- Division of Hematology and Oncology, Department of Medicine, University of California San Francisco, San Francisco, CA, United States
The FLT3 receptor is overexpressed on the majority of acute myeloid leukemia (AML) blasts. Mutations in FLT3 are the most common genetic alteration in AML, identified in approximately one third of newly diagnosed patients. FLT3 internal tandem duplication mutations (FLT3-ITD) are associated with increased relapse and inferior overall survival. Multiple small molecule inhibitors of FLT3 signaling have been identified, two of which (midostaurin and gilteritinib) are currently approved in the United States, and many more of which are in clinical trials. Despite significant advances, resistance to FLT3 inhibitors through secondary FLT3 mutations, upregulation of parallel pathways, and extracellular signaling remains an ongoing challenge. Novel therapeutic strategies to overcome resistance, including combining FLT3 inhibitors with other antileukemic agents, development of new FLT3 inhibitors, and FLT3-directed immunotherapy are in active clinical development. Multiple questions regarding FLT3-mutated AML remain. In this review, we highlight several of the current most intriguing controversies in the field including the role of FLT3 inhibitors in maintenance therapy, the role of hematopoietic cell transplantation in FLT3-mutated AML, use of FLT3 inhibitors in FLT3 wild-type disease, significance of non-canonical FLT3 mutations, and finally, emerging concerns regarding clonal evolution.
FLT3 Epidemiology, Biology, and Prognostic Associations
Acute Myeloid Leukemia (AML) is an aggressive hematologic malignancy characterized by a heterogenous genetic landscape and complex clonal evolution (1). Fms-like tyrosine kinase 3 (FLT3), a member of the receptor tyrosine kinase family, is widely expressed in hematopoietic progenitor cells and is overexpressed on the majority of AML blasts (2). Upon binding to the FLT3 ligand, FLT3 receptors activate and dimerize, leading to conformational change, cellular proliferation, and inhibition of apoptosis and differentiation (3). Mutations in FLT3 are the most common genomic alteration in AML, identified in approximately one-third of newly diagnosed adult patients (4), and are common in pediatric AML as well (5).
FLT3 mutations can be subdivided into internal tandem duplicates (ITD), present in approximately 25% of patients, and point mutations in the tyrosine kinase domain (TKD), present in approximately 5%. Both FLT3-ITD and FLT3-TKD mutations are constitutively activating, leading to ligand-independent FLT3 signaling and cellular proliferation (3).
FLT3-ITD and FLT3-TKD
FLT3-ITD mutations are in-frame duplications of variable size, ranging from 3 to >1,000 nucleotides, and are located within the receptor’s autoinhibitory juxatamembrane domain. In wild type (WT) FLT3, the FLT3 juxtamembrane domain inhibits receptor activation; the presence of ITDs disrupts this inhibitory effect, resulting in constitutive activation (6). Clinically, FLT3-ITD mutated AML is associated with higher rates of relapse and inferior overall survival, although the full prognostic impact is affected both by mutant allele burden and presence of co-existing mutations (7). High allele ratio (AR; FLT3-ITDhigh), generally defined as a FLT3-ITD to FLT3-WT ratio of ≥0.5, is associated with higher disease risk. Low AR (FLT3-ITDlow) is associated with favorable risk in patients with a co-occurrent nucleophosmin 1 (NPM1) mutations and intermediate risk in patients with NPM1-WT. These associations are reflected in the 2017 European LeukemiaNet (ELN) risk stratification of AML (8).
FLT3-TKD mutations are point mutations within the receptor’s activation loop which stabilize the active kinase conformation and also result in constitutive kinase activation (3). In contrast with FLT3-ITD mutant AML, the prognostic relevance of FLT3-TKD mutations is less clear and may be dependent on the presence of co-occurring mutations and cytogenetic changes (9, 10). Currently, the presence of a FLT3-TKD mutation does not alter formal AML risk assessment (8).
Testing Considerations
Given the prognostic and treatment implications, testing for FLT3-ITD in patients with newly diagnosed AML is recommended by both ELN and National Comprehensive Cancer Network (NCCN) guidelines (8, 11). In addition, given the clonal evolution observed in AML, FLT3 mutations can be gained or lost at disease relapse and progression. In an individual patient, the presence of FLT3 mutations will often need to be re-assessed over time.
Currently, there remains considerable variability in FLT3 assay types, associated sensitivity and specificity, and turnaround time among treating centers (12). There are two main methods for determining FLT3 status are polymerase chain reaction (PCR)-based assays and next generation sequencing (NGS). Due to competition from the FLT3-WT allele, the sensitivity of standard PCR-based assays may be lower than NGS-based methods, although this can be overcome using patient-specific PCR primers (13).
The FLT3 allelic frequency (AF) has also been used to define FLT3-ITD positivity, primarily in research studies. Unlike the AR, which calculates the ratio of the area under the curve (AUC) of mutant to WT alleles (FLT3-ITD/FLT3-WT), the AF calculates the fraction of mutant alleles as a percentage of all FLT3 alleles (FLT3-ITD/FLT3-WT + FLT3-ITD). For example, a FLT3 AR of 0.5 (0.5 ITD/1.0 WT) would be equal to a FLT3 AR of 0.33 (0.5 ITD/0.5 ITD + 1.0 WT = 0.33 AF). NGS studies are typically reported as VAF while PCR assays typically report AR.
Historically, FLT3-ITD has been inherently difficult to detect using NGS. NGS relies on the reconstruction of short (<300 base pair) sequences, and longer length ITDs may not be detected (14, 15) although this can be overcome using novel bioinformatic approaches (16). NGS is becoming more commonly used, especially in monitoring for FLT3+ minimal residual disease (MRD) following treatment. An NGS-based FLT3 assay is now commercially available and is currently used in in an ongoing trial of gilteritinib maintenance therapy following hematopoietic cell transplantation (HCT) (NCT02997202).
Both PCR and NGS-based assays are supported by the current NCCN guidelines (8); however, ELN risk stratification is dependent upon FLT3 AR, which can only be determined by PCR. There is currently no standardized methodology or laboratory reference values for AR determination, and the current cut-off of ≥0.5 has not been prospectively validated. In retrospective analyses, higher AR is generally associated with inferior clinical outcomes (17), although these studies are prior to the widespread use of FLT3 inhibitors. It is likely that impact of AR on prognosis exists on a continuum, rather than a discrete cut-off. Finally, many patients do not receive FLT3 testing at all. In a large survey by the American College of Pathologists in 2015, only 51% of new AML referrals received FLT3 testing (18).
Overview of Currently Established FLT3 Inhibitors
Given the prevalence and poor prognosis of FLT3-ITD mutated AML, targeting FLT3 signaling through small molecule inhibitors is a promising therapeutic strategy. FLT3 inhibitors can be stratified using two primary schema: first vs second generation FLT3 inhibitors and type I vs type II FLT3 inhibitors. FLT3 inhibitors currently in use or in active development, including toxicity profiles, are detailed in Table 1.
Current usage and ongoing trials of established FLT3 inhibitors in newly diagnosed and relapsed/refractor (R/R) AML are summarized in Tables 2 and 3, respectively.
Stratification of FLT3 Inhibitors
First generation FLT3 inhibitors include sorafenib, midostaurin, lestaurtinib, sunitinib, and tandutinib. These multi-kinase inhibitors target not only FLT3 but other kinases as well, including PKC, SYK, FLK-1, AKT, PKA, KIT, FGR, SRC, PDGFRα/β, and VEGFR 1/2 (midostaurin) and RAF, VEGFR 1/2/3, PDGFRβ, KIT, and RET (sorafenib). The antileukemic effects of these non-specific inhibitors likely derive not only from FLT3 inhibition, but from inhibition of targets in these parallel pathways as well. Similarly, due to their multiple off-target effects, first generation FLT3 inhibitor may also be associated with increased toxicities. In contrast, second generation FLT3 inhibitors are more potent, FLT3-specific and thus have fewer off-target effects at clinically relevant doses. Second generation FLT3 inhibitors include gilteritinib, quizartinib, and crenolanib.
FLT3 inhibitors can also be subdivided based upon how they interact with the intracellular kinase domain (KD) of the FLT3 receptor. In normal physiology, FLT3 ligand binds to the extracellular domain, causing the FLT3 receptor to dimerize, assume an enzymatically active conformation and subsequently activate downstream signaling. Type I FLT3 inhibitors, which include midostaurin, gilteritinib, lestaurtinib, and crenolanib, bind the receptor in the active conformation, thus inhibiting both FLT3-TKD and FLT3-ITD mutated receptors. Type II inhibitors, including quizartinib and sorafenib, bind to a region adjacent to the ATP-binding pocket and only inhibit the receptor in the inactive conformation. Type II inhibitors are inactive against most FLT3-TKD mutations as these mutations bias the active kinase conformation of FLT3. This distinction is key in understanding mechanisms of FLT3 inhibitor resistance, as discussed later in this review.
First-Generation FLT3 Inhibitors
Midostaurin
Midostaurin was one of the first FLT3 inhibitors to be studied in AML. Early studies of single-agent midostaurin in R/R AML demonstrated the drug was well-tolerated, but had limited efficacy (19, 31).
Results of midostaurin in combination therapy have been more promising. In the phase III RATIFY trial, midostaurin was evaluated in combination with standard induction and consolidation chemotherapy in adults <60 years with FLT3-mutated AML. This combination demonstrated significant improvement in the primary endpoint of overall survival (OS), with a median OS of 74.7 months in patients receiving midostaurin plus chemotherapy vs 25.6 months in patients receiving placebo plus chemotherapy (p = 0.009) (20). In 2017, over two decades since FLT3 mutations were first described in AML, midostaurin gained US Food and Drug Administration (FDA) approval, becoming first agent to achieve FDA approval for AML since the year 2000.
While the results of RATIFY were promising, there are some important caveats to consider. In RATIFY, 23% of the study population had a FLT3-TKD mutation, significantly larger than that seen in the general population, and perhaps biasing the results toward this less-aggressive disease subtype. In addition, while patients in RATIFY were younger (median age 48), FDA approval was extended to all age groups (20). The phase II AMLSG 16-10 trial is currently evaluating midostaurin in combination with induction and consolidation chemotherapy in adults up to age 70 (NCT01477606), with initial results suggesting that older age does not significantly impact outcomes, despite the majority of patients requiring midostaurin dose reduction (32).
Sorafenib
Unlike RATIFY, trials of sorafenib in combination with chemotherapy have not been conducted in only FLT3-mutant patients and can thus be more challenging to interpret. Early phase I/II results of sorafenib in combination with induction chemotherapy demonstrated promising results, with a 75% complete response (CR) rate in all patients and a 93% CR rate in the subset with FLT3-ITD mutant AML (22). These findings, however, were not replicated in follow-up randomized studies. In 2013, sorafenib plus chemotherapy was evaluated in older adults (61–80 years), but did not show benefit in either the primary endpoint of EFS or in OS, including in FLT3-ITD subgroup analysis (median EFS 7 vs 5 months, p = 0.12). Furthermore, sorafenib demonstrated marked toxicity, presumably due to off-target effects (33).
In 2015, sorafenib plus chemotherapy was evaluated in adults <60 years in the randomized phase II SORAML trial. While sorafenib demonstrated improvement in the primary endpoint of EFS compared to placebo in all patients regardless of FLT3 status (median EFS 21 vs 9 months, p = 0.013) (23), there was ultimately no difference in OS (5y OS 61 vs 52%, p = 0.23) (34).
Sorafenib may have efficacy as a single agent. Early phase I and retrospective studies of sorafenib monotherapy in R/R AML irrespective of FLT3 status demonstrated acceptable toxicity profiles but mixed CR rates, ranging from 10 to 48% (35, 36). In studies of FLT3-ITD R/R AML, response rates of sorafenib monotherapy were 23–92% (37–39) with some post-transplant patients achieving sustained remission for multiple years (39, 40). Sorafenib does not have regulatory approval for AML but can be used off-label in the US as it is approved for hepatocellular, renal cell, and thyroid cancer.
Sunitinib, Lestaurtinib, Tandutinib
Other first-generation FLT3 inhibitors have demonstrated limited antileukemic effect as monotherapy and mixed results when combined with chemotherapy. As single-agent therapy for patients with R/R disease, sunitinib (41), lestaurtinib (42), and tandutinib (43) have all demonstrated limited and short-lived responses. In combination with chemotherapy, a phase I/II study of sunitinib plus frontline chemotherapy in adults >60 demonstrated a 59% CR rate; however, multiple patients experienced dose-limiting toxicities (44). To contrast, a randomized phase III trial of lestaurtinib plus frontline induction and consolidation chemotherapy in patients with FLT3-mutated AML demonstrated no difference in primary endpoints of OS (5-year OS 46% lestaurtinib vs 45% control, p = 0.3) or RFS (5-year RFS 40 vs 36%, p = 0.30) (45). Similarly, a randomized phase III trial of chemotherapy with or without lestaurtinib in patients with FLT3-mutated AML in first relapse demonstrated no difference in the primary endpoint of CR rates (26 vs 21%, p = 0.35) (42). Currently, none of these agents are approved for AML and development in AML has been abandoned.
Second-Generation FLT3 Inhibitors
Unlike midostaurin and sorafenib, second-generation FLT3 inhibitors are more specific to the FLT3 receptor, exhibit greater potency, and have thus been far more efficacious as single-agent therapy. Both gilteritinib and crenolanib are type I inhibitors, active against both the active and inactive conformation of the FLT3 receptor, while quizartinib is a type II inhibitor, active only against the inactive form.
Quizartinib
Quizartinib has demonstrated improved potency and selectivity against FLT3-ITD in preclinical cellular assays, and targets KIT and PDGFR as well as FLT3 (46). Early phase II studies of quizartinib monotherapy in R/R disease were highly promising, with CR rates of 46–56% and median OS of 25 weeks in FLT3-ITD mutated AML (25, 47). Quizartinib also demonstrated an acceptable safety profile aside from QTc prolongation, leading to an additional phase IIb study which explored dose reduction to 60 and 90 mg daily vs up to 200 mg daily (47).
Quizartinib was subsequently evaluated in the phase III randomized QuANTUM-R trial, which randomized patients with R/R FLT3-ITD AML to single-agent quizartinib vs salvage chemotherapy (26). Quizartinib was associated with a significantly longer primary endpoint of overall survival (6.2 vs 4.7 months, p = 0.02), and a greater proportion of patients were able to proceed to hematopoietic cell transplantation (HCT; 32 vs 11%). Total treatment-associated toxicities were similar between the two arms, although 2% of patients receiving quizartinib experienced Grade 3 QTc prolongation. Based on these results, the authors of QuANTUM-R concluded that quizartinib monotherapy should be considered a standard of care option in R/R FLT3-ITD mutated AML (26).
