- 1Department of Medical Oncology, IRCCS San Raffaele Scientific Institute, Milan, Italy
- 2Division of Experimental Oncology, IRCCS San Raffaele Scientific Institute, Milan, Italy
- 3Pancreatic Surgery Unit, IRCCS San Raffaele Scientific Institute, Milan, Italy
- 4Faculty of Medicine and Surgery, Vita-Salute San Raffaele University, Milan, Italy
- 5Diabetes Research Institute, IRCCS San Raffaele Scientific Institute, Milan, Italy
- 6Division of Immunology, IRCCS San Raffaele Scientific Institute, Milan, Italy
- 7Experimental Imaging Center, IRCCS San Raffaele Scientific Institute, Milan, Italy
Chromogranin A (CgA), a secretory protein released in the blood by the neuroendocrine system, consists of a mixture of full-length molecules and fragments endowed of vasoregulatory activity. The extent and the role of CgA fragmentation were investigated in patients with locally advanced or metastatic pancreatic ductal adenocarcinoma (PDAC, n=172). Multivariate analysis showed that full-length CgA was associated with better progression free and overall survival, whereas CgA C-terminal fragmentation was associated with worse prognosis. In vitro studies showed that PDAC cells can promote the cleavage of CgA C-terminal region by activating plasminogen to plasmin. Limited digestion of full-length CgA with plasmin abolished its anti-angiogenic activity and generated pro-angiogenic molecules. The fragmentation of CgA C-terminal region was increased also in murine models of PDAC. In these models, the inhibition of CgA fragmentation with aprotinin, an inhibitor of plasmin and other serine proteases, or the blockade of pro-angiogenic fragments with specific antibodies inhibited the growth of PDAC implanted subcutaneously in mice. Finally, administration of full-length CgA to mice bearing orthotopic PDAC reduced tumor perfusion, as measured by contrast-enhanced ultrasound. These findings suggest that PDAC can promote the cleavage of circulating CgA C-terminal region to generate fragments that regulate the tumor vascular biology and that may represent new potential therapeutic targets.
Introduction
Pancreatic ductal adenocarcinoma (PDAC), the most common type of pancreatic cancer, is an aggressive malignancy arising from the exocrine epithelial component of the pancreas (1). PDAC currently represents the fourth cause of cancer-related death (2). Despite the increasing incidence, the prognosis of this neoplasm is still poor and the therapeutic options are very limited (3, 4). Thus, studies aimed at investigating the mechanisms of PDAC growth and the identification of new prognostic markers and therapeutic targets are of great experimental and clinical relevance.
A growing body of evidence suggests that circulating chromogranin A (CgA), an acidic secretory protein, may represent an important regulating factor of the vascular biology of solid tumors (5–8). This protein is stored in the secretory granules of many normal neuroendocrine cells and neurons (9, 10) and is exocytotically released in circulation, reaching 0.2-1 nM in healthy subjects (7, 11). Higher CgA levels have been detected in patients with neoplastic, cardiovascular, renal, gastrointestinal, and inflammatory diseases, with, in some cases, important diagnostic and prognostic implications (9, 11). Structural studies have shown that circulating CgA consists of a mixture of full-length CgA (CgA1-439) and CgA-derived fragments of different size, including peptides lacking part or the entire CgA410-439 region and shorter fragments (12). CgA1-439 and some of its fragments (e.g., vasostatin-1, catestatin and serpinin) can exert a variety of biological functions in the regulation of cardiovascular system, metabolism and innate immunity (13). Regarding their vascular functions, recent studies have shown that CgA1-439 can exert anti-angiogenic effects at physiological concentrations (5, 6, 8). An active anti-angiogenic site is located in its C-terminal region, whereas a latent (or less active) site is located in the N-terminal region, this site requiring cleavage of the Q76-K77 peptide bond to generate CgA1-76 (vasostatin-1), an anti-angiogenic fragment (5). In contrast, cleavage of R373-R374 site in the C-terminal region generates CgA1-373, a pro-angiogenic fragment (5, 14). The pro-angiogenic activity of CgA1-373 requires the binding of its Pro-Gly-Pro-Leu-Arg373 site (PGPQLR373) to neuropilin-1: accordingly, antibodies against this site (which is cryptic in CgA1-439) can reduce angiogenesis and tumor growth in various animal models of solid tumors (8). Further processing of CgA1-373 to CgA1-372 and consequent loss of the C-terminal arginine, which can occur in the blood, causes loss of neuropilin-1 binding and gain of anti-angiogenic activity (8). Thus, cleavage of circulating CgA in tumors and then in the blood may represent a complex mechanism for the spatio-temporal regulation of the tumor vascular biology. According to this view, a significant correlation between CgA fragmentation at the R373-R374 site and tumor microvessel density has been observed in the bone marrow of patients with multiple myeloma (12). However, the prognostic value of CgA cleavage on tumor progression and patient survival still remains unexplored. These notions prompted us to investigate the extent of CgA N- and C-terminal proteolytic processing in patients diagnosed with PDAC and to explore its prognostic value and function. We show that cleavage of circulating CgA C-terminal region is increased in patients and that CgA cleavage predicts worse progression-free and overall survival. Furthermore, using in vitro and in vivo models of PDAC, we provide data suggesting a role of the plasminogen activator-plasmin system in CgA cleavage and a function of CgA fragmentation in the regulation of PDAC vascular biology.
Materials and Methods
Patients and Plasma Samples
Patients with cyto/histologically proven stage III or IV untreated PDAC were enrolled in this study. Additional eligibility criteria included: age 18–70 years and Karnofsky performance status >60, or 71–75 years and Karnofsky performance status >70; absolute neutrophil count ≥1500/mm3, platelet count ≥100,000/mm3, hemoglobin ≥10 g/dl; serum creatinine ≤1.5 mg/dl; total bilirubin ≤1.5 mg/dl; transaminases ≤3 times the upper limit of normal. This study was approved by the local ethics committee (OSR-Prot. PACT-19) and was conducted at the San Raffaele Hospital of Milan (authorized organization) following the principles of good clinical trial practice according to the Declaration of Helsinki. A written informed consent was obtained from each patient.
Five milliliters of peripheral blood were collected at baseline, before the beginning of chemotherapy, in a sterile test-tube and centrifuged at 2,500xg for 10 min. Plasma samples were stocked in 0.5 ml aliquots in cryovials and stored at -80°C until measurement.
Recombinant CgA, Fragments, Antibodies, and Immunoassays
Human CgA1-439 and CgA1-373, were prepared by recombinant DNA technology and characterized by mass spectrometry analysis (sequence), SDS-PAGE, and western blotting analyses as previously reported (5, 6, 12, 15). Polyclonal and monoclonal antibodies against various epitopes of CgA located in the N-terminal, central and C-terminal regions of CgA have been prepared as described in Supplementary Materials. Rabbit IgGs against the CgA368-373 epitope (PGPQLR) were purified by affinity chromatography on protein A-sepharose as previously described (8). CgA1-439 and fragments were detected in plasma samples using five sandwich ELISAs based on antibodies against different CgA regions (see Supplementary Methods and Figure S1 for schematic representation of antibody epitopes and assays, and Table S1 for analyte specificity of each assay) (8, 12). These assays can selectively detect: a) full-length CgA (CgA1-439−ELISA); b) full-length CgA with or without the C-terminal sequence 437-439 (CgA1-436/439−ELISA); c) CgA1-372 and CgA1-373 (CgA1-372/373−ELISA); d) CgA1-76 (CgA1-76−ELISA); e) full-length CgA and fragments containing the N-terminal region plus part or the entire central and C-terminal regions, but not CgA1-76 (defined here as “CgAtotal”) (CgAtotal−ELISA) (12).
The cumulative amounts of large fragments lacking the region 410-430 region (e.g., CgA1-409, CgA1-394, CgA1-373, plus other potential fragments with unknown C-terminus), were calculated as the difference between CgAtotal and CgA1-436/439 and collectively called CgA1-x.
Cells
Human PDAC cells BxPC-3, Hs766T, MiaPaCa-2, PT45, A8184, murine PDAC cells DT6606, cancer-associated fibroblasts, and human monocytes were obtained and cultured as described in Supplementary Methods.
Endothelial Spheroid Capillary Sprouting Assay
Endothelial cell spheroid assays were performed using human umbilical vein endothelial cells as described (6, 8) (see also Supplementary Methods).
In Vivo Studies in PDAC Animal Models
All procedures on mice were approved by the San Raffaele Institutional Animal Care and Use Committee, and by the Ministero della Salute of Italy (Author. 596/2016PR and 637). The study was performed at the San Raffaele Hospital (authorized organization) according to institutional guidelines and in compliance with national and international law and policies. Eight-week-old Rag2-/-γc-/- male mice (CIEA and Taconic, Japan) were challenged subcutaneously with 5x106 BxPC-3 or HS766T cells, resuspended in 0.9% sodium chloride and mixed with Cultrex® Basement Membrane Matrix. Blood samples from animals were collected weekly until day 35 and, in the case of HS766T-tumor bearing mice, at day 68. Eight-week-old C57BL/6/N female mice (Charles River Laboratories Italia S.p.A., Calco, Italy) were challenged with subcutaneous injection of 2x106 Panc02 cells in 0.9% sodium chloride. Tumor growth was monitored weekly by measuring tumor volumes with calipers. The animals were sacrificed when tumor diameter reached 1–1.5 cm.
Syngeneic eight-week-old C57BL/6N male mice (Charles River) were orthotopically injected with DT6606 PDAC cells. To this aim 1x106 cells/50 µl in phosphate-buffered saline containing 25% Growth Factor Reduced Matrigel (BD Biosciences) were injected into the pancreas of mice as previously described, with minor modification (16). Mice were treated with CgA (1.5 µg/mouse, i.p. twice a week, in 0.9% sodium chloride) or with vehicle alone. Tumor perfusion was monitored by contrast-enhanced ultrasound analysis using a high-performance ultrasonographic scanner designed for small animal imaging (Vevo 2100; Visual Sonics) as described (6, 17).
