- 1Department of Thyroid Surgery, The First Affiliated Hospital of Zhengzhou University, Zhengzhou, China
- 2Department of Thyroid Surgery, Key Discipline Laboratory of Clinical Medicine of Henan, Zhengzhou, China
- 3Academy of Medical Sciences, Zhengzhou University, Zhengzhou, China
The incidence of thyroid cancer (TC) is rapidly increasing worldwide. The diagnostic accuracy and dynamics of TC need to be improved, and traditional treatments are not effective enough for patients with poorly differentiated thyroid cancer. Exosomes are membrane vesicles secreted specifically by various cells and are involved in intercellular communication. Recent studies have shown that exosomes secreted by TC cells contribute to tumor progression, angiogenesis and metastasis. Exosomes in liquid biopsies can reflect the overall molecular information of tumors, and have natural advantages in diagnosing TC. Exosomes also play an important role in tumor therapy due to their special physicochemical properties. TC patients will benefit as more exosome patterns are discovered. In this review, we discuss the role of TC-derived exosomes in tumorigenesis and development, and describe the application of exosomes in the diagnosis and treatment of TC.
Introduction
Thyroid cancer (TC), one of the fastest-growing cancers in the world, has become the most common malignancy of the endocrine system in recent years (1–3). More than 90% of TCs diagnosed annually are differentiated thyroid cancer (DTC), which histologically includes papillary thyroid cancer (PTC) and follicular thyroid cancer (FTC), whereas the remaining subset of TC comprises medullary thyroid cancer (MTC) and anaplastic thyroid cancer (ATC); however, these two subtypes are associated with a poor prognosis (4, 5). Through common treatment methods, including surgery, radioactive iodine therapy, chemotherapy, external irradiation, and targeted therapy, most cases of TC have high cure rates and low tumor recurrence (6). However, there are still not adequate treatment options for advanced TC (7, 8). The median survival time of patients with ATC is less than 6 months regardless of stage. Although aggressive therapy has been used, it has not significantly changed in recent years (9). The exploration of new treatment strategies is mandatory to improve the survival of these patients and guarantee good quality of life (8, 10).
Extracellular vesicles (EVs) are heterogeneous groups of vesicles with membranous subcellular structures released by almost all cell types. They can be found in various physiological fluids including blood, urine, saliva, lymph, and seminal fluid (11, 12). Based on the origin and diameter of vesicles, EVs can be divided into three major subclasses: exosomes, microvesicles, and apoptotic bodies. Exosomes are of endosomal origin and range in size from 30 to 120 nm and can be released by both eukaryotic and prokaryotic cells (13–15). Exosomes produced by tumor cells can create favorable conditions for tumor progression, such as induction of the angiogenic switch, increased vascular permeability, enhanced drug resistance and instigation of immune escape (16, 17). In addition, different types of cells, including mesenchymal stem cells (MSCs), adipocytes, fibroblasts, and immune cells in the tumor microenvironment (TME), can also induce the release of exosomes that promote tumor progression (18, 19). Understanding the role of exosomes in these cells will help unravel the mechanism underlying cancer. Furthermore, exosomes and their functions will provide more ways to diagnose, predict, and treat cancer (20). The utility of exosome detection in liquid biopsies is particularly promising for TC, and exploration of the use of exosomes as vehicles for the delivery of drug payloads is actively in progress. With the vast amount of information on exosomes emerging in the literature, these particles will likely be increasingly used in organisms. This review describes the function, separation methods, and biomedical applications of exosomes as well as current information on exosomes in TC.
The Biogenesis and Isolation of Exosomes
The general understanding of EVs continued to improve for decades since they were first defined as "platelet dust" in the 1960s (21). There has been a paradigm shift from garbage carriers to new modes of intercellular communication and clinical applications (22–24). EVs are nanoparticles surrounded by lipid membranes that contain proteins, lipids, metabolites, and biologically active nucleic acids, which include DNA, mRNA, and noncoding RNA (ncRNA). These contents can be transported to recipient cells and interact with them to trigger biological responses (25, 26). In response to various internal and external conditions, the molecular properties and contents of EVs are often altered (11). Exosomes are an important class of EVs and an attractive tool for diagnosing and treating diseases, with special biological significance. Exosomes play critical roles in a variety of pathways, including cancer (27), cardiovascular disease (28), nervous system disorders (29), infectious diseases (30), and immunological diseases (31). However, normal bodily functions are also dependent on exosomes (32). For example, exosomes can protect host cells in vitro by acting as scavenging agents for a variety of toxins (33, 34). TSHR exosomes from patients with Graves' disease can reduce autoantibody-mediated activation of thyroid function (35). The dichotomous behavior and plasticity of exosomes have encouraged researchers to put more efforts into manipulating exosomes and determining how they can harm or benefit an organism.