Despite these positive results, in 2019, both the FDA and the European Medicines Agency (EMA) rejected approval for quizartinib, although regulatory approval was granted in Japan. Although the FDA Oncologic Drug Advisory Committee (ODAC) raised concerns that up to four deaths in the quizartinib arm were attributed to cardiotoxicity, the decision to decline was ultimately due to concerns regarding trial design (48). These concerns included (1) an imbalance in patients who were randomized but not treated, with 23% of patients randomized to chemotherapy not receiving treatment vs 11% of patients randomized to quizartinib and (2) in stratified analysis, a significant survival benefit only when quizartinib was compared against low-intensity therapy (low-dose cytarabine) and not against high-intensity therapy (MEC or FLAG-Ida). The ODAC concluded that, while a modest survival benefit of 6 weeks was still meaningful, especially if more patients were bridged to HCT, ultimately additional data would be needed for quizartinib to be approved in this setting (48). The phase II Q-HAM trial, which has not yet begun recruiting, will evaluate quizartinib in combination with salvage chemotherapy in R/R FLT3-ITD AML (NCT03989713).
In newly diagnosed AML, quizartinib is currently being evaluated in the randomized phase III QuANTUM-First trial, which will compare quizartinib vs placebo in combination with induction and consolidation chemotherapy (NCT02668653). Additional phase I/II studies evaluating quizartinib in combination with frontline cytotoxic chemotherapy are ongoing as well (NCT03723681, NCT03135054, NCT04047641).
Gilteritinib
Gilteritinib is a selective and potent type I FLT3 inhibitor which also has activity against AXL, ALT, and ALK (49). Gilteritinib is a particularly promising agent due to its ability to target KD mutations, including the D835 residue, the development of which is a key mechanism of both quizartinib (50) and sorafenib (51) resistance. In the phase I/II CHRYSALIS trial, gilteritinib demonstrated promising results as monotherapy in R/R FLT3-mutant AML with an overall response rate (ORR) of 40% and a median OS of 25 weeks (52). Notably, patients with FLT3-D835 mutations also responded to gilteritinib, albeit at lower rates than patients with FLT3-ITD mutations, with an ORR of 55% in FLT-ITD-mutated patients, 17% in FLT3-D835-mutated patients, and 62% in patients with both FLT3-ITD and FLT-D835 mutations (52).
Following these results, the randomized phase III ADMIRAL trial compared single-agent gilteritinib vs salvage chemotherapy in R/R FLT3-mutated AML (27) with co-primary endpoints of CR rate and OS. Compared to standard salvage chemotherapy, gilteritinib demonstrated significantly greater CR rate (34 vs 15%, p = 0.0001) and improvement in OS (9.3 vs 5.6 months, p < 0.001). While prior use of midostaurin or sorafenib was allowed, as the trial enrolled prior to midostaurin’s approval and widespread use, the majority (88%) of patients had not received a prior FLT3 inhibitor, limiting the ability of this trial to evaluate the ability of gilteritinib to overcome midostaurin resistance; however, results were similar in patients with FLT3-ITD and FLT3-TKD mutated disease (27). Based on a pre-planned interim analysis, in 2018, the FDA approved gilteritinib as single-agent therapy for R/R FLT3-mutated AML.
It is unknown whether gilteritinib is similarly beneficial in newly diagnosed AML. Gilteritinib is currently being studied in a phase I study in combination with induction and consolidation chemotherapy in newly diagnosed AML (NCT02236013); interim results indicate this approach is safe and feasible (53). The phase III HOVON 156 trial, which is actively accruing, will compare gilteritinib vs midostaurin in combination with chemotherapy followed by FLT3 inhibitor maintenance (NCT04027309).
Crenolanib
Crenolanib is a potent type I inhibitor with activity against PDGFRβ, FLT3-ITD, and FLT3-TKD mutations, including at D835 (54). Two smaller phase II studies have demonstrated efficacy of single-agent crenolanib in R/R FLT3-mutated AML, with a CR rate of 23−39% in patients naïve to FLT3 inhibitors, and 5% patients with prior FLT3 exposure (29, 30).
Crenolanib also demonstrates promising results in combination with chemotherapy. A phase II trial of crenolanib plus chemotherapy in newly diagnosed FLT3-mutated AML demonstrated a high CR rate of 85%; notably, 70% of patients remained alive and disease free with a median follow-up of 29.3 months (55). Crenolanib plus chemotherapy is also efficacious in older adults. In a phase II trial of adults 61–75 years with newly diagnosed FLT3-mutated AML, crenolanib plus chemotherapy demonstrated a CR rate of 86% and was relatively well-tolerated, although four of 14 patients did require dose-reductions due to toxicity (56). A phase III trial of crenolanib vs midostaurin plus chemotherapy in newly diagnosed FLT3-mutated AML is currently recruiting (NCT03258931).
Resistance to FLT3 Inhibitors
Despite the relative success of established FLT3 inhibitors, responses are frequently short-lived and therapeutic resistance poses an ongoing challenge. Mechanisms of FLT3 inhibitor resistance differ based on drug type, but broadly can be subdivided into cell intrinsic and extrinsic mechanisms. Intrinsic resistance can be further sub-divided into on-target secondary mutations within FLT3 and off-target mutations in downstream or parallel signaling pathways. These mutations may develop de novo or via expansion of pre-existing subclones (57). Extrinsic resistance can occur through altered expression or metabolism of the FLT3 ligand or changes in the interactions between the leukemic blast and the bone marrow microenvironment. Figure 1 illustrates common resistance pathways and mechanisms.
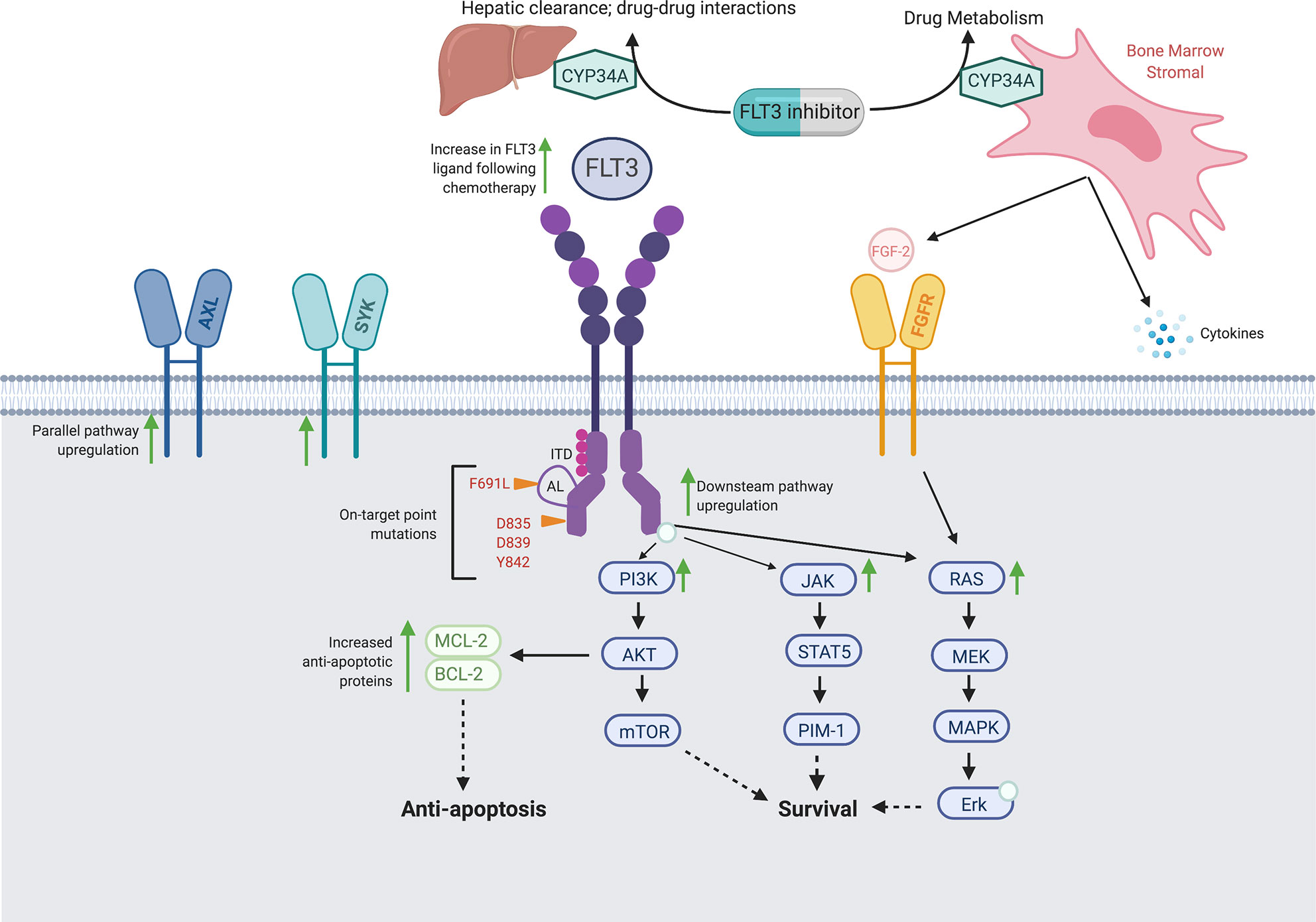
Figure 1 Proposed Intrinsic and Extrinsic Mechanisms of FLT3 Inhibitor Resistance. Schematic of FLT3 inhibitor resistance mechanisms, including on-target secondary FLT3 mutations, off-target mutations in parallel and/or downstream signaling pathways, and extrinsic alterations in drug metabolism and the bone marrow microenvironment.
On-Target Secondary Mutations
One common mechanism of FLT3 inhibitor resistance is development of a secondary FLT3 mutation, most commonly in the KD (58). These mutations commonly occur at gatekeep F691 and activation loop (AL) D835 residues, but can involve other KD residues I836, D839, and Y842, among others (59). This mechanism is most relevant for type II FLT3 inhibitors, which interact weakly with the active receptor formation caused by some KD mutations. Type I inhibitors gilteritinib and crenolanib are both active against D835 mutations (60, 61).
To contrast, the gatekeeper F691L mutation confers resistance not only to quizartinib and sorafenib, but also to gilteritinib and crenolanib as well (51, 60–62). In practice, the impact of F691L mutations on type I inhibitor resistance may be relatively minor. In studies of both single-agent gilteritinib (62, 63) and single-agent crenolanib (64) resistance in patients with R/R FLT3-mutated AML, only 12% of patients receiving gilteritinib, and 11% of patients receiving crenolanib developed F691L mutations at the time of resistance.
In most cases, on-target mutations are not detected prior to FLT3 inhibitor treatment (65). In a recent study of 11 patients treated with quizartinib monotherapy, single-cell sequencing revealed 7/11 patients developed KD mutations at relapse and no patients had these mutations prior to FLT3 inhibition, suggesting TK mutations typically evolve de novo (66) or exist at levels undetectable by current sequencing methods.
Off-Target Resistance in Parallel or Downstream Pathways
KD mutations only partly explain FLT3 inhibitor resistance, and expansion or emergence of non-FLT3 mutant clones is a key resistance mechanism. In patients treated with gilteritinib and crenolanib, sequencing of paired patient samples pre- and post-therapy demonstrated a wide variety of mutations at resistance, including genes involved in the RAS pathways (NRAS, KRAS, PTPN11), ASXL1, TP53, TET2, and IDH1/2. These mutations occurred not only in cells expressing the FLT3-mutant allele, but in FLT3-WT cells as well (63, 64). These off-target mutations are not limited to type I inhibitors. In a study using single-cell sequencing to analyze 11 patients treated with quizartinib, 3/11 demonstrated off-target mutations at relapse, all of which were present in small clonal populations prior to FLT3 inhibitor therapy (66).
Of these off-target pathways, activation of the downstream Ras and associated PI3K/Akt/mTOR and MAPK/Erk pathways are particularly common in clinical gilteritinib and crenolanib resistance (62–64), and in vitro studies have demonstrated mutations in these pathways can confer resistance to FLT3 inhibitors in FLT3-mutant cell lines (67, 68). Development of the BCR-ABL1 fusion gene has been describe in patients with gilteritinib resistance (63, 69).
Upregulation of parallel AXL tyrosine kinase signaling is another mechanism of FLT3 inhibitor resistance. In one study, blasts from a patient with FLT3-ITD AML were exposed to both midostaurin and quizartinib and were found to have increased AXL phosphorylation upon development of FLT3 inhibitor resistance. This resistance could be overcome with use of the AXL inhibitor TP-0903 (70, 71) and a phase I trial of TP-0903 with or without azacitidine in FLT3-mutated AML has recently opened (NCT04518345).
Together, these studies suggest off-target resistance mechanisms are common to all FLT3 inhibitors and frequently arise via selection of pre-existing sub-clones harboring survival advantages under the selective pressure of FLT3 inhibition.
Extrinsic Mechanisms of Resistance
The majority of leukemic blasts, regardless of mutational status, express the FLT3 receptor and proliferate in response to FLT3 ligand. Increased levels of FLT3 ligand in the bone marrow microenvironment have been demonstrated during induction and consolidation chemotherapy, and can lead to increased signaling via the native FLT3 receptor, even in the presence of FLT3 inhibitors (72, 73). FLT3 inhibitors may also have decreased efficacy due to decreased drug availability, either due to enhanced CYP34A expression on BM stromal cells (74) or iatrogenic drug-drug interactions (75).
The bone marrow microenvironment can also directly contribute to FLT3 inhibitor resistance. In addition to directly secreting FLT3 ligand, bone marrow stromal cells can also upregulate Ras/MAPK signaling independent of the FLT3 receptor via secretion of FGF2 (76). In one study of patients treated with quizartinib, stromal cell expression of FGF2 conferred FLT3 inhibitor resistance, and could be overcame by FGFR inhibition (76, 77). Multiple dual FLT3/FGFR inhibitors are in pre-clinical development (78, 79) with MAX-40279 currently in phase I clinical trials (NCT03412292, NCT04187495). In addition, ponatinib, a multikinase inhibitor approved in CML, has activity against both FLT3 and FGFR (80).
Novel FLT3 Inhibitor Combinations
One strategy to overcome resistance and provide durable remissions is to use FLT3 inhibitors in novel combinations with other antileukemic agents (Tables 4, 5; Figure 2).