Statistical Methods
Study endpoints were progression-free and overall survival. Cox proportional hazards models with backward selection were used to estimate independent predictors of progression-free and overall survival. Continuous variables were compared using Mann-Whitney U test or Student’s t-test, as appropriate. Statistical analysis was performed using the SPSS 25.0 for Mac software (SPSS Inc., Illinois, USA). P values <0.05 were considered significant.
Results
Full-Length CgA and Various Fragments Are Present in the Peripheral Blood of Healthy Subjects and Patients with PDAC
Plasma levels of CgA and its fragments were measured in 172 patients with locally advanced or metastatic PDAC (Table S2) and 37 age-matched healthy subjects. To this aim we used five different ELISAs (CgA1-439–, CgA1-436/439–, CgA1-372/373–, CgA1-76–, and CgAtotal–ELISA) based on antibodies against different CgA epitopes. These assays and the analytes detected are described in Table S1 and schematically represented in Figure S1.
The results (Table 1) suggest that in heathy subjects circulating CgA consists of a mixture of full-length CgA1-439 (median 0.19 nM) and fragments (CgAtotal, 0.77 nM). CgA1-436/439, which include large molecules containing N-terminal, central and part or entire C-terminal region, accounted to about a half of CgAtotal, suggesting that a conspicuous amount of the circulating CgA (about a half) consisted of CgA1-x, a family of large fragments lacking the entire C-terminal region, some with an undefined C-terminus. Of note, the CgA1-372/373 component of the CgA1-x family was undetectable in normal subjects (Table 1), suggesting that most CgA1-x fragments in healthy subjects were different from CgA1-372/373. The results also show that the N-terminal fragment CgA1-76 was present in the blood of healthy subjects, suggesting that partial cleavage of CgA N- and C-terminal regions occurs in healthy conditions.
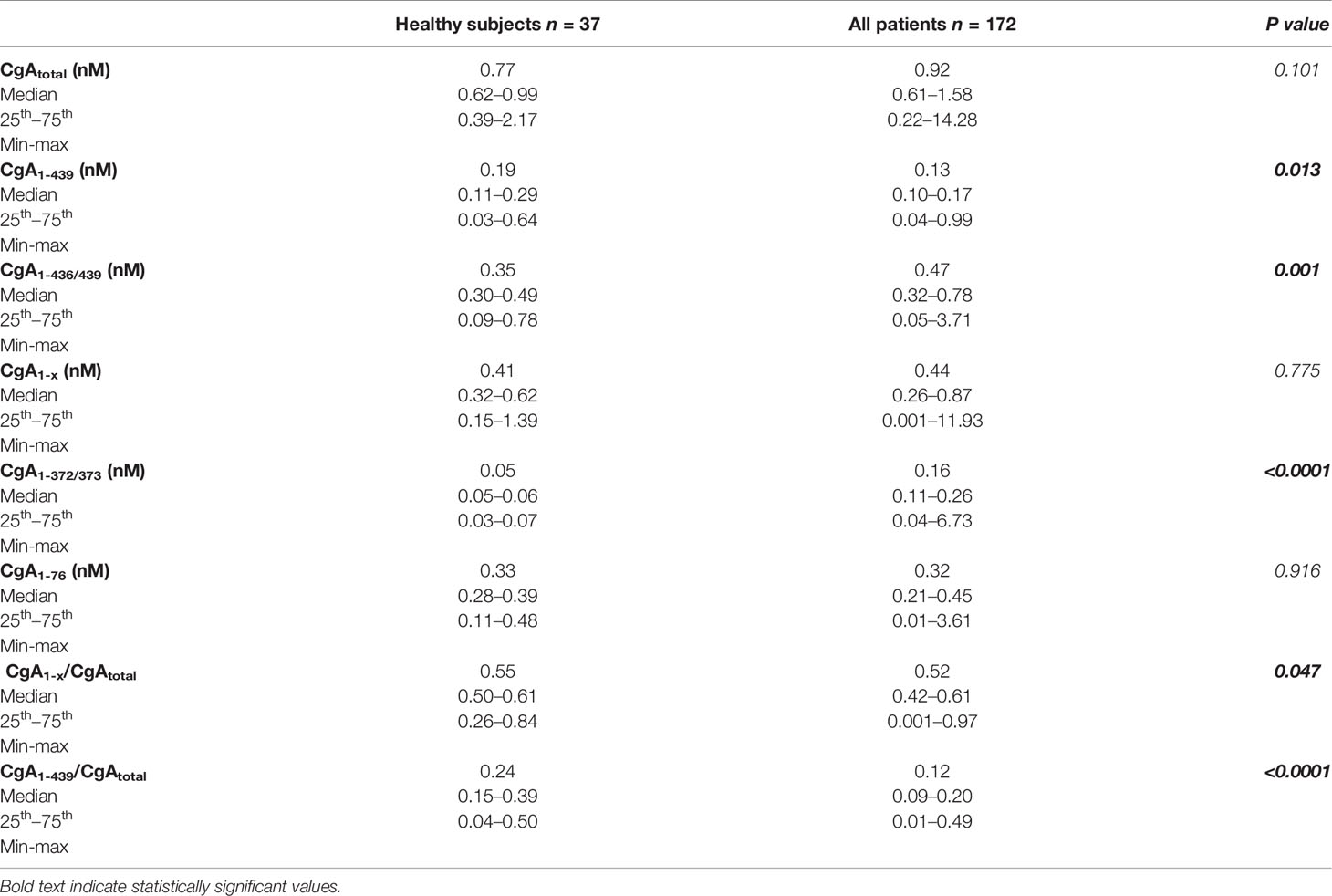
Table 1 Plasma levels of CgA and fragments in patients with pancreatic ductal adenocarcinoma (PDAC) and in healthy subjects.
Table 1 also shows the results of assays performed on plasma samples from PDAC patients. Although CgAtotal was not significantly different in healthy subjects and patients, some differences were observed in terms of fragmentation, particularly at the C-terminal region. Indeed, full-length CgA1-439 was significantly decreased in patients, while CgA1-372/373 was significantly increased (Table 1 and Figure S2). In contrast, similar levels of CgA1-76 were observed in patients and controls. These data suggest that increased CgA fragmentation at its C-terminal region, but not at its N-terminal regions, occurred in patients. Of note, a modest non-significant increase of CgA1-x was observed, suggesting that CgA1-372/373 (which belongs to the CgA1-x family) mainly derive from another larger CgA1-x fragment and in part from full-length CgA. These results, overall, indicate that an important change in the balance between full-length CgA and fragments, particularly those lacking the C-terminal region, occurred in PDAC patients.
Proton Pump Inhibitor Administration Is Not the Cause of Increased CgA Fragmentation
Considering that 56% of the PDAC patients were treated with proton pump inhibitors, a class of drugs that may induce the release of CgA from enterochromaffin-like cells of the gastric mucosa (18, 19), the impact of this treatment on circulating CgA was then assessed. Of note, CgA1-436/439 levels were significantly higher in patients treated with proton pump inhibitors, compared to untreated patients (0.49 vs 0.42 nM, P=0.042), while CgA1-372/373 were similar (Table S3). Furthermore, the CgA1-x/CgAtotal ratio, which represents a sort of C-terminal fragmentation index, was significantly lower in proton pump inhibitors-treated patients (0.49 vs 0.54, P=0.032). Thus, proton pump inhibitor administration was not the cause of increased CgA fragmentation observed in these patients.
Enhanced C-Terminal Cleavage of CgA Predicts Progression-Free and Overall Survival in PDAC Patients
After blood collection, 148 patients (86%) received a combination of cisplatin, epirubicin, capecitabine and gemcitabine, and 24 patients (14%) received the same association with docetaxel in place of epirubicin, for 6 months or until disease progression. Follow up data, progression-free and overall survival are shown in Table S4. Patients with locally advanced PDAC had a median progression-free and overall survival of 10 and 18 months, whereas patients with metastatic disease had a median progression-free and overall survival of 6 and 10 months, respectively. A univariate Cox-regression analysis showed that stage of disease, Karnofsky performance status, and baseline CA19.9, but not CgA and its fragments, were significantly associated with progression-free and overall survival (Table S5). However, multivariate analysis showed that CgA and its fragments, in addition to age, stage, Karnofsky Performance Status, baseline CA19.9, and chemotherapy regimen, were all significantly associated with progression-free and overall survival (Table 2). Full-length CgA (CgA1-439, min-max 0.04–0.99 nM) was a strong predictor of better outcome, both in terms of progression-free survival (hazard ratio: 0.040, P<0.0001) and overall survival (hazard ratio: 0.025, P<0.0001). These data indicate that every unit increase of this analyte provides a 96% risk reduction for progression and a 97.5% risk reduction for death due to any cause. In contrast, a high CgA1-x/CgAtotal ratio, an index of CgA fragmentation in the C-terminal region, was a strong predictor of poor overall survival (hazard ratio: 3.533, P=0.015), with every unit of this index providing a 253% risk increase for death due to any cause. An association with a worse prognosis was found also for CgA1-372/373 (progression-free survival, hazard ratio: 1.522, P=0.020) and for CgA1-436/439 (overall survival, hazard ratio: 1.595, P=0.006). No association was observed with CgA1-76 or CgA1-76/CgAtotal ratio. In conclusion, the results of the immunoassays suggest that enhanced fragmentation of circulating CgA and consequent decrease of full-length CgA and increase of fragments lacking the C-terminal region is associated with worse outcome in PDAC patients.
