospective clinical validation (90).
Perspectives
If trimodality can already be used in some indications, its usefulness remains to be confirmed. To this goal, several clinical studies are in progress. Concerning prostate cancer, our team is exploring in the ongoing DEMETER study (NCT03734757), which will include 20 patients, the interest of the association of PET/CT with 18F-choline and MRI compared to standard initial staging (CT, MRI and bone scan) to determine radio-therapeutic volumes. Another recently opened phase 2 study (NCT04402151), which aims to include 50 patients, will explore the interest of combination of PSMA PET/MRI to radiation delivery with a MRI-Linac. This study is based on the principle that the combination of PET PSMA and MRI allows for better delineation of intraprostatic nodules and greater diagnostic accuracy for the detection of metastatic disease. Moreover, MRI-Linac also allows adaptive radiotherapy in addition to the planning. For cervical cancer, a prospective observational study including 237 patients (NCT01992861) is exploring the role of MRI, including DCE, DWI, and spectrometry, and FDG PET performed before, during and after radiotherapy and chemotherapy. These could help to predict patient’s response to treatment and plan treatment. Finally, for head and neck cancers, our team is performing a prospective observational study with 60 inclusions planned called TRIMODAL (NCT03897166). Many questions will be explored in this study, including the comparison of the volumes determined on FDG PET/CT and on FDG PET/CT/MRI, the quality of image registration (in particular by using an air-cushion transfer system) and the use of algorithms for anthropometric measurements in MRI and CT scanners (with Dual x-ray absorptiometry as reference standard).
Conclusion
We have shown in this brief review the ways of carrying out a trimodality PET/CT/MRI for radiotherapy and potential clinical applications. Trimodality PET/CT/MRI, combining the strengths of the techniques and limiting the respective weaknesses, reinforces the role of imaging in guiding radiation therapy. Although this multimodality is recent, it is already possible in clinical routine with a high clinical impact and good inter-observer agreement. Clinical studies are still needed to confirm its role in clinical routine.
Author Contributions
PD, PH, PV, OV, PG, ST helped in conceptualization and project administration. PD and PH contributed to data curation. PD and PH wrote the main manuscript text and prepared the figures. All authors contributed to the article and approved the submitted version.
Conflict of Interest
The authors declare that the research was conducted in the absence of any commercial or financial relationships that could be construed as a potential conflict of interest.
References
1. Herrmann H, Seppenwoolde Y, Georg D, Widder J. Image guidance: past and future of radiotherapy. Radiologe (2019) 59:21–7. doi: 10.1007/s00117-019-0573-y
2. Bortfeld T. IMRT: a review and preview. Phys Med Biol (2006) 51:R363–79. doi: 10.1088/0031-9155/51/13/R21
3. Beaton L, Bandula S, Gaze MN, Sharma RA. How rapid advances in imaging are defining the future of precision radiation oncology. Br J Cancer (2019) 120:779–90. doi: 10.1038/s41416-019-0412-y
4. Tree AC, Khoo VS, Eeles RA, Ahmed M, Dearnaley DP, Hawkins MA, et al. Stereotactic body radiotherapy for oligometastases. Lancet Oncol (2013) 14:e28–37. doi: 10.1016/S1470-2045(12)70510-7