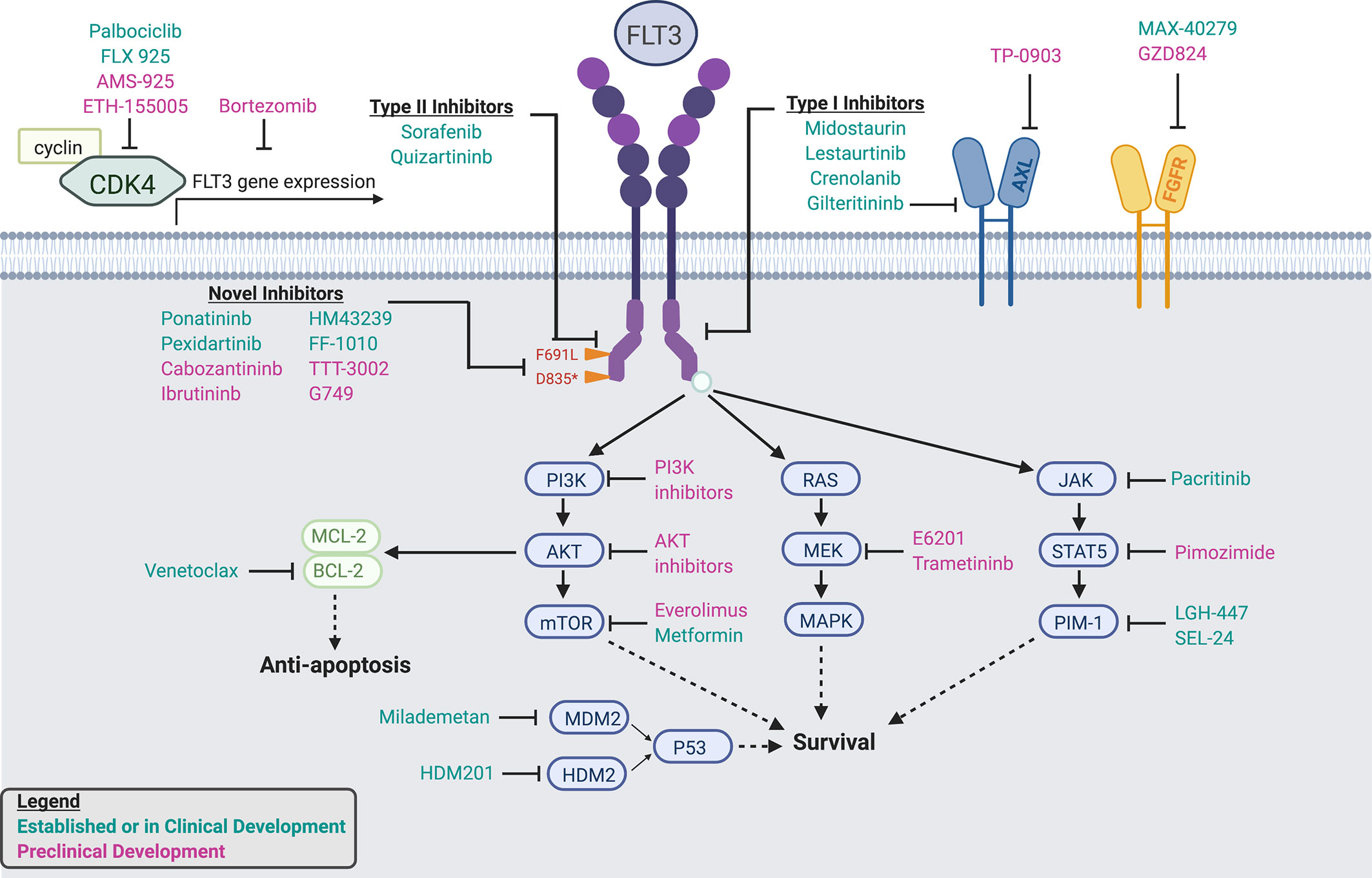
Figure 2 Established and In-Development FLT3 inhibitors, dual inhibitors, and combination agents. Schematic detailing mechanisms of action of established and in-development FLT3 inhibitors, dual and multikinase inhibitors, and combination agents.
Hypomethylating Agents
Aside from conventional cytotoxic chemotherapy, the most well-studied FLT3 inhibitor combination is with the hypomethylating agents (HMA) azacitidine or decitabine. This combination is particularly attractive, both due to the synergistic cytotoxicity in preclinical studies as well as the established tolerability and durable responses of HMAs in older adults (81, 82).
Multiple phase I/II trials have demonstrated the combination of midostaurin and HMA to be feasible in adults with FLT-mutated AML who are unfit for traditional chemotherapy in the frontline setting (83) and in R/R disease (82, 84). Additional trials of midostaurin plus HMA for older/unfit adults have opened, but have terminated due to inability to accrue (NCT01846624. NCT02634827). There is an ongoing trial of midostaurin plus azacitidine for newly diagnosed AML regardless of FLT3 mutational status (NCT01093573), with primary endpoints of tolerability and ORR.
Sorafenib plus HMA have also been shown to be safe and efficacious in single-arm and retrospective studies in both the R/R (85, 86) and frontline settings (87). In the frontline setting, sorafenib plus HMA demonstrated an ORR of 78%, the study’s primary endpoint, and a and median response duration of 14.5 (87). Similarly, a phase I/II study of sorfenib plus azacitidine demonstrated an ORR of 46%, the secondary endpoint for the trial’s phase II portion, and median response duration of 2.3 months (85). Notably, FLT3 ligand levels remained low with this combination, which is intriguing as increase in ligand expression has been suggested as a possible mechanism of FLT3 inhibitor resistance (72, 85).
Of the second generation FLT3 inhibitors, an interim analysis of a phase I/II trial of quizartinib plus azacitidine in unfit patients with newly diagnosed or in R/R FLT3-ITD AML demonstrated an ORR of 75%, the secondary endpoint for the trial’s phase II portion, including in four/five patients with prior FLT3 inhibitor exposure (88). A phase II study of gilteritinib plus azacitidine in unfit patients with newly diagnosed FLT3-ITD AML with primary endpoint of OS is currently accruing (NCT02752035) with interim results of secondary endpoints demonstrating CR and ORR rates of 67 and 80%, respectively (89).
Venetoclax
Venetoclax, an inhibitor of the anti-apoptotic protein Bcl-2, is particularly intriguing in combination with FLT3 inhibitors. Preclinical studies have shown FLT3-ITD mutated blasts have higher Bcl-2 expression compared to FLT3-WT blasts (90) and upregulation of antiapoptotic proteins is a mechanism of FLT3 inhibitor resistance (91). In preclinical mouse models, venetoclax has demonstrated synergistic antileukemic activity with midostaurin (92), gilteritinib (92), and quizartinib (93). In addition, FLT3-ITD mutations were frequently observed at progression in trials of venetoclax monotherapy in AML (94, 95).
Gilteritinib is currently being studied in combination with venetoclax in R/R AML (NCT03625505). Quizartinib is being evaluated in combination with venetoclax and decitabine in poor-risk newly diagnosed or R/R FLT3- mutated AML (NCT03661307) and in combination with venetoclax alone in R/R FLT3-ITD AML (NCT03735875).
Proteosome Inhibitors
Proteosome inhibitors, including bortezomib, have demonstrated cytotoxicity toward FLT3-ITD mutant cells in preclinical studies (96) and a phase I study of midostaurin in combination with bortezomib and chemotherapy in R/R AML demonstrated activity, albeit with marked toxicity (97). Sorafenib plus bortezomib was also studied in a combination with vorinostat, a histone deacetylase inhibitor, in a phase I/II trial of R/R AML and demonstrated a modest ORR of 28% in all patients and 60% in patients with FLT3-ITD AML (98). Ongoing studies of this combination include a phase III trial of sorafenib plus bortezomib in younger adults (up to age 29) with newly diagnosed FLT3-ITD AML (NCT01371981) and sorafenib plus bortezomib followed by decitabine in newly diagnosed or R/R AML regardless of FLT3 status (NCT01861314).
Additional Targeted Agents
Multiple agents targeting signaling pathways downstream of FLT3 signaling, including those frequently implicated in FLT3 inhibitor resistance, have been studied in combination therapies.
JAK/STAT5/Pim-1 is a key signaling pathway parallel and downstream of FLT3. Pim kinase inhibitors inhibit Pim-1, a kinase which promotes FLT3 signaling via positive feedback (99, 100). In preclinical models, Pim kinase and FLT3 inhibitors demonstrate synergistic cytotoxicity (100, 101). The Pim kinase inhibitor LGH447 is being studied in combination with midostaurin in a phase I trial (NCT02078609), as is the novel dual Pim kinase/FLT3 inhibitor SEL24 (NCT03008187). Other agents, including the dual JAK/FLT3 inhibitor pacritinib, have demonstrated efficacy in phase I trials as well (102).
mTOR inhibitors, such as everolimus, target the PI3K/AKT/mTOR pathways, which is similarly downstream of FLT3 signaling. Concomitant inhibition of both mTOR and FLT3 demonstrate synergistic cytotoxicity (103) and mTOR is upregulated in AML blasts resistant to FLT3 inhibitors (68). A phase I study of midostaurin plus everolimus is active, but not currently recruiting (NCT00819546). In addition, metformin, a drug long approved in diabetes, can also down-regulate the P31K/Akt/mTOR pathways, and has shown to act synergistically with sorafenib in FLT3-mutated cell lines (104).
Cyclin-dependent kinase 6 (CKD6) is a key regulator of cell cycle progression, part of a transcriptional complex that promotes leukemogenesis, and is found at the promoter of both FLT3 and PIM1 genes (105, 106). A phase I study of the CDK4/6 inhibitor palbociclib, which is approved in breast cancer, in combination with sorafenib is actively recruiting (NCT03132454). In addition, multiple novel dual FLT3/CDK4 inhibitors are in active preclinical development, including AMS-925 (107) (Keegan), ETH-155008 (108), and FLX925 (109), which recently completed a phase I dose-escalation trial (NCT02335814).
Finally, the tumor suppressor p53 is increasingly recognized as a mechanism of FLT3 inhibitor resistance, particularly to crenolanib (64). Milademetan, an inhibitor of the p53-regulatory protein MDM2, has demonstrated synergistic anti-leukemic activity with quizartinib in FLT3-mutant cell lines (110) and a phase I trial is actively recruiting (NCT03552029). Similarly, HDM-201, an inhibitor of the p53-regulatory protein HDM2, is actively investigated in combination with midostaurin (NCT04496999).
Immunotherapy Combinations
Compared to lymphoid and many solid malignancies, AML has thus far demonstrated limited response to immunotherapies. Exploratory studies have indicated that elevated programmed cell death 1 (PD-1) and PD-1 ligand (PD-L1) are associated with inferior OS in AML, including in patients with FLT3-mutated disease (111). A phase I/II trial of gilteritinib in combination with the checkpoint inhibitor atezolizumab in R/R FLT-mutated AML is ongoing (NCT03730012).
Novel FLT3 Inhibitors in Development
In addition to novel FLT3 inhibitor combinations, multiple novel FLT3 inhibitors are in active preclinical and clinical development (Table 5; Figure 2). These include multikinase inhibitors approved for non-AML malignancies as well as specific, next-generation agents. In addition to overcoming pre-established mechanisms of resistance, including F691 mutations, these novel agents also offer alternative and potentially more desirable toxicity profiles.
Multikinase Inhibitors
Ponatinib is approved to target BCR-ABL in chronic myelogenous leukemia (CML), but is also a type II FLT3 inhibitor with activity against F691L (112). Ponatinib demonstrated modest efficacy in a phase I trial of heavily-pretreated R/R AML patients (113), and is being actively investigated in combination with chemotherapy (NCT02428543).
Cabozantinib is a multikinase inhibitor currently approved for medullary thyroid and renal cell carcinomas. Cabozantinib is selectively cytotoxic to FLT3-ITD mutant cells in culture (114) and a phase I trial demonstrated sustained inhibition of FLT3-ITD and -F691 mutant cells, although no treated patients had a formal disease response (115).
The multikinase inhibitor pexidartinib (PLX3397) has been studied in multiple solid tumors and also demonstrates activity against FLT3, including F691L (50). Recently, a phase I/II trial of single-agent pexidartinib in R/R FLT3-ITD AML demonstrated an ORR of 21% and CR rate of 11%; furthermore, several patients were successfully bridged to transplant (116).
Finally, the BTK inhibitor ibrutinib, currently approved for lymphoid malignancies, also demonstrates type II FLT3 inhibitory effects (117). A phase I trial of CG-806, a dual BTK/FLT3 inhibitor, recently opened for patients with R/R AML (NCT04477291).
Novel FLT3 Inhibitors
One of the most promising novel agents is FF-10101, the first covalently-binding FLT3 inhibitor. In pre-clinical studies, FF-10101 demonstrated potent activity against quizartinib-resistant AL and gatekeeper F691 mutations (118). Other agents in development include TTT-3002, G-749, MZH-29, and HM43239, all highly selective FLT3 inhibitors with activity against D835 and F691 residues (119–122). In preclinical studies, these agents have demonstrated activity against AML blasts resistant to sorafenib and quizartinib (119) or midostaurin and quizartinib (120), and may represent options for refractory disease. Phase I/II trials of both FF-10101 and HM43239 are ongoing (NCT03194685, NCT03850574).
Biologic Agents
To date, pharmacologic targeting of FLT3-mutant AML has primarily focused on signaling inhibition via small molecules; however, multiple immunotherapeutic approaches are in development.
Ongoing trials are investigating targeting FLT3 through an IgG1 antibody (FLYSYN; NCT02789254), with promising preliminary efficacy data (123), as well as via an anti-FLT3 antibody drug conjugate (124) (NCT02864290). In addition, an anti-FLT3/anti-CD3 bi-specific antibody has shown promise in preclinical models as well (125). Finally, FLT3 may represent a potential target for chimeric antigen receptor T cell (CART) therapy (126), including in combination with established FLT3 inhibitors (127). A phase I trial of a FLT3-directed CART has recently opened (NCT03904069).
Ongoing Questions and Controversies
Despite the considerable advances in treating FLT3-mutant AML, many outstanding questions and controversies remain. We have summarized a few of the most intriguing and timely questions below.
What is the Benefit of Maintenance Therapy in FLT3-Mutant AML?
Of all the questions regarding the clinical use of FLT3 inhibitors, one of the most pressing is the role of maintenance therapy. Use of FLT3 inhibitor maintenance, either during remission for patients who do not undergo HCT or during post-HCT remission, is currently not standard of care; however, there are data to suggest this may be a promising approach. Table 6 describes ongoing clinical trials of maintenance therapy.