Exosomes arise from either intraluminal vesicles (ILVs) released after multivesicular bodies (MVBs) fusion with the plasma membrane or other means (22, 27, 32) (Figure 1). The formation of exosomes begins with the emergence of the initial endosomes through the endocytosis of extracellular components and then their gradual maturation into late sorting endosomes before they finally become MVBs, which contain several ILVs (22, 36). Concomitant with this process, proteins, DNA and RNA can be selectively loaded into MVBs, after which the exosomes are released into the extracellular space by MVBs. Furthermore, MVBs can also be degraded by fusion with lysosomes or recovered by the plasma membrane through the back-fusion pathway (32, 37). The released exosomes can deliver cargo to target cells through endocytosis, receptor-ligand interactions, and fusion with the plasma membrane. Indeed, the biogenesis of exosomes and the sorting of exosome cargoes are complex and worthy of further investigation. Both lipids, such as lysophospholipids and ceramides, and proteins, such as CD81, CD9, Rab, Ral, Alix, and the endosomal sorting complexes required for transport (ESCRT), participate in these processes (20, 27, 37). It should be emphasized that protein classification in endosomes is crucial for protein stability, endosome maturation, etc., and the ESCRT mechanism is an important contributor to this activity (38). ESCRT located on the MVB membrane encompasses four complexes: 0, I, II, and III. ESCRT-0 combined with ESCRT-I recruits ESCRT-II and cargoes, and then ESCRT-II recruits ESCRT-III to assist with ILV budding. Other studies have shown that proteins containing Bro1 domains, such as ALIX, HD-PTP, and yeast Bro1, can bypass ESCRT-II and ESCRT-I and promote the assembly of ESCRT-III components (38–40). Interestingly, there are also ESCRT-independent mechanisms that can influence exosome occurrence. For example, RAB31 drives the formation of ILV independently of the ESCRT machinery and tetraspanin pathway (41). In general, multiple molecules and mechanisms are involved in the biological processes of exosomes, but their control pathways are still poorly understood. Due to their cellular origin, intrinsic biology, microenvironment and other factors, the size, content, inclusion, and function of exosomes may also differ (20). Basic research on exosomes is key for their clinical development; however, technical barriers to exosome extraction procedures limit the basic and applied research of exosomes to a certain extent (42).
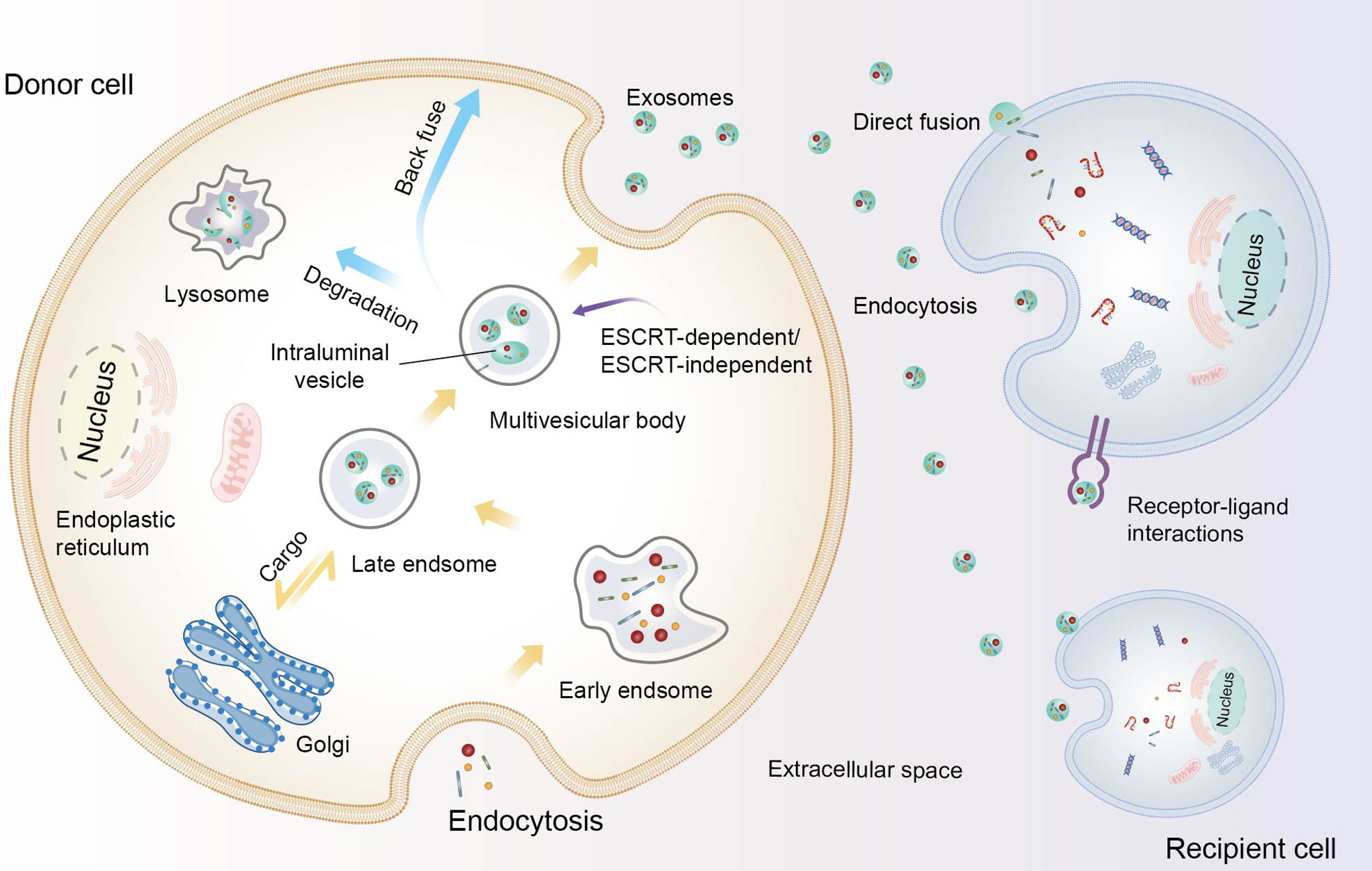