5. Vera P, Mihailescu S-D, Lequesne J, Modzelewski R, Bohn P, Hapdey S, et al. Radiotherapy boost in patients with hypoxic lesions identified by 18F-FMISO PET/CT in non-small-cell lung carcinoma: can we expect a better survival outcome without toxicity? [RTEP5 long-term follow-up]. Eur J Nucl Med Mol Imaging (2019) 46:1448–56. doi: 10.1007/s00259-019-04285-9
6. Decazes P, Thureau S, Dubray B, Vera P. How to use PET/CT in the evaluation of response to radiotherapy. Q J Nucl Med Mol Imaging (2018) 62:152–64. doi: 10.23736/S1824-4785.17.03033-3
7. Vogel WV, Lam MGEH, Pameijer FA, van der Heide UA, van de Kamer JB, Philippens ME, et al. Functional Imaging in Radiotherapy in the Netherlands: Availability and Impact on Clinical Practice. Clin Oncol (2016) 28:e206–15. doi: 10.1016/j.clon.2016.09.003
8. Richard P, Sandison G, Dang Q, Johnson B, Wong T, Parvathaneni U. Dental amalgam artifact: Adverse impact on tumor visualization and proton beam treatment planning in oral and oropharyngeal cancers. Pract Radiat Oncol (2015) 5:e583–8. doi: 10.1016/j.prro.2015.04.007
9. Rundo L, Stefano A, Militello C, Russo G, Sabini MG, D’Arrigo C, et al. A fully automatic approach for multimodal PET and MR image segmentation in gamma knife treatment planning. Comput Methods Programs Biomed (2017) 144:77–96. doi: 10.1016/j.cmpb.2017.03.011
10. Ak S, Kiliç C, Özlügedik S. Correlation of PET-CT, MRI and histopathology findings in the follow-up of patients with nasopharyngeal cancer. Braz J Otorhinolaryngol (2020) 20:30004–5. doi: 10.1016/j.bjorl.2019.12.004
11. Chopra S, Engineer R, Shah S, Shukla R, Dora T, Gupta P, et al. MRI- and PET-Guided Interstitial Brachytherapy for Postsurgical Vaginal Recurrences of Cervical Cancer: Results of Phase II Study. Int J Radiat Oncol Biol Phys (2020) 106:310–9. doi: 10.1016/j.ijrobp.2019.10.037
12. Yang J, Beadle BM, Garden AS, Schwartz DL, Aristophanous M. A multimodality segmentation framework for automatic target delineation in head and neck radiotherapy: Automatic target delineation in head and neck radiotherapy. Med Phys (2015) 42:5310–20. doi: 10.1118/1.4928485
13. Reynaert N. PET and MRI based RT treatment planning: Handling uncertainties. Cancer Radiothér (2019) 23:753–60. doi: 10.1016/j.canrad.2019.08.002
14. Paulus DH, Oehmigen M, Grüneisen J, Umutlu L, Quick HH. Whole-body hybrid imaging concept for the integration of PET/MR into radiation therapy treatment planning. Phys Med Biol (2016) 61:3504–20. doi: 10.1088/0031-9155/61/9/3504
15. Leibfarth S, Mönnich D, Welz S, Siegel C, Schwenzer N, Schmidt H, et al. A strategy for multimodal deformable image registration to integrate PET/MR into radiotherapy treatment planning. Acta Oncol (2013) 52:1353–9. doi: 10.3109/0284186X.2013.813964
16. Winter RM, Leibfarth S, Schmidt H, Zwirner K, Mönnich D, Welz S, et al. Assessment of image quality of a radiotherapy-specific hardware solution for PET/MRI in head and neck cancer patients. Radiother Oncol (2018) 128:485–91. doi: 10.1016/j.radonc.2018.04.018
17. Paulus DH, Thorwath D, Schmidt H, Quick HH. Towards integration of PET/MR hybrid imaging into radiation therapy treatment planning. Med Phys (2014) 41:072505. doi: 10.1118/1.4881317
18. Wiesinger F, Bylund M, Yang J, Kaushik S, Shanbhag D, Ahn S, et al. Zero TE-based pseudo-CT image conversion in the head and its application in PET/MR attenuation correction and MR-guided radiation therapy planning. Magn Reson Med (2018) 80:1440–51. doi: 10.1002/mrm.27134