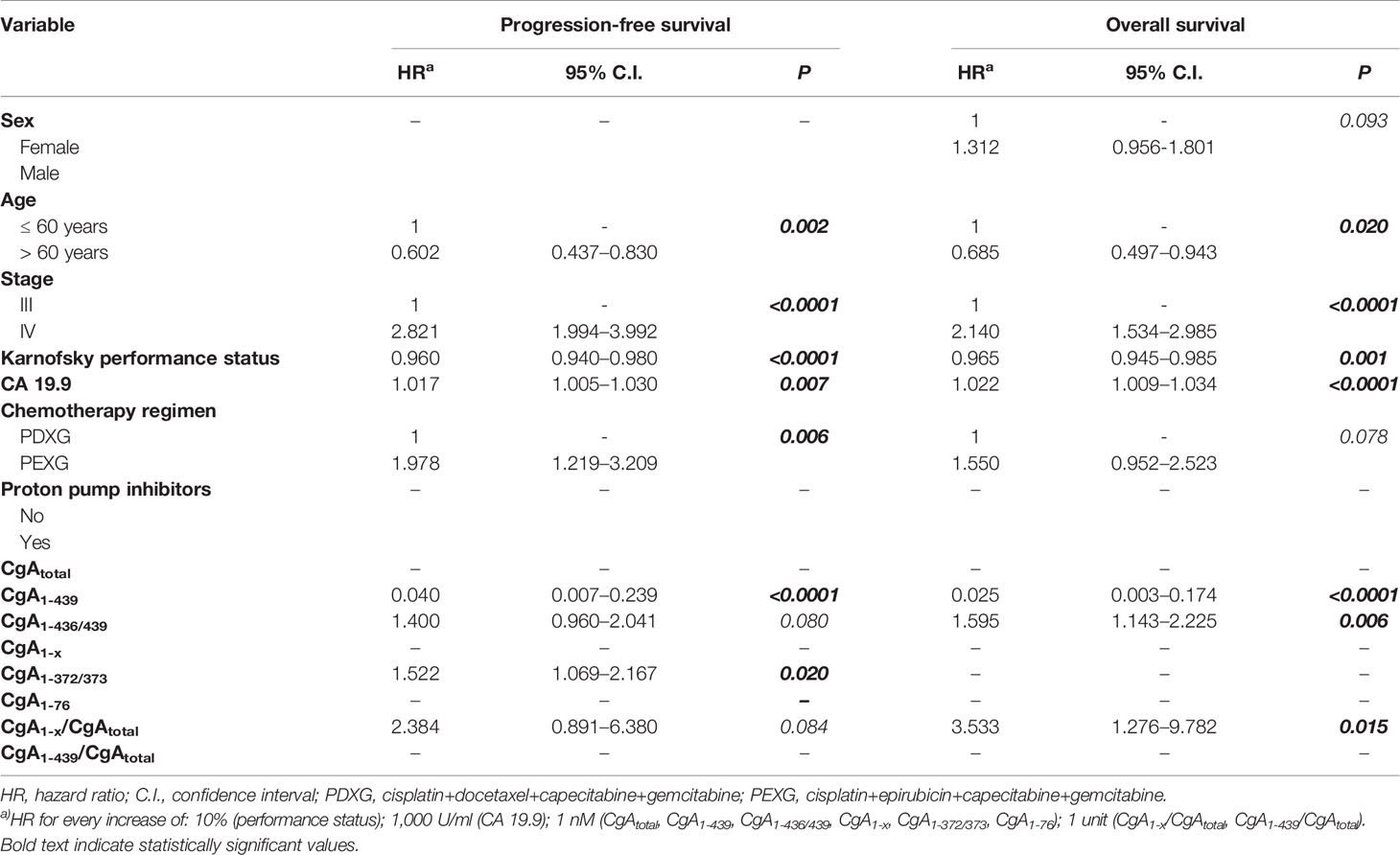
Table 2 Multivariate Cox-regression analysis evaluating predictors of progression-free survival and overall survival (OS) in patients with locally advanced or metastatic pancreatic ductal adenocarcinoma (PDAC) (n=172).
Pancreatic Cancer Cell Lines Promote CgA C-Terminal Cleavage By Activating Plasminogen to Plasmin
The mechanism of CgA fragmentation was then investigated. To investigate the role of cancer cells on CgA fragmentation we incubated recombinant CgA1-439 in the presence or absence of various human PDAC cell lines (Hs766T, MiaPaCa-2, BxPC-3, A8184, PT45, and PaCa44 cells) or murine Panc02 cells. CgA fragmentation in cell supernatants was monitored using CgA1-439– and CgAtotal–ELISA. After 30 min of incubation, little or no changes in CgA1-439/CgAtotal ratio were observed with all cell lines tested, arguing against a direct role of PDAC cells in CgA fragmentation. However, when plasminogen was added to the cultures, a marked reduction of CgA1-439/CgAtotal ratio was observed with the majority of PDAC cell lines (Figure 1). The addition of plasminogen activator inhibitor 1 (PAI-1) or of aprotinin (an inhibitor of plasmin and other serine proteases) significantly inhibited the degradation of CgA by most PDAC cell lines, pointing to a crucial role of plasminogen activators and plasmin in this process (Figure 1). These data suggest that PDAC cells can promote CgA cleavage by releasing plasminogen activators and, consequently, by converting plasminogen into active plasmin. Similar experiments were performed with human cancer-associated fibroblasts isolated from two PDAC (CAF-1 and CAF-2) or with human monocyte-derived macrophages. No significant degradation was observed with cancer-associated fibroblasts after 30 min, even in the presence of plasminogen (Figure 1). However, partial degradation was observed after 3 h of incubation, suggesting that also these cells may contribute to CgA degradation, although less efficiently than cancer cells. The same applies also for human monocyte-derived macrophages (Figure 1). Considering that both cell types may represent a large component of the tumor tissues, their contribution to CgA degradation could also be relevant.
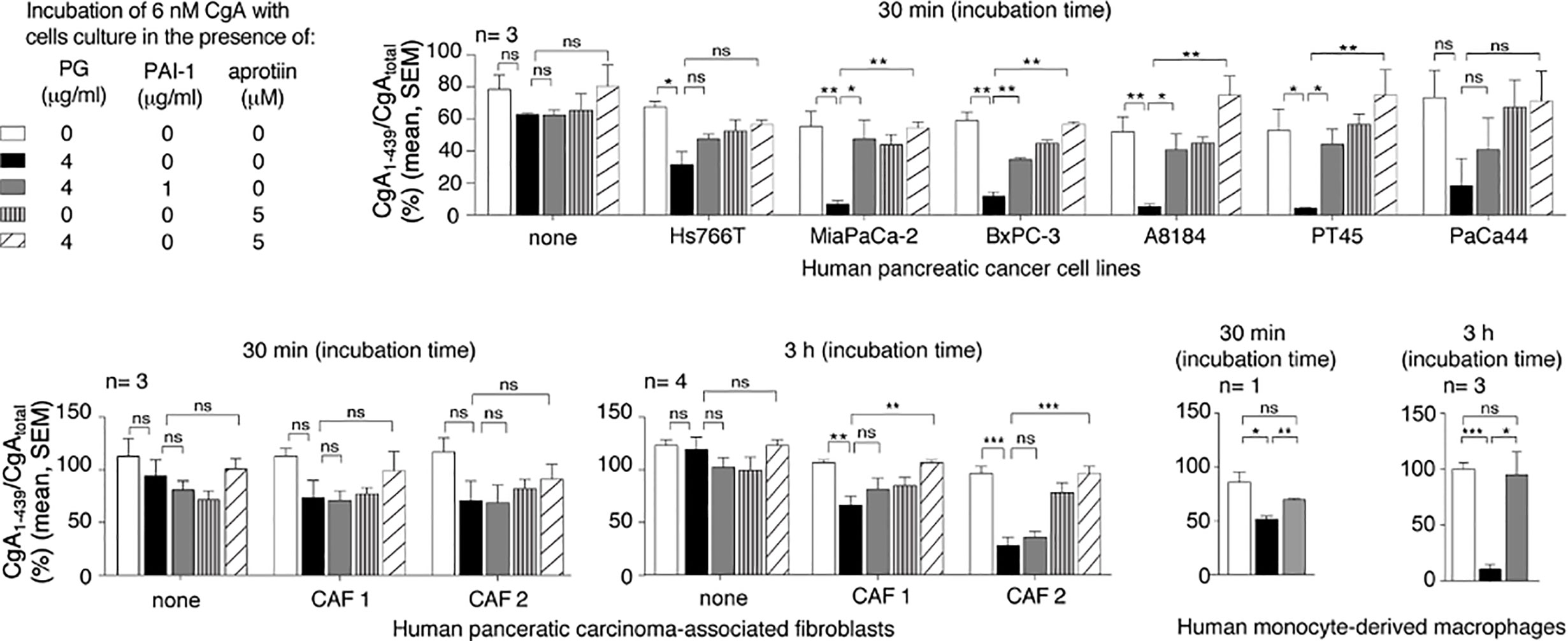
Figure 1 Effect of pancreatic ductal adenocarcinoma (PDAC) cells, pancreatic adenocarcinoma-associated fibroblasts and human monocyte-derived macrophages on CgA cleavage. Human PDAC cells (Hs766T, MiaPaCa-2, BxPC-3, A8184, PT45, PaCa44), PDAC-associated fibroblasts (CAF1 and CAF2) and monocyte-derived macrophages (5x105 cells/well of 96-well plates) were cultured for 24–48 h at 37°C. After washing, recombinant CgA1-439 (6 nM), plasminogen (PG) (4 µg/ml), plasminogen activator inhibitor-1 (PAI-1) (1 µg/ml) and aprotinin (5 µM) were added to cell culture medium containing 0.5% of bovine serum albumin, and further incubated for 30 min or 3 h at 37°C, as indicated. The supernatants were collected and analysed by CgA1-439– and CgAtotal–ELISAs. The CgA1-439/CgAtotal ratio, which represent an index of CgA integrity, is shown. Bars (mean ± SEM); *P < 0.05; **P < 0.01; ***P < 0.001 (t-test, two tails); ns, not significant.