Figure 1 The biogenesis of exosomes and their communication with recipient cells. Constituents from the extracellular space enter the cell by endocytosis, leading to the formation of early endosomes and gradually maturing into late endosomes. After the endosomes undergo inward budding and the cargo is further packaged, intraluminal vesicles (ILVs) form and constitute multivesicular bodies (MVBs). Subsequently, MVBs degrade in lysosomes, are transported back to the plasma membrane, or fuse with the plasma membrane to release ILVs as exosomes. These loaded exosomes release proteins, nucleic acids, and other contents to recipient cells though direct fusion, internalization, and ligand-receptor interactions.
Current exosome separation strategies include ultracentrifugation, ultrafiltration, size-exclusion chromatography, immunoaffinity capture, charge neutralization-based polymer precipitation, and microfluidic techniques, but none of them are sufficient for high-yield extractions (43, 44). Although ultracentrifugation is the most widely used primary isolation method for exosomes, it is also susceptible to contamination, and has low reproducibility, and low sample throughput (45, 46). Exosome heterogeneity also remains a research challenge. Because the current exosome separation technology is still limited and there is no particularly uniform standard, the term "exosome" used in this paper is not specifically distinguished from a mixture of EVs, as suggested in the guidelines of the International Society for Extracellular Vesicles (ISEV) (47). Appropriate separation techniques or combination applications will help researchers solve many of the problems facing exosome research (43). Standardized exosome separation criteria will need to be developed in the future to meet the growing need for the specific screening of different sample types and for accurate exosome characterization.
Exosome Biomarkers for TC
The diagnosis of TC is a challenging issue. Multiple methods, including radionuclide scanning, high-resolution ultrasonography, and fine needle aspiration cytology (FNAC), are used to diagnose TC (48) (Figure 2). FNAC is a reliable application but not perfect, and a small ratio of indeterminate samples results in unnecessary surgeries or missed diagnoses (49). In oncology, liquid biopsy is an alternative, complementary diagnostic and prognostic tool that can overcome some weaknesses of tissue biopsy. It lacks the pitfalls of invasive and nonrepeatable sampling and may have more advantages in safety, early diagnosis, and dynamic monitoring (50, 51). In liquid biopsies, compared with circulating tumor cells (CTCs), circulating tumor DNA (ctDNA), cell-free DNA (cfDNA), and cell-free RNA (cfRNA), exosomes are more likely to have unique advantages in patients sometimes (52, 53). For example, KRAS mutations can be detected in tumor exosomes at a higher rate than in cfDNA for patients with pancreatic ductal adenocarcinoma (54).