19. Botman R, Tetar SU, Palacios MA, Slotman BJ, Lagerwaard FJ, Bruynzeel AME. The clinical introduction of MR-guided radiation therapy from a RTT perspective. Clin Transl Radiat Oncol (2019) 18:140–5. doi: 10.1016/j.ctro.2019.04.019
20. Peng Y, Chen S, Qin A, Chen M, Gao X, Liu Y, et al. Magnetic resonance-based synthetic computed tomography images generated using generative adversarial networks for nasopharyngeal carcinoma radiotherapy treatment planning. Radiother Oncol (2020) 150:217–24. doi: 10.1016/j.radonc.2020.06.049
21. Maspero M, Savenije MHF, Dinkla AM, Seevinck PR, Intven MPW, Jurgenliemk-Schulz IM, et al. Dose evaluation of fast synthetic-CT generation using a generative adversarial network for general pelvis MR-only radiotherapy. Phys Med Biol (2018) 63:185001. doi: 10.1088/1361-6560/aada6d
22. Veit-Haibach P, Kuhn FP, Wiesinger F, Delso G, von Schulthess G. PET-MR imaging using a tri-modality PET/CT-MR system with a dedicated shuttle in clinical routine. MAGMA (2013) 26:25–35. doi: 10.1007/s10334-012-0344-5
23. Samarin A, Kuhn FP, Brandsberg F, von Schulthess G, Burger IA. Image registration accuracy of an in-house developed patient transport system for PET/CT+MR and SPECT+CT imaging. Nucl Med Commun (2015) 36:194. doi: 10.1097/MNM.0000000000000229
24. Lotterie J-A, Duthil P, Januel A-C, Redon A, Menegalli D, Blond S, et al. [Stereotactic and diagnostic imaging in radiosurgery]. Cancer Radiother (2012) 16 Suppl:S10–25. doi: 10.1016/j.canrad.2011.09.005
25. Daisne J-F, Sibomana M, Bol A, Cosnard G, Lonneux M, Grégoire V. Evaluation of a multimodality image (CT, MRI and PET) coregistration procedure on phantom and head and neck cancer patients: accuracy, reproducibility and consistency. Radiother Oncol (2003) 69:237–45. doi: 10.1016/j.radonc.2003.10.009
26. Wang X, Li L, Hu C, Qiu J, Xu Z, Feng Y. A comparative study of three CT and MRI registration algorithms in nasopharyngeal carcinoma. J Appl Clin Med Phys (2009) 10:2906. doi: 10.1120/jacmp.v10i2.2906
27. Moore CS, Liney GP, Beavis AW. Quality assurance of registration of CT and MRI data sets for treatment planning of radiotherapy for head and neck cancers. J Appl Clin Med Phys (2004) 5:25–35. doi: 10.1120/jacmp.v5i1.1951
28. Roberson PL, McLaughlin PW, Narayana V, Troyer S, Hixson GV, Kessler ML. Use and uncertainties of mutual information for computed tomography/ magnetic resonance (CT/MR) registration post permanent implant of the prostate. Med Phys (2005) 32:473–82. doi: 10.1118/1.1851920
29. Guo L, Shen S, Harris E, Wang Z, Jiang W, Guo Y, et al. A Tri-Modality Image Fusion Method for Target Delineation of Brain Tumors in Radiotherapy. PloS One (2014) 9:e112187. doi: 10.1371/journal.pone.0112187
30. Veninga T, Huisman H, van der Maazen RWM, Huizenga H. Clinical validation of the normalized mutual information method for registration of CT and MR images in radiotherapy of brain tumors. J Appl Clin Med Phys (2004) 5:66–79. doi: 10.1120/jacmp.v5i3.1959
31. Park HS, Choi JK, Park K-R, Kim KS, Lee S-H, Ye JC, et al. Metal artifact reduction in CT by identifying missing data hidden in metals. J Xray Sci Technol (2013) 21:357–72. doi: 10.3233/XST-130384
32. Lee M-Y, Song K-H, Lee J-W, Choe B-Y, Suh TS. Metal artifacts with dental implants: Evaluation using a dedicated CT/MR oral phantom with registration of the CT and MR images. Sci Rep (2019) 9:754. doi: 10.1038/s41598-018-36227-0