The C-Terminal Region of CgA Is More Prone to Plasmin Cleavage Than the N-Terminal Region
To gain more information on the sites cleaved by plasmin, full-length CgA was incubated with a small amount of this enzyme for various time intervals. The products were then analysed by SDS-PAGE and mass spectrometry. After 6 h of incubation, SDS-PAGE showed major bands corresponding to undigested CgA (70 kDa), large fragments of about 60–65 kDa, and ~10 kDa small fragments (Figure 2A, left). The large 60–65 kDa fragments, but not the ~10 kDa fragments, were recognized by an antiserum against the central region of CgA (αFRs), by western blot analysis (Figure 2A, right), pointing to N- or C-terminal cleavage. The large fragments were not detectable by mass spectrometry for unknown reasons. However, this assay could identify a series of other sub-fragments, present in smaller amounts, which were consistent with limited digestion at various sites in the C-terminal region (residues 246–439), but not in the N-terminal region (1–245) of CgA (Figure 2B). Notably, fragmentation of the N-terminal part required longer time to occur (96 h), which implies that the N-terminal part is more resistant than the C-terminal part to plasmin digestion. Taking into consideration the molecular weight of the major fragments (60–65 kDa, determined by SDS-PAGE) and the cleavage sites identified by mass spectrometry after 6 h, we estimate that the large fragments observed by SDS-PAGE and western blotting correspond to CgA cleavage in the C-terminal region, presumably at residues 394, 373, and/or 355/359. Indeed, cleavage at these sites is expected to shorten the molecule of 45–84 residues (~5-10 kDa), as observed by SDS-PAGE. In conclusion, these results suggest that limited digestion with plasmin can generate large fragments ending with residues 394, 373, and/or 355/359, which are all components of the CgA1-x family. This view is in keeping with previous observation showing that an antibody specific for the PGPQLR epitope of CgA1-373 (cryptic in intact CgA) could recognize a 60–62 kDa band by western blotting analysis of CgA digested with plasmin for 2–6 h (12), likely corresponding to the CgA1-373 analyte detected in plasma samples of PDAC patients (Figure S2).
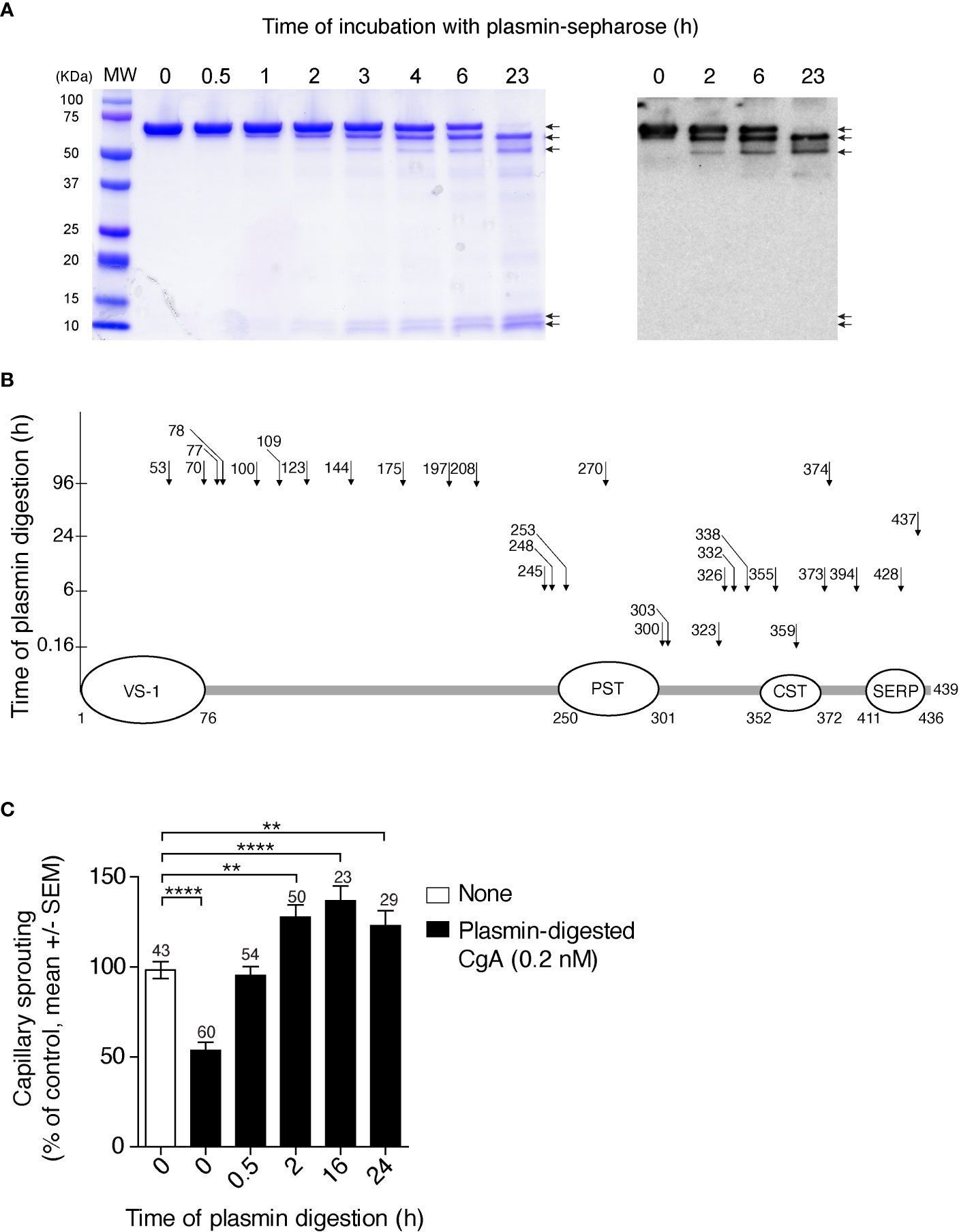
Figure 2 Time course of CgA cleavage by plasmin, cleavage sites, and anti-/pro-angiogenic activity before and after cleavage. (A) SDS-PAGE and western blotting analysis of CgA before and after digestion with plasmin. Recombinant full-length CgA1-439 (9 µM, 100 µl) was incubated with plasmin-Sepharose beads (BioVision) (10 µl) in 0.05 M sodium chloride, 0.15 M sodium phosphate buffer, pH 7.3, for the indicated time. At various time points, the beads were removed, by centrifugation, and the supernatants were analysed by SDS-PAGE (Coomassie dye staining) (left panel) and western blotting with the αFRs antiserum against the central region of CgA (right panel). MW, molecular markers. (B) Schematic representation of full-length CgA and its plasmin cleavage sites (arrows and number of cleaved residue) identified by mass spectrometry analysis of the low molecular weight fragments obtained by limited and extensive digestion of CgA with plasmin-sepharose. The time of digestion is indicated on the y axis. As shown the C-terminal region is more prone to proteolysis than the N-terminal region, the latter requiring 96 h of incubation for cleavage. The regions corresponding to vasostatin-1 (VS-1); pancreastatin (PST); catestatin (CST); and serpinin (SERP) are indicated. (C) Effect of plasmin cleavage on the anti-angiogenic activity of full-length CgA, as measured using the endothelial-spheroid capillary-sprouting assay. In a separate digestion experiment, plasmin-Sepharose was removed by centrifugation at the indicated time points; the supernatants, diluted to 0.2 nM CgA, were incubated overnight with endothelial cell spheroids. Each bar represents the number of capillaries sprouting from the spheroids (mean ± SEM, see Methods). The number of spheroids analysed for each time point is indicated on each bar. **P <0.01; ****P <0.0001 (t-test, two tails).
Limited Digestion With Plasmin Converts Anti-Angiogenic Full-Length CgA Into Pro-Angiogenic Fragments
We have previously shown that CgA1-439 and CgA1-373 are anti- and pro-angiogenic molecules, respectively (5). To assess the impact of plasmin digestion on the biological activity of CgA1-439, we analysed its anti-/pro-angiogenic activity before and after exposure to plasmin, using the endothelial cell spheroid capillary sprouting assay. As expected, limited digestion of CgA1-439 caused loss of anti-angiogenic activity and gain of pro-angiogenic effects, as indicated by the lower number of capillary-like sprouts induced by CgA before digestion and by the higher number of sprouts after 2–16 h of incubation, compared to untreated spheroids (Figure 2C). Extensive digestion for 24 h reduced the pro-angiogenic effects. These results suggest that limited digestion of CgA C-terminal region by plasmin is associated with a marked change of function, from anti- to pro-angiogenic.
CgA C-Terminal Fragmentation Is Increased in Mouse Models of PDAC
To assess whether PDAC cells can cause CgA fragmentation also in vivo, we monitored CgA levels in plasma samples obtained from C57BL/6 mice bearing subcutaneous Panc02 tumors (Figure 3A), using CgA1-439– and CgAtotal–ELISA (taking advantage from antibody cross-reactivity with the murine full-length CgA1-445 and CgAtotal). A significant decrease of full-length CgA/CgAtotal ratio was observed from day 3 to day 31-48 after tumor implantation (Figure 3B, left). This finding is consistent with an increased proteolytic processing of circulating CgA upon tumor growth. Of note, the full-length CgA/CgAtotal ratio negatively correlated with tumor volume (Figure 3B, right), likely because large amounts of protease are produced by large tumors. Similar results were observed also in other models based on human BxPC-3 or Hs766T PDAC cells implanted subcutaneously in Rag2-/-γc-/- mice (Figures 4A–C). Interestingly, in the BxPC-3 model the tumor burden positively correlated with CgA1-x/CgAtotal ratio (Figure 4B, right), pointing again to enhanced C-terminal fragmentation by larger tumors. These findings suggest that also in these models tumor growth was associated with an increased CgA fragmentation, particularly in the C-terminal region, as observed in patients.
