- 1Medical Center of Hematology, The Xinqiao Hospital of Third Military Medical University, Chongqing, China
- 2State Key Laboratory of Trauma, Burns and Combined Injury, Third Military Medical University, Chongqing, China
- 3Chongqing Sub-center of National Clinical Research Center for Hematologic Disease, Chongqing, China
- 4Irell and Manella Graduate School of Biological Sciences of City of Hope, Duarte, CA, United States
- 5Department of Chemical Defense Medicine, School of Military Preventive Medicine, Third Military Medical University, Chongqing, China
The mTOR pathway plays a central role in many cellular processes, such as cellular growth, protein synthesis, glucose, and lipid metabolism. Aberrant regulation of mTOR is a hallmark of many cancers, including hematological malignancies. mTOR inhibitors, such as Rapamycin and Rapamycin analogs (Rapalogs), have become a promising class of agents to treat malignant blood diseases—either alone or in combination with other treatment regimens. This review highlights experimental evidence underlying the molecular mechanisms of mTOR inhibitors and summarizes their evolving role in the treatment of hematologic disease, including leukemia, lymphoma, myeloma, immune hemocytopenia, and graft-versus-host disease (GVHD). Based on data presented in this review, we believe that mTOR inhibitors are becoming a trusted therapeutic in the clinical hematologist’s toolbelt and should be considered more routinely in combination therapy for the management of hematologic disease.
The mammalian target of rapamycin (mTOR) is a downstream target of multiple signaling pathways involved in biological activities, including the familiar PI3K/Akt/mTOR signaling pathway, which plays an important role in cell growth, differentiation, metastasis and survival, and has become an important target of cancer treatment. Rapamycin (Rapa), the first mTOR inhibitor, was initially used as an immunosuppressive drug in the field of solid organ transplantation (1). In-depth studies of rapamycin and its analogs (Rapalogs), and of the mTOR signaling pathway, have led to the understanding that Rapalogs can not only induce tumor cell apoptosis, cell cycle arrest and signal transduction inhibition, but that they also affect gene transcription and epigenetic regulation. The mTOR signaling pathway is critical in normal myeloid and lymphoid development and function. Moreover, hyperactivation of mTOR is a hallmark of many hematological diseases and provides a strong rationale for the use of mTOR inhibitors (mTORi), such as Rapalogs. The primary focus of this review is to 1) review the literature on the regulation and outcome of the mTOR signaling pathways from a basic research perspective, and 2) summarize the use of mTOR inhibitors to target aberrant mTOR activation and signaling in hematological diseases, such as acute leukemia, Hodgkin lymphoma, non-Hodgkin lymphoma, multiple myeloma, GVHD, and Waldenström macroglobulinemia. We also provide our perspective on the evolution of this field, as the application and efficacy of mTOR inhibitors becomes more widely recognized in the field of blood disorders.
mTOR And mTOR Inhibitors
The mammalian target of rapamycin (mTOR) is a large (289 kDa), conserved serine protein kinase, belonging to the PI3K kinase family. mTOR can integrate the stimulatory signals from nutrients, growth factors and environmental pressure, and regulate cell growth, proliferation, differentiation, and cell cycle progression. In the immune system, mTOR can transmit and integrate signals from the immune microenvironment, and is considered a key regulator of immune metabolism and function (2). At present, it is believed that there are two dominant, upstream signaling pathways for mTOR, one is the PI3K/Akt/mTOR pathway, which interacts with mTOR to positively regulate, and the other is the LKB1/AMPK/mTOR pathway, which has primarily been reported as a negative regulator of mTOR (3, 4).
mTOR exerts its activity via the formation of two catalytically distinct complexes, mTORC1 and mTORC2 (5). mTORC1 complex is composed of mLST8, PRAS40, Deptor, and Raptor. mTORC2 complex is composed of Rictor, mSIN1, Protor, mLST8, and Deptor. In general, mTORC1 plays a more dominant role in regulating cellular anabolic processes, while the effect of the mTORC2 signaling pathway is primarily through Akt Studies have shown that mTORC1 signaling pathway plays an important role in the metabolism of glucose, lipids, nucleotides, and proteins as well as in the regulation of mitochondrial biogenesis (6). The downstream signaling molecules of mTORC1 are primarily P70S6K (p70 ribosomal S6 kinase) and 4E-BP1 (eukaryotic transcription initiation factor binding protein), by which protein translation and synthesis are increased (Figure 1). On the other hand, mTORC2 is primarily involved in the insulin signaling pathway to regulate glucose and lipid metabolism (7). Importantly, when mTORC1 is completely inhibited by mTOR inhibitors, mTORC2 signaling is preserved (8).
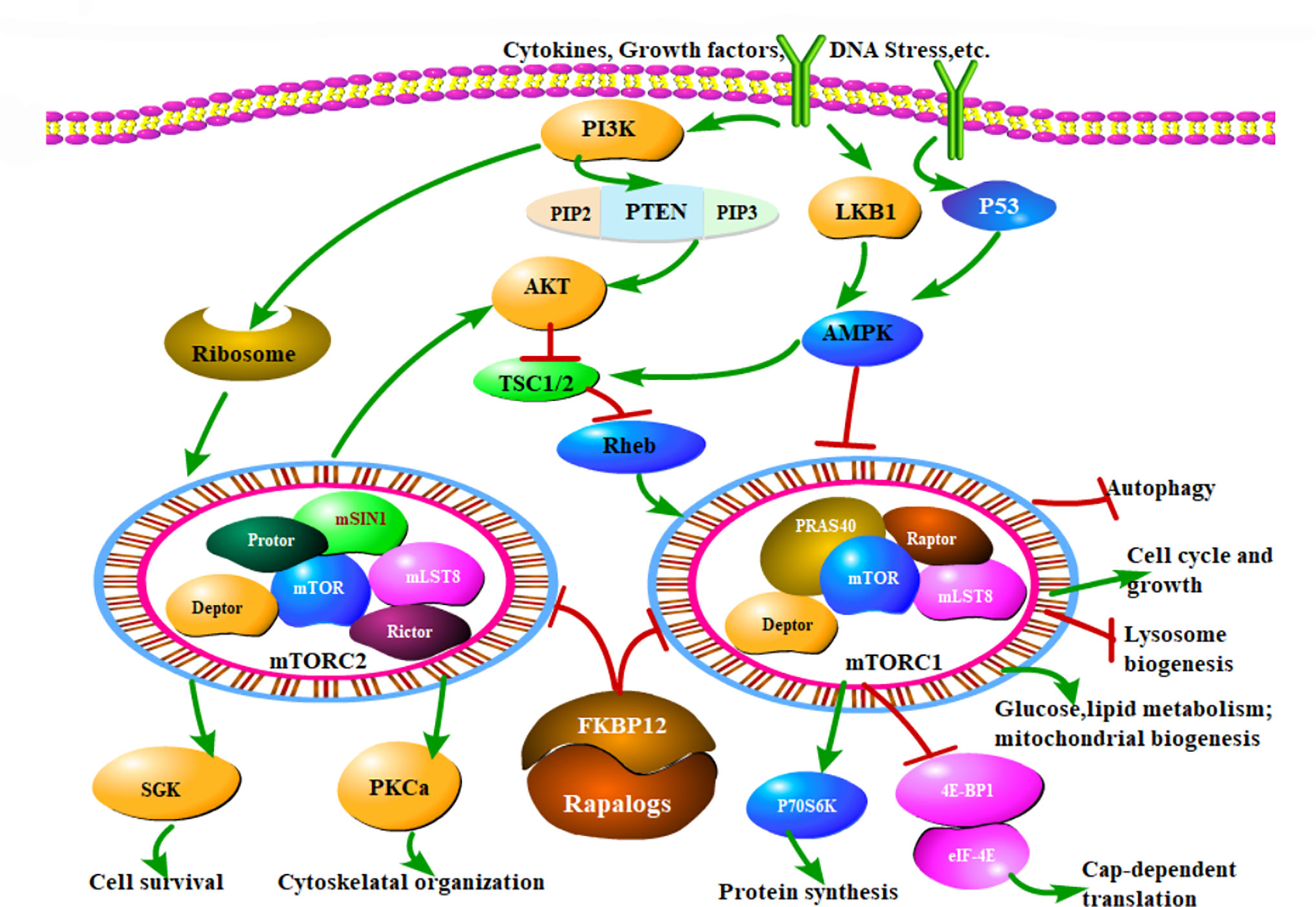
Figure 1 The mTOR signaling pathway and Rapalog therapeutic mechanisms. mTOR modulates a variety of cellular activities through mTORC1 and mTORC2. MTOR can respond to extracellular stimuli, such as cytokines, growth factors and DNA stress, which mainly regulate cell growth, cell cycle, and other physiological activities through PI3K/Akt/mTOR pathway. Normally, TSC1/TSC2 forms a dimer complex, which is an inhibitor of small GTPase Rheb, and Rheb is a necessary stimulating protein for mTOR activation. Hence the mTORC1 activity is critically inhibited by TSC1/TSC2 complex. When Akt is activated, it can phosphorylate ser939 and thr1462 of TSC2 and inhibit the formation of TSC1/TSC2 complex, thus relieving the inhibition of Rheb, then activated Rheb sensitized mTOR function. Additionally, PI3K activates the ribosome to increase mTORC2 reaction. In a word, PI3K promotes the mTOR activates. Another important pathway of mTOR is regulated by AMPK. Firstly, AMPK directly phosphorylates Raptor to inhibit mTORC1 activity. Raptor is the downstream molecule of AMPK, and TSC2 is not involved in this process. Secondly, AMPK can also activate TSC1/TSC2 complex and inhibit mTORC1 activity as described above. Therefore, unlike the PI3K, AMPK restrains the mTOR function. Rapalogs can combine the FKBP12 and inhibit the kinase activity of mTORC1 and mTORC2, ultimately regulate various biological functions of cells, that is the mechanism of mTOR inhibitors. Arrows indicate activation and bars represent inhibition.
Several pharmacological agents have been developed which inhibit mTOR (mTOR inhibitors; referred to broadly here as mTORi), including Rapamycin and its analogs. Rapamycin, clinically named sirolimus (SRL), was a macrolide antibiotic discovered in the 1970s (9). SRL has many functional properties, including inhibition of yeast growth, anti-cancer, anti-aging and anti-atherosclerotic effects as well as immune regulation (10–12). In the context of immune regulation, SRL can inhibit T cell activation and proliferation, and restrain B cell activation and antibody production caused by antigens and cytokines (13). In the clinic, SRL is primarily used in the prevention of organ rejection after solid organ transplantation, as well as for the treatment of autoimmune diseases (14). With the development of Rapamycin analogs in recent years, Rapalogs, many studies have reported their therapeutic effects on hematological diseases (15).