33. Fransson A, Andreo P, Pötter R. Aspects of MR image distortions in radiotherapy treatment planning. Strahlenther Onkol (2001) 177:59–73. doi: 10.1007/pl00002385
34. Weygand J, Fuller CD, Ibbott GS, Mohamed ASR, Ding Y, Yang J, et al. Spatial Precision in Magnetic Resonance Imaging-Guided Radiation Therapy: The Role of Geometric Distortion. Int J Radiat Oncol Biol Phys (2016) 95:1304–16. doi: 10.1016/j.ijrobp.2016.02.059
35. Putz F, Mengling V, Perrin R, Masitho S, Weissmann T, Rösch J, et al. Magnetic resonance imaging for brain stereotactic radiotherapy: A review of requirements and pitfalls. Strahlenther Onkol (2020) 196:444–56. doi: 10.1007/s00066-020-01604-0
36. Pappas EP, Alshanqity M, Moutsatsos A, Lababidi H, Alsafi K, Georgiou K, et al. MRI-Related Geometric Distortions in Stereotactic Radiotherapy Treatment Planning: Evaluation and Dosimetric Impact. Technol Cancer Res Treat (2017) 16:1120–9. doi: 10.1177/1533034617735454
37. Moreau A, Febvey O, Mognetti T, Frappaz D, Kryza D. Contribution of Different Positron Emission Tomography Tracers in Glioma Management: Focus on Glioblastoma. Front Oncol (2019) 9:1134. doi: 10.3389/fonc.2019.01134
38. Todeschi J, Bund C, Cebula H, Chibbaro S, Lhermitte B, Pin Y, et al. Diagnostic value of fusion of metabolic and structural images for stereotactic biopsy of brain tumors without enhancement after contrast medium injection. Neurochirurgie (2019) 65:357–64. doi: 10.1016/j.neuchi.2019.08.002
39. Lundemann M, Munck af Rosenschöld P, Muhic A, Larsen VA, Poulsen HS, Engelholm S-A, et al. Feasibility of multi-parametric PET and MRI for prediction of tumour recurrence in patients with glioblastoma. Eur J Nucl Med Mol Imaging (2019) 46:603–13. doi: 10.1007/s00259-018-4180-3
40. Scranton RA, Sadrameli S, Butler EB, Farach A, Wang H-C, Teh BS, et al. Coregistration of Magnetic Resonance and [18F] Fludeoxyglucose–Positron Emission Tomography Imaging for Stereotactic Radiation Therapy Planning: Case Report in a Previously Irradiated Brain Metastasis With Recurrent Tumor and Radiation Necrosis. Pract Radiat Oncol (2020) 10:133–7. doi: 10.1016/j.prro.2019.11.008
41. Akkus Yildirim B, Onal C, Erbay G, Cem Guler O, Karadeli E, Reyhan M, et al. Prognostic values of ADC mean and SUV max of the primary tumour in cervical cancer patients treated with definitive chemoradiotherapy. J Obstet Gynaecol (2019) 39:224–30. doi: 10.1080/01443615.2018.1492528
42. Lucia F, Visvikis D, Desseroit M-C, Miranda O, Malhaire J-P, Robin P, et al. Prediction of outcome using pretreatment 18F-FDG PET/CT and MRI radiomics in locally advanced cervical cancer treated with chemoradiotherapy. Eur J Nucl Med Mol Imaging (2018) 45:768–86. doi: 10.1007/s00259-017-3898-7
43. Iizuka Y, Matsuo Y, Umeoka S, Nakamoto Y, Ueki N, Mizowaki T, et al. Prediction of clinical outcome after stereotactic body radiotherapy for non-small cell lung cancer using diffusion-weighted MRI and 18F-FDG PET. Eur J Radiol (2014) 83:2087–92. doi: 10.1016/j.ejrad.2014.07.018
44. Huang Y-S, Chen JL-Y, Chen J-Y, Lee Y-F, Huang J-Y, Kuo S-H, et al. Predicting tumor responses and patient survival in chemoradiotherapy-treated patients with non-small-cell lung cancer using dynamic contrast-enhanced integrated magnetic resonance–positron-emission tomography. Strahlenther Onkol (2019) 195:707–18. doi: 10.1007/s00066-018-1418-8