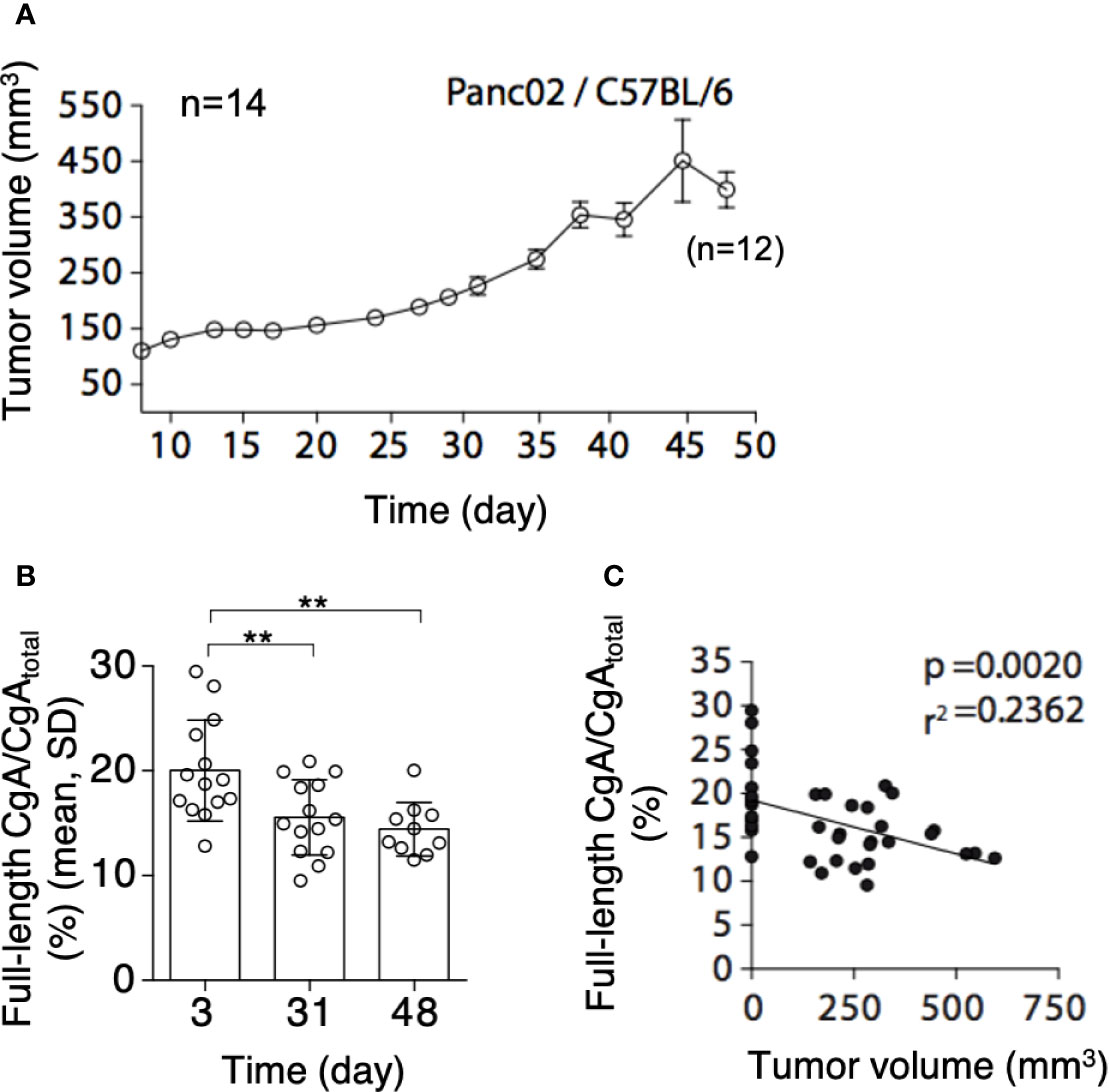
Figure 3 Tumor growth and CgA fragmentation in the murine Panc02 model. (A) Growth rate of Panc02 tumors implanted subcutaneously in syngeneic mice. Tumor volumes were measured with calipers (mean± SEM, n=14). As 2 mice died at day 48, the data reported at this time point represent the tumor volumes of 12 mice. (B) Endogenous full-length CgA/CgAtotal ratio (an index of CgA integrity) as measured at day 3, 31 and 48 after tumor implantation. Endogenous full-length CgA and CgAtotal were measured by ELISA of plasma samples collected at day 3 and 31 (n=14). Only 10 plasma samples (from 12 surviving mice) were collected in sufficient amount for analysis at day 48. Bars (mean ± SD); **, P <0.01 (unpaired t-test). (C) Correlation between full-length CgA/CgAtotal ratio and tumor volumes at day 3, 31, and 48. The tumor volumes at day 3, undetectable, were considered <1 mm3.
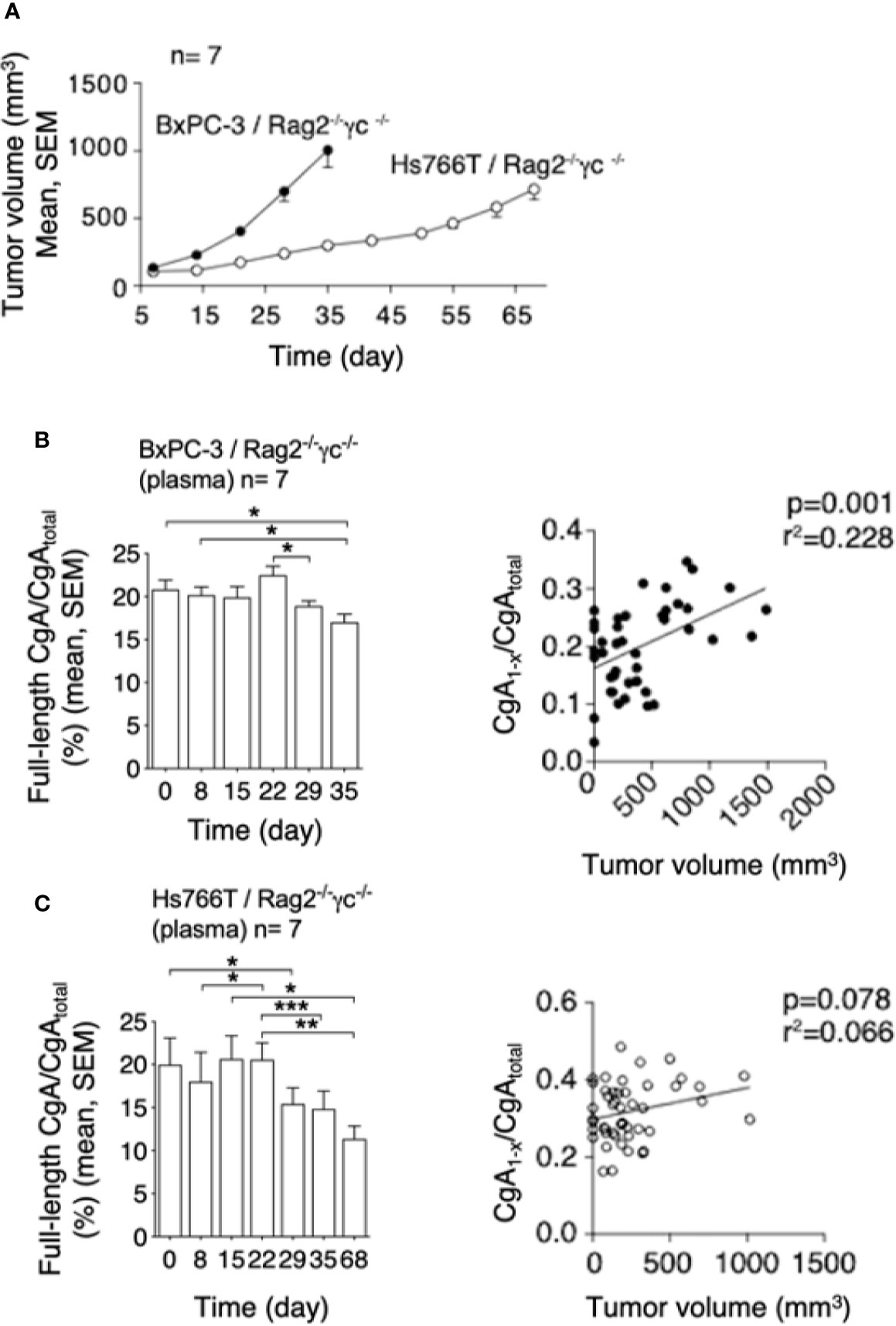
Figure 4 Tumor growth and CgA fragmentation in the human BxPC-3 and Hs766T models. (A) Growth rate of BxPC-3 and Hs766T tumors implanted subcutaneously in Rag2-/-γc-/- mice (n=7). Tumor growth was monitored with calipers. (B, C) Left panels: endogenous full-length CgA/CgAtotal ratio (an index of CgA integrity) at the indicated time points after tumor implantation. Circulating full-length CgA and CgAtotal were measured by ELISA of plasma samples collected at the indicated time. Bars (mean ± SEM); *P <0.05; **P <0.01; ***P <0.001 (paired t-test). Right panels: correlation between CgA1-x/CgAtotal ratio (an index of CgA C-terminal degradation) and tumor volume, as measured in various mice at various times.
The Growth of Subcutaneous Panc02 Tumors Is Inhibited By Administration of Aprotinin or Anti-PGPQLR Antibodies
To assess the hypothesis that CgA fragmentation has a role in the regulation of tumor progression, mice bearing subcutaneous Panc02 tumors were treated with aprotinin (to inhibit CgA fragmentation). A significant reduction of the full-length CgA/CgAtotal ratio was observed from day 24 to 39 in mice treated with the diluent, pointing to CgA cleavage, but not in mice treated with aprotinin (Figure 5A, left). These data suggest a role of a serine protease, possibly plasmin, in CgA cleavage. Notably, aprotinin could also reduce the tumor growth rate (Figure 5A, right), suggesting that CgA fragmentation contributed to tumor growth regulation.