Post-Chemotherapy Maintenance
Perhaps most notably, in the experimental arm of the phase III RATIFY study, patients could receive up to one year of midostaurin maintenance following induction and consolidation chemotherapy plus midostaurin (20). In unplanned post-hoc analysis of the subset of patients who received either midostaurin or placebo maintenance, there was no benefit seen with midostaurin, although it was well-tolerated (128). Importantly, the median duration of midostaurin exposure was 3 months, as the majority (59%) of patients randomized to midostaurin received allogeneic HCT and thus received only two to three cycles of therapy, limiting the ability to draw conclusions from midostaurin maintenance data (20). Maintenance midostaurin after chemotherapy did not receive US FDA approval; however, regulatory approval for maintenance midostaurin was granted by the EMA.
Currently, both the single-arm AMLSG 16–10 trials, which will also evaluate midostaurin in combination with chemotherapy in older adults, and the phase III HOVON 156 AML trial, which will compare midostaurin vs gilteritinib plus chemotherapy in newly diagnosed AML, will each evaluate up to one year of midostaurin maintenance following chemotherapy (NCT01477606, NCT04027309). Of note, neither of these trials will directly compare maintenance midostaurin against placebo, which will make it difficult to isolate the true benefit of post-chemotherapy midostaurin maintenance.
Multiple ongoing trials are also evaluating second generation FLT3 inhibitors as post-chemotherapy maintenance. In the HOVON 156 AML trial, patients may receive up to one year of gilteritinib maintenance, although again, this study will not compare gilteritinib against a placebo control. A separate randomized phase III trial will compare gilteritinib vs placebo maintenance for up to two years following induction and consolidation therapy in patients who are not proceeding to HCT accrual (NCT02927262). Similarly, the QuANTUM-First trial will include up to three years of post-chemotherapy quizartinib maintenance and will be placebo-controlled.
Post-HCT Maintenance
In the post-HCT setting, midostaurin was evaluated in a phase II trial as single-agent maintenance following midostaurin plus chemotherapy and subsequent HCT. Of the 56% of patients who ultimately received HCT and subsequent maintenance, midostaurin maintenance was associated with improved OS compared to a historical control group; however, given this comparison was relative to historic controls it must be interpreted with caution (129). Midostaurin maintenance was also associated with significant toxicities, particularly in older adults.
In the phase II RADIUS trial, patients were randomized to receive up to one year of maintenance midostaurin vs standard care following HCT. Although preliminary reports suggested that addition of midostaurin could reduce risk of relapse at 18 months post-HCT by 46%, 63% of patients receiving midostaurin required dose modifications and 25% discontinued midostaurin due to toxicity. RADIUS ultimately had inadequate enrollment to detect a statistically significant difference in 18-month relapse-free survival (RFS), the study’s primary endpoint (estimated 18-month RFS 89% in midostaurin arm vs 76% in standard-of-care arm, p = 0.27) (130).
Similarly, post-HCT sorafenib maintenance was shown to be tolerable and potentially efficacious in both retrospective and prospective phase I/II studies, although frequent dose adjustments were needed (131, 132). More recently, the phase II SORMAIN trial randomized patients with FLT3-ITD AML in remission following HCT to maintenance with two years of sorafenib vs placebo (133). The HR for RFS, the primary endpoint, demonstrated a significant benefit with sorafenib vs placebo (HR 0.39, 95% CI 0.18–0.85, p = 0.013); however, only 9/43 (21%) of patients receiving sorafenib had received a frontline FLT3 inhibitor, so it remains unclear whether the same benefit would be seen in patients who received a FLT3 inhibitor in the frontline setting. Similar to the RADIUS trial, study drug discontinuation was more common in the sorafenib arm (22 vs 5%), although this difference was not significant. Finally, and again similar to the RADIUS trail, SORMAIN did not reach is target accrual and was terminated prematurely.
Building on the results of RADIUS and SORMAIN, there the multiple ongoing trails evaluating post-HCT maintenance therapy. The phase II, single-arm MAURITUS trial will evaluate midostaurin maintenance following HCT in MRD-positive FLT3-mutated AML (NCT03951961) and a phase III trial with compare post-HCT midostaurin vs crenolanib maintenance (NCT03258931).
For the second generation FLT3 inhibitors, BMT CTN 1506, a randomized phase III trial of gilteritinib vs placebo for FLT3-ITD mutated AML following HCT is ongoing (NCT02997202). Importantly, although prior FLT3 inhibitor treatment is not an inclusion criterion, since enrollment occurred after the widespread use of midostaurin in the frontline setting, the majority of patients enrolled on BMT CTN 1506 will likely have received prior FLT3 inhibitor therapy, answering a key question raised by SORMAIN. In addition, quizartinib was shown to be well-tolerated as single-agent maintenance following HCT in a phase I study (134) and this strategy will be further explored in the QuANTUM-First trial. Finally, a non-randomized trial is evaluating the efficacy of crenolanib post-HCT in FLT3-mutated AML (NCT02400255).
Maintenance Therapy: Where Do We Go From Here?
The role of FLT3 inhibitor maintenance, while promising, remains unknown, and placebo-controlled randomized trials are necessary to establish the efficacy of this approach. Multiple questions remain, including: How can we identify the patients for which FLT3 inhibitor maintenance is most beneficial? In SORMAIN, the strongest benefit from sorafenib maintenance was in patients with undetectable MRD pre-HCT and detectable MRD post-HCT (133). Notably, the ongoing BMT 1506 trial will include correlative MRD analysis to determine if the presence of MRD is predictive of benefit from FLT3 inhibitor maintenance. What is the optimal duration of maintenance therapy? In a correlative analysis of RADIUS, decreased levels of phosphorylated FLT3 were associated with improved OS (135), suggesting this may be one potential biomarker for determining maintenance duration. What type of FLT3 inhibitor is most efficacious as a maintenance agent, a multikinase, first-generation agent, or a more targeted, second generation one? In the post-chemotherapy setting, does maintenance serve as a bridge to transplant, or obviate the need? Finally, what is the effect of maintenance therapy on health-associated quality of life? Given that this patient population may not have active disease, studies specifically investigating health-associated quality of life are needed to fully inform the benefit of prolonged maintenance therapy.
What Is the Role of Transplant in FLT3-Mutant AML?
Historically, FLT3-mutated AML has been considered adverse risk disease, and patients with FLT3-ITD have been recommended to undergo HCT in the first CR (136, 137). More recently, the ELN has re-classified FLT3-mutated AML such that patients with FLT3-ITDlow (AR < 0.5), normal cytogenetics, and mutated NPM1 are considered low risk, suggesting these patients may have good prognosis without HCT (138). This is not widely accepted, however, and retrospective data have demonstrated that ‘low risk’ FLT3-ITD AML, with FLT3-IT low AR and positive NPM1 mutational status, still conveys poor prognosis, with a 5-year OS of 41.3%, and OS is improved by HCT (139). A similar retrospective study found that HCT in first CR improves OS in all FLT3-ITD AML, regardless of AR or NPM1 status (140). Importantly, both these studies and the ELN guidelines were written prior to widespread FLT3 inhibitor use, and the role of HCT in FLT3-mutated AML today remains an open question.
In a retrospective analysis of the RATIFY trial, the beneficial effect of midostaurin was seen across all ELN subgroups; however, the benefit of HCT was only seen in patients with adverse risk disease (138). This should be interpreted with caution as RATIFY was not powered for these subgroup analyses. Nonetheless, this study provides support that in low or even intermediate risk FLT3-ITD AML, HCT could potentially be delayed until first relapse or MRD positivity. Of note, in RATIFY, patients that did not receive HCT did receive post-consolidation midostaurin maintenance, an indication that was not approved as discussed above.
More recently, in a phase II study of crenolanib plus chemotherapy followed by crenolanib maintenance in newly diagnosed FLT3-mutated AML, 85% of patients achieved CR. Of the 27 patients on trial, 7/27 received consolidation but not HCT; of those, 6/7 remained in long-term remission. OS was similar between patients who underwent HCT vs those who did not (55). While the number of patients is small and the ELN risk category not specified, this again raises the question of whether HCT is needed in all patients with FLT3-mutated AML.
In a recent position statement by the European Society for Blood and Marrow Transplantation (EBMT), HCT in first CR was recommended for patients with intermediate or adverse risk FLT3-ITD AML and could be considered in low risk disease. Furthermore, in the absence of GVHD, post-HCT FLT3 inhibitor maintenance was recommended, ideally on a clinical trial (141). Randomized trials are needed to further clarify these algorithms. Multiple trials of FLT3 inhibitors plus chemotherapy in newly diagnosed AML are actively accruing, and it will be interesting to see the role of HCT in these studies.
Given New FLT3-Directed Therapies, Will We Need to Re-Think Risk Stratification?
The current ELN criteria were developed before FLT3 inhibitors were routinely used. Prior to widespread FLT3 inhibitor use, the prognosis for FLT3-ITD AML was dismal. Historically, while patients with FLT3-ITD responded to induction chemotherapy with similar remission rates as other AML subtypes, patients were more likely to relapse and had inferior OS (7, 142).
In a retrospective analysis of the RATIFY trial, for all enrolled patients, OS differed significantly among ELN risk groups. In all risk groups, midostaurin significantly improved OS, with 5-year OS probabilities for the midostaurin arm of 0.53 and 0.52 for intermediate- and adverse-risk, respectively (138). More recently crenolanib plus chemotherapy in newly diagnosed AML has demonstrated a 3-year OS of 0.76 in adults ≤60 (55) and a 1-year OS of 0.67 in adults 61–75 (56). As a comparison, in at retrospective validation of ELN risk stratification in newly-diagnosed patients receiving conventional chemotherapy, 5-year OS probabilities were 0.36 for FLT3-ITDlow/NPM1WT and 0.29 for FLT3-ITDhigh/NPM1mutated (intermediate risk) and 0.09 for FLT3-ITDhigh/NPM-WT (adverse risk) (143). While historic comparisons must be interpreted with caution, this may suggest FLT3 inhibitors have shifted the risk associated with FLT3-mutated AML.
Given the multiple FLT3-directed therapies both approved and in development, the prognosis of FLT3-mutated AML may be changing. Will FLT3 mutations in AML eventually be analogous to HER2 amplification in breast cancer or BCR-ABL1 fusions in acute lymphoblastic leukemia? In both of these cases, the development of targeted therapies has dramatically improved the prognosis of a previously poor-risk subtype, and a similar pattern may evolve with FLT3 as well.
What Is the Prognostic and Therapeutic Impact of Non-Canonical FLT3 Mutations?
As sequencing technology improves, FLT3 mutations outside of the ITD and D835 regions have been described. These non-canonical (NC) mutations are frequently in exon 14 of the juxtamembrane (JM) domain, where ITDs occur, or in the KD domain adjacent to D835; however NC mutations in other sites, including the extracellular (EC) domain, have been described as well. Select NC mutations identified in the clinical literature are summarized in Table 7.
In the pediatric literature, whole genome sequencing of 799 pediatric AML patients revealed a 7.6% prevalence of NC FLT3 point mutations and insertions-deletions compared to a 23% total prevalence of all FLT3 mutations (including FLT-ITD and D835). This included 9 JM mutations with activating potential (E598D, E573D/G, L576R, T582N, D586Y, Y589H, E596K/G, Y599C, D600G), many which occurred as co-mutations with FLT3-ITD (145). Similarly, in a large study of 1,540 adult AML patients, targeting genetic sequencing identified four NC FLT3 driver mutation, including two EC (S451F and V491L) and two JM (V592A, E598D) mutations (4).
It is unclear to whether NC mutations confer FLT3 inhibitor susceptibility, resistance, or are simply passenger mutations unimportant to disease biology. In one study of 222 AML patients without FLT3-ITD or -D835 mutations, four NC driver mutations were identified which had variable sensitivity to midostaurin (144). Similarly, in a study of 18 patients treated with crenolanib, pre- and post-treatment sequencing identified small populations of four NC mutations at baseline which were eliminated over the course of treatment (A833S, D839Y/G, N841K, Y842C) (64). In a recent in vitro study, gilteritinib was active against multiple NC mutations, including mutations like N676, which are associated with resistance to midostaurin and quizartinib (149). Finally, in a recent case series, two patients with non-FLT3-ITD or D835 AML were found to have JM FLT3-V592G and KD FLT3-N676K mutations, both of which clinically responded to sorafenib (146).
To contrast, maintenance or development of NC mutations have also been observed in conjunction with FLT3 inhibitor resistance. In patients treated with crenolanib, four NC FLT3 mutations (D200N, K429E, Y572C, L601F) were maintained at time of relapse (64). In an analysis of 40 patients with FLT3-mutated AML treatment with gilteritinib on the ADMIRAL trial, six patients had new FLT3 mutations at time of relapse, five of which were in gatekeeper F691L and two at the NC site JM E598D (62).
Many questions remain regarding the nature of NC mutations, including their true prevalence (as they are not detected outside of whole-exome or genome sequencing), prognostic impact, and role in the FLT3 inhibitor susceptibility and resistance.
Is There a Role for FLT3 Inhibitors in FLT3-WT Disease?
Although only one-quarter of AML patients harbor FLT3 mutations, the FLT3 receptor is over-expressed on leukemic blast regardless of mutational status (2), and early studies of FLT3 inhibitors observed blast reduction in patients with FLT3-WT disease (19).
Early trials of FLT3 inhibitors were not limited to patients with FLT3-mutated AML, and results from these studies may indicate benefit in targeting FLT3-WT. In the RATIFY trial, using an arbitrary AR cut-off of ≥0.7, post-hoc analysis noted a similar OS benefit withFLT3-ITDlow, FLT3-ITDhigh, and FLT3-TKD AML (20). Similarly, in the SORAML trial, EFS benefit and trend toward OS benefit were demonstrated irrespective of FLT3 mutation status (23, 34).
Given that both midostaurin and sorafenib are multikinase inhibitors, it is possible these results are due to inhibition of alternative kinase-dependent pathways. For example, both sorafenib and midostaurin also inhibit KIT, and KIT mutations are seen in 30–46% of core binding factor (CBF) AML and may impact prognosis (150, 151). The use of midostaurin in CBF AML is currently being explored in a phase II study (NCT03686345), and a trial of midostaurin in c-KIT or FLT3-ITD mutated t(8,21) AML recently completed accrual (NCT01830361).
Efficacy in FLT3-WT disease has also been demonstrated in second generation FLT3 inhibitors, which are more specific to the FLT3 receptor. While the initial phase I/II study of gilteritinib demonstrated strongest antileukemic effect in patients with FLT3-mutated disease, a 12% ORR was seen in FLT3-WT AML (52). Similarly, quizartinib monotherapy demonstrated 54 and 32% ORR in patients with FLT3-ITD and FLT3-WT disease, respectively (152).