To date, the most common Rapalogs are: Everolimus (Eve, RAD-001), Deforolimus (Def, Ridaforolimus), Zotarolimus (Zot), and Temsirolimus (Tem, CCI-779) (Figure 2). Everolimus is an mTOR inhibitor developed by Novartis (Switzerland), which is a 40-o-(2-hydroxyethyl) derivative of rapamycin, with the molecular formula C53H83NO14, (958 kDa). In March 2009, the drug received accelerated approval from the FDA for the treatment of kidney cancer (16, 17). Deforolimus is a C40 derivative of Rapa, which is a semi-synthetic derivative designed by Computer-aided drug design (CADD) with molecular formula C53H84NO14P (990 kDa) (18). Def is still considered an investigational agent in the management of advanced solid tumors, such as sarcoma (19) and breast cancer (20). Only clinical study reported using Def for the treatment of the hematological malignancy lymphoma, and the overall response rate (ORR) was 50% (21). Zotarolimus is a Rapa derivative developed by Abbott and Medtronic. It is a C40 tetrazole substitute of Rapa, with molecular formula C52H79N5O12 (966 kDa). Compared with Rapa, Zot has a shorter half-life in vivo. The mechanism of Zot is similar to Rapa. Zot combines with FKBP12 to form a complex, which bind to mTOR protein kinase to form a trimer, inhibits the activity of mTOR by preventing its phosphorylation, and prevents cell cycle progression from G1 to S phase (22). It was designed for use as a coating in stents with phosphorylcholine as a carrier. Temsirolimus, also named 42-[3-hydroxy-2- (hydroxymethyl)-2-methylpropionate], is a Rapa derivative with molecular formula C56H87NO16 (1030 kDa). It is a Rapa soluble esterified derivative officially approved by the US FDA in 2007, which inhibits the translation of several key proteins for cell cycle regulation. These effects lead to cell cycle arrest in the G1 phase, therefore also known as Cell Cycle Inhibitor-779 (CCI-779). In August 2007, FDA approved temsirolimus as a first-line treatment for advanced renal cancer. At present, it is marketed as a treatment of renal cancer, and is being investigated in the clinic for the treatment of breast cancer (23), lymphoma (24), lung cancer (25), and many other tumors (26).
Applications of mTORi in Hematological Malignancies
Acute Leukemia
Acute myeloid leukemia (AML) is a type of cancer of the bone marrow in which myeloid stem cells differentiate and accumulate as abnormal myeloblasts in the bone marrow, impair erythropoiesis/hematopoiesis and eventually migrate throughout the body. Although mTOR activation is frequently observed in AML blasts, the precise function and the downstream targets of mTOR in this disease are poorly understood. Feng et al. revealed that PFKFB3 was a novel downstream substrate of mTOR signaling pathway, and found that up-regulation of PFKFB3 via aberrant mTOR signaling was essential for AML cell survival. Moreover, PFKFB3 inhibitor PFK15 and rapamycin synergistically ablated AML cell proliferation (THP1 and OCI-AML3 cells) (27). Xu et al. found that PI3K/Akt/mTOR pathway was up-regulated in most AML cells. Rapa combined with etoposide, a chemotherapy, enhanced the effect of etoposide on primary AML cells; moreover, leukemia progenitor cells were more sensitive to Rapa than normal progenitor cells (28, 29). Brown et al. have found that rapamycin inhibited growth of B-precursor ALL cell lines in vitro, as evidenced by apoptotic cell death. Mice with advanced ALL and were treated with rapamycin as a single agent exhibited enhanced survival compared to control-treated ALL-bearing animals (30). Avellino et al. reported SRL (+/- doxorubicin) can promote the apoptosis of primary pediatric ALL blast cells in vitro, indicating that rapamycin targets two pathways that are crucial for cell survival and chemoresistance of malignant lymphoblasts (PI3k/Akt and FKBP51/NF-kB) (31). In a phase I/II study of everolimus in combination with HyperCVAD in patients with R/R ALL, ORR was in 41% and significantly higher in first salvage patients as compared with second salvage or beyond. Moreover the inhibition of phosphorylation of S6K1 did not correlate with response, and the combination regimen did not increased toxicity than HyperCVAD alone (32). Thus, the combination of Rapalogs with some chemotherapies may be an effective regimen for AML and ALL.
Results of clinical trials using Rapalogs as a single agent have shown very limited response in AML patients, such as sirolimus (33), deforolimus (21), or everolimus (34). There are also reports of combination strategies; for example, SRL was combined with MEC (mitoxantrone, etoposide, cytarabine) to treat patients with relapsed, refractory, or untreated high-risk AML. The ORR to the combination regimen was 16%–47% (35–37), which suggested SRL and MEC may be an effective option as a treatment regimen for AML. However, Burnett et al. added everolimus to consolidation therapy in AML, and reported there was no difference in relapse-free survival and overall survival (38). This study suggests that the addition of everolimus to chemotherapy provides no benefit (38). Interestingly, Recher et al. found that some primary AML cells have innate Rapa-resistance, due to several mechanisms, including FKBP-12 mutations, high eIF-4/4E-BP1 ratio, defective regulation of p27kip1, and c-myc amplification (33). Taken together, combination of Rapalogs with other chemotherapies might be an effective regimen for patients with high-risk acute leukemia; however, stratification of patients who are likely to respond to these regimens, perhaps based on underlying mutation patterns, must be better understood.
Myelodysplastic Syndrome
Myelodysplastic syndrome (MDS) is a group of hematopoietic stem cell disorders, characterized by ineffective clonal hematopoiesis leading to blood cytopenias, and convey a variable risk of progression to AML (39). Activation of the mTOR pathway in CD33+ cells from MDS patients by the amino acid L-leucine in 5q- syndrome was reported by Yip BH et al. (40). Importantly, in primary cells from high-risk MDS patients, not only was mTOR activated but also its downstream targets, P70S6K and 4E-BP1. Treatment with Rapa significantly increased CD33+ cells apoptosis from high-risk MDS patients, but not cells from healthy donors or those patients with low-risk MDS (41). Mutated GSTT1 gene was detected in some MDS patients and this mutation creates a sequence that is 63% homologous to human FKBP-rapamycin associated protein (FRAP). To examine the effect of mutant GSTT-1, two cell lines (K562 and HL-60) were stably transfected with the mutant type GSTT1 gene. Expectedly, rapamycin induced significant growth inhibition of those two cell lines, suggesting that rapamycin could be included as a potential therapeutic modality for high-risk MDS patients (42).
Platzbecker et al. (43) treated 19 MDS patients with SRL as a single agent, and demonstrated that SRL might have some activity in patients with more advanced MDS but lacked efficacy in low-risk MDS patients. Martin et al. (44) treated 20 MDS patients with temsirolimus at a weekly dose of 25 mg; however, only four (20%) reached the response assessment after 4 months without hematological improvement. Based on these this response assessment, they concluded that Temsirolimus has no beneficial effects in elderly MDS patients. However, because MDS is a highly heterogeneous disease, a more in-depth study on the therapeutic effect of Rapalogs in patients with MDS is warranted.
Chronic Myeloid Leukemia
CML is a hematopoietic disorder characterized by the malignant expansion of bone marrow stem cells, with the presence of a reciprocal translocation between chromosomes 9 and 22 resulting in activation of fusion gene BCR-ABL expression (45). It has been found that BCR/ABL, a common driver mutation in CML, mediates the expression of VEGF and its transcriptional activator HIF1 through PI3K and mTOR pathways (46). It also found that BCR/ABL regulates P70S6K and 4E-BP1 through mTOR activation (47–49). In vitro, Rapamycin (combined with celecoxib) induced cell cycle arrest and apoptosis of the CML cell line K562. As we know, tyrosine kinase inhibitors (TKIs) are the first line therapy for CML, implying that a combination of TKI with mTORi could enhance the antitumor effects of TKI treatment on CML cells (50). Several groups have, indeed, demonstrated this synergistic effect, combining imatinib with rapamycin (47, 51), or everolimus (52) to overcome TKI resistance in vitro, including cell line K562, murine CML model and CML patients samples. However, whether Rapalogs represent a new treatment opportunity for TKI resistant CML remains to be investigated in the clinic.
Myeloproliferative Neoplasms
MPNs often begin with an abnormal gene mutation or change in a stem cell in the bone marrow, resulting in hemocytopenia and splenomegaly, etc. Types of MPN include myelofibrosis, polycythemia vera and essential thrombocythemia (53). Bartalucci et al. found that mTOR signaling pathway was activated in MPN including myelofibrosis (MF), which was located downstream of JAK2 signaling pathway (54). Guglielmelli et al. (55) carried out treatment of patients with myelofibrosis with everolimus, resulting in 20% of patients’ spleens shrinking > 50%, 40% of patients’ spleens shrinking > 30%, and 15%–25% of patients with hematological reaction. In other studies (56, 57), JAK2/V617F mutated leukemia cell lines (HEL and SET2) and patients’ samples of PV or PMF were sensitive to everolimus. Moreover, the combination of JAKs inhibitor (ruxolitinib) and everolimus, showed synergy in inducing cell-cycle arrest and blockade of cell proliferation (58), which is promising for MPN treatment.
Hodgkin Lymphoma
Aberrant activation of the PI3K/Akt/mTOR pathway is a hallmark of lymphomas, including Hodgkin lymphoma (HL) (59, 60) and non-Hodgkin lymphomas (NHLs) (61, 62). Abnormal activation of upstream or downstream molecules of mTOR can cause disease development. mTOR mutations have been described in diffuse large B-cell lymphoma (DLBCL) samples (63), and activated ABC-DLBCL cell lines expressed high level of S6K1, which is a downstream target of mTOR (64). mTOR directly mediates Cyclin D1 downregulation through glycogen synthase kinase (GSK)-3b in mantle cell lymphoma (MCL) (65). Based on these observations and preclinical data, clinical trial using Rapalogs have been carried out in patients with lymphoma (66).
Everolimus has significant activity in relapsed/refractory (R/R) HL and is currently listed as a treatment option for HL in the National Comprehensive Cancer Network (NCCN) guidelines (67). Everolimus demonstrates single-agent activity in treating Hodgkin (67–71) and non-Hodgkin lymphoma (72–76), with responses ranging from 20% to 47%. In a small phase 2 study, 19 patients with R/R HL were treated with single-agent everolimus (10 mg/d), with an ORR of 47% (69). This was recapitulated in a larger multicenter study of 57 patients with an ORR of 42%, including five complete responses (CRs) and a median PFS of 9 months (68). The combination of sirolimus and HDAC inhibitor (vorinostat) is well-tolerated with encouraging activity in very heavily pretreated patients with Hodgkin lymphoma who are refractory to standard therapies. In 28 patients enrolled and accepted SRL and vorinostat scheme, the ORR was 57% (CR 32%+PR 25%) (77, 78). The emergence of effective new drugs in the treatment HL, including CD30 mAbs and checkpoint inhibitors, such as PD-(L)1 mAbs, may limit the broad application of mTORi in this setting. However, mTORi may be useful in later lines of therapy for difficult-to-treat, multidrug-exposed patients with R/R HL.