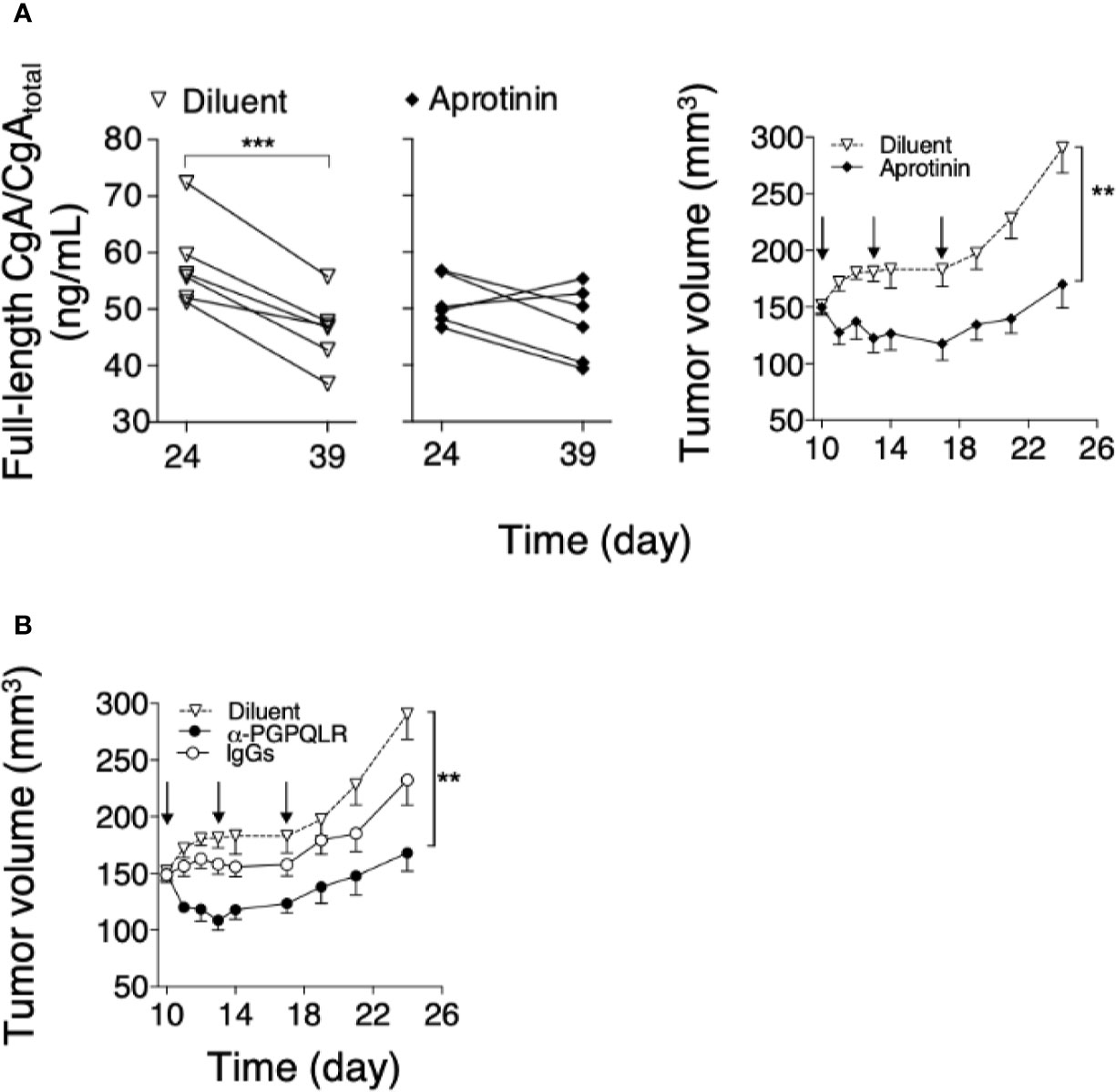
Figure 5 Effect of aprotinin or anti-PGPQLR antibodies on Panc0-tumor growth in mice. (A) Effect of aprotinin on the degradation of circulating CgA (left) and on the growth of Panc02-tumors (right). Panc02 tumor-bearing mice (n=6/group) were treated at day 10, 13 and 17 (arrows) with intra-peritoneal injections of aprotinin (100 μg/mouse) or diluent (saline solution). Plasma samples were collected from each mouse at day 24 and 39 and analyzed by full-length CgA–ELISA and CgAtotal–ELISA. The full-length CgA/CgAtotal ratio (an index of C-terminal integrity) was then calculated (left panels); ***P <0.001 (paired t-test). Tumor volumes (right panel) were measured with calipers (mean ± SEM); **P <0.01 (unpaired t-test). (B) Effect of anti-PGPQLR (Pro-Gly-Pro-Gln-Leu-Arg) antibodies (capable of neutralizing the neuropilin-1 binding site of CgA1-373) on Panc02-tumor growth. Tumor-bearing mice were treated (i.p) at the indicated time (arrows) with 50 μg/mouse of rabbit anti-PGPQLR IgGs or control IgGs, or saline solution (Diluent). Tumor volumes (mean ± SEM); **P <0.01 (two tailed t-test).
To assess this hypothesis, Panc02-bearing mice were injected with anti-PGPQLR IgGs, previously shown to a) neutralize the neuropilin-1 binding site of human CgA1-373 (PGPQLR373), b) cross-react with the corresponding site of its murine counterpart (100% conserved), and c) inhibit tumor growth in various murine models of solid tumors (8). Systemic administration of these antibodies, but not of normal rabbit IgGs, significantly reduced tumor growth (Figure 5B). As these antibodies cannot cross-react with the cryptic PGPQLR site of full-length CgA (8), these data support the hypothesis that CgA cleavage at this site contributed to tumor growth.
Recombinant CgA Can Affect Tumor Perfusion in an Orthotopic Model
Finally, we investigated the effect of exogenous CgA on tumor vasculature and perfusion using DT6606 PDAC cells implanted orthotopically in the pancreas of syngeneic mice. To this aim, tumor-bearing mice were treated twice a week with 1.5 µg of full-length CgA (i.p.). Tumor perfusion was monitored one day before treatment (day 13) and three days after the last treatment (day 41), by contrast-enhanced ultrasound analysis with microbubbles. A significant decrease of the final/basal ratio of the wash-in area under the curve (WiAUC) and rise time (RT) of microbubbles was noted in animals treated with CgA (Figure 6). These data suggest that full-length CgA could affect the tumor vascular physiology and reduce perfusion of tumor tissues in this PDAC model.
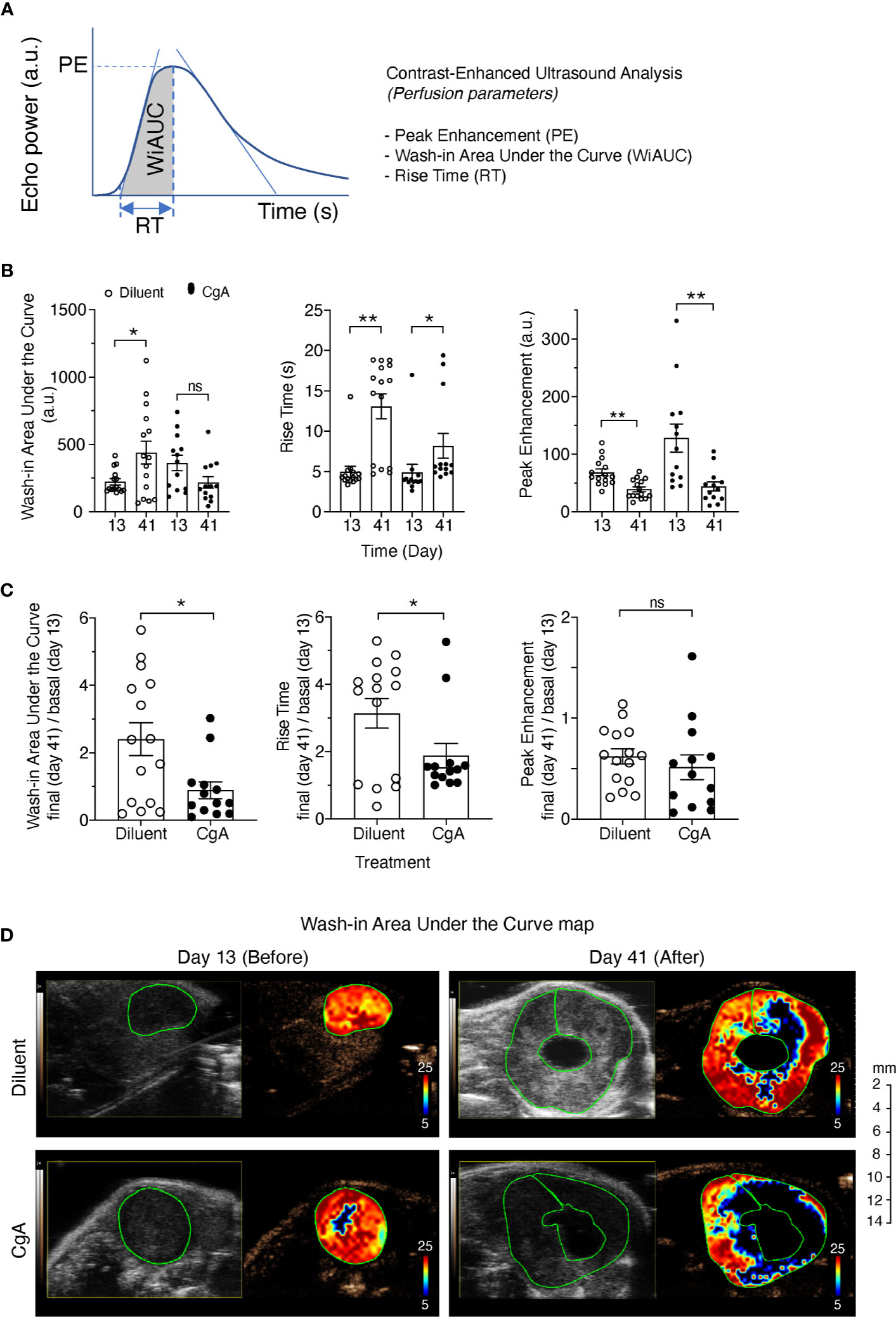
Figure 6 Effect of CgA on the perfusion of orthotopic DT6606 pancreatic ductal adenocarcinoma (PDAC). DT6606 cells were implanted in the pancreas of syngeneic mice and treated with recombinant CgA1-439 (1.5 µg/mouse, i.p. twice a week from day 14 to day 38, eight times) in 0.9% sodium chloride (Diluent) or with Diluent alone (n=15 mice/group). Tumor perfusion was monitored by contrast-enhanced ultrasound analysis one day before treatment (day 13) and three days after the last treatment (day 41). Perfusion parameters were calculated using a region of interest (ROI) corresponding to the entire tumor area [see (A) for a graph showing the parameters analyzed]. Perfusion parameters were: Peak Enhancement (PE, difference between the maximum amplitude in the curve and the baseline level, indicative of relative blood volume); Wash-in Area Under the Curve (WiAUC, area under the curve from starting enhancement to peak enhancement); rise time (RT, time frame during which intensity varies from 5%–95% of PE). (B) Tumor perfusion parameters at day 13 and 41 of mice treated with CgA or with Diluent. (C) Relative values of perfusion parameters at day 41 (final) over day 13 (basal). Outlier points were identified using the Prism software (ROUT, Q=5%) and excluded. *P <0.05; **P <0.01 (two-tailed t-test); ns: not significant. (D) Gray scale tumor images and color-coded Wash-in Area Under the Curve (WiAUC) maps of representative tumors (with intra-tumoral cystic lesions) at day 13 and day 41, treated with Diluent or CgA as indicated. Green lines indicate the ROI analyzed.