Trials of FLT3 inhibitors in FLT3-WT AML are ongoing, including a randomized phase III trial of chemotherapy +/− midostaurin (NCT03512197) and chemotherapy +/− quizartinib (NCT04107727), both in patients with newly-diagnosed FLT3-WT AML. In addition, many phase I trials of novel combination therapies, dual FLT3 inhibitors, and biologic agents are not restricted to FLT3-mutant disease.
How Should We Approach Tumor Heterogeneity in FLT3-Mutant AML?
Increasingly, AML is understood as a heterogenous disease with multiple genetically distinct subclones, dynamically evolving under pressure of therapy. Clonal evolution is particularly relevant in FLT3-mutated AML as FLT3 mutations can be gained with disease progression, and development of a new FLT3-ITD is an independent negative prognostic factor (153).
Development of FLT3-mutated clones can arise under targeted therapy. In patients with R/R AML treated with venetoclax, analysis of pre- and post-treatment samples demonstrated 4/20 patients developed new FLT3-ITD clones following therapy (94). In a larger study of 81 patients treated with frontline venetoclax-based combinations, 5/25 patients showed increased FLT3-ITD clonal burden at relapse, two of which had newly acquired FLT3-ITD clones (95). Similarly, in patients with IDH1-mutated AML treated with ivosidenib, bulk NGS at time of progression identified multiple patients with new FLT3-ITD or -TKD mutations not present at therapy initiation (154), and in patients with IDH2-mutated AML treated with enasidenib, analysis of paired pre- and post-treatment samples described several cases with increased FLT3 variant allele frequency at relapse (155).
Complex clonal evolution has also been observed following FLT3 inhibition. In an analysis of patients treated with single agent gilteritinib on the phase III ADMIRAL or phase II CHRYSALIS trial, targeted NGS identified emerging clones with mutations activating RAS/MAPK signaling, including NRAS and KRAS. Serial single-cell sequencing confirmed early selection for RAS-mutant subclones under gilteritinib pressure (63). In an analysis of paired pre- and post-treatment samples of patients treated with quizartinib, NRAS development was similarly noted at relapse; furthermore, single cell sequencing confirmed these distinct subclones existed in small populations prior to therapy and expanded under FLT3 inhibition (66).
It is unclear how to best address clonal evolution and associated adaptive resistance in AML. Is the treatment of multiple clones best approached through blunt approaches, such as cytotoxic chemotherapy or broad, triple-therapy combinations, such as HMA/Venetoclax/Gilteritinib? Alternatively, would it be more beneficial to target individual subclones sequentially, perhaps focusing on the highest-risk or fastest-growing subclones first? As genomic technologies such as single cell sequencing become more widely adopted, available genomic information will increase dramatically. Looking forward, innovative bioinformatic and machine learning-based approaches may be employed to rationally treat the complex clonal architecture in FLT3-mutated AML.
Treating FLT3-Mutated AML Today
The current standard of care for an adult with newly diagnosed FLT3-mutated AML with AR ≥0.05 who is eligible for treatment is induction and consolidation chemotherapy in combination with midostaurin. As FLT3 AR is not universally reported, if not available the presence of a FLT3-ITD or TKD mutation should warrant treatment with a FLT3 inhibitor. This is generally followed by HCT, as the largest survival benefit in the RATIFY trial was observed in patients who underwent HCT in first remission (20), although whether this approach holds in patients with low-AR FLT3-ITD, FLT3-TKD, or concomitant NPM1 mutations is debatable.
While not standard of care, use of single-agent maintenance FLT3 inhibitors, either in remission for patients not going to HCT or in post-HCT remission can be considered. This would ideally be done in a clinical trial setting, although off-label use of sorafenib or midostaurin maintenance is routinely practiced in the US (156, 157). We await the results of ongoing clinical trials to help determine in which settings FLT3 inhibitor maintenance is most useful.
Should a patient with FLT3-ITD AML relapse or a patient with FLT3-WT AML develop a new FLT3-mutated clone, then single-agent gilteritinib is standard salvage therapy world-wide and single-agent quizartinib could be considered in certain practice locations. If response if achieved, HCT would be recommended in all fit patients. If a patient remains refractory or develops relapse, then multiple clinical trials could be considered, including FLT3 inhibitor combinations, novel FLT3 inhibitors, or FLT3-directed biologics (Figure 3). Although both gilteritinib and venetoclax are FDA-approved, and there is promising pre-clinical data on this combination (92), this regimen can be associated with marked myelosuppression and we would not recommend it outside of a clinical trial. Similarly, the triplet combination of HMA/Gilteritinib/Venetoclax should only be used as part of clinical trial until the toxicities associated with this novel combination are better understood.
Finally, special consideration should be given to older adults, who may be intolerant of intensive induction chemotherapy as well as the side effects associated with multikinase inhibitors. As described above, multiple studies are actively investigating FLT3 inhibitors in combination with lower-intensity therapies currently approved as frontline therapy for older adults, including HMAs as well as liposomal cytarabine and anthracycline (CPX-351, Vyxeos).
Concluding Remarks
While this provides a baseline treatment paradigm, survival remains poor in FLT3-mutated AML, and additional treatment options are needed. This includes increasing the diversity of approved FLT3, investigating FLT3 inhibitors in new combinations and treatment settings, and development of novel agents. Given the diversity in FLT3 inhibitor potency, resistance patterns, and toxicity profiles, ideally the oncologist will have a range of FLT3-directed therapies to choose from, similar to TKI selection in chronic myelogenous leukemia, so that a particular FLT3 inhibitor could be matched to an individual patient’s needs.
Author Contributions
VK and CS both contributed to the writing of this manuscript. All authors contributed to the article and approved the submitted version.
Conflict of Interest
The authors declare that the research was conducted in the absence of any commercial or financial relationships that could be construed as a potential conflict of interest.
References
1. Dohner H, Weisdorf DJ, Bloomfield CD. Acute Myeloid Leukemia. N Engl J Med (2015) 373(12):1136–52. doi: 10.1056/NEJMra1406184
2. Carow CE, Levenstein M, Kaufmann SH, Chen J, Amin S, Rockwell P, et al. Expression of the hematopoietic growth factor receptor FLT3 (STK-1/Flk2) in human leukemias. Blood (1996) 87(3):1089–96. doi: 10.1182/blood.V87.3.1089.bloodjournal8731089
3. Gilliland DG, Griffin JD. The roles of FLT3 in hematopoiesis and leukemia. Blood (2002) 100(5):1532–42. doi: 10.1182/blood-2002-02-0492
4. Papaemmanuil E, Gerstung M, Bullinger L, Gaidzik VI, Paschka P, Roberts ND, et al. Genomic Classification and Prognosis in Acute Myeloid Leukemia. N Engl J Med (2016) 374(23):2209–21. doi: 10.1056/NEJMoa1516192
5. Bolouri H, Farrar JE, Triche T Jr., Ries RE, Lim EL, Alonzo TA, et al. The molecular landscape of pediatric acute myeloid leukemia reveals recurrent structural alterations and age-specific mutational interactions. Nat Med (2018) 24(1):103–12. doi: 10.1038/nm.4439
6. Schnittger S, Bacher U, Haferlach C, Alpermann T, Kern W, Haferlach T. Diversity of the juxtamembrane and TKD1 mutations (exons 13-15) in the FLT3 gene with regards to mutant load, sequence, length, localization, and correlation with biological data. Genes Chromosomes Cancer (2012) 51(10):910–24. doi: 10.1002/gcc.21975
7. Yanada M, Matsuo K, Suzuki T, Kiyoi H, Naoe T. Prognostic significance of FLT3 internal tandem duplication and tyrosine kinase domain mutations for acute myeloid leukemia: a meta-analysis. Leukemia (2005) 19(8):1345–9. doi: 10.1038/sj.leu.2403838
8. Dohner H, Estey E, Grimwade D, Amadori S, Appelbaum FR, Buchner T, et al. Diagnosis and management of AML in adults: 2017 ELN recommendations from an international expert panel. Blood (2017) 129(4):424–47. doi: 10.1182/blood-2016-08-733196
9. Mead AJ, Linch DC, Hills RK, Wheatley K, Burnett AK, Gale RE. FLT3 tyrosine kinase domain mutations are biologically distinct from and have a significantly more favorable prognosis than FLT3 internal tandem duplications in patients with acute myeloid leukemia. Blood (2007) 110(4):1262–70. doi: 10.1182/blood-2006-04-015826
10. Bacher U, Haferlach C, Kern W, Haferlach T, Schnittger S. Prognostic relevance of FLT3-TKD mutations in AML: the combination matters–an analysis of 3082 patients. Blood (2008) 111(5):2527–37. doi: 10.1182/blood-2007-05-091215
11. Tallman MS, Wang ES, Altman JK, Appelbaum FR, Bhatt VR, Bixby D, et al. Acute Myeloid Leukemia, Version 3.2019, NCCN Clinical Practice Guidelines in Oncology. J Natl Compr Canc Netw (2019) 17(6):721–49. doi: 10.6004/jnccn.2019.0028
12. Daver N, Schlenk RF, Russell NH, Levis MJ. Targeting FLT3 mutations in AML: review of current knowledge and evidence. Leukemia (2019) 33(2):299–312. doi: 10.1038/s41375-018-0357-9
13. Levis MJ, Perl AE, Altman JK, Gocke CD, Bahceci E, Hill J, et al. A next-generation sequencing-based assay for minimal residual disease assessment in AML patients with FLT3-ITD mutations. Blood Adv (2018) 2(8):825–31. doi: 10.1182/bloodadvances.2018015925
14. Spencer DH, Abel HJ, Lockwood CM, Payton JE, Szankasi P, Kelley TW, et al. Detection of FLT3 internal tandem duplication in targeted, short-read-length, next-generation sequencing data. J Mol Diagn (2013) 15(1):81–93. doi: 10.1016/j.jmoldx.2012.08.001
15. Bolli N, Manes N, McKerrell T, Chi J, Park N, Gundem G, et al. Characterization of gene mutations and copy number changes in acute myeloid leukemia using a rapid target enrichment protocol. Haematologica (2015) 100(2):214–22. doi: 10.3324/haematol.2014.113381
16. Au CH, Wa A, Ho DN, Chan TL, Ma ES. Clinical evaluation of panel testing by next-generation sequencing (NGS) for gene mutations in myeloid neoplasms. Diagn Pathol (2016) 11:11. doi: 10.1186/s13000-016-0456-8
17. Schlenk RF, Kayser S, Bullinger L, Kobbe G, Casper J, Ringhoffer M, et al. Differential impact of allelic ratio and insertion site in FLT3-ITD-positive AML with respect to allogeneic transplantation. Blood (2014) 124(23):3441–9. doi: 10.1182/blood-2014-05-578070
18. George TI, Tworek JA, Thomas NE, Fatheree LA, Souers RJ, Nakhleh RE, et al. Evaluation of Testing of Acute Leukemia Samples: Survey Result From the College of American Pathologists. Arch Pathol Lab Med (2017) 141(8):1101–6. doi: 10.5858/arpa.2016-0398-CP
19. Fischer T, Stone RM, Deangelo DJ, Galinsky I, Estey E, Lanza C, et al. Phase IIB trial of oral Midostaurin (PKC412), the FMS-like tyrosine kinase 3 receptor (FLT3) and multi-targeted kinase inhibitor, in patients with acute myeloid leukemia and high-risk myelodysplastic syndrome with either wild-type or mutated FLT3. J Clin Oncol (2010) 28(28):4339–45. doi: 10.1200/JCO.2010.28.9678
20. Stone RM, Mandrekar SJ, Sanford BL, Laumann K, Geyer S, Bloomfield CD, et al. Midostaurin plus Chemotherapy for Acute Myeloid Leukemia with a FLT3 Mutation. N Engl J Med (2017) 377(5):454–64. doi: 10.1056/NEJMoa1614359