Non-Hodgkin Lymphomas
There are also clinical trials using Rapalogs to treat NHL, for example, temsirolimus is used for relapsed MCL with an ORR 38-47% (79, 80). A phase I b clinical trial investigated temsirolimus in association with R-CHOP (R-CHOP-T), or high-dose cytarabine plus rituximab (R-DHA-T), or fludarabine, cyclophosphamide plus rituximab (R-FC-T). Regarding efficacy, ORR during treatment period was 40% for R-CHOP-T including two patients who reached CR, 43% for R-FC-T including three patients who reached CR, and 47% for R-DHA-T including six patients who reached CR (81). In another study, Temsirolimus combined with bortezomib in DLBCL achieved an ORR of 31% (82). Yet another study investigated single-agent temsirolimus 25 mg weekly administrated in three groups of patients with relapsed aggressive and indolent lymphomas: group A (diffuse large B-cell lymphoma, transformed follicular lymphoma), group B (follicular lymphoma), and group C (chronic lymphocytic leukemia/small lymphocytic lymphoma, and other indolent lymphomas). Group A had an overall and complete response rate of 28.1% and 12.5%, respectively, and median PFS of 2.6 months and median OS of 7.2 months. Group B had overall and complete response rates of 53.8% and 25.6%, respectively, and median PFS of 12.7 months; median OS has not yet been reached. Group C had a partial response rate of 11% with no complete responders (83). Everolimus has been used to treat R/R marginal zone lymphomas (MZLs) with an ORR of 25% (75). Temsirolimus and everolimus are not very effective in lymphoma, which may be due to inhibition of mTORC1 but not mTORC2, resulting in feedback activation of PI3K/Akt (84). As for chronic lymphocytic leukemia (CLL), Decker et al. found that Rapamycin mediates the arrest of B cells of CLL in G1 phase (85). Three cases of lymphoproliferative diseases after transplantation have been successfully treated with rituximab and Rapamycin (86). Clinical evidence indicate that everolimus has a positive effect in patients with lymphoplasmacytic lymphoma/Waldenström macroglobulinemia (LPL/WM) and ORR can reach 70% (87). Taken together, subtypes of lymphoma may respond differently to mTORi; additional studies are needed to clarify the benefit/risk ratio of mTORi use in these settings.
Multiple Myeloma
Multiple myeloma (MM) is a hematological malignancy characterized by abnormal production of immunoglobulins by malignant plasma cells (PCs). Previous studies have indicated that the PI3K/Akt/mTOR signaling pathway is aberrantly activated in MM cells (88, 89). MM blast cells secrete growth factor IL-6 and IGF-1 to activate PI3K/Akt, which induce abnormal expression of mTOR (90). Following curcumin treatment, mRNA and protein expression levels of mTOR were decreased, inducing apoptosis in MM cell lines, indicating a potential novel therapy for MM (91). Lamanuzzi A et al. (92) evaluated endothelial cells (ECs) from 20 patients with monoclonal gammopathy of undetermined significance (MGUS) and 47 patients with MM, and found higher activation of mTORC2 downstream effectors, suggesting a major role of mTORC2 in the angiogenic switch to MM. Inhibition of mTORC2 with lenalidomide and bortezomib exhibited a synergistic anti-angiogenic effect (92). Combining rapamycin with resveratrol has a synergistic effect in inhibition of myeloma cell line viability (93). Everolimus shows synergistic anti-myeloma effects with bortezomib through inhibition of the Akt/mTOR pathway in both the MM cell lines and MM-bearing mice model (94, 95).
In a clinical trial, 17 patients with R/R MM were administered single-agent everolimus; the ORR was 59% including eight patients with stable disease (SD), one with partial response (PR), one with minor remission (MR) (96). In another trial, 26 patients were administered everolimus and lenalidomide for 21 days with a 7 days break between cycles. The ORR was 65%, the median PFS was 5.5 months and median overall survival (OS) was 29.5 months (97). Temsirolimus has also been combined with lenalidomide in a phase I study in patients with relapsed MM. In total, 21 patients were enrolled, two patients (10%) achieved PR, and 15 patients (71%) had SD (98). In another phase I/II study evaluated temsirolimus in combination with weekly bortezomib in R/R MM patients, 14 of 43 (33%) patients achieved ORR (99). There are currently multiple approved drugs to treat multiple myeloma in front-line therapy; however, there is still no cure for this serious disease. Thus, more studies are needed to evaluate the potential benefit of incorporating mTORi into late-line regimens in R/R MM patients.
Applications in Non-Malignant Hemopathies
Immune Thrombocytopenic Purpura
The pathogenesis of immune thrombocytopenic purpura (ITP) includes abnormal activation of autoreactive T and B cells, which leads to immune destruction of platelets, providing a theoretical basis for the application of rapamycin in ITP. Wang CY et al. (100) found that platelet autophagy was diminished in ITP patients, and platelet autophagy in ITP was regulated by the PI3K/Akt/mTOR pathway. Rapamycin induced autophagy and alleviated the destruction of ITP platelets (100). Rapamycin and prednisone were used to treat adult chronic ITP (101). After comparing the remission rates of rapamycin group and cyclosporine group, as well as the levels of Treg cells, IL-10, and TGF - β cytokines in responders, it was concluded that rapamycin combined with low-dose dexamethasone could not improve the remission rate, but the sustained remission time of those treated with Rapamycin was significantly longer than that of cyclosporine-treated patients, and the Rapa-responders’ Treg cell level and Foxp3 mRNA expression level were significantly higher than those treated with cyclosporine.
Bride et al. (102) conducted a multicenter prospective study (NCT00392951) on rapamycin as a single therapy for refractory autoimmune pancytopenia of 30 patients. The result showed that rapamycin had a significant effect on secondary multicellular hemocytopenia, such as secondary Hypogammaglobulinemia, Evans Syndrome (ES), and SLE (systemic lupus erythematosus), especially thrombocytopenia, with a CR rate of 67% (8/12). In this report, all children (12) with autoimmune lymphoproliferative syndrome (ALPS) achieved a durable CR, including rapid improvement in autoimmune disease, lymphadenopathy, and splenomegaly within 1–3 months of starting sirolimus. Double-negative T cells were no longer detectable in most, suggesting a targeted effect of sirolimus. Jasinski et al. (103) treated 17 patients with sirolimus, among which were 12 ITP and 5 ES cases. As a result, 73% of ITP patients achieved a CR by 3 months, while 50% of ES patients had a CR. Of the patients that achieved CR, 90% remained off all therapy for a median of 2 years.
Miano et al. (104) retrospectively analyzed primary ITP (10 cases) and ITP secondary to autoimmune lymphoproliferative syndrome (nine cases) who received sirolimus treatment, and had previously failed mycophenolate mofetil (MMF) therapy. As a result, 5/10 primary ITP patients (50%) and 8/9 (89%) secondary ITP patients responded to SRL respectively. Our center initialed a prospective, single-arm clinical trial, in which 86 patients were included and given SRL administration. The results showed that SRL treatment obtained an ORR of 85% at the 3rd month without serious drug toxicity. After 12 months follow-up, the ORR remained at 65%. Importantly, in patients who responded, SRL treatment was associated with a reduction in the percentage of Th2, Th17 cells, and increase in the percentage of monocytic-Myeloid-Derived Suppressor Cells (M-MDSCs) and T regulatory cells (Tregs), indicating that SRL may re-establish peripheral tolerance. To date, this is the largest prospective study to date evaluating SRL as a rescue therapy in patients with R/R ITP (105). ITP is a heterogeneous disease with a complex pathobiology; based on our experience and others’, we believe that mTORi can be effective in treating refractory ITP, but more randomized controlled trials (RCTs) are needed to support these observations.
Autoimmune Hemolytic Anemia
Sirolimus has benefited patients with primary autoimmune cytopenias, possibly by stimulating Tregs (105, 106). In the pathogenesis of AIHA, increased expansion or function of Treg cells can inhibit the over-activation of effector T cells and other immune cells, thus regulating the production of excess RBC antibodies (107, 108). Mqadmi et al. (109) used CD25 monoclonal antibody to treat C57/B16 mice to deplete CD4+CD25+ Treg cells in vivo, followed by injection allogeneic red blood cells into the abdominal cavity of C57/B16 mice to establish a murine AIHA model. These experiments showed that incidence of AIHA in Treg deficient mice increased from 30% to 90%. CD4+CD25+ Treg cells purified from the spleen of mice were re-injected into the diseased mice, leading to a reduction in hemolysis and autoantibodies. Thus, CD4+ CD25+ Treg cells play an important role in the pathogenesis of AIHA in animal models (110, 111).
Miano et al. reported that sirolimus was effective in five children with AIHA who did not respond to MMF previously. In their center, sirolimus is used as a rescue treatment, given at the dose of 2–3 mg/m2 once a day, for at least 3 month (112). In refractory AIHA after intestinal transplant, four patients were reported who had marked improvement of hemolysis, after discontinuation of calcineurin inhibitor (CNI) and initiation of sirolimus (113). It was recommended sirolimus as the second-line treatment of AIHA in the case of failure to steroid treatment in 2016 of ASH (American Society of Hematology) meeting (114). Our center used sirolimus to treat the relapsed and refractory AIHA. Sixteen (16) patients were included and followed for at least 12 months; after 12 months, SRL was still effective for 9/12 patients (56.25%), with 7/9 patients achieving CR and 2/9 achieving PR. Five (5) of these patients had been discontinued with no relapse, and the longest withdrawal time without relapse was 24 months. The side effects of SRL were mild and tolerable to the patients. In conclusion, SRL is effective for R/R AIHA in adults, with mild side effects, and should be considered as frontline therapy for treatment of the disease (115).
Autoimmune Lymphoproliferative Syndrome
Autoimmune lymphoproliferative syndrome (ALPS) is mainly caused by mutations in FAS mediated apoptosis pathway (116, 117). The pathogenesis of ALPS is that the double negative T cells (DNT), expressing CD3+TCRαβ+ CD4-CD8-, cannot be effectively removed due to apoptosis defects caused by FAS mutation, resulting in abnormal activation of immature T cell accumulation causes hepatosplenic lymphadenopathy. At the same time, over-activation of B lymphocyte leads to the increase of autoantibody production and the occurrence of autoimmunity (118). mTOR signaling activity was also enhanced in DNT cells, and mTOR inhibitors could specifically reduce DNT in vivo, indicating that the mTOR pathway is the main regulatory mechanism of lymphocyte proliferation and abnormal differentiation of ALPS (119). In 2006, the results of an experimental study in animals provided the first evidence that SRL can significantly improve the clinical symptoms of ALPS rats (120). Sirolimus has been described as useful in ALPS patients, in which it also reduced the count of DNTs (121).
Currently, there are some cases of successful application of sirolimus to control ALPS in adults and children (102, 122, 123). In one case-study, a 9-month-old female infant diagnosed ALPS with massive lymphadenopathy was treated with corticosteroids and splenectomy, but the curative effect was poor. Treatment with SRL (3 mg/m2) was initiated and the symptoms of lymphadenopathy were relieved rapidly. After 6 weeks, lymphadenopathy decreased and blood cell count improved significantly (124). In another center, four steroid-resistant ALPS patients were treated with SRL for 4 weeks; all had a rapid complete or near complete response (123). SRL was used as single agent in 12 ALPS patients for at least 6 months. The results showed that SRL was effective in all ALS patients. It not only improved the decreased blood cells, but also improved the hyperplasia of lymphoid tissue (102). In a report, 16 ALPS patients were treated with SRL as the second or further line, after the multi-drug treatment failed. As a result, 12 (75%) patients responded effectively (125). Recently, IL-6 stimulation augmented mTOR activation in idiopathic multicentric Castleman disease (iMCD) patients was reported, furthermore, the degree of mTOR activation in iMCD was comparable to ALPS. This finding supports inhibition of mTOR activation as a novel therapeutic target for iMCD, and is currently under investigation in a clinical study (NCT03933904) (126).