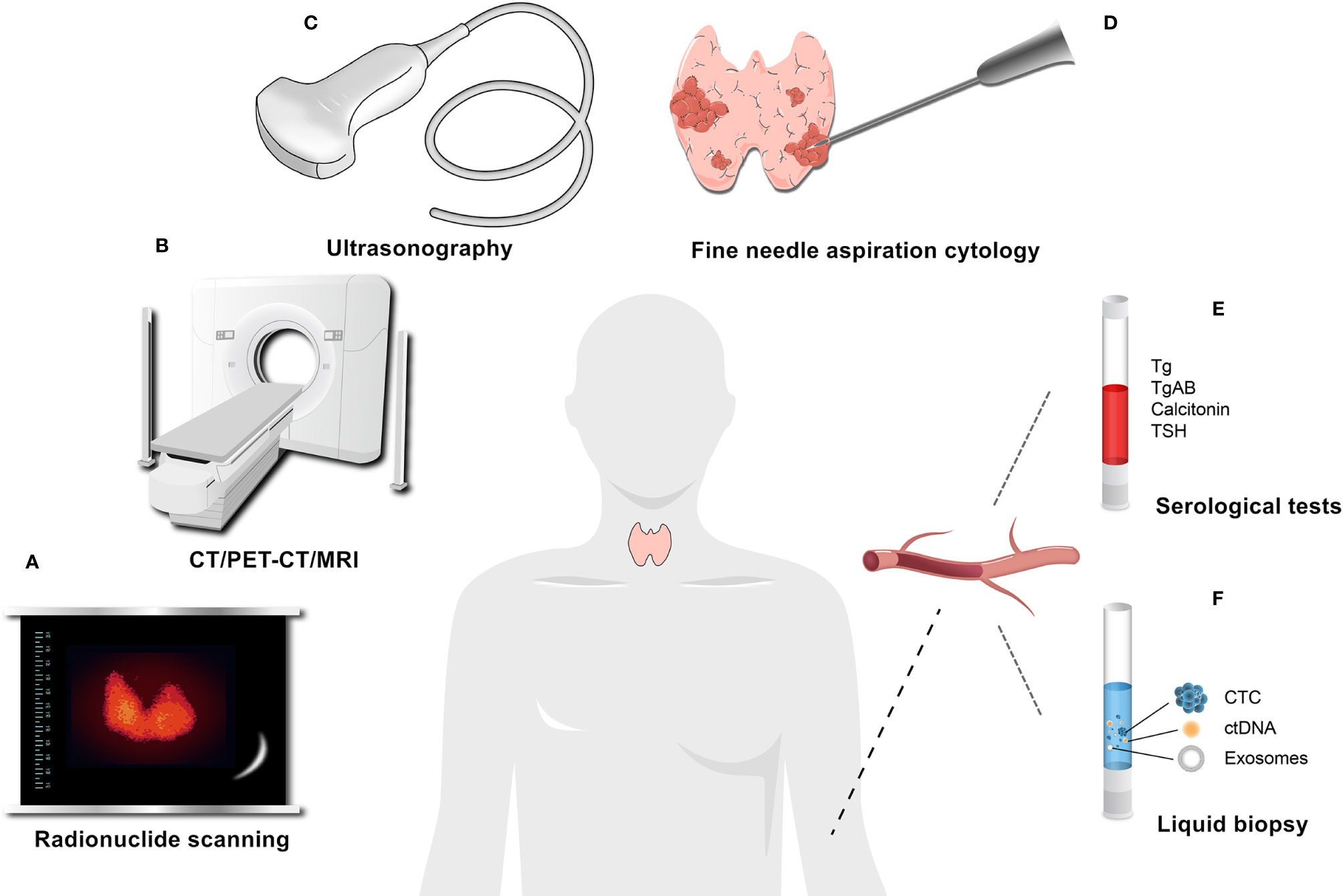
Figure 2 Common auxiliary examinations for thyroid cancer. (A) Radionuclide scanning. (B) CT/PET-CT/MRI. (C) Ultrasonography. (D) Fine needle aspiration cytology (FNAC). (E) Serological tests, such as Tg and Calcitonin, which are commonly used serum markers, can be used for the postoperative assessment and monitoring of DTC and the diagnosis and follow-up of MTC, respectively. (F) Liquid biopsy.