Discussion
The results show that fragmentation of the circulating pool of CgA is associated with shorter progression-free and overall survival in patients with PDAC. In particular, a decrease of full-length CgA and a relative increase of fragments lacking all or part of the C-terminal region (CgA1-x) were found in plasma samples of patients with worse prognosis (Table 2). Although the N-terminal fragment CgA1-76 was also detected in these patients, only the CgA1-x/CgAtotal ratio, and not the CgA1-76/CgAtotal (i.e., C- and N-terminal fragmentation indexes, respectively), was associated with patient prognosis. It appears, therefore, that fragmentation of the CgA C-terminal region is more relevant than fragmentation of the N-terminal region for patient prognosis.
Considering that administration of proton pump inhibitors is known to induce enterochromaffin-like cell hyperplasia and CgA secretion from the gastric mucosa (18, 19), the question arises as to whether these drugs induced the change in the circulating pool of full-length CgA and fragments observed in PDAC patients. This hypothesis is ruled out by the observation that patients taking proton pump inhibitors had a lower C-terminal fragmentation index, compared to untreated patients (Table S3). These and the above findings raise the question as to whether enhanced CgA cleavage in PDAC patients, e.g., at residue R373, occurred in neuroendocrine secretory cells (by intragranular or extracellular proteases), in the blood, or in tumors, by locally produced proteases. The results of a series of in vitro and in vivo studies performed with PDAC experimental models suggest that CgA fragments were produced in tumor tissues, by tumor-derived proteases. In particular, in vitro studies performed with various cultured human PDAC cell lines showed that these cells can promote the cleavage of exogenous full-length CgA added to the cell cultures (Figure 1). Interestingly, no cleavage was observed when plasminogen was omitted or when plasminogen activator inhibitor-1 was added to the cell cultures, pointing to an important role of plasminogen activators and plasmin in CgA cleavage. Accordingly, also aprotinin, an inhibitor of plasmin and other serine proteases, could inhibit CgA cleavage by these cells. CgA cleavage was observed also with cultured human monocyte-derived macrophages or with cancer-associated fibroblasts derived from PDAC. Although macrophages and cancer-associated fibroblasts required much longer time than cancer cells to induce CgA cleavage, also these cells might significantly contribute to CgA cleavage in patients, given their considerable abundance in the tumor stroma. Thus, various kinds of cells potentially present in the tumor tissue may contribute, to a different extent, to CgA cleavage.
This view is further supported by the results of in vivo studies, showing that the growth of murine Panc02, human BxPC3, or human Hs766T PDAC cells implanted subcutaneously in mice is associated with increased fragmentation of circulating CgA (Figures 3 and 4). Notably, a significant correlation between tumor volume and CgA cleavage was observed in the Panc02 and BxPC3 models, possibly because large tumors could release more proteolytic enzymes. Furthermore, in the Panc02 model, aprotinin could significantly inhibit CgA fragmentation (Figure 5), pointing again to a role of a serine protease in CgA cleavage.
The capability of plasmin to preferentially cleave the C-terminal region of CgA is supported by the results of SDS-PAGE, western blotting, and mass spectrometry analysis of CgA fragments obtained after limited or extensive digestion of recombinant CgA with plasmin (Figure 2). Indeed, analysis of the time course of CgA degradation with these techniques showed that certain sites located in the C-terminal region, e.g., at residue 355, 359, 373, and/or 394, are more prone to plasmin cleavage than sites located in the N-terminal region, the latter sites requiring a markedly longer time of incubation for cleavage. Thus, the results of in vitro and in vivo studies suggest that PDAC can promote the cleavage of CgA C-terminal region by activating plasminogen to plasmin, which in turn preferentially cleaves CgA in the C-terminal region. Interestingly, previous studies have shown that PDAC cells are characterized by increased expression of urokinase-type plasminogen activator (uPA) and uPA receptor, compared to normal pancreatic tissue, and by reduced expression of plasminogen activator inhibitor-1 (PAI-1) (20–22), indicating that indeed this proteolytic machinery is activated in PDAC. Of course, we cannot exclude that other proteases are also involved.
Given these premises, the question arises as to whether CgA cleavage is just an epiphenomenon of tumor growth or whether it can affect tumor physiology. The following observations suggest that CgA cleavage may contribute to regulate tumor growth. First, we have previously shown that CgA1-439 and CgA1-76 can regulate the adhesion of fibroblasts and endothelial cells, enhance the endothelial barrier function, and inhibit angiogenesis, whereas the fragment CgA1-373 can stimulate angiogenesis (5, 23, 24). In particular, full-length CgA and the N-terminal fragment CgA1-76 can inhibit endothelial cell migration, motility, sprouting, invasion and capillary-like structure formation induced by vascular endothelial growth factor, as well as angiogenesis induced by fibroblast growth factor-2 (7, 11). Vasostatin-1 can also inhibit, in endothelial cells, the nuclear translocation of hypoxia inducible factor-1α, a master regulator of angiogenesis (7, 11). On the other hand, other fragments, such as CgA1-373 and CgA352-372, can induce the release of fibroblast growth factor-2 from endothelial cells and promote angiogenesis (5, 14). Thus, CgA-related circulating polypeptides may form a balance of vasoactive factors, sometimes endowed of opposite effects, tightly regulated by N- and C-terminal proteolysis. According to this view, our results show that limited digestion of CgA with plasmin can progressively convert its anti-angiogenic into pro-angiogenic activity in the endothelial cell-spheroid capillary-sprouting assay, whereas extensive digestion abrogated both effects (Figure 2C). It appears, therefore, that CgA degradation by plasmin represents a mechanism for the fine regulation of PDAC vascular biology and growth. This hypothesis is further supported by the results of in vivo studies showing that inhibition of CgA cleavage in Panc02-bearing mice, by systemic administration of aprotinin, is associated with a lower tumor-growth rate. Furthermore, systemic administration of antibodies against the binding site of CgA1-373 for neuropilin-1 (PGPQLR), an important receptor for its pro-angiogenic activity (8), significantly reduced Panc02-tumor growth (Figure 5). Notably, these antibodies cannot react with the cryptic PGPQLR site of full-length CgA (8), suggesting that this epitope, exposed in fragments, might represent a therapeutic target for specific antibodies. Finally, the results of contrast-enhanced ultrasound analysis of tumors implanted in the pancreas of mice, showing that systemic administration of exogenous low-dose CgA1-439 can cause a significant reduction of tumor perfusion with microbubbles (Figure 6), lends further support to the hypothesis that this protein can indeed regulate the tumor vascular biology.
In conclusion, our findings suggest that PDAC can promote the cleavage of circulating CgA C-terminal region into vasoactive fragments, with important implications for PDAC pathophysiology and patient prognosis. Because of its potential pathophysiological function in the regulation of tumor biology and growth, CgA may represent a potential therapeutic target that warrants further exploration.
Data Availability Statement
The raw data supporting the conclusions of this article will be made available by the authors, without undue reservation.
Ethics Statement
The studies involving human participants were reviewed and approved by San Raffaele Hospital Ethics Committee. The patients/participants provided their written informed consent to participate in this study. The animal study was reviewed and approved by San Raffaele Hospital Ethics Committee and by Ministero della Salute of Italy.
Author Contributions
Conception/design: MR, AC. Data acquisition: VA, AG, ED, BC, MM, MB, AD, ACi, EA, MP, and FC. Data analysis/interpretation: MR, VA, AMG, ED, MM, MB, AD, ACi, EA, MPP, AE, MF, FC, LP, and AC. Drafting: MR, VA, and AC. Critical revision: MR, VA, AG, ED, BC, MM, MB, AD, ACi, EA, MP, AE, MF, FC, LP, and AC. All authors contributed to the article and approved the submitted version.
Funding
This work was supported by Associazione Italiana per la Ricerca sul Cancro (AIRC, grant IG-19220 and IG-23470, P.I. A Corti) and Fondazione AIRC under 5 per Mille 2019 – ID. 22737 program – P.I. MC Bonini, Group leader, A Corti.