Acquired Aplastic Anemia and Pure Red Cell Aplasia
Acquired aplastic anemia is characterized by a hypoplastic, fatty bone marrow with profound reductions in hematopoietic stem/progenitor cells that lead to defective mature blood cell production and peripheral pancytopenia (127). In clinical observation, it was found that the IFN-γ level in serum and bone marrow of was increased in ~30% of AA patients increased, while cytotoxic T cells (CD8+ T cells) production of IFN-γ promoted CD34+ pluripotent hematopoietic progenitor cell apoptosis (128). The attack of CD34+ cells by CD8+ T cells is the basis of AA, the effect of CD8+ T cells can be eliminated by SRL mediated CD4+CD25+ Treg cells, which inhibit the process and promote the proliferation of hematopoietic stem cells (129, 130). Rapamycin ameliorated this phenotype in an immune-mediated AA mice model and inhibited the proliferation of T cells by preventing cell cycle transition from G0 to G1 phase (131). Reports also show that rapamycin can significantly enhance autophagy of Bone Marrow Stromal Cells (BMSCs). Rapamycin can inhibit the adipogenic differentiation of BM-MSCs in AA patients in a dose-dependent manner, and the inhibition rate can reach 50%-85%. Rapamycin can reduce the ratio of adipose tissue to bone marrow hematopoietic tissue, so as to improve the hematopoietic microenvironment of bone marrow. However, SRL may also promote the apoptosis of MSC; accordingly, rapamycin should be strictly controlled in clinical treatment (132). He et al. reported two case of AA responsive to sirolimus (1 mg/d) combined with cyclosporine (2 mg/kg/d) (133). In fact, controversial results are also presented. A study observed 35 AA patients received h-ATG/CsA/sirolimus, and the ORR at 3 months was 37% and 51% at 6 months, at last, it concluded that sirolimus (2 mg/day in adults and 1 mg/m2/day in children) did not improve the response rate in patients with severe AA when compared to standard h-ATG/CsA treatment (134).
Sirolimus may not directly stimulate the growth and differentiation of red blood cells, but it can antagonize the inhibitory effect of PRCA patients’ serum on red blood cells, and may play a role by inhibiting the components in serum (135). In 2007, ASH meeting, Sirolimus (1 mg/day) successfully treated a refractory case of congenital PRCA was reported (136). Reportedly, three patients of multi-resistant PRCA treated with SRL (2 mg/day), all responded well to SRL, and natural killer cell (CD16+CD56+) count was decreased after long-term administration of sirolimus (137). In 2018, 21 patients with refractory/relapsed acquired PRCA administered with SRL, and 76.2% of the patients was responsive and 42.9% was complete response (138). At present, there is still a need for multi-center and large-scale RCT to verify the positive role of rapamycin in acquired AA and PRCA.
Graft-Versus-Host Disease
SRL has been widely used to inhibit graft rejection after solid organ transplantation; however, increasing evidence demonstrate that SRL plays an active role in the prevention and treatment of graft-versus-host disease (GVHD) following hematopoietic stem cell transplantation (HSCT). GVHD is an exaggerated inflammatory reaction mediated by donor lymphocytes against host tissues. The conditioning regimen damages host tissues and causes release of pro-inflammatory soluble factors such as TNFα, IFN-γ, IL-1, IL-6, and nitric oxide. Increased levels of these factors lead to activation of host antigen presenting cells (APCs). Host APCs migrate into lymphoid tissue and subsequently activate donor immune cells, including T, B, and NK cells. This process elicits a complex cascade of both lymphocyte subsets and soluble inflammatory mediators. Lastly, these soluble and cellular mediators synergize to amplify local tissue injury and further promote inflammation and target tissue destruction (139, 140). Coenen JJ et al. (141), reported rapamycin, not cyclosporine, permits thymic generation and peripheral preservation of CD4+CD25+FoxP3+ T cells. Treatment with cyclosporine led to a reduced generation of CD4+FoxP3+ T cells in GVHD mice model, whereas prolonged rapamycin treatment allowed for thymic generation of those cells. As peripheral tolerance induction is a prerequisite for successful treatment outcome after stem cell transplantation (SCT), this may challenge the use of cyclosporine as standard drug of choice (141).
In 2008, Armand et al. (142) found that sirolimus combined with calcineurin inhibitor prevented GVHD in lymphoma patients after bone marrow transplantation. The OS of patients in sirolimus group was significantly prolonged (3-year OS, 66% for sirolimus group vs. 38% for no sirolimus group). In 2016, Armand and his colleagues (143), again reported a multicenter randomized trial comparing sirolimus, tacrolimus and methotrexate to standard method (tacrolimus, methotrexate and mycophenolate mofetil) in lymphoma patients after SCT. As a result, there was no difference in 2-year OS, PFS, relapse, and chronic GVHD. However, the sirolimus-containing arm had a significantly lower incidence of grade II‐IV acute GVHD. In 2019, another multi-center, randomized, phase 3 trial was reported. After HSCT, standard GVHD prophylaxis regimen (cyclosporine and mycophenolate mofetil) or the triple-drug combination regimen (cyclosporine, mycophenolate mofetil, and sirolimus) was used to test the efficacy of SRL. Consistent with previous findings, the incidence of II‐IV aGVHD in the three-drug group (26%) was lower than that in the standard group (52%) at 100 days. The new discoveries were as following: The Non Recurrent Mortality (NRM) rate (16%) of the three drug groups was lower than that of the standard group (32%) (p=0.021) at 4 years; The OS rate in the three drug groups was higher than that in the standard group (p=0.035); The PFS rates in the three drug groups were higher than those in the standard group (p=0.045); The incidence of Grade III to IV aGVHD and chronic GVHD was of no differences (144). A meta-analysis included five RCTs to assess the efficacy and safety of sirolimus-based GVHD prophylaxis in patients after allogeneic HSCT, as the result, SIR was observed to significantly decrease the incidence of Grades II to IV aGVHD; However, the incidence of Grades III to IV aGVHD and chronic GVHD was not decreased. SRL significantly increased sinusoidal obstructive syndrome, Moreover, SRL did not improve event-free survival and overall survival (145).
In addition to the above reports on the prevention of GVHD by SRL, the following is the story of using sirolimus to treat GVHD. Pidala et al. (146) initiated a multicenter randomized phase II trial to assess the CR/PR rates of SRL versus prednisone to treat patients with acute GVHD of standard risk. A total of 127 patients were enrolled and randomized. The day 28 CR/PR rates were similar for sirolimus 64.8% vs. 73% for prednisone. Interestingly, the day 28 rate of CR/PR with prednisone (≤0.25 mg/kg/day) was significantly lower than sirolimus (31.7% vs. 66.7%; P<0.001). Importantly, SRL was associated with reduced steroid exposure and hyperglycemia, reduced grades 2 to 3 infections, improvement in immune suppression discontinuation and patient-reported quality of life. For chronic GVHD treatment, Carpenter PA et al. (147), claimed that the two-drug (prednisone/sirolimus) and three-drug combination (prednisone/sirolimus/CNI) did not differ in rates of 6-month CR and PR. In other words, the results are the same whether CNI is not added to the two-drug regimen. Importantly, the two-drug regimen is easier to administer and is better tolerated. In our center, sirolimus was used to treat steroid- resistant/steroid- dependent extensive cGVHD. A total of 27 patients were enrolled and given sirolimus combined with cyclosporine or tacrolimus to observe the clinical efficacy and adverse events. Following the 6-month follow-up, the ORR was 55.6%. At the 1-year follow-up, there were five cases of CR and 11 cases of PR, ORR was 59.3%, PFS-12 reached 62.9% (17/27), and OS-12 was 100% (148).
In summary, SRL has demonstrated clinical benefit in both the prevention and treatment of GVHD and may be preferred to other regimens for patients after HSCT. There is currently extensive clinical investigation of Rapalogs in the GVHD setting (Table 1).
Conclusions and Future Directions
Rapalogs, as inhibitors of mTOR, have demonstrated clinical value, not only in the regulation of abnormal immune responses found in benign hematologic diseases, but also for the treatment of hematological malignancies (Table 2). However, there are still some challenges in the application of Rapalogs. First, at present, most of the studies of Rapalogs are case reports of three-line or further line drugs, which are not widely used in large-scale populations, so there remains a lack of evidence—effectiveness and long-term safety of Rapalogs need to be further studied. Whether SRL can be used as a more advanced drug in the treatment paradigm(s) needs to be evaluated by more extensive clinical research. Second, it is necessary to improve the efficacy and safety of Rapalogs, establishing the proper dosing regiments, maintaining appropriate blood concentrations model to optimize the current treatment. Third, many cytotoxic drugs are limited in the pediatric setting. The question arises, can Rapalogs demonstrate an advantage over current therapies in convenience, safety, and efficacy on pediatric patients? Finally, in clinical studies to date, we found that there are inevitably some patients that do not responded to Rapalogs, irrespective of primary disease. Can we achieve precise treatment? Before treatment, can we accurately stratify patients which are likely responders to Rapalogs? Perhaps by detecting the activity of mTOR, specific mutations responsible of driving mTOR activity or a deeper prognostic biomarker? Additional work is required in this exciting and evolving area.
Author Contributions
YF wrote the original draft and revised the manuscript. XC, KC, ZZ, ZW, SY, and XZ edited this review. XZ and YF: funded the work. All authors contributed to the article and approved the submitted version.
Funding
This work was supported by the Science and Technology Innovation Capacity Promotion Project of Army Medical University (2019XLC3014), Natural Science Foundation of Chongqing (cstc2019jcyj-msxmX0273 and cstc2020jcyj-msxmX1086), Special Projects in the Frontier of Military Medicine Natural Science of Xinqiao Hospital (2018YQYLY002), National Key Research Program (2017YFA0105502).
Conflict of Interest
The authors declare that the research was conducted in the absence of any commercial or financial relationships that could be construed as a potential conflict of interest.