45. Nishioka T, Shiga T, Shirato H, Tsukamoto E, Tsuchiya MDK, Kato T, et al. Image fusion between 18 FDG-PET and MRI/CT for radiotherapy planning of oropharyngeal and nasopharyngeal carcinomas. Int J Radiat Oncol Biol Phys (2002) 53:1051–7. doi: 10.1016/S0360-3016(02)02854-7
46. Gardner M, Halimi P, Valinta D, Plantet M-M, Alberini J-L, Wartski M, et al. Use of single MRI and 18F-FDG PET-CT scans in both diagnosis and radiotherapy treatment planning in patients with head and neck cancer: Advantage on target volume and critical organ delineation. Head Neck (2009) 31:461–7. doi: 10.1002/hed.21005
47. Zwirner K, Thorwarth D, Winter RM, Welz S, Weiss J, Schwenzer NF, et al. Voxel-wise correlation of functional imaging parameters in HNSCC patients receiving PET/MRI in an irradiation setup. Strahlenther Onkol (2018) 194:719–26. doi: 10.1007/s00066-018-1292-4
48. Prestwich RJD, Sykes J, Carey B, Sen M, Dyker KE, Scarsbrook AF. Improving Target Definition for Head and Neck Radiotherapy: A Place for Magnetic Resonance Imaging and 18-Fluoride Fluorodeoxyglucose Positron Emission Tomography? Clin Oncol (2012) 24:577–89. doi: 10.1016/j.clon.2012.04.002
49. Bird D, Scarsbrook AF, Sykes J, Ramasamy S, Subesinghe M, Carey B, et al. Multimodality imaging with CT, MR and FDG-PET for radiotherapy target volume delineation in oropharyngeal squamous cell carcinoma. BMC Cancer (2015) 15:844. doi: 10.1186/s12885-015-1867-8
50. Ligtenberg H, Jager EA, Caldas-Magalhaes J, Schakel T, Pameijer FA, Kasperts N, et al. Modality-specific target definition for laryngeal and hypopharyngeal cancer on FDG-PET, CT and MRI. Radiother Oncol (2017) 123:63–70. doi: 10.1016/j.radonc.2017.02.005
51. Samołyk-Kogaczewska N, Sierko E, Zuzda K, Gugnacki P, Szumowski P, Mojsak M, et al. PET/MRI-guided GTV delineation during radiotherapy planning in patients with squamous cell carcinoma of the tongue. Strahlenther Onkol (2019) 195:780–91. doi: 10.1007/s00066-019-01480-3
52. Houweling AC, Wolf AL, Vogel WV, Hamming-Vrieze O, van Vliet-Vroegindeweij C, van de Kamer JB, et al. FDG-PET and diffusion-weighted MRI in head-and-neck cancer patients: Implications for dose painting. Radiother Oncol (2013) 106:250–4. doi: 10.1016/j.radonc.2013.01.003
53. Rasmussen JH, Nørgaard M, Hansen AE, Vogelius IR, Aznar MC, Johannesen HH, et al. Feasibility of Multiparametric Imaging with PET/MR in Head and Neck Squamous Cell Carcinoma. J Nucl Med (2017) 58:69–74. doi: 10.2967/jnumed.116.180091
54. Cardoso M, Min M, Jameson M, Tang S, Rumley C, Fowler A, et al. Evaluating diffusion-weighted magnetic resonance imaging for target volume delineation in head and neck radiotherapy. J Med Imaging Radiat Oncol (2019) 63:399–407. doi: 10.1111/1754-9485.12866
55. Helali M, Moreau M, Le Fèvre C, Heimburger C, Bund C, Goichot B, et al. 18F-FDOPA PET/CT Combined with MRI for Gross Tumor Volume Delineation in Patients with Skull Base Paraganglioma. Cancers (2019) 11:54. doi: 10.3390/cancers11010054
56. Rieken S, Habermehl D, Giesel FL, Hoffmann C, Burger U, Rief H, et al. Analysis of FET-PET imaging for target volume definition in patients with gliomas treated with conformal radiotherapy. Radiother Oncol (2013) 109:487–92. doi: 10.1016/j.radonc.2013.06.043