Conflict of Interest
The authors declare that the research was conducted in the absence of any commercial or financial relationships that could be construed as a potential conflict of interest.
Acknowledgments
VA PhD studentship was supported by Ms Bianca Costanza Gioja Legacy Donation. We thank Laura Perani (Preclinical Imaging Facility) for the technical support provided for the in-vivo CEUS analysis of mice, and Annapaola Andolfo (Proteomic and Metabolomic Facility of San Raffaele Institute) for mass spectrometry analyses.
Supplementary Material
The Supplementary Material for this article can be found online at: https://www.frontiersin.org/articles/10.3389/fonc.2020.613582/full#supplementary-material
Abbreviations
CgA, chromogranin A; CTS, catestatin; PDAC, pancreatic ductal adenocarcinoma; PPI, proton pump inhibitors; PST, pancreastatin; SERP, serpinin; VS1, vasostatin-1.
References
1. Kleeff J, Korc M, Apte M, La Vecchia C, Johnson CD, Biankin AV, et al. Pancreatic cancer. Nat Rev Dis Primers (2016) 2:16022. doi: 10.1038/nrdp.2016.22
2. Siegel RL, Miller KD, Jemal A. Cancer statistics, 2018. CA Cancer J Clin (2018) 68(1):7–30. doi: 10.3322/caac.21442
3. Rawla P, Sunkara T, Gaduputi V. Epidemiology of Pancreatic Cancer: Global Trends, Etiology and Risk Factors. World J Oncol (2019) 10(1):10–27. doi: 10.14740/wjon1166
4. Conroy T, Desseigne F, Ychou M, Bouche O, Guimbaud R, Becouarn Y, et al. Tumeurs Digestives of and P. Intergroup: FOLFIRINOX versus gemcitabine for metastatic pancreatic cancer. N Engl J Med (2011) 364(19):1817–25. doi: 10.1056/NEJMoa1011923
5. Crippa L, Bianco M, Colombo B, Gasparri AM, Ferrero E, Loh YP, et al. A new chromogranin A-dependent angiogenic switch activated by thrombin. Blood (2013) 121(2):392–402. doi: 10.1182/blood-2012-05-430314
6. Curnis F, Dallatomasina A, Bianco M, Gasparri A, Sacchi A, Colombo B, et al. Regulation of tumor growth by circulating full-length chromogranin A. Oncotarget (2016) 7(45):72716–32. doi: 10.18632/oncotarget.12237
7. Helle KB, Corti A. Chromogranin A: a paradoxical player in angiogenesis and vascular biology. Cell Mol Life Sci (2015) 78:339–48. doi: 10.1007/s00018-014-1750-9
8. Dallatomasina A, Gasparri AM, Colombo B, Sacchi A, Bianco M, Daniele T, et al. Spatiotemporal Regulation of Tumor Angiogenesis by Circulating Chromogranin A Cleavage and Neuropilin-1 Engagement. Cancer Res (2019) 79(8):1925–37. doi: 10.1158/0008-5472.CAN-18-0289
9. Taupenot L, Harper KL, O’Connor DT. The chromogranin-secretogranin family. N Engl J Med (2003) 348(12):1134–49. doi: 10.1056/NEJMra021405
10. Banks P, Helle KB. The release of protein from the stimulated adrenal medulla. Biochem J (1965) 97:40C–1C. doi: 10.1042/bj0970040C
11. Corti A, Marcucci F, Bachetti T. Circulating chromogranin A and its fragments as diagnostic and prognostic disease markers. Pflugers Arch (2018) 470(1):199–210. doi: 10.1007/s00424-017-2030-y
12. Bianco M, Gasparri AM, Colombo B, Curnis F, Girlanda S, Ponzoni M, et al. Chromogranin A is preferentially cleaved into pro-angiogenic peptides in the bone marrow of multiple myeloma patients. Cancer Res (2016) 76:1781–91. doi: 10.1158/0008-5472.CAN-15-1637
13. Mahata SK, Corti A. Chromogranin A and its fragments in cardiovascular, immunometabolic, and cancer regulation. Ann N Y Acad Sci (2019) 1455(1):34–58. doi: 10.1111/nyas.14249
14. Theurl M, Schgoer W, Albrecht K, Jeschke J, Egger M, Beer AG, et al. The neuropeptide catestatin acts as a novel angiogenic cytokine via a basic fibroblast growth factor-dependent mechanism. Circ Res (2010) 107(11):1326–35. doi: 10.1161/CIRCRESAHA.110.219493
15. Rocca C, Grande F, Granieri MC, Colombo B, De Bartolo A, Giordano F, et al. The chromogranin A1-373 fragment reveals how a single change in the protein sequence exerts strong cardioregulatory effects by engaging neuropilin-1. Acta Physiol (Oxf) (2020) e13570. doi: 10.1111/apha.13570
16. Kim MP, Evans DB, Wang H, Abbruzzese JL, Fleming JB, Gallick GE. Generation of orthotopic and heterotopic human pancreatic cancer xenografts in immunodeficient mice. Nat Protoc (2009) 4(11):1670–80. doi: 10.1038/nprot.2009.171
17. Needles A, Arditi M, Rognin NG, Mehi J, Coulthard T, Bilan-Tracey C, et al. Nonlinear contrast imaging with an array-based micro-ultrasound system. Ultrasound Med Biol (2010) 36(12):2097–106. doi: 10.1016/j.ultrasmedbio.2010.08.012
18. Mosli HH, Dennis A, Kocha W, Asher LJ, Van Uum SH. Effect of short-term proton pump inhibitor treatment and its discontinuation on chromogranin A in healthy subjects. J Clin Endocrinol Metab (2012) 97(9):E1731–5. doi: 10.1210/jc.2012-1548
19. Giusti M, Sidoti M, Augeri C, Rabitti C, Minuto F. Effect of short-term treatment with low dosages of the proton-pump inhibitor omeprazole on serum chromogranin A levels in man. Eur J Endocrinol (2004) 150(3):299–303. doi: 10.1530/eje.0.1500299
20. Harvey SR, Hurd TC, Markus G, Martinick M II, Penetrante RM, Tan D, et al. Evaluation of urinary plasminogen activator, its receptor, matrix metalloproteinase-9, and von Willebrand factor in pancreatic cancer. Clin Cancer Res (2003) 9(13):4935–43.
21. Xue A, Scarlett CJ, Jackson CJ, Allen BJ, Smith RC. Prognostic significance of growth factors and the urokinase-type plasminogen activator system in pancreatic ductal adenocarcinoma. Pancreas (2008) 36(2):160–7. doi: 10.1097/MPA.0b013e31815750f0
22. Warnecke-Eberz U, Prenzel KL, Baldus SE, Metzger R, Dienes HP, Bollschweiler E, et al. Significant down-regulation of the plasminogen activator inhibitor 1 mRNA in pancreatic cancer. Pancreas (2008) 36(2):173–7. doi: 10.1097/MPA.0b013e31815ac538
23. Ferrero E, Scabini S, Magni E, Foglieni C, Belloni D, Colombo B, et al. Chromogranin A protects vessels against tumor necrosis factor alpha-induced vascular leakage. FASEB J (2004) 18(3):554–6. doi: 10.1096/fj.03-0922fje
24. Gasparri A, Sidoli A, Sanchez LP, Longhi R, Siccardi AG, Marchisio PC, et al. Chromogranin A fragments modulate cell adhesion. Identification and characterization of a pro-adhesive domain. J Biol Chem (1997) 272(33):20835–43. doi: 10.1074/jbc.272.33.20835
25. Corti A, Longhi R, Gasparri A, Chen F, Pelagi M, Siccardi AG. Antigenic regions of human chromogranin A and their topographic relationships with structural/functional domains. Eur J Biochem (1996) 235(1-2):275–80. doi: 10.111/j.1432-1033.1996.00275.x
26. Ratti S, Curnis F, Longhi R, Colombo B, Gasparri A, Magni F, et al. Structureactivity relationships of chromogranin A in cell adhesion. Identification of an adhesion site for fibroblasts and smooth muscle cells. J Biol Chem (2000) 275(38):29257–63. doi: 10.1074/jbc.M003796200
27. Celesti G, Di Caro G, Bianchi P, Grizzi F, Marchesi F, Basso G, et al. Early expression of the fractalkine receptor CX3CR1 in pancreatic carcinogenesis. Br J Cancer (2013) 109(9):2424–33. doi: 10.1038/bjc.2013.565
Keywords: pancreatic ductal adenocarcinoma, chromogranin A, proteolytic processing, plasminogen activators, vasostatin-1
Citation: Reni M, Andreasi V, Gasparri AM, Dugnani E, Colombo B, Macchini M, Bianco M, Dallatomasina A, Citro A, Assi E, Protti MP, Esposito A, Falconi M, Curnis F, Piemonti L and Corti A (2020) Circulating Chromogranin A Is Cleaved Into Vasoregulatory Fragments in Patients With Pancreatic Ductal Adenocarcinoma. Front. Oncol. 10:613582. doi: 10.3389/fonc.2020.613582
Received: 02 October 2020; Accepted: 13 November 2020;
Published: 23 December 2020.
Edited by:
Jai Prakash, University of Twente, NetherlandsCopyright © 2020 Reni, Andreasi, Gasparri, Dugnani, Colombo, Macchini, Bianco, Dallatomasina, Citro, Assi, Protti, Esposito, Falconi, Curnis, Piemonti and Corti. This is an open-access article distributed under the terms of the Creative Commons Attribution License (CC BY). The use, distribution or reproduction in other forums is permitted, provided the original author(s) and the copyright owner(s) are credited and that the original publication in this journal is cited, in accordance with accepted academic practice. No use, distribution or reproduction is permitted which does not comply with these terms.
*Correspondence: Michele Reni, reni.michele@hsr.it; Angelo Corti, corti.angelo@hsr.it