References
1. Kelchtermans J, Chang J, Glaberson W, DeFreitas M, Alba-Sandoval M, Chandar J. A Pediatric Case of Sirolimus-Associated Pneumonitis After Kidney Transplantation. J Pediatr Pharmacol Ther (2020) 25:459–64. doi: 10.5863/1551-6776-25.5.459
2. Dufour M, Dormond-Meuwly A, Demartines N, Dormond O. Targeting the Mammalian Target of Rapamycin (mTOR) in Cancer Therapy: Lessons from Past and Future Perspectives. Cancers (Basel) (2011) 3:2478–500. doi: 10.3390/cancers3022478
3. Tan FH, Bai Y, Saintigny P, Darido C. mTOR Signalling in Head and Neck Cancer: Heads Up. Cells (2019) 8:333. doi: 10.3390/cells8040333
4. Adjei AA, Hidalgo M. Intracellular signal transduction pathway proteins as targets for cancer therapy. J Clin Oncol (2005) 23:5386–403. doi: 10.1200/JCO.2005.23.648
5. Sarbassov DD, Ali SM, Kim DH, Guertin DA, Latek RR, Erdjument-Bromage H, et al. Rictor, a novel binding partner of mTOR, defines a rapamycin-insensitive and raptor-independent pathway that regulates the cytoskeleton. Curr Biol (2004) 14:1296–302. doi: 10.1016/j.cub.2004.06.054
6. Laplante M, Sabatini DM. mTOR signaling in growth control and disease. Cell (2012) 149:274–93. doi: 10.1016/j.cell.2012.03.017
7. Pearce LR, Sommer EM, Sakamoto K, Wullschleger S, Alessi DR. Protor-1 is required for efficient mTORC2-mediated activation of SGK1 in the kidney. Biochem J (2011) 436:169–79. doi: 10.1042/BJ20102103
8. Sarbassov DD, Ali SM, Sengupta S, Sheen JH, Hsu PP, Bagley AF, et al. Prolonged rapamycin treatment inhibits mTORC2 assembly and Akt/PKB. Mol Cell (2006) 22:159–68. doi: 10.1016/j.molcel.2006.03.029
9. Meng LH, Zheng XF. Toward rapamycin analog (rapalog)-based precision cancer therapy. Acta Pharmacol Sin (2015) 36:1163–9. doi: 10.1038/aps.2015.68
10. Malagelada C, Jin ZH, Jackson-Lewis V, Przedborski S, Greene LA. Rapamycin protects against neuron death in in vitro and in vivo models of Parkinson’s disease. J Neurosci (2010) 30:1166–75. doi: 10.1523/JNEUROSCI.3944-09.2010
11. Ma KL, Ruan XZ, Powis SH, Moorhead JF, Varghese Z. Anti-atherosclerotic effects of sirolimus on human vascular smooth muscle cells. Am J Physiol Heart Circ Physiol (2007) 292:H2721–8. doi: 10.1152/ajpheart.01174.2006
12. Goudar RK, Shi Q, Hjelmeland MD, Keir ST, McLendon RE, Wikstrand CJ, et al. Combination therapy of inhibitors of epidermal growth factor receptor/vascular endothelial growth factor receptor 2 (AEE788) and the mammalian target of rapamycin (RAD001) offers improved glioblastoma tumor growth inhibition. Mol Cancer Ther (2005) 4:101–12.
13. Liu Y, Zhang DT, Liu XG. mTOR Signaling in T Cell Immunity and Autoimmunity. Int Rev Immunol (2015) 34:50–66. doi: 10.3109/08830185.2014.933957
14. Cutler C, Antin JH. Sirolimus immunosuppression for graft-versus-host disease prophylaxis and therapy: an update. Curr Opin Hematol (2010) 17:500–4. doi: 10.1097/MOH.0b013e32833e5b2e
15. Chapuis N, Tamburini J, Green AS, Willems L, Bardet V, Park S, et al. Perspectives on inhibiting mTOR as a future treatment strategy for hematological malignancies. Leukemia (2010) 24:1686–99. doi: 10.1038/leu.2010.170
16. Kirchner GI, Meier-Wiedenbach I, Manns MP. Clinical pharmacokinetics of everolimus. Clin Pharmacokinet (2004) 43:83–95. doi: 10.2165/00003088-200443020-00002
17. Kovarik JM. Everolimus - A proliferation signal inhibitor targeting primary causes of allograft dysfunction. Drugs Today (2004) 40:101–9. doi: 10.1358/dot.2004.40.2.799422
18. Sessa C, Tosi D, Vigano L, Albanell J, Hess D, Maur M, et al. Phase Ib study of weekly mammalian target of rapamycin inhibitor ridaforolimus (AP23573; MK-8669) with weekly paclitaxel. Ann Oncol (2010) 21:1315–22. doi: 10.1093/annonc/mdp504
19. Chawla SP, Staddon AP, Baker LH, Schuetze SM, Tolcher AW, D’Amato GZ, et al. Phase II study of the mammalian target of rapamycin inhibitor ridaforolimus in patients with advanced bone and soft tissue sarcomas. J Clin Oncol (2012) 30:78–84. doi: 10.1200/JCO.2011.35.6329
20. Seiler M, Ray-Coquard I, Melichar B, Yardley DA, Wang RX, Dodion PF, et al. Oral ridaforolimus plus trastuzumab for patients with HER2+ trastuzumab-refractory metastatic breast cancer. Clin Breast Cancer (2015) 15:60–5. doi: 10.1016/j.clbc.2014.07.008
21. Rizzieri DA, Feldman E, Dipersio JF, Gabrail N, Stock W, Strair R, et al. A phase 2 clinical trial of deforolimus (AP23573, MK-8669), a novel mammalian target of rapamycin inhibitor, in patients with relapsed or refractory hematologic malignancies. Clin Cancer Res (2008) 14:2756–62. doi: 10.1158/1078-0432.CCR-07-1372
22. Serruys PW, Silber S, Garg S, van Geuns RJ, Richardt G, Buszman PE, et al. Comparison of zotarolimus-eluting and everolimus-eluting coronary stents. N Engl J Med (2010) 363:136–46. doi: 10.1056/NEJMoa1004130
23. Chan S, Scheulen ME, Johnston S, Mross K, Cardoso F, Dittrich C, et al. Phase II study of temsirolimus (CCI-779), a novel inhibitor of mTOR, in heavily pretreated patients with locally advanced or metastatic breast cancer. J Clin Oncol (2005) 23:5314–22. doi: 10.1200/JCO.2005.66.130
24. Witzens-Harig M, Memmer ML, Dreyling M, Hess G. A phase I/II trial to evaluate the safety, feasibility and activity of salvage therapy consisting of the mTOR inhibitor Temsirolimus added to standard therapy of Rituximab and DHAP for the treatment of patients with relapsed or refractory diffuse large cell B-Cell lymphoma - the STORM trial. BMC Cancer (2013) 13:308. doi: 10.1186/1471-2407-13-308
25. Pandya KJ, Dahlberg S, Hidalgo M, Cohen RB, Lee MW, Schiller JH, et al. A randomized, phase II trial of two dose levels of temsirolimus (CCI-779) in patients with extensive-stage small-cell lung cancer who have responding or stable disease after induction chemotherapy: a trial of the Eastern Cooperative Oncology Group (E1500). J Thorac Oncol (2007) 2:1036–41. doi: 10.1097/JTO.0b013e318155a439
26. Dancey JE, Curiel R, Purvis J. Evaluating temsirolimus activity in multiple tumors: a review of clinical trials. Semin Oncol (2009) 36 Suppl 3:S46–58. doi: 10.1053/j.seminoncol.2009.10.010
27. Feng YH, Wu LS. mTOR up-regulation of PFKFB3 is essential for acute myeloid leukemia cell survival. Biochem Biophys Res Commun (2017) 483:897–903. doi: 10.1016/j.bbrc.2017.01.031
28. Xu Q, Simpson SE, Scialla TJ, Bagg A, Carroll M. Survival of acute myeloid leukemia cells requires PI3 kinase activation. Blood (2003) 102:972–80. doi: 10.1182/blood-2002-11-3429
29. Xu Q, Thompson JE, Carroll M. mTOR regulates cell survival after etoposide treatment in primary AML cells. Blood (2005) 106:4261–8. doi: 10.1182/blood-2004-11-4468
30. Brown VI, Fang JJ, Alcorn K, Barr R, Kim JM, Wasserman R, et al. Rapamycin is active against B-precursor leukemia in vitro and in vivo, an effect that is modulated by Il-7-mediated signalling. Proc Natl Acad Sci U S A (2003) 100:15113–8. doi: 10.1073/pnas.2436348100
31. Avellino R, Romano S, Parasole R, Bisogni R, Lamberti A, Poggi V, et al. Rapamycin stimulates apoptosis of childhood acute lymphoblastic leukemia cells. Blood (2005) 106:1400–6. doi: 10.1182/blood-2005-03-0929
32. Daver N, Boumber Y, Kantarjian H, Ravandi F, Cortes J, Rytting ME, et al. A Phase I/II Study of the mTOR Inhibitor Everolimus in Combination with HyperCVAD Chemotherapy in Patients with Relapsed/Refractory Acute Lymphoblastic Leukemia. Clin Cancer Res (2015) 21:2704–14. doi: 10.1158/1078-0432.CCR-14-2888
33. Recher C, Beyne-Rauzy O, Demur C, Chicanne G, Dos Santos C, Mansat-De Mas V, et al. Antileukemic activity of rapamycin in acute myeloid leukemia. Blood (2005) 105:2527–34. doi: 10.1182/blood-2004-06-2494
34. Yee KWL, Zeng ZH, Konopleva M, Verstovsek S, Ravandi F, Ferrajoli A, et al. Phase I/II study of the mammalian target of rapamycin inhibitor everolimus (RAD001) in patients with relapsed or refractory hematologic malignancies. Clin Cancer Res (2006) 12:5165–73. doi: 10.1158/1078-0432.CCR-06-0764
35. Litzow MR, Wang XV, Carroll MP, Karp JE, Ketterling RP, Zhang YM, et al. A randomized trial of three novel regimens for recurrent acute myeloid leukemia demonstrates the continuing challenge of treating this difficult disease. Am J Hematol (2019) 94:111–7. doi: 10.1002/ajh.25333
36. Kasner MT, Mick R, Jeschke GR, Carabasi M, Filicko-O’Hara J, Flomenberg N, et al. Sirolimus enhances remission induction in patients with high risk acute myeloid leukemia and mTORC1 target inhibition. Invest New Drugs (2018) 36:657–66. doi: 10.1007/s10637-018-0585-x
37. Perl AE, Kasner MT, Tsai DE, Vogl DT, Loren AW, Schuster SJ, et al. A Phase I Study of the Mammalian Target of Rapamycin Inhibitor Sirolimus and MEC Chemotherapy in Relapsed and Refractory Acute Myelogenous Leukemia. Clin Cancer Res (2009) 15:6732–9. doi: 10.1158/1078-0432.CCR-09-0842
38. Burnett AK, Das Gupta E, Knapper S, Khwaja A, Sweeney M, Kjeldsen L, et al. Addition of the mammalian target of rapamycin inhibitor, everolimus, to consolidation therapy in acute myeloid leukemia: experience from the UK NCRI AML17 trial. Haematologica (2018) 103:1654–61. doi: 10.3324/haematol.2018.189514
39. Robin M, Fenaux P. Which lower risk myelodysplastic syndromes should be treated with allogeneic hematopoietic stem cell transplantation? Leukemia (2020) 34(10):2552–60. doi: 10.1038/s41375-020-0967-x
40. Yip BH, Vuppusetty C, Attwood M, Giagounidis A, Germing U, Lamikanra AA, et al. Activation of the mTOR signaling pathway by L-leucine in 5q-syndrome and other RPS14-deficient erythroblasts. Leukemia (2013) 27:1760–3. doi: 10.1038/leu.2013.20
41. Follo MY, Mongiorgi S, Bosi C, Cappenini A, Finelli C, Chiarini F, et al. The Akt/mammalian target of rapamycin signal transduction pathway is activated in high-risk myelodysplastic syndromes and influences cell survival and proliferation. Cancer Res (2007) 67:4287–94. doi: 10.1158/0008-5472.CAN-06-4409
42. Maeda Y, Yamaguchi T, Ueda S, Matsuo K, Morita Y, Naiki Y, et al. Mutant type glutathione S-transferase theta 1 gene homologue to mTOR in myelodysplastic syndrome: Possible clinical application of rapamycin. Leuk Lymphoma (2003) 44:1179–85. doi: 10.1080/1042819031000077052
43. Platzbecker U, Haase M, Herbst R, Hanel A, Voigtmann K, Thiede CH, et al. Activity of sirolimus in patients with myelodysplastic syndrome–results of a pilot study. Br J Haematol (2005) 128:625–30. doi: 10.1111/j.1365-2141.2005.05360.x
44. Wermke M, Schuster C, Nolte F, Al-Ali HK, Kiewe P, Schonefeldt C, et al. Mammalian-target of rapamycin inhibition with temsirolimus in myelodysplastic syndromes (MDS) patients is associated with considerable toxicity: results of the temsirolimus pilot trial by the German MDS Study Group (D-MDS). Br J Haematol (2016) 175:917–24. doi: 10.1111/bjh.14345
45. Annunziata M, Bonifacio M, Breccia M, Castagnetti F, Gozzini A, Iurlo A, et al. Current Strategies and Future Directions to Achieve Deep Molecular Response and Treatment-Free Remission in Chronic Myeloid Leukemia. Front Oncol (2020) 10:883. doi: 10.3389/fonc.2020.00883
46. Mayerhofer M, Valent P, Sperr WR, Griffin JD, Sillaber C. BCR/ABL induces expression of vascular endothelial growth factor and its transcriptional activator, hypoxia inducible factor-1alpha, through a pathway involving phosphoinositide 3-kinase and the mammalian target of rapamycin. Blood (2002) 100:3767–75. doi: 10.1182/blood-2002-01-0109
47. Ly C, Arechiga AF, Melo JV, Walsh CM, Ong ST. Bcr-Abl kinase modulates the translation regulators ribosomal protein S6 and 4E-BP1 in chronic myelogenous leukemia cells via the mammalian target of rapamycin. Cancer Res (2003) 63:5716–22.