21. Novartis Pharmaceuticals Corporation. RYDAPT® product monograph. East Hanover, New Jersey (2018).
22. Ravandi F, Cortes JE, Jones D, Faderl S, Garcia-Manero G, Konopleva MY, et al. Phase I/II study of combination therapy with sorafenib, idarubicin, and cytarabine in younger patients with acute myeloid leukemia. J Clin Oncol (2010) 28(11):1856–62. doi: 10.1200/JCO.2009.25.4888
23. Rollig C, Serve H, Huttmann A, Noppeney R, Muller-Tidow C, Krug U, et al. Addition of sorafenib versus placebo to standard therapy in patients aged 60 years or younger with newly diagnosed acute myeloid leukaemia (SORAML): a multicentre, phase 2, randomised controlled trial. Lancet Oncol (2015) 16(16):1691–9. doi: 10.1016/S1470-2045(15)00362-9
25. Cortes J, Perl AE, Dohner H, Kantarjian H, Martinelli G, Kovacsovics T, et al. Quizartinib, an FLT3 inhibitor, as monotherapy in patients with relapsed or refractory acute myeloid leukaemia: an open-label, multicentre, single-arm, phase 2 trial. Lancet Oncol (2018) 19(7):889–903. doi: 10.1016/S1470-2045(18)30240-7
26. Cortes JE, Khaled S, Martinelli G, Perl AE, Ganguly S, Russell N, et al. Quizartinib versus salvage chemotherapy in relapsed or refractory FLT3-ITD acute myeloid leukaemia (QuANTUM-R): a multicentre, randomised, controlled, open-label, phase 3 trial. Lancet Oncol (2019) 20(7):984–97. doi: 10.1016/S1470-2045(19)30150-0
27. Perl AE, Martinelli G, Cortes JE, Neubauer A, Berman E, Paolini S, et al. Gilteritinib or Chemotherapy for Relapsed or Refractory FLT3-Mutated AML. N Engl J Med (2019) 381(18):1728–40. doi: 10.1056/NEJMoa1902688
29. Cortes JE, Kantarzian HM, Kadia TM, Borthakur G, Konopleva M, Garcia-Manero G, et al. Crenolanib besylate, a type I pan-FLT3 inhibitor, to demonstrate clinical activity in multiply relapsed FLT3-ITD and D835 AML. J Clin Oncol (2016) 34(15_suppl):7008–7008. doi: 10.1200/JCO.2016.34.15_suppl.7008
30. Randhawa JK KH, Borthakur G, Thompson PA, Konopleva M, Daver N, et al. Results of a Phase II Study of Crenolanib in Relapsed/Refractory Acute Myeloid Leukemia Patients (Pts) with Activating FLT3 Mutations. Blood (2014) 124(21):389. doi: 10.1182/blood.V124.21.389.389
31. Stone RM, DeAngelo DJ, Klimek V, Galinsky I, Estey E, Nimer SD, et al. Patients with acute myeloid leukemia and an activating mutation in FLT3 respond to a small-molecule FLT3 tyrosine kinase inhibitor, PKC412. Blood (2005) 105(1):54–60. doi: 10.1182/blood-2004-03-0891
32. Schlenk RF, Fiedler W, Salih HR, Wulf G, Thol F, Kundgen A, et al. Impact of age and midostaurin-dose on response and outcome in acute myeloid leukemia with FLT3-ITD: interim analyses of the AMLSG 16-10 Trial. Blood (2016) 128:449. doi: 10.1182/blood.V128.22.449.449
33. Serve H, Krug U, Wagner R, Sauerland MC, Heinecke A, Brunnberg U, et al. Sorafenib in combination with intensive chemotherapy in elderly patients with acute myeloid leukemia: results from a randomized, placebo-controlled trial. J Clin Oncol (2013) 31(25):3110–8. doi: 10.1200/JCO.2012.46.4990
34. Rollig C, Serve H, Huttmann A, Noppeney R, Muller-Tidow C, Krug U, et al. The addition of sorafenib to standard AML treatment results in substantial reduction in relapse risks and improved survival. Updated results from long-term follow-up of the randomized-controlled SORAML trial. Blood (2017) 130:721–1. doi: 10.1182/blood.V130.Suppl_1.721.721
35. Crump M, Hedley D, Kamel-Reid S, Leber B, Wells R, Brandwein J, et al. A randomized phase I clinical and biologic study of two schedules of sorafenib in patients with myelodysplastic syndrome or acute myeloid leukemia: a NCIC (National Cancer Institute of Canada) Clinical Trials Group Study. Leuk Lymphoma (2010) 51(2):252–60. doi: 10.3109/10428190903585286
36. Borthakur G, Kantarjian H, Ravandi F, Zhang W, Konopleva M, Wright JJ, et al. Phase I study of sorafenib in patients with refractory or relapsed acute leukemias. Haematologica (2011) 96(1):62–8. doi: 10.3324/haematol.2010.030452
37. Metzelder SK, Schroeder T, Finck A, Scholl S, Fey M, Gotze K, et al. High activity of sorafenib in FLT3-ITD-positive acute myeloid leukemia synergizes with allo-immune effects to induce sustained responses. Leukemia (2012) 26(11):2353–9. doi: 10.1038/leu.2012.105
38. De Freitas T, Marktel S, Piemontese S, Carrabba MG, Tresoldi C, Messina C, et al. High rate of hematological responses to sorafenib in FLT3-ITD acute myeloid leukemia relapsed after allogeneic hematopoietic stem cell transplantation. Eur J Haematol (2016) 96(6):629–36. doi: 10.1111/ejh.12647
39. Tschan-Plessl A, Halter JP, Heim D, Medinger M, Passweg JR, Gerull S. Synergistic effect of sorafenib and cGvHD in patients with high-risk FLT3-ITD+AML allows long-term disease control after allogeneic transplantation. Ann Hematol (2015) 94(11):1899–905. doi: 10.1007/s00277-015-2461-5
40. Metzelder SK, Schroeder T, Lubbert M, Ditschkowski M, Gotze K, Scholl S, et al. Long-term survival of sorafenib-treated FLT3-ITD-positive acute myeloid leukaemia patients relapsing after allogeneic stem cell transplantation. Eur J Cancer (2017) 86:233–9. doi: 10.1016/j.ejca.2017.09.016
41. Fiedler W, Serve H, Dohner H, Schwittay M, Ottmann OG, O’Farrell AM, et al. A phase 1 study of SU11248 in the treatment of patients with refractory or resistant acute myeloid leukemia (AML) or not amenable to conventional therapy for the disease. Blood (2005) 105(3):986–93. doi: 10.1182/blood-2004-05-1846
42. Levis M, Ravandi F, Wang ES, Baer MR, Perl A, Coutre S, et al. Results from a randomized trial of salvage chemotherapy followed by lestaurtinib for patients with FLT3 mutant AML in first relapse. Blood (2011) 117(12):3294–301. doi: 10.1182/blood-2010-08-301796
43. DeAngelo DJ, Stone RM, Heaney ML, Nimer SD, Paquette RL, Klisovic RB, et al. Phase 1 clinical results with tandutinib (MLN518), a novel FLT3 antagonist, in patients with acute myelogenous leukemia or high-risk myelodysplastic syndrome: safety, pharmacokinetics, and pharmacodynamics. Blood (2006) 108(12):3674–81. doi: 10.1182/blood-2006-02-005702
44. Fiedler W, Kayser S, Kebenko M, Janning M, Krauter J, Schittenhelm M, et al. A phase I/II study of sunitinib and intensive chemotherapy in patients over 60 years of age with acute myeloid leukaemia and activating FLT3 mutations. Br J Haematol (2015) 169(5):694–700. doi: 10.1111/bjh.13353
45. Knapper S, Russell N, Gilkes A, Hills RK, Gale RE, Cavenagh JD, et al. A randomized assessment of adding the kinase inhibitor lestaurtinib to first-line chemotherapy for FLT3-mutated AML. Blood (2017) 129(9):1143–54. doi: 10.1182/blood-2016-07-730648
46. Zarrinkar PP, Gunawardane RN, Cramer MD, Gardner MF, Brigham D, Belli B, et al. AC220 is a uniquely potent and selective inhibitor of FLT3 for the treatment of acute myeloid leukemia (AML). Blood (2009) 114(14):2984–92. doi: 10.1182/blood-2009-05-222034
47. Cortes JE, Tallman MS, Schiller GJ, Trone D, Gammon G, Goldberg SL, et al. Phase 2b study of 2 dosing regimens of quizartinib monotherapy in FLT3-ITD-mutated, relapsed or refractory AML. Blood (2018) 132(6):598–607. doi: 10.1182/blood-2018-01-821629
48. 21266 N. Quizartininb. (2019). FDA Briefing Document of the Oncologic Drugs Advisory Committee (ODAC).
49. Ueno Y, Kaneko N, Saito R, Kondoh Y, Shimada I, Mori M, et al. ASP2215, a novel FLT3/AXL inhibitor: Preclinical evaluation in combination with cytarabine and anthracycline in acute myeloid leukemia (AML). J Clin Oncol (2014) 32(15_suppl):7071–7071. doi: 10.1200/jco.2014.32.15_suppl.7071
50. Smith CC, Zhang C, Lin KC, Lasater EA, Zhang Y, Massi E, et al. Characterizing and Overriding the Structural Mechanism of the Quizartinib-Resistant FLT3 “Gatekeeper” F691L Mutation with PLX3397. Cancer Discov (2015) 5(6):668–79. doi: 10.1158/2159-8290.CD-15-0060
51. Baker SD, Zimmerman EI, Wang YD, Orwick S, Zatechka DS, Buaboonnam J, et al. Emergence of polyclonal FLT3 tyrosine kinase domain mutations during sequential therapy with sorafenib and sunitinib in FLT3-ITD-positive acute myeloid leukemia. Clin Cancer Res (2013) 19(20):5758–68. doi: 10.1158/1078-0432.CCR-13-1323
52. Perl AE, Altman JK, Cortes J, Smith C, Litzow M, Baer MR, et al. Selective inhibition of FLT3 by gilteritinib in relapsed or refractory acute myeloid leukaemia: a multicentre, first-in-human, open-label, phase 1-2 study. Lancet Oncol (2017) 18(8):1061–75. doi: 10.1016/S1470-2045(17)30416-3
53. Pratz KCM, Altman JK, Cooper B, Cruz JC, Juric JG, Levis MJ, et al. Updated Results from a Phase 1 Study of Gilteritininb in Combination with Induction and Consolidation Chemotherapy in Subjects with Newly Diagnosed Acute Myeloid Leukemia (AML). Blood (2018) 132(Supplement 1):564. doi: 10.1182/blood-2018-99-110975
54. Galanis A, Ma H, Rajkhowa T, Ramachandran A, Small D, Cortes J, et al. Crenolanib is a potent inhibitor of FLT3 with activity against resistance-conferring point mutants. Blood (2014) 123(1):94–100. doi: 10.1182/blood-2013-10-529313
55. Goldberg AD, Coombs CC, Wang ES, Walter RB, Karanes C, Vigil CE, et al. Younger Patients with Newly Diagnosed FLT3-Mutant AML Treated with Crenolanib Plus Chemotherapy Achieve Adequate Free Crenolanib Levels and Durable Remissions. Blood (2019) 134(Supplement_1):1326–6. doi: 10.1182/blood-2019-130863
56. Wang ES, Griffiths EA, Walter RB, Tallman MS, Goldberg AD, Messahel B, et al. Tolerability and Efficacy of Crenolanib and Cytarabine/Anthracycline Chemotherapy in Older Patients (Aged 61 to 75) with Newly Diagnosed FLT3-Mutated Acute Myeloid Leukemia (AML). Blood (2019) 134(Supplement_1):3829–9. doi: 10.1182/blood-2019-130536
57. Leung AY, Man CH, Kwong YL. FLT3 inhibition: a moving and evolving target in acute myeloid leukaemia. Leukemia (2013) 27(2):260–8. doi: 10.1038/leu.2012.195
58. Daver N, Cortes J, Ravandi F, Patel KP, Burger JA, Konopleva M, et al. Secondary mutations as mediators of resistance to targeted therapy in leukemia. Blood (2015) 125(21):3236–45. doi: 10.1182/blood-2014-10-605808
59. Cools J, Mentens N, Furet P, Fabbro D, Clark JJ, Griffin JD, et al. Prediction of resistance to small molecule FLT3 inhibitors: implications for molecularly targeted therapy of acute leukemia. Cancer Res (2004) 64(18):6385–9. doi: 10.1158/0008-5472.CAN-04-2148
60. Lee LY, Hernandez D, Rajkhowa T, Smith SC, Raman JR, Nguyen B, et al. Preclinical studies of gilteritinib, a next-generation FLT3 inhibitor. Blood (2017) 129(2):257–60. doi: 10.1182/blood-2016-10-745133
61. Smith CC, Lasater EA, Lin KC, Wang Q, McCreery MQ, Stewart WK, et al. Crenolanib is a selective type I pan-FLT3 inhibitor. Proc Natl Acad Sci U S A (2014) 111(14):5319–24. doi: 10.1073/pnas.1320661111
62. Smith CC, Levis MJ, Perl AE, Martinelli G, Neubauer A, Berman E, et al. Emerging Mutations at Relapse in Patients with FLT3-Mutated Relapsed/Refractory Acute Myeloid Leukemia Who Received Gilteritinib Therapy in the Phase 3 Admiral Trial. Blood (2019) 134(Supplement_1):14–4. doi: 10.1182/blood-2019-122620
63. McMahon CM, Ferng T, Canaani J, Wang ES, Morrissette JJD, Eastburn DJ, et al. Clonal Selection with RAS Pathway Activation Mediates Secondary Clinical Resistance to Selective FLT3 Inhibition in Acute Myeloid Leukemia. Cancer Discov (2019) 9(8):1050–63. doi: 10.1158/2159-8290.CD-18-1453
64. Zhang H, Savage S, Schultz AR, Bottomly D, White L, Segerdell E, et al. Clinical resistance to crenolanib in acute myeloid leukemia due to diverse molecular mechanisms. Nat Commun (2019) 10(1):244. doi: 10.1038/s41467-018-08263-x
65. Smith CC, Wang Q, Chin CS, Salerno S, Damon LE, Levis MJ, et al. Validation of ITD mutations in FLT3 as a therapeutic target in human acute myeloid leukaemia. Nature (2012) 485(7397):260–3. doi: 10.1038/nature11016
66. Cohler Peretz CA ML, Kumar TF, Jackson LH, Jacob J, Durruthy R, et al. Single Cell Sequencing Reveals Evolution of Tumor Heterogeneity of Acute Myeloid Leukemia on Quizartinib. Blood (2019) 134(Supplement_1):1440. doi: 10.1182/blood-2019-132105
67. Piloto O, Wright M, Brown P, Kim KT, Levis M, Small D. Prolonged exposure to FLT3 inhibitors leads to resistance via activation of parallel signaling pathways. Blood (2007) 109(4):1643–52. doi: 10.1182/blood-2006-05-023804
68. Lindblad O, Cordero E, Puissant A, Macaulay L, Ramos A, Kabir NN, et al. Aberrant activation of the PI3K/mTOR pathway promotes resistance to sorafenib in AML. Oncogene (2016) 35(39):5119–31. doi: 10.1038/onc.2016.41