57. Kosztyla R, Chan EK, Hsu F, Wilson D, Ma R, Cheung A, et al. High-Grade Glioma Radiation Therapy Target Volumes and Patterns of Failure Obtained From Magnetic Resonance Imaging and 18F-FDOPA Positron Emission Tomography Delineations From Multiple Observers. Int J Radiat Oncol Biol Phys (2013) 87:1100–6. doi: 10.1016/j.ijrobp.2013.09.008
58. Harat M, Małkowski B, Makarewicz R. Pre-irradiation tumour volumes defined by MRI and dual time-point FET-PET for the prediction of glioblastoma multiforme recurrence: A prospective study. Radiother Oncol (2016) 120:241–7. doi: 10.1016/j.radonc.2016.06.004
59. Hirata T, Kinoshita M, Tamari K, Seo Y, Suzuki O, Wakai N, et al. 11C-methionine-18F-FDG dual-PET-tracer–based target delineation of malignant glioma: evaluation of its geometrical and clinical features for planning radiation therapy. J Neurosurg (2019) 131:676–86. doi: 10.3171/2018.4.JNS1859
60. Lohmann P, Stavrinou P, Lipke K, Bauer EK, Ceccon G, Werner J-M, et al. FET PET reveals considerable spatial differences in tumour burden compared to conventional MRI in newly diagnosed glioblastoma. Eur J Nucl Med Mol Imaging (2019) 46:591–602. doi: 10.1007/s00259-018-4188-8
61. Hayes AR, Jayamanne D, Hsiao E, Schembri GP, Bailey DL, Roach PJ, et al. Utilizing 18F-fluoroethyltyrosine (FET) positron emission tomography (PET) to define suspected nonenhancing tumor for radiation therapy planning of glioblastoma. Pract Radiat Oncol (2018) 8:230–8. doi: 10.1016/j.prro.2018.01.006
62. Thorwarth D, Müller A-C, Pfannenberg C, Beyer T. “Combined PET/MR Imaging Using 68Ga-DOTATOC for Radiotherapy Treatment Planning in Meningioma Patients,”. In: Baum RP, Rösch F, editors. Theranostics, Gallium-68, and Other Radionuclides Recent Results in Cancer Research. Berlin, Heidelberg: Springer Berlin Heidelberg (2012). p. 425–39. doi: 10.1007/978-3-642-27994-2_23
63. Graf R, Plotkin M, Steffen IG, Wurm R, Wust P, Brenner W, et al. Magnetic resonance imaging, computed tomography, and 68Ga-DOTATOC positron emission tomography for imaging skull base meningiomas with infracranial extension treated with stereotactic radiotherapy - a case series. Head Face Med (2012) 8:1. doi: 10.1186/1746-160X-8-1
64. Stade F, Dittmar J-O, Jäkel O, Kratochwil C, Haberkorn U, Debus J, et al. Influence of 68Ga-DOTATOC on sparing of normal tissue for radiation therapy of skull base meningioma: differential impact of photon and proton radiotherapy. Radiat Oncol (2018) 13:58. doi: 10.1186/s13014-018-1008-z
65. Maclean J, Fersht N, Sullivan K, Kayani I, Bomanji J, Dickson J, et al. Simultaneous 68 Ga DOTATATE Positron Emission Tomography/Magnetic Resonance Imaging in Meningioma Target Contouring: Feasibility and Impact Upon Interobserver Variability Versus Positron Emission Tomography/Computed Tomography and Computed Tomography/Magnetic Resonance Imaging. Clin Oncol (2017) 29:448–58. doi: 10.1016/j.clon.2017.03.004
66. Chun SG, Hu C, Choy H, Komaki RU, Timmerman RD, Schild SE, et al. Impact of Intensity-Modulated Radiation Therapy Technique for Locally Advanced Non-Small-Cell Lung Cancer: A Secondary Analysis of the NRG Oncology RTOG 0617 Randomized Clinical Trial. J Clin Oncol (2017) 35:56–62. doi: 10.1200/JCO.2016.69.1378
67. Giraud P, Morvan E, Claude L, Mornex F, Le Pechoux C, Bachaud J-M, et al. Respiratory gating techniques for optimization of lung cancer radiotherapy. J Thorac Oncol (2011) 6:2058–68. doi: 10.1097/JTO.0b013e3182307ec2