48. Carayol N, Vakana E, Sassano A, Kaur S, Goussetis DJ, Glaser H, et al. Critical roles for mTORC2- and rapamycin-insensitive mTORC1-complexes in growth and survival of BCR-ABL-expressing leukemic cells. Proc Natl Acad Sci U S A (2010) 107:12469–74. doi: 10.1073/pnas.1005114107
49. Li J, Xue L, Hao H, Han Y, Yang J, Luo J. Rapamycin provides a therapeutic option through inhibition of mTOR signaling in chronic myelogenous leukemia. Oncol Rep (2012) 27:461–6. doi: 10.3892/or.2011.1502
50. Li J, Xue L, Hao H, Li R, Luo J. Rapamycin combined with celecoxib enhanced antitumor effects of mono treatment on chronic myelogenous leukemia cells through downregulating mTOR pathway. Tumour Biol (2014) 35:6467–74. doi: 10.1007/s13277-014-1820-5
51. Mohi MG, Boulton C, Gu TL, Sternberg DW, Neuberg D, Griffin JD, et al. Combination of rapamycin and protein tyrosine kinase (PTK) inhibitors for the treatment of leukemias caused by oncogenic PTKs. Proc Natl Acad Sci U S A (2004) 101:3130–5. doi: 10.1073/pnas.0400063101
52. Alves R, Goncalves AC, Jorge J, Alves J, Alves da Silva A, Freitas-Tavares P, et al. Everolimus in combination with Imatinib overcomes resistance in Chronic myeloid leukaemia. Med Oncol (2019) 36:30. doi: 10.1007/s12032-019-1253-5
53. Palumbo GA, Stella S, Pennisi MS, Pirosa C, Fermo E, Fabris S, et al. The Role of New Technologies in Myeloproliferative Neoplasms. Front Oncol (2019) 9:321. doi: 10.3389/fonc.2019.00321
54. Bartalucci N, Tozzi L, Bogani C, Martinelli S, Rotunno G, Villeval JL, et al. Co-targeting the PI3K/mTOR and JAK2 signalling pathways produces synergistic activity against myeloproliferative neoplasms. J Cell Mol Med (2013) 17:1385–96. doi: 10.1111/jcmm.12162
55. Guglielmelli P, Barosi G, Rambaldi A, Marchioli R, Masciulli A, Tozzi L, et al. Safety and efficacy of everolimus, a mTOR inhibitor, as single agent in a phase 1/2 study in patients with myelofibrosis. Blood (2011) 118:2069–76. doi: 10.1182/blood-2011-01-330563
56. Bogani C, Bartalucci N, Martinelli S, Tozzi L, Guglielmelli P, Bosi A, et al. mTOR inhibitors alone and in combination with JAK2 inhibitors effectively inhibit cells of myeloproliferative neoplasms. PLoS One (2013) 8:e54826. doi: 10.1371/journal.pone.0054826
57. Vannucchi AM, Bogani C, Bartalucci N, Tozzi L, Martinelli S, Guglielmelli P, et al. Inhibitors of PI3K/Akt and/or mTOR Inhibit the Growth of Cells of Myeloproliferative Neoplasms and Synergize with JAK2 Inhibitor and Interferon. Blood (2011) 118:1638–9. doi: 10.1182/blood.V118.21.3835.3835
58. Bartalucci N, Guglielmelli P, Vannucchi AM. Rationale for targeting the PI3K/Akt/mTOR pathway in myeloproliferative neoplasms. Clin Lymphoma Myeloma Leuk (2013) 13 Suppl 2:S307–309. doi: 10.1016/j.clml.2013.07.011
59. Dutton A, Reynolds GM, Dawson CW, Young LS, Murray PG. Constitutive activation of phosphatidyl-inositide 3 kinase contributes to the survival of Hodgkin’s lymphoma cells through a mechanism involving Akt kinase and mTOR. J Pathol (2005) 205:498–506. doi: 10.1002/path.1725
60. Mark A, Hajdu M, Varadi Z, Sticz TB, Nagy N, Csomor J, et al. Characteristic mTOR activity in Hodgkin-lymphomas offers a potential therapeutic target in high risk disease–a combined tissue microarray, in vitro and in vivo study. BMC Cancer (2013) 13:250. doi: 10.1186/1471-2407-13-250
61. Uddin S, Hussain AR, Siraj AK, Manogaran PS, Al-Jomah NA, Moorji A, et al. Role of phosphatidylinositol 3’-kinase/AKT pathway in diffuse large B-cell lymphoma survival. Blood (2006) 108:4178–86. doi: 10.1182/blood-2006-04-016907
62. Hasselblom S, Hansson U, Olsson M, Toren L, Bergstrom A, Nilsson-Ehle H, et al. High immunohistochemical expression of p-AKT predicts inferior survival in patients with diffuse large B-cell lymphoma treated with immunochemotherapy. Br J Haematol (2010) 149:560–8. doi: 10.1111/j.1365-2141.2010.08123.x
63. Zhang J, Grubor V, Love CL, Banerjee A, Richards KL, Mieczkowski PA, et al. Genetic heterogeneity of diffuse large B-cell lymphoma. Proc Natl Acad Sci U S A (2013) 110:1398–403. doi: 10.1073/pnas.1205299110
64. Ezell SA, Wang S, Bihani T, Lai Z, Grosskurth SE, Tepsuporn S, et al. Differential regulation of mTOR signaling determines sensitivity to AKT inhibition in diffuse large B cell lymphoma. Oncotarget (2016) 7:9163–74. doi: 10.18632/oncotarget.7036
65. Dal Col J, Zancai P, Terrin L, Guidoboni M, Ponzoni M, Pavan A, et al. Distinct functional significance of Akt and mTOR constitutive activation in mantle cell lymphoma. Blood (2008) 111:5142–51. doi: 10.1182/blood-2007-07-103481
66. Calimeri T, Ferreri AJM. m-TOR inhibitors and their potential role in haematological malignancies. Br J Haematol (2017) 177:684–702. doi: 10.1111/bjh.14529
67. Hoppe RT, Advani RH, Ai WZ, Ambinder RF, Aoun P, Bello CM, et al. Hodgkin Lymphoma Version 1.2017, NCCN Clinical Practice Guidelines in Oncology. J Natl Compr Canc Netw (2017) 15:608–38. doi: 10.6004/jnccn.2017.0064
68. Johnston PB, Pinter-Brown L, Rogerio J, Warsi G, Chau Q, Ramchandren R. Everolimus for Relapsed/Refractory Classical Hodgkin Lymphoma: Multicenter, Open-Label, Single-Arm, Phase 2 Study. Blood (2012) 120:2740. doi: 10.1182/blood.V120.21.2740.2740
69. Johnston PB, Inwards DJ, Colgan JP, Laplant BR, Kabat BF, Habermann TM, et al. A Phase II trial of the oral mTOR inhibitor everolimus in relapsed Hodgkin lymphoma. Am J Hematol (2010) 85:320–4. doi: 10.1002/ajh.21664
70. Rocha T, Fortier SC, Fischer T, Perini GF, Gaiolla RD, Fogliatto L, et al. Everolimus as a single agent in refractory or relapsed Hodgkin’s lymphoma: the Brazilian Named Patient Program Experience. Rev Bras Hematol Hemoter (2017) 39:216–22. doi: 10.1016/j.bjhh.2017.03.008
71. Johnston PB, Pinter-Brown LC, Warsi G, White K, Ramchandren R. Phase 2 study of everolimus for relapsed or refractory classical Hodgkin lymphoma. Exp Hematol Oncol (2018) 7:12. doi: 10.1186/s40164-018-0103-z
72. Witzig TE, Reeder CB, LaPlant BR, Gupta M, Johnston PB, Micallef IN, et al. A phase II trial of the oral mTOR inhibitor everolimus in relapsed aggressive lymphoma. Leukemia (2011) 25:341–7. doi: 10.1038/leu.2010.226
73. Bennani NN, LaPlant BR, Ansell SM, Habermann TM, Inwards DJ, Micallef IN, et al. Efficacy of the oral mTORC1 inhibitor everolimus in relapsed or refractory indolent lymphoma. Am J Hematol (2017) 92:448–53. doi: 10.1002/ajh.24671
74. Renner C, Zinzani PL, Gressin R, Klingbiel D, Dietrich PY, Hitz F, et al. A multicenter phase II trial (SAKK 36/06) of single-agent everolimus (RAD001) in patients with relapsed or refractory mantle cell lymphoma. Haematologica (2012) 97:1085–91. doi: 10.3324/haematol.2011.053173
75. Conconi A, Raderer M, Franceschetti S, Devizzi L, Ferreri AJ, Magagnoli M, et al. Clinical activity of everolimus in relapsed/refractory marginal zone B-cell lymphomas: results of a phase II study of the International Extranodal Lymphoma Study Group. Br J Haematol (2014) 166:69–76. doi: 10.1111/bjh.12845
76. Padrnos L, Ernst B, Dueck AC, Kosiorek HE, Ginos BF, Toro A, et al. A Novel Combination of the mTORC1 Inhibitor Everolimus and the Immunomodulatory Drug Lenalidomide Produces Durable Responses in Patients With Heavily Pretreated Relapsed Lymphoma. Clin Lymphoma Myeloma Leuk (2018) 18:664–72.e662. doi: 10.1016/j.clml.2018.06.013
77. Janku F, Oki Y, Falchook GS, Subbiah V, Naing A, Bravo VMV, et al. Activity of the mTOR inhibitor sirolimus and HDAC inhibitor vorinostat in heavily pretreated refractory Hodgkin lymphoma patients. J Clin Oncol (2014) 32:8508. doi: 10.1200/jco.2014.32.15_suppl.8508
78. Janku F, Garrido-Laguna I, Velez-Bravo V, Falchook GS, Subbiah V, Hong DS, et al. Significant Activity Of The mTOR Inhibitor Sirolimus and HDAC Inhibitor Vorinostat In Heavily Pretreated Refractory Hodgkin Lymphoma Patients. Blood (2013) 122:3048. doi: 10.1182/blood.V122.21.3048.3048
79. Witzig TE, Geyer SM, Ghobrial I, Inwards DJ, Fonseca R, Kurtin P, et al. Phase II trial of single-agent temsirolimus (CCI-779) for relapsed mantle cell lymphoma. J Clin Oncol (2005) 23:5347–56. doi: 10.1200/JCO.2005.13.466
80. Ansell SM, Inwards DJ, Rowland KM Jr., Flynn PJ, Morton RF, Moore DF Jr., et al. Low-dose, single-agent temsirolimus for relapsed mantle cell lymphoma: a phase 2 trial in the North Central Cancer Treatment Group. Cancer (2008) 113:508–14. doi: 10.1002/cncr.23580
81. Tessoulin B, Bouabdallah K, Burroni B, Lamy T, Gressin R, Cartron G, et al. Safety and efficacy of temsirolimus in combination with three different immuno-chemotherapy regimens in relapse and refractory mantle cell lymphoma, final results of the T(3) phase IB trial of the LYSA. Ann Hematol (2020) 99:1771–8. doi: 10.1007/s00277-020-04159-3