69. Kasi PM, Litzow MR, Patnaik MM, Hashmi SK, Gangat N. Clonal evolution of AML on novel FMS-like tyrosine kinase-3 (FLT3) inhibitor therapy with evolving actionable targets. Leuk Res Rep (2016) 5:7–10. doi: 10.1016/j.lrr.2016.01.002
70. Park IK, Mundy-Bosse B, Whitman SP, Zhang X, Warner SL, Bearss DJ, et al. Receptor tyrosine kinase Axl is required for resistance of leukemic cells to FLT3-targeted therapy in acute myeloid leukemia. Leukemia (2015) 29(12):2382–9. doi: 10.1038/leu.2015.147
71. Jeon JY, Garrison D, Thomas MZ, Buelow D, Whatcott C, Warner S, et al. Activity of TP-0903 in FLT3 inhibitor resistant AML models. FASEB J (2019) 33(1_supplement):675.4-675.4. doi: 10.1096/fasebj.2019.33.1_supplement.675.4
72. Sato T, Yang X, Knapper S, White P, Smith BD, Galkin S, et al. FLT3 ligand impedes the efficacy of FLT3 inhibitors in vitro and in vivo. Blood (2011) 117(12):3286–93. doi: 10.1182/blood-2010-01-266742
73. Yang X, Sexauer A, Levis M. Bone marrow stroma-mediated resistance to FLT3 inhibitors in FLT3-ITD AML is mediated by persistent activation of extracellular regulated kinase. Br J Haematol (2014) 164(1):61–72. doi: 10.1111/bjh.12599
74. Chang YT HD, Ghiaur G, Levis MJ, Jones RJ. Bone Marrow Stroma Protects FLT3 Acute Myeloid Leukemia (AML) through CYP3A4-Mediated Drug Metabolization of FLT3 Tyrosine Kinase Inhibitors (TKIs). Blood (2017) 130(Supplement 1):2519. doi: 10.1182/blood.V130.Suppl_1.2519.2519
75. Dutreix C, Munarini F, Lorenzo S, Roesel J, Wang Y. Investigation into CYP3A4-mediated drug-drug interactions on midostaurin in healthy volunteers. Cancer Chemother Pharmacol (2013) 72(6):1223–34. doi: 10.1007/s00280-013-2287-6
76. Traer E, Martinez J, Javidi-Sharifi N, Agarwal A, Dunlap J, English I, et al. FGF2 from Marrow Microenvironment Promotes Resistance to FLT3 Inhibitors in Acute Myeloid Leukemia. Cancer Res (2016) 76(22):6471–82. doi: 10.1158/0008-5472.CAN-15-3569
77. Javidi-Sharifi N, Martinez J, English I, Joshi SK, Scopim-Ribeiro R, Viola SK, et al. FGF2-FGFR1 signaling regulates release of Leukemia-Protective exosomes from bone marrow stromal cells. Elife (2019) 8:8. doi: 10.7554/eLife.40033
78. Wang Y BH, Zhang N, Wang F, He Y, Wu T. Preclinical Evaluation of MAX-40279, a FLT3/FGFR Dual Kinase Inhibitor for Treatment of Acute Myeloid Leukemia. BLood (2018) 132(Supplement 1):3997. doi: 10.1182/blood-2018-99-110414
79. Wang Y, Zhang L, Tang X, Luo J, Tu Z, Jiang K, et al. GZD824 as a FLT3, FGFR1 and PDGFRalpha Inhibitor Against Leukemia In Vitro and In Vivo. Transl Oncol (2020) 13(4):100766. doi: 10.1016/j.tranon.2020.100766
80. Gozgit JM, Wong MJ, Wardwell S, Tyner JW, Loriaux MM, Mohemmad QK, et al. Potent activity of ponatinib (AP24534) in models of FLT3-driven acute myeloid leukemia and other hematologic malignancies. Mol Cancer Ther (2011) 10(6):1028–35. doi: 10.1158/1535-7163.MCT-10-1044
81. Chang E, Ganguly S, Rajkhowa T, Gocke CD, Levis M, Konig H. The combination of FLT3 and DNA methyltransferase inhibition is synergistically cytotoxic to FLT3/ITD acute myeloid leukemia cells. Leukemia (2016) 30(5):1025–32. doi: 10.1038/leu.2015.346
82. Williams CB, Kambhampati S, Fiskus W, Wick J, Dutreix C, Ganguly S, et al. Preclinical and phase I results of decitabine in combination with midostaurin (PKC412) for newly diagnosed elderly or relapsed/refractory adult patients with acute myeloid leukemia. Pharmacotherapy (2013) 33(12):1341–52. doi: 10.1002/phar.1316
83. Tomlinson BK, Gallogly MM, Kane DM, Metheny L, Lazarus HM, William BM, et al. A Phase II Study of Midostaurin and 5-Azacitidine for Untreated Elderly and Unfit Patients With FLT3 Wild-type Acute Myelogenous Leukemia. Clin Lymphoma Myeloma Leuk (2020) 20(4):226–33.e1. doi: 10.1016/j.clml.2019.10.018
84. Strati P, Kantarjian H, Ravandi F, Nazha A, Borthakur G, Daver N, et al. Phase I/II trial of the combination of midostaurin (PKC412) and 5-azacytidine for patients with acute myeloid leukemia and myelodysplastic syndrome. Am J Hematol (2015) 90(4):276–81. doi: 10.1002/ajh.23924
85. Ravandi F, Alattar ML, Grunwald MR, Rudek MA, Rajkhowa T, Richie MA, et al. Phase 2 study of azacytidine plus sorafenib in patients with acute myeloid leukemia and FLT-3 internal tandem duplication mutation. Blood (2013) 121(23):4655–62. doi: 10.1182/blood-2013-01-480228
86. Muppidi MR GE, Thompson JE, Ford LA, Freyer CW, Wetzler M, Wang ES. Decitabine and Sorafenib Therapy in Patients with FLT3-ITD Mutant Acute Myeloid Leukemia Is Associated with High Response Rates—a Single Institute Experience. Blood (2014) (12421):5284. doi: 10.1182/blood.V124.21.5284.5284
87. Ohanian M, Garcia-Manero G, Levis M, Jabbour E, Daver N, Borthakur G, et al. Sorafenib Combined with 5-azacytidine in Older Patients with Untreated FLT3-ITD Mutated Acute Myeloid Leukemia. Am J Hematol (2018) 93(9):1136–41. doi: 10.1002/ajh.25198
88. Swaminathan M, Kantarjian H, Daver N, Borthakur G, Ohanian M, Kadia T, et al. The Combination of Quizartinib with Azacitidine or Low Dose Cytarabine Is Highly Active in Patients (Pts) with FLT3-ITD Mutated Myeloid Leukemias: Interim Report of a Phase I/II Trial. Blood (2017) 130(Supplement 1):723. doi: 10.1182/blood.V130.Suppl_1.723.723
89. Esteve J SR, Del Castillo TB, Lee J, Wang E, Dinner S, Minden M, et al. Open-label study of gilteritinib, gilteritinib plus azacitidine, or azacitidine alone in newly diagnosed FLT3-mutated AML patients ineligible for intensive chemotherapy: results from the safety cohort. In: European Hematology Association Annual Meeting. EHA Library (2019). 266682; PS1065. doi: 10.1097/01.HS9.0000562556.40615.f8
90. Bagrintseva K, Geisenhof S, Kern R, Eichenlaub S, Reindl C, Ellwart JW, et al. FLT3-ITD-TKD dual mutants associated with AML confer resistance to FLT3 PTK inhibitors and cytotoxic agents by overexpression of Bcl-x(L). Blood (2005) 105(9):3679–85. doi: 10.1182/blood-2004-06-2459
91. Kohl TM, Hellinger C, Ahmed F, Buske C, Hiddemann W, Bohlander SK, et al. BH3 mimetic ABT-737 neutralizes resistance to FLT3 inhibitor treatment mediated by FLT3-independent expression of BCL2 in primary AML blasts. Leukemia (2007) 21(8):1763–72. doi: 10.1038/sj.leu.2404776
92. Ma J, Zhao S, Qiao X, Knight T, Edwards H, Polin L, et al. Inhibition of Bcl-2 Synergistically Enhances the Antileukemic Activity of Midostaurin and Gilteritinib in Preclinical Models of FLT3-Mutated Acute Myeloid Leukemia. Clin Cancer Res (2019) 25(22):6815–26. doi: 10.1158/1078-0432.CCR-19-0832
93. Mali RS, Zhang Q, DeFilippis R, Cavazos A, Kuruvilla VM, Raman J, et al. Venetoclax combines synergistically with FLT3 inhibition to effectively target leukemic cells in FLT3-ITD+ acute myeloid leukemia models. Haematologica (2020) haematol.2019.244020. doi: 10.3324/haematol.2019.244020
94. Chyla B, Daver N, Doyle K, McKeegan E, Huang X, Ruvolo V, et al. Genetic Biomarkers Of Sensitivity and Resistance to Venetoclax Monotherapy in Patients With Relapsed Acute Myeloid Leukemia. Am J Hematol (2018) 93(8):E202–5. doi: 10.1002/ajh.25146
95. DiNardo CD, Tiong IS, Quaglieri A, MacRaild S, Loghavi S, Brown FC, et al. Molecular patterns of response and treatment failure after frontline venetoclax combinations in older patients with AML. Blood (2020) 135(11):791–803. doi: 10.1182/blood.2019003988
96. Larrue C, Saland E, Boutzen H, Vergez F, David M, Joffre C, et al. Proteasome inhibitors induce FLT3-ITD degradation through autophagy in AML cells. Blood (2016) 127(7):882–92. doi: 10.1182/blood-2015-05-646497
97. Walker AR, Wang H, Walsh K, Bhatnagar B, Vasu S, Garzon R, et al. Midostaurin, bortezomib and MEC in relapsed/refractory acute myeloid leukemia. Leuk Lymphoma (2016) 57(9):2100–8. doi: 10.3109/10428194.2015.1135435
98. Saliba AN, Boswell HS, Cripe LD, Abu Zaid MI, Weisenbach J, Sayar H. Final Results of Phase I/II Study of Combination of Sorafenib, Vorinostat, and Bortezomib in Acute Myeloid Leukemia with FLT3-ITD Mutation or Poor-Risk Cytogenetics. Blood (2017) 130(Supplement 1):3897–7. doi: 10.1182/blood.V130.Suppl_1.3897.3897
99. Kim KT, Baird K, Ahn JY, Meltzer P, Lilly M, Levis M, et al. Pim-1 is up-regulated by constitutively activated FLT3 and plays a role in FLT3-mediated cell survival. Blood (2005) 105(4):1759–67. doi: 10.1182/blood-2004-05-2006
100. Natarajan K, Xie Y, Burcu M, Linn DE, Qiu Y, Baer MR. Pim-1 kinase phosphorylates and stabilizes 130 kDa FLT3 and promotes aberrant STAT5 signaling in acute myeloid leukemia with FLT3 internal tandem duplication. PloS One (2013) 8(9):e74653. doi: 10.1371/journal.pone.0074653
101. Kapoor S, Natarajan K, Baldwin PR, Doshi KA, Lapidus RG, Mathias TJ, et al. Concurrent Inhibition of Pim and FLT3 Kinases Enhances Apoptosis of FLT3-ITD Acute Myeloid Leukemia Cells through Increased Mcl-1 Proteasomal Degradation. Clin Cancer Res (2018) 24(1):234–47. doi: 10.1158/1078-0432.CCR-17-1629
102. Jeon JY, Zhao Q, Buelow DR, Phelps M, Walker AR, Mims AS, et al. Preclinical activity and a pilot phase I study of pacritinib, an oral JAK2/FLT3 inhibitor, and chemotherapy in FLT3-ITD-positive AML. Invest New Drugs (2020) 38(2):340–9. doi: 10.1007/s10637-019-00786-4
103. Mohi MG, Boulton C, Gu TL, Sternberg DW, Neuberg D, Griffin JD, et al. Combination of rapamycin and protein tyrosine kinase (PTK) inhibitors for the treatment of leukemias caused by oncogenic PTKs. Proc Natl Acad Sci U S A (2004) 101(9):3130–5. doi: 10.1073/pnas.0400063101
104. Wang F, Liu Z, Zeng J, Zhu H, Li J, Cheng X, et al. Metformin synergistically sensitizes FLT3-ITD-positive acute myeloid leukemia to sorafenib by promoting mTOR-mediated apoptosis and autophagy. Leuk Res (2015) 39(12):1421–7. doi: 10.1016/j.leukres.2015.09.016
105. Kollmann K, Heller G, Schneckenleithner C, Warsch W, Scheicher R, Ott RG, et al. A Kinase-Independent Function of CDK6 Links the Cell Cycle to Tumor Angiogenesis. Cancer Cell (2016) 30(2):359–60. doi: 10.1016/j.ccell.2016.07.003
106. Uras IZ, Walter GJ, Scheicher R, Bellutti F, Prchal-Murphy M, Tigan AS, et al. Palbociclib treatment of FLT3-ITD+ AML cells uncovers a kinase-dependent transcriptional regulation of FLT3 and PIM1 by CDK6. Blood (2016) 127(23):2890–902. doi: 10.1182/blood-2015-11-683581
107. Keegan K, Li C, Li Z, Ma J, Ragains M, Coberly S, et al. Preclinical evaluation of AMG 925, a FLT3/CDK4 dual kinase inhibitor for treating acute myeloid leukemia. Mol Cancer Ther (2014) 13(4):880–9. doi: 10.1158/1535-7163.MCT-13-0858
108. Liu C LB, Xu C, Zhang P, Li B, Ji B, Zhang B, et al. ETH-155008, a Novel Selective Dual Inhibitor of FLT3 and CDK4/6 in Preclinical Treatment of Acute Myeloid Leukemia. Blood (2019) 134(Supplement_1):5141. doi: 10.1182/blood-2019-123589
109. Daver N, Pollyea DA, Rizzieri DA, Palmer J, Rampal RK, Dinner S, et al. A Phase I Study of FLX925, a Dual FLT3 and CDK4/6 Inhibitor in Patients with Relapsed or Refractory Acute Myeloid Leukemia (AML). Blood (2017) 130(Supplement 1):1343. doi: 10.1182/blood.V130.Suppl_1.1343.1343
110. Andreeff M ZW, Kumar P, Zernovak O, Daver NG, Isoyama T, Iwanaga K, et al. Synergistic Anti-Leukemic Activity with Combination of FLT3 Inhibitor Quizartinib and MDM2 Inhibitor Milademetan in FLT3-ITD Mutant/p53 Wild-Type Acute Myeloid Leukemia Models. Blood (2018) 132(Supplement 1):2720. doi: 10.1182/blood-2018-99-115183
111. Brodska B, Otevrelova P, Salek C, Fuchs O, Gasova Z, Kuzelova K. High PD-L1 Expression Predicts for Worse Outcome of Leukemia Patients with Concomitant NPM1 and FLT3 Mutations. Int J Mol Sci (2019) 20(11):2823. doi: 10.3390/ijms20112823
112. Smith CC, Lasater EA, Zhu X, Lin KC, Stewart WK, Damon LE, et al. Activity of ponatinib against clinically-relevant AC220-resistant kinase domain mutants of FLT3-ITD. Blood (2013) 121(16):3165–71. doi: 10.1182/blood-2012-07-442871
113. Shah NP, Talpaz M, Deininger MW, Mauro MJ, Flinn IW, Bixby D, et al. Ponatinib in patients with refractory acute myeloid leukaemia: findings from a phase 1 study. Br J Haematol (2013) 162(4):548–52. doi: 10.1111/bjh.12382
114. Lu JW, Wang AN, Liao HA, Chen CY, Hou HA, Hu CY, et al. Cabozantinib is selectively cytotoxic in acute myeloid leukemia cells with FLT3-internal tandem duplication (FLT3-ITD). Cancer Lett (2016) 376(2):218–25. doi: 10.1016/j.canlet.2016.04.004
115. Fathi AT, Blonquist TM, Hernandez D, Amrein PC, Ballen KK, McMasters M, et al. Cabozantinib is well tolerated in acute myeloid leukemia and effectively inhibits the resistance-conferring FLT3/tyrosine kinase domain/F691 mutation. Cancer (2018) 124(2):306–14. doi: 10.1002/cncr.31038