68. Sindoni A, Minutoli F, Pontoriero A, Iatì G, Baldari S, Pergolizzi S. Usefulness of four dimensional (4D) PET/CT imaging in the evaluation of thoracic lesions and in radiotherapy planning: Review of the literature. Lung Cancer (2016) 96:78–86. doi: 10.1016/j.lungcan.2016.03.019
69. Dyk P, Jiang N, Sun B, DeWees TA, Fowler KJ, Narra V, et al. Cervical Gross Tumor Volume Dose Predicts Local Control Using Magnetic Resonance Imaging/Diffusion-Weighted Imaging—Guided High-Dose-Rate and Positron Emission Tomography/Computed Tomography—Guided Intensity Modulated Radiation Therapy. Int J Radiat Oncol Biol Phys (2014) 90:794–801. doi: 10.1016/j.ijrobp.2014.07.039
70. Zhang S, Xin J, Guo Q, Ma J, Ma Q, Sun H. Comparison of Tumor Volume Between PET and MRI in Cervical Cancer With Hybrid PET/MR. Int J Gynecol Cancer (2014) 24:744–50. doi: 10.1097/IGC.0000000000000097
71. Han K, Croke J, Foltz W, Metser U, Xie J, Shek T, et al. A prospective study of DWI, DCE-MRI and FDG PET imaging for target delineation in brachytherapy for cervical cancer. Radiother Oncol (2016) 120:519–25. doi: 10.1016/j.radonc.2016.08.002
72. Lai AYT, Perucho JAU, Xu X, Hui ES, Lee EYP. Concordance of FDG PET/CT metabolic tumour volume versus DW-MRI functional tumour volume with T2-weighted anatomical tumour volume in cervical cancer. BMC Cancer (2017) 17:825. doi: 10.1186/s12885-017-3800-9
73. Zhang S, Xin J, Guo Q, Ma J, Ma Q, Sun H, et al. Defining PET tumor volume in cervical cancer with hybrid PET/MRI: a comparative study. Nucl Med Commun (2014) 35:712–9. doi: 10.1097/MNM.0000000000000113
74. Moghanaki D, Turkbey B, Vapiwala N, Ehdaie B, Frank SJ, McLaughlin PW, et al. Advances in Prostate Cancer Magnetic Resonance Imaging and Positron Emission Tomography-Computed Tomography for Staging and Radiotherapy Treatment Planning. Semin Radiat Oncol (2017) 27:21–33. doi: 10.1016/j.semradonc.2016.08.008
75. Grubmüller B, Baltzer P, Hartenbach S, D’Andrea D, Helbich TH, Haug AR, et al. PSMA Ligand PET/MRI for Primary Prostate Cancer: Staging Performance and Clinical Impact. Clin Cancer Res (2018) 24:6300–7. doi: 10.1158/1078-0432.CCR-18-0768
76. Walacides D, Meier A, Knöchelmann AC, Meinecke D, Derlin T, Bengel FM, et al. Comparison of 68 Ga-PSMA ligand PET/CT versus conventional cross-sectional imaging for target volume delineation for metastasis-directed radiotherapy for metachronous lymph node metastases from prostate cancer. Strahlenther Onkol (2019) 195:420–9. doi: 10.1007/s00066-018-1417-9
77. Zamboglou C, Wieser G, Hennies S, Rempel I, Kirste S, Soschynski M, et al. MRI versus 68Ga-PSMA PET/CT for gross tumour volume delineation in radiation treatment planning of primary prostate cancer. Eur J Nucl Med Mol Imaging (2016) 43:889–97. doi: 10.1007/s00259-015-3257-5
78. Zamboglou C, Klein CM, Thomann B, Fassbender TF, Rischke HC, Kirste S, et al. The dose distribution in dominant intraprostatic tumour lesions defined by multiparametric MRI and PSMA PET/CT correlates with the outcome in patients treated with primary radiation therapy for prostate cancer. Radiat Oncol (2018) 13:65. doi: 10.1186/s13014-018-1014-1
79. Pathmanathan AU, Alexander EJ, Huddart RA, Tree AC. The delineation of intraprostatic boost regions for radiotherapy using multimodality imaging. Future Oncol (2016) 12:2495–511. doi: 10.2217/fon-2016-0129
80. Zamboglou C, Thomann B, Koubar K, Bronsert P, Krauss T, Rischke HC, et al. Focal dose escalation for prostate cancer using 68Ga-HBED-CC PSMA PET/CT and MRI: a planning study based on histology reference. Radiat Oncol (2018) 13:81. doi: 10.1186/s13014-018-1036-8