82. Fenske TS, Shah NM, Kim KM, Saha S, Zhang C, Baim AE, et al. A phase 2 study of weekly temsirolimus and bortezomib for relapsed or refractory B-cell non-Hodgkin lymphoma: A Wisconsin Oncology Network study. Cancer (2015) 121:3465–71. doi: 10.1002/cncr.29502
83. Smith SM, van Besien K, Karrison T, Dancey J, McLaughlin P, Younes A, et al. Temsirolimus has activity in non-mantle cell non-Hodgkin’s lymphoma subtypes: The University of Chicago phase II consortium. J Clin Oncol (2010) 28:4740–6. doi: 10.1200/JCO.2010.29.2813
84. Sun SY, Rosenberg LM, Wang X, Zhou Z, Yue P, Fu H, et al. Activation of Akt and eIF4E survival pathways by rapamycin-mediated mammalian target of rapamycin inhibition. Cancer Res (2005) 65:7052–8. doi: 10.1158/0008-5472.CAN-05-0917
85. Decker T, Hipp S, Ringshausen I, Bogner C, Oelsner M, Schneller F, et al. Rapamycin-induced G(1) arrest in cycling B-CLL cells is associated with reduced expression of cyclin D3, cyclin E, cyclin A, and survivin. Blood (2003) 101:278–85. doi: 10.1182/blood-2002-01-0189
86. Panwalkar A, Verstovsek S, Giles FJ. Mammalian target of rapamycin inhibition as therapy for hematologic malignancies. Cancer (2004) 100:657–66. doi: 10.1002/cncr.20026
87. Ghobrial IM, Gertz M, Laplant B, Camoriano J, Hayman S, Lacy M, et al. Phase II trial of the oral mammalian target of rapamycin inhibitor everolimus in relapsed or refractory Waldenstrom macroglobulinemia. J Clin Oncol (2010) 28:1408–14. doi: 10.1200/JCO.2009.24.0994
88. Wang F, Zhang W, Guo L, Bao W, Jin N, Liu R, et al. Gambogic acid suppresses hypoxia-induced hypoxia-inducible factor-1alpha/vascular endothelial growth factor expression via inhibiting phosphatidylinositol 3-kinase/Akt/mammalian target protein of rapamycin pathway in multiple myeloma cells. Cancer Sci (2014) 105:1063–70. doi: 10.1111/cas.12458
89. Adachi M, Hoshino Y, Izumi Y, Sakai H, Takagi S. Effects of inhibitors of vascular endothelial growth factor receptor 2 and downstream pathways of receptor tyrosine kinases involving phosphatidylinositol 3-kinase/Akt/mammalian target of rapamycin or mitogen-activated protein kinase in canine hemangiosarcoma cell lines. Can J Vet Res (2016) 80:209–16.
90. Shi Y, Gera J, Hu L, Hsu JH, Bookstein R, Li W, et al. Enhanced sensitivity of multiple myeloma cells containing PTEN mutations to CCI-779. Cancer Res (2002) 62:5027–34.
91. Chen JQ, Ying YL, Zhu HJ, Zhu TJ, Qu CS, Jiang JH, et al. Curcumin-induced promoter hypermethylation of the mammalian target of rapamycin gene in multiple myeloma cells. Oncol Lett (2019) 17:1108–14. doi: 10.3892/ol.2018.9662
92. Lamanuzzi A, Saltarella I, Desantis V, Frassanito MA, Leone P, Racanelli V, et al. Inhibition of mTOR complex 2 restrains tumor angiogenesis in multiple myeloma. Oncotarget (2018) 9:20563–77. doi: 10.18632/oncotarget.25003
93. Jin HG, Wu GZ, Wu GH, Bao YG. Combining the mammalian target of rapamycin inhibitor, rapamycin, with resveratrol has a synergistic effect in multiple myeloma. Oncol Lett (2018) 15:6257–64. doi: 10.3892/ol.2018.8178
94. Li J, Liu ZY, Li YQ, Jing Q, Wang HL, Liu H, et al. Everolimus shows synergistic antimyeloma effects with bortezomib via the AKT/mTOR pathway. J Investig Med (2019) 67:39-47 doi: 10.1136/jim-2018-000780
95. Li J, Liu ZY, Li YQ, Jing Q, Wang HL, Liu H, et al. Everolimus shows synergistic antimyeloma effects with bortezomib via the AKT/mTOR pathway. J Invest Med (2019) 67:39–47. doi: 10.1136/jim-2018-000780
96. Gunther A, Baumann P, Burger R, Kellner C, Klapper W, Schmidmaier R, et al. Activity of everolimus (RAD001) in relapsed and/or refractory multiple myeloma: a phase I study. Haematologica (2015) 100:541–7. doi: 10.3324/haematol.2014.116269
97. Yee AJ, Hari P, Marcheselli R, Mahindra AK, Cirstea DD, Scullen TA, et al. Outcomes in patients with relapsed or refractory multiple myeloma in a phase I study of everolimus in combination with lenalidomide. Br J Haematol (2014) 166:401–9. doi: 10.1111/bjh.12909
98. Hofmeister CC, Yang X, Pichiorri F, Chen P, Rozewski DM, Johnson AJ, et al. Phase I trial of lenalidomide and CCI-779 in patients with relapsed multiple myeloma: evidence for lenalidomide-CCI-779 interaction via P-glycoprotein. J Clin Oncol (2011) 29:3427–34. doi: 10.1200/JCO.2010.32.4962
99. Ghobrial IM, Weller E, Vij R, Munshi NC, Banwait R, Bagshaw M, et al. Weekly bortezomib in combination with temsirolimus in relapsed or relapsed and refractory multiple myeloma: a multicentre, phase 1/2, open-label, dose-escalation study. Lancet Oncol (2011) 12:263–72. doi: 10.1016/S1470-2045(11)70028-6
100. Wang CY, Ma S, Bi SJ, Su L, Huang SY, Miao JY, et al. Enhancing autophagy protects platelets in immune thrombocytopenia patients. Ann Transl Med (2019) 7:134. doi: 10.21037/atm.2019.03.04
101. Li J, Wang Z, Dai L, Cao L, Su J, Zhu M, et al. Effects of rapamycin combined with low dose prednisone in patients with chronic immune thrombocytopenia. Clin Dev Immunol (2013) 2013:548085. doi: 10.1155/2013/548085
102. Bride KL, Vincent T, Smith-Whitley K, Lambert MP, Bleesing JJ, Seif AE, et al. Sirolimus is effective in relapsed/refractory autoimmune cytopenias: results of a prospective multi-institutional trial. Blood (2016) 127:17–28. doi: 10.1182/blood-2015-07-657981
103. Jasinski S, Weinblatt ME, Glasser CL. Sirolimus as an Effective Agent in the Treatment of Immune Thrombocytopenia (ITP) and Evans Syndrome (ES): A Single Institution’s Experience. J Pediatr Hematol Oncol (2017) 39:420–4. doi: 10.1097/MPH.0000000000000818
104. Miano M, Rotulo GA, Palmisani E, Giaimo M, Fioredda F, Pierri F, et al. Sirolimus as a rescue therapy in children with immune thrombocytopenia refractory to mycophenolate mofetil. Am J Hematol (2018) 93:E175–7. doi: 10.1002/ajh.25119
105. Feng YM, Xiao YS, Yan HJ, Wang P, Zhu W, Cassady K, et al. Sirolimus as Rescue Therapy for Refractory/Relapsed Immune Thrombocytopenia: Results of a Single-Center, Prospective, Single-Arm Study. Front Med (2020) 7:110. doi: 10.3389/fmed.2020.00110
106. Neely J, von Scheven E. Autoimmune haemolytic anaemia and autoimmune thrombocytopenia in childhood-onset systemic lupus erythematosus: updates on pathogenesis and treatment. Curr Opin Rheumatol (2018) 30:498–505. doi: 10.1097/BOR.0000000000000523
107. Park JA, Lee HH, Kwon HS, Baik CR, Song SA, Lee JN. Sirolimus for Refractory Autoimmune Hemolytic Anemia after Allogeneic Hematopoietic Stem Cell Transplantation: A Case Report and Literature Review of the Treatment of Post-Transplant Autoimmune Hemolytic Anemia. Transfus Med Rev (2016) 30:6–14. doi: 10.1016/j.tmrv.2015.09.001
108. Salama A. Treatment Options for Primary Autoimmune Hemolytic Anemia: A Short Comprehensive Review. Transfus Med Hemother (2015) 42:294–301. doi: 10.1159/000438731
109. Mqadmi A, Zheng XY, Yazdanbakhsh K. CD4(+) CD25(+) regulatory T cells control induction of autoimmune hemolytic anemia. Blood (2005) 105:3746–8. doi: 10.1182/blood-2004-12-4692
110. Richards AL, Kapp LM, Wang X, Howie HL, Hudson KE. Regulatory T Cells Are Dispensable for Tolerance to RBC Antigens. Front Immunol (2016) 7:348. doi: 10.3389/fimmu.2016.00348
111. Kamesaki T. Molecular mechanisms of autoimmune hemolytic anemia. Rinsho Ketsueki (2015) 56:846–54. doi: 10.11406/rinketsu.56.846
112. Miano M. How I manage Evans Syndrome and AIHA cases in children. Br J Haematol (2016) 172:524–34. doi: 10.1111/bjh.13866
113. Acquazzino MA, Fischer RT, Langnas A, Coulter DW. Refractory autoimmune hemolytic anemia after intestinal transplant responding to conversion from a calcineurin to mTOR inhibitor. Pediatr Transplant (2013) 17:466–71. doi: 10.1111/petr.12101
114. Kalfa TA. Warm antibody autoimmune hemolytic anemia. Hematol Am Soc Hematol Educ Program (2016) 2016:690–7. doi: 10.1182/asheducation-2016.1.690
115. Yan H, Wang P, Quan Y, Gao L, Liu Y, Zhang C, et al. Clinical efficacy of sirolimus in treatment of relapsed and refractory autoimmune hemolytic anemia in adults. J Third Mil Med Univ (2020) 42:1757–62. doi: 10.16016/j.1000-5404.202005102
116. Li P, Huang P, Yang Y, Hao M, Peng H, Li F. Updated Understanding of Autoimmune Lymphoproliferative Syndrome (ALPS). Clin Rev Allergy Immunol (2016) 50:55–63. doi: 10.1007/s12016-015-8466-y
117. Cooling LL, Sherbeck J, Mowers JC, Hugan SL. Development of red blood cell autoantibodies following treatment with checkpoint inhibitors: a new class of anti-neoplastic, immunotherapeutic agents associated with immune dysregulation. Immunohematology (2017) 33:15–21.