116. Smith CC, Levis MJ, Frankfurt O, Pagel JM, Roboz GJ, Stone RM, et al. A phase 1/2 study of the oral FLT3 inhibitor pexidartinib in relapsed/refractory FLT3-ITD-mutant acute myeloid leukemia. Blood Adv (2020) 4(8):1711–21. doi: 10.1182/bloodadvances.2020001449
117. Wu H, Hu C, Wang A, Weisberg EL, Wang W, Chen C, et al. Ibrutinib selectively targets FLT3-ITD in mutant FLT3-positive AML. Leukemia (2016) 30(3):754–7. doi: 10.1038/leu.2015.175
118. Yamaura T, Nakatani T, Uda K, Ogura H, Shin W, Kurokawa N, et al. A novel irreversible FLT3 inhibitor, FF-10101, shows excellent efficacy against AML cells with FLT3 mutations. Blood (2018) 131(4):426–38. doi: 10.1182/blood-2017-05-786657
119. Ma HS, Nguyen B, Duffield AS, Li L, Galanis A, Williams AB, et al. FLT3 kinase inhibitor TTT-3002 overcomes both activating and drug resistance mutations in FLT3 in acute myeloid leukemia. Cancer Res (2014) 74(18):5206–17. doi: 10.1158/0008-5472.CAN-14-1028
120. Lee HK, Kim HW, Lee IY, Lee J, Lee J, Jung DS, et al. G-749, a novel FLT3 kinase inhibitor, can overcome drug resistance for the treatment of acute myeloid leukemia. Blood (2014) 123(14):2209–19. doi: 10.1182/blood-2013-04-493916
121. Xu B, Zhao Y, Wang X, Gong P, Ge W. MZH29 is a novel potent inhibitor that overcomes drug resistance FLT3 mutations in acute myeloid leukemia. Leukemia (2017) 31(4):913–21. doi: 10.1038/leu.2016.297
122. Daver NG LK, Yoon S, Jung CW, Kang HJ, Jung S, Seo S, et al. HM43239, a Novel Potent Small Molecule FLT3 Inhibitor, in Acute Myeloid Leukemia (AML) with FMS-like Tyrosine Kinase 3 (FLT3) Mutations: Phase 1 /2 Study. Blood (2019) 134(Supplement_1):1331. doi: 10.1182/blood-2019-129670
123. Kayser S HJ, Dörfel D, Thol F, Heuser M, Märklin M, Müller-Tidow C, et al. Interim Results of a First in Man Study with the Fc-Optimized FLT3 Antibody Flysyn for Treatment of Acute Myeloid Leukemia with Minimal Residual Disease. Blood (2019) 134(Supplement_1):3928. doi: 10.1182/blood-2019-125327
124. Rudra-Ganguly N LC, Virata C, Leavitt M, Jin L, Mendelsohn B, Snyder J, et al. AGS62P1, a Novel Anti-FLT3 Antibody Drug Conjugate, Employing Site Specific Conjugation, Demonstrates Preclinical Anti-Tumor Efficacy in AML Tumor and Patient Derived Xenografts. Blood (2015) 12623):3806. doi: 10.1182/blood.V126.23.3806.3806
125. Yeung YA, Krishnamoorthy V, Dettling D, Sommer C, Poulsen K, Ni I, et al. An Optimized Full-Length FLT3/CD3 Bispecific Antibody Demonstrates Potent Anti-leukemia Activity and Reversible Hematological Toxicity. Mol Ther (2020) 28(3):889–900. doi: 10.1016/j.ymthe.2019.12.014
126. Wang Y, Xu Y, Li S, Liu J, Xing Y, Xing H, et al. Targeting FLT3 in acute myeloid leukemia using ligand-based chimeric antigen receptor-engineered T cells. J Hematol Oncol (2018) 11(1):60. doi: 10.1186/s13045-018-0603-7
127. Jetani H, Garcia-Cadenas I, Nerreter T, Thomas S, Rydzek J, Meijide JB, et al. CAR T-cells targeting FLT3 have potent activity against FLT3(-)ITD(+) AML and act synergistically with the FLT3-inhibitor crenolanib. Leukemia (2018) 32(5):1168–79. doi: 10.1038/s41375-018-0009-0
128. Larson R, Mandrekar S, Sanford BL, Laumann K, Geyer SM, Bloomfield CD, et al. An Analysis of Maintenance Therapy and Post-Midostaurin Outcomes in the International Prospective Randomized, Placebo-Controlled, Double-Blind Trial (CALGB 10603/RATIFY [Alliance]) for Newly Diagnosed Acute Myeloid Leukemia (AML) Patients with FLT3 Mutations. Blood (2017) 2017130(Supplement 1):145. doi: 10.1182/blood.V130.Suppl_1.145.145
129. Schlenk RF, Weber D, Fiedler W, Salih HR, Wulf G, Salwender H, et al. Midostaurin added to chemotherapy and continued single-agent maintenance therapy in acute myeloid leukemia with FLT3-ITD. Blood (2019) 133(8):840–51. doi: 10.1182/blood-2018-08-869453
130. Maziarz RTT PM, Scott BL, Mohan SR, Deol A, Rowley SD, Kim D, et al. Radius: A Phase 2 Randomized Trial Investigating Standard of Care ± Midostaurin after Allogeneic Stem Cell Transplant in FLT3-ITD-Mutated AML. Blood (2018) 132(Supplement 1):662. doi: 10.1182/blood-2018-99-113582
131. Brunner AM, Li S, Fathi AT, Wadleigh M, Ho VT, Collier K, et al. Haematopoietic cell transplantation with and without sorafenib maintenance for patients with FLT3-ITD acute myeloid leukaemia in first complete remission. Br J Haematol (2016) 175(3):496–504. doi: 10.1111/bjh.14260
132. Chen YB, Li S, Lane AA, Connolly C, Del Rio C, Valles B, et al. Phase I trial of maintenance sorafenib after allogeneic hematopoietic stem cell transplantation for fms-like tyrosine kinase 3 internal tandem duplication acute myeloid leukemia. Biol Blood Marrow Transplant (2014) 20(12):2042–8. doi: 10.1016/j.bbmt.2014.09.007
133. Burchert A, Bug G, Fritz LV, Finke J, Stelljes M, Rollig C, et al. Sorafenib Maintenance After Allogeneic Hematopoietic Stem Cell Transplantation for Acute Myeloid Leukemia With FLT3-Internal Tandem Duplication Mutation (SORMAIN). J Clin Oncol (2020) 38(26):2993–3002. doi: 10.1200/JCO.19.03345
134. Sandmaier BM, Khaled S, Oran B, Gammon G, Trone D, Frankfurt O. Results of a phase 1 study of quizartinib as maintenance therapy in subjects with acute myeloid leukemia in remission following allogeneic hematopoietic stem cell transplant. Am J Hematol (2018) 93(2):222–31. doi: 10.1002/ajh.24959
135. Maziarz RT, Fernandez H, Patnaik MM, Scott BL, Mohan S, Deol A, et al. Radius: Midostaurin (mido) Plus Standard of Care (SOC) after Allogeneic Stem Cell Transplant (alloSCT) in Patients (pts) with FLT3-Internal Tandem Duplication (ITD)–Mutated Acute Myeloid Leukemia (AML). Biol Blood Marrow Transplant (2019) 25(3):S11–2. doi: 10.1016/j.bbmt.2018.12.077
136. Bornhauser M, Illmer T, Schaich M, Soucek S, Ehninger G, Thiede C, et al. Improved outcome after stem-cell transplantation in FLT3/ITD-positive AML. Blood (2007) 109(5):2264–5. doi: 10.1182/blood-2006-09-047225
137. DeZern AE, Sung A, Kim S, Smith BD, Karp JE, Gore SD, et al. Role of allogeneic transplantation for FLT3/ITD acute myeloid leukemia: outcomes from 133 consecutive newly diagnosed patients from a single institution. Biol Blood Marrow Transplant (2011) 17(9):1404–9. doi: 10.1016/j.bbmt.2011.02.003
138. Dohner K, Thiede C, Jahn N, Panina E, Gambietz A, Larson RA, et al. Impact of NPM1/FLT3-ITD genotypes defined by the 2017 European LeukemiaNet in patients with acute myeloid leukemia. Blood (2020) 135(5):371–80. doi: 10.1182/blood.2019002697
139. Sakaguchi M, Yamaguchi H, Najima Y, Usuki K, Ueki T, Oh I, et al. Prognostic impact of low allelic ratio FLT3-ITD and NPM1 mutation in acute myeloid leukemia. Blood Adv (2018) 2(20):2744–54. doi: 10.1182/bloodadvances.2018020305
140. Oran B, Cortes J, Beitinjaneh A, Chen HC, de Lima M, Patel K, et al. Allogeneic Transplantation in First Remission Improves Outcomes Irrespective of FLT3-ITD Allelic Ratio in FLT3-ITD-Positive Acute Myelogenous Leukemia. Biol Blood Marrow Transplant (2016) 22(7):1218–26. doi: 10.1016/j.bbmt.2016.03.027
141. Bazarbachi A, Bug G, Baron F, Brissot E, Ciceri F, Dalle IA, et al. Clinical practice recommendation on hematopoietic stem cell transplantation for acute myeloid leukemia patients with FLT3-internal tandem duplication: a position statement from the Acute Leukemia Working Party of the European Society for Blood and Marrow Transplantation. Haematologica (2020) 105(6):1507–16. doi: 10.3324/haematol.2019.243410
142. Kayser S, Schlenk RF, Londono MC, Breitenbuecher F, Wittke K, Du J, et al. Insertion of FLT3 internal tandem duplication in the tyrosine kinase domain-1 is associated with resistance to chemotherapy and inferior outcome. Blood (2009) 114(12):2386–92. doi: 10.1182/blood-2009-03-209999
143. Herold T, Rothenberg-Thurley M, Grunwald VV, Janke H, Goerlich D, Sauerland MC, et al. Validation and refinement of the revised 2017 European LeukemiaNet genetic risk stratification of acute myeloid leukemia. Leukemia (2020) 34:3161–72. doi: 10.1038/s41375-020-0806-0
144. Frohling S, Scholl C, Levine RL, Loriaux M, Boggon TJ, Bernard OA, et al. Identification of driver and passenger mutations of FLT3 by high-throughput DNA sequence analysis and functional assessment of candidate alleles. Cancer Cell (2007) 12(6):501–13. doi: 10.1016/j.ccr.2007.11.005
145. Tarlock K HM, Hylkema T, Ries R, Farrar JE, Auvil JG, Gerhard DS, et al. Discovery and Functional Validation of Novel Pediatric Specific FLT3 Activating Mutations in Acute Myeloid Leukemia: Results from the COG/NCI Target Initiative. Blood (2015) 126(23):87. doi: 10.1182/blood.V126.23.87.87
146. Daver N, Price A, Benton CB, Patel K, Zhang W, Konopleva M, et al. First Report of Sorafenib in Patients With Acute Myeloid Leukemia Harboring Non-Canonical FLT3 Mutations [Case Report]. Front Oncol (2020) 10:1538(1538):1538. doi: 10.3389/fonc.2020.01538
147. Heidel F, Solem FK, Breitenbuecher F, Lipka DB, Kasper S, Thiede MH, et al. Clinical resistance to the kinase inhibitor PKC412 in acute myeloid leukemia by mutation of Asn-676 in the FLT3 tyrosine kinase domain. Blood (2006) 107(1):293–300. doi: 10.1182/blood-2005-06-2469
148. Pauwels D, Sweron B, Cools J. The N676D and G697R mutations in the kinase domain of FLT3 confer resistance to the inhibitor AC220. Haematologica (2012) 97(11):1773–4. doi: 10.3324/haematol.2012.069781
149. Tarver TC, Hill JE, Rahmat L, Perl AE, Bahceci E, Mori K, et al. Gilteritinib is a clinically active FLT3 inhibitor with broad activity against FLT3 kinase domain mutations. Blood Adv (2020) 4(3):514–24. doi: 10.1182/bloodadvances.2019000919
150. Malaise M, Steinbach D, Corbacioglu S. Clinical implications of c-Kit mutations in acute myelogenous leukemia. Curr Hematol Malig Rep (2009) 4(2):77–82. doi: 10.1007/s11899-009-0011-8
151. Chen W, Xie H, Wang H, Chen L, Sun Y, Chen Z, et al. Prognostic Significance of KIT Mutations in Core-Binding Factor Acute Myeloid Leukemia: A Systematic Review and Meta-Analysis. PloS One (2016) 11(1):e0146614. doi: 10.1371/journal.pone.0146614
152. Levis MJ, Perl AE, Dombret H, Döhner H, Steffen B, Rousselot P, et al. Final Results of a Phase 2 Open-Label, Monotherapy Efficacy and Safety Study of Quizartinib (AC220) in Patients with FLT3-ITD Positive or Negative Relapsed/Refractory Acute Myeloid Leukemia After Second-Line Chemotherapy or Hematopoietic Stem Cell Transplantation. Blood (2012) 120(21):673–3. doi: 10.1182/blood.V120.21.673.673
153. Wattad M, Weber D, Dohner K, Krauter J, Gaidzik VI, Paschka P, et al. Impact of salvage regimens on response and overall survival in acute myeloid leukemia with induction failure. Leukemia (2017) 31(6):1306–13. doi: 10.1038/leu.2017.23
154. Choe S, Wang H, DiNardo CD, Stein EM, de Botton S, Roboz GJ, et al. Molecular mechanisms mediating relapse following ivosidenib monotherapy in IDH1-mutant relapsed or refractory AML. Blood Adv (2020) 4(9):1894–905. doi: 10.1182/bloodadvances.2020001503
155. Quek L, David MD, Kennedy A, Metzner M, Amatangelo M, Shih A, et al. Clonal heterogeneity of acute myeloid leukemia treated with the IDH2 inhibitor enasidenib. Nat Med (2018) 24(8):1167–77. doi: 10.1038/s41591-018-0115-6
156. Abbas HA, Alfayez M, Kadia T, Ravandi-Kashani F, Daver N. Midostaurin In Acute Myeloid Leukemia: An Evidence-Based Review And Patient Selection. Cancer Manag Res (2019) 11:8817–28. doi: 10.2147/CMAR.S177894.Citedin:Pubmed
Keywords: Acute Myeloid Leukemia, FLT3 inhibitor, FLT3 resistance, FLT3 inhibitor maintenance, non-canonical FLT3 mutation
Citation: Kennedy VE and Smith CC (2020) FLT3 Mutations in Acute Myeloid Leukemia: Key Concepts and Emerging Controversies. Front. Oncol. 10:612880. doi: 10.3389/fonc.2020.612880
Received: 01 October 2020; Accepted: 19 November 2020;
Published: 23 December 2020.
Edited by:
Alessandro Isidori, AORMN Hospital, ItalyReviewed by:
Adolfo De La Fuente, MD Anderson Cancer Center Madrid, SpainHussein A. Abbas, M D Anderson Cancer Center, United States
Copyright © 2020 Kennedy and Smith. This is an open-access article distributed under the terms of the Creative Commons Attribution License (CC BY). The use, distribution or reproduction in other forums is permitted, provided the original author(s) and the copyright owner(s) are credited and that the original publication in this journal is cited, in accordance with accepted academic practice. No use, distribution or reproduction is permitted which does not comply with these terms.
*Correspondence: Vanessa E. Kennedy, dmFuZXNzYS5rZW5uZWR5QHVjc2YuZWR1