81. Bettermann AS, Zamboglou C, Kiefer S, Jilg CA, Spohn S, Kranz-Rudolph J, et al. [68Ga-]PSMA-11 PET/CT and multiparametric MRI for gross tumor volume delineation in a slice by slice analysis with whole mount histopathology as a reference standard – Implications for focal radiotherapy planning in primary prostate cancer. Radiother Oncol (2019) 141:214–9. doi: 10.1016/j.radonc.2019.07.005
82. Bhargava P, Ravizzini G, Chapin BF, Kundra V. Imaging Biochemical Recurrence After Prostatectomy: Where Are We Headed? Am J Roentgenol (2020) 214:1–11. doi: 10.2214/AJR.19.21905
83. Roels S, Slagmolen P, Nuyts J, Lee JA, Loeckx D, Maes F, et al. Biological Image-Guided Radiotherapy in Rectal Cancer: Challenges and Pitfalls. Int J Radiat Oncol Biol Phys (2009) 75:782–90. doi: 10.1016/j.ijrobp.2008.11.031
84. Brændengen M, Hansson K, Radu C, Siegbahn A, Jacobsson H, Glimelius B. Delineation of Gross Tumor Volume (GTV) for Radiation Treatment Planning of Locally Advanced Rectal Cancer Using Information From MRI or FDG-PET/CT: A Prospective Study. Int J Radiat Oncol Biol Phys (2011) 81:e439–45. doi: 10.1016/j.ijrobp.2011.03.031
85. Gwynne S, Mukherjee S, Webster R, Spezi E, Staffurth J, Coles B, et al. Imaging for Target Volume Delineation in Rectal Cancer Radiotherapy — A Systematic Review. Clin Oncol (2012) 24:52–63. doi: 10.1016/j.clon.2011.10.001
86. Wang YY, Zhe H. Clinical application of multimodality imaging in radiotherapy treatment planning for rectal cancer. Cancer Imaging (2013) 13:495–501. doi: 10.1102/1470-7330.2013.0046
87. Thureau S, Briens A, Decazes P, Castelli J, Barateau A, Garcia R, et al. PET and MRI guided adaptive radiotherapy: Rational, feasibility and benefit. Cancer Radiother (2020) 24:635–44. doi: 10.1016/j.canrad.2020.06.017
88. van Timmeren JE, Chamberlain M, Krayenbuehl J, Wilke L, Ehrbar S, Bogowicz M, et al. Treatment plan quality during online adaptive re-planning. Radiat Oncol (2020) 15:203. doi: 10.1186/s13014-020-01641-0
89. Fan Q, Nanduri A, Mazin S, Zhu L. Emission guided radiation therapy for lung and prostate cancers: a feasibility study on a digital patient. Med Phys (2012) 39:7140–52. doi: 10.1118/1.4761951
Keywords: computed tomography, magnetic resonance imaging, positron emission tomography, radiotherapy, hybrid imaging
Citation: Decazes P, Hinault P, Veresezan O, Thureau S, Gouel P and Vera P (2021) Trimodality PET/CT/MRI and Radiotherapy: A Mini-Review. Front. Oncol. 10:614008. doi: 10.3389/fonc.2020.614008
Received: 04 October 2020; Accepted: 22 December 2020;
Published: 04 February 2021.
Edited by:
Romain-David Seban, Institut Curie, FranceReviewed by:
Antoine Schernberg, Hôpital Tenon AP-HP, FranceHamid Mammar, Institut Curie, France
Alexandre Escande, Oscar Lambret Cancer Center, France
Copyright © 2021 Decazes, Hinault, Veresezan, Thureau, Gouel and Vera. This is an open-access article distributed under the terms of the Creative Commons Attribution License (CC BY). The use, distribution or reproduction in other forums is permitted, provided the original author(s) and the copyright owner(s) are credited and that the original publication in this journal is cited, in accordance with accepted academic practice. No use, distribution or reproduction is permitted which does not comply with these terms.
*Correspondence: Pierre Decazes, pierre.decazes@chb.unicancer.fr