118. Gu H, Wu RH. Advance of Mechanisms and Clinical Applications about Rapamycin for Treating Immune Mediated Hemocytopenia. Zhongguo Shi Yan Xue Ye Xue Za Zhi (2018) 26:1836–40. doi: 10.7534/j.issn.1009-2137.2018.06.043
119. Volkl S, Rensing-Ehl A, Allgauer A, Schreiner E, Lorenz MR, Rohr J, et al. Hyperactive mTOR pathway promotes lymphoproliferation and abnormal differentiation in autoimmune lymphoproliferative syndrome. Blood (2016) 128:227–38. doi: 10.1182/blood-2015-11-685024
120. Teachey DT, Obzut DA, Axsom K, Choi JK, Goldsmith KC, Hall J, et al. Rapamycin improves lymphoproliferative disease in murine autoimmune lymphoproliferative syndrome (ALPS). Blood (2006) 108:1965–71. doi: 10.1182/blood-2006-01-010124
121. Teachey DT, Seif AE, Grupp SA. Advances in the management and understanding of autoimmune lymphoproliferative syndrome (ALPS). Br J Haematol (2010) 148:205–16. doi: 10.1111/j.1365-2141.2009.07991.x
122. George LA, Teachey DT. Optimal Management of Autoimmune Lymphoproliferative Syndrome in Children. Pediatr Drugs (2016) 18:261–72. doi: 10.1007/s40272-016-0175-3
123. Teachey DT, Greiner R, Seif A, Attiyeh E, Bleesing J, Choi J, et al. Treatment with sirolimus results in complete responses in patients with autoimmune lymphoproliferative syndrome. Br J Haematol (2009) 145:101–6. doi: 10.1111/j.1365-2141.2009.07595.x
124. Dragana JM, Dimitrije BC, Srda JJ, Lidija DB, Nada KR, Nada KKJ. Rapid Regression of Lymphadenopathy upon Rapamycin Treatment in a Child With Autoimmune Lymphoproliferative Syndrome. Pediatr Blood Cancer (2009) 53:1117–9. doi: 10.1002/pbc.22151
125. Miano M, Scalzone M, Perri K, Palmisani E, Caviglia I, Micalizzi C, et al. Mycophenolate mofetil and Sirolimus as second or further line treatment in children with chronic refractory Primitive or Secondary Autoimmune Cytopenias: a single centre experience. Br J Haematol (2015) 171:247–53. doi: 10.1111/bjh.13533
126. Arenas DJ, Floess K, Kobrin D, Pai RL, Srkalovic MB, Tamakloe MA, et al. Increased mTOR activation in idiopathic multicentric Castleman disease. Blood (2020) 135:1673–84. doi: 10.1182/blood.2019002792
127. Medinger M, Drexler B, Lengerke C, Passweg J. Pathogenesis of Acquired Aplastic Anemia and the Role of the Bone Marrow Microenvironment. Front Oncol (2018) 8:587. doi: 10.3389/fonc.2018.00587
128. Young NS. Current concepts in the pathophysiology and treatment of aplastic anemia. Hematol-Am Soc Hematol Educ Program (2013) 2013(1):76–81. doi: 10.1182/asheducation-2013.1.76
129. Gill H, Wong RSM, Kwong YL. From chronic immune thrombocytopenia to severe aplastic anemia: recent insights into the evolution of eltrombopag. Ther Adv Hematol (2017) 8:159–74. doi: 10.1177/2040620717693573
130. Boddu PC, Kadia TM. Updates on the pathophysiology and treatment of aplastic anemia: a comprehensive review. Expert Rev Hematol (2017) 10:433–48. doi: 10.1080/17474086.2017.1313700
131. Liu SL, Zhou YM, Tang DB, Zhou N, Zheng WW, Tang ZH, et al. Rapamycin ameliorates immune-mediated aplastic anemia by inhibiting the proliferation and metabolism of T cells. Biochem Biophys Res Commun (2019) 518:212–8. doi: 10.1016/j.bbrc.2019.08.034
132. Wang X, Ma FX, Lu SH, Chi Y, Chen F, Li X, et al. Effects of rapamycin on biological characteristics of bone marrow mesenchymal stem cells from patients with aplastic anemia. Zhongguo Shi Yan Xue Ye Xue Za Zhi (2014) 22:762–6. doi: 10.7534/j.issn.1009-2137.2014.03.035
133. He G, Zhang X, Wu D, Sun A, Wang X. Relapse of aplastic anemia responsive to sirolimus combined with cyclosporine. Pediatr Blood Cancer (2011) 56:1133–5. doi: 10.1002/pbc.22865
134. Scheinberg P, Wu CO, Nunez O, Scheinberg P, Boss C, Sloand EM, et al. Treatment of severe aplastic anemia with a combination of horse antithymocyte globulin and cyclosporine, with or without sirolimus: a prospective randomized study. Haematologica (2009) 94:348–54. doi: 10.3324/haematol.13829
135. Yang C, Chen FF, Long ZB, Du YL, Li HM, Chen M, et al. Effect of sirolimus on erythropoiesis of K562 cell line and patients with pure red cell aplasia in vitro. Zhonghua Xue Ye Xue Za Zhi (2018) 39:310–3. doi: 10.3760/cma.j.issn.0253-2727.2018.04.011
136. Ovilla R. Succesful treatment with Sirolimus in a refractory case of pure red cell aplasia (PRCA). Blood (2007) 110:11b–b. doi: 10.1182/blood.V110.11.3778.3778
137. Jiang H, Zhang H, Wang Y, Qi W, Cao Q, Xing L, et al. Sirolimus for the treatment of multi-resistant pure red cell aplasia. Br J Haematol (2019) 184:1055–8. doi: 10.1111/bjh.15245
138. Long ZB, Yu F, Du YL, Li HM, Chen M, Zhuang JL, et al. Successful treatment of refractory/relapsed acquired pure red cell aplasia with sirolimus. Ann Hematol (2018) 97:2047–54. doi: 10.1007/s00277-018-3431-5
139. Ferrara JLM, Levine JE, Reddy P, Holler E. Graft-versus-host disease. Lancet (2009) 373:1550–61. doi: 10.1016/S0140-6736(09)60237-3
140. Ghimire S, Weber D, Mavin E, Wang XN, Dickinson AM, Holler E. Pathophysiology of GvHD and Other HSCT-Related Major Complications. Front Immunol (2017) 8:79. doi: 10.3389/fimmu.2017.00079
141. Coenen JJ, Koenen HJ, van Rijssen E, Kasran A, Boon L, Hilbrands LB, et al. Rapamycin, not cyclosporine, permits thymic generation and peripheral preservation of CD4+ CD25+ FoxP3+ T cells. Bone Marrow Transplant (2007) 39:537–45. doi: 10.1038/sj.bmt.1705628
142. Armand P, Gannamaneni S, Kim HT, Cutler CS, Ho VT, Koreth J, et al. Improved survival in lymphoma patients receiving sirolimus for graft-versus-host disease prophylaxis after allogeneic hematopoietic stem-cell transplantation with reduced-intensity conditioning. J Clin Oncol (2008) 26:5767–74. doi: 10.1200/JCO.2008.17.7279
143. Armand P, Kim HT, Sainvil MM, Lange PB, Giardino AA, Bachanova V, et al. The addition of sirolimus to the graft-versus-host disease prophylaxis regimen in reduced intensity allogeneic stem cell transplantation for lymphoma: a multicentre randomized trial. Br J Haematol (2016) 173:96–104. doi: 10.1111/bjh.13931
144. Sandmaier BM, Kornblit B, Storer BE, Olesen G, Maris MB, Langston AA, et al. Addition of sirolimus to standard cyclosporine plus mycophenolate mofetil-based graft-versus-host disease prophylaxis for patients after unrelated non-myeloablative haemopoietic stem cell transplantation: a multicentre, randomised, phase 3 trial. Lancet Haematol (2019) 6:e409–18. doi: 10.1016/S2352-3026(19)30088-2
145. Wang L, Gu Z, Zhai R, Li D, Zhao S, Luo L, et al. The efficacy and safety of sirolimus-based graft-versus-host disease prophylaxis in patients undergoing allogeneic hematopoietic stem cell transplantation: a meta-analysis of randomized controlled trials. Transfusion (Paris) (2015) 55:2134–41. doi: 10.1111/trf.13110
146. Pidala J, Hamadani M, Dawson P, Martens M, Alousi AM, Jagasia M, et al. Randomized multicenter trial of sirolimus vs prednisone as initial therapy for standard-risk acute GVHD: the BMT CTN 1501 trial. Blood (2020) 135:97–107. doi: 10.1182/blood.2019003125
147. Carpenter PA, Logan BR, Lee SJ, Weisdorf DJ, Johnston L, Costa LJ, et al. A phase II/III randomized, multicenter trial of prednisone/sirolimus versus prednisone/sirolimus/calcineurin inhibitor for the treatment of chronic graft-versus-host disease: BMT CTN 0801. Haematologica (2018) 103:1915–24. doi: 10.3324/haematol.2018.195123
Keywords: rapamycin, Rapalogs, mTOR pathway, malignant and benign hemopathies, graft-versus-host disease
Citation: Feng Y, Chen X, Cassady K, Zou Z, Yang S, Wang Z and Zhang X (2021) The Role of mTOR Inhibitors in Hematologic Disease: From Bench to Bedside. Front. Oncol. 10:611690. doi: 10.3389/fonc.2020.611690
Received: 29 September 2020; Accepted: 27 November 2020;
Published: 08 January 2021.
Edited by:
Liren Qian, Sixth Medical Center of PLA General Hospital, ChinaReviewed by:
Leland Metheny, University Hospitals of Cleveland, United StatesCristian Berce, Iuliu Hațieganu University of Medicine and Pharmacy, Romania
Narendranath Epperla, The Ohio State University, United States
Copyright © 2021 Feng, Chen, Cassady, Zou, Yang, Wang and Zhang. This is an open-access article distributed under the terms of the Creative Commons Attribution License (CC BY). The use, distribution or reproduction in other forums is permitted, provided the original author(s) and the copyright owner(s) are credited and that the original publication in this journal is cited, in accordance with accepted academic practice. No use, distribution or reproduction is permitted which does not comply with these terms.
*Correspondence: Yimei Feng, eWltZWlmZW5nQDE2My5jb20=; Xi Zhang, Wmhhbmd4eGlAc2luYS5jb20=
†Present address: Kaniel Cassady, Regeneron Pharmaceuticals, Tarrytown, NY, United States