Recent studies have shown that exosomal microRNAs (miRNAs) are an appropriate and promising marker for the clinical diagnosis of tumors. The advantage of miRNAs in diagnosis is that they are highly stable, protected by a bilayer membrane, and contain key information related to the biological response of the tumor (55, 56). Lee and his collaborator found that the levels of miR-146b and miR-222 in TPC-1 exosomes were higher than those in NTHY cells, indicating that these two miRNAs may be biomarkers for PTC recurrence (57). Interestingly, another study detected plasma exosomes in PTC patients with or without lymph node metastasis (LNM), confirming that circulating exosomal miR-146b-5p and miR-222-3p have high diagnostic value for predicting LNM in PTC patients (58). Although most PTC patients have a good prognosis, LNM often occurs in the early stage, and predicting and diagnosing LNM in advance can prevent patients from experiencing unnecessary pain. The discrimination of follicular lesions is another difficulty in TC, and the current traditional diagnostic methods are often inadequate (52, 59). Samsonov et al. compared patients with benign thyroid nodules, and found that miR-31 in the serum exosomes of PTC patients was significantly upregulated. Moreover, miR-21 in the serum exosomes of FTC patients also showed the same changes. In addition, compared with FTC patients, PTC patients have reduced miR-21 levels in serum exosomes but significantly upregulated miR-181a-5p content. Therefore, these miRNAs in exosomes can be used as diagnostic markers for PTC and FTC (60). With the continuous improvement in high-throughput assays, more miRNAs were subsequently discovered. Wang ZY et al. conducted a plasma miRNA profile analysis on PTC patients and healthy subjects and then verified the experimental results. Among the candidate miRNAs, miR-346, miR-34a-5p, and miR-10a-5p were found to be upregulated in PTC plasma exosomes (61). Pan Q et al. isolated exosomes from the plasma of patients with PTC and nodular goiters through small RNA sequencing and a comprehensive analysis and identified a group of miRNAs in plasma exosomes as candidate biomarkers for the diagnosis of thyroid nodules; among these, miR-5189-3p had the best effect on diagnosing PTC (55). Dai D et al. discovered that miR-485-3p and miR-4433a-5p might serve as biomarkers for PTC diagnosis. Plasma exosomal miR-485-3p could enable discrimination between high-risk and low-risk PTCs (62). Li MH et al. screened a panel of miRNAs in plasma exosomes by analyzing exosomes from different patients and found that the combination of these miRNAs is more effective in identifying PTC and thyroid nodules than any single marker (63). These results suggest that the comprehensive detection of multiple exosome contents may be more advantageous.
Exosomes contain a large amount of ncRNAs that are involved in every stage of cancer progression. Circular RNAs (circRNAs) are a class of covalently closed endogenous biomolecules of ncRNAs. Special structural features make them highly stable (64). Under the protection of exosomes, circRNAs have been proposed as novel predictive biomarkers with clinical promise (65). Wu G et al. investigated the expression of circRNAs in PTC tissues and normal tissues by microarray analysis and demonstrated that circRASSF2 was upregulated in serum exosomes from PTC patients, suggesting the importance of circRASSF2 in tumor progression (66). Yang C et al. identified altered circRNA expression in serum exosomes from patients with PTC by high-throughput sequencing. circ_007293, circ_031752, and circ_020135 have been shown to be differentially expressed in the plasma of patients with PTC (67). In addition to serum exosomes, thyroglobulin in urine exosomes has also been found to be an emerging diagnostic marker for TC (68). These results suggest that the application of exosomes detected in TC could contribute to its diagnosis. However, due to the complex fluid composition of tumors, the dynamics of biomarkers, and the need for efficient enrichment and high sensitivity, the application of exosomes in cancer diagnosis remains challenging (26, 51). In recent years, the rapid development of nanotechnologies, including single‐exosome analysis used for the rapid and readable detection and molecular analysis of exosomes, may provide a great opportunity for expanding the use of exosomes (23, 51, 69). The combined application of multiple diagnostic methods, including exosomes, will accelerate the arrival of an era of more precise and refined thyroid diagnoses.
TC-Derived Exosomes in Tumor Progression
The development of tumors is a complex process, and malignant cells constantly interact with their surroundings (70). During this process, exosomes act as connectors and regulators to help the tumor grow. We have come to realize that the biological changes that occur in exosomes reflect their cell state (including oncogenic transformation) (11). A large body of evidence suggests that tumor cells often secrete more exosomes than do healthy cells, and the onset of cancer may cause changes in the molecular cargo of exosomes (71, 72). The number of exosomes is significantly higher in TC patients than in healthy subjects (53, 73), suggesting that exosomes are an important mediator in the progression of TC (Figure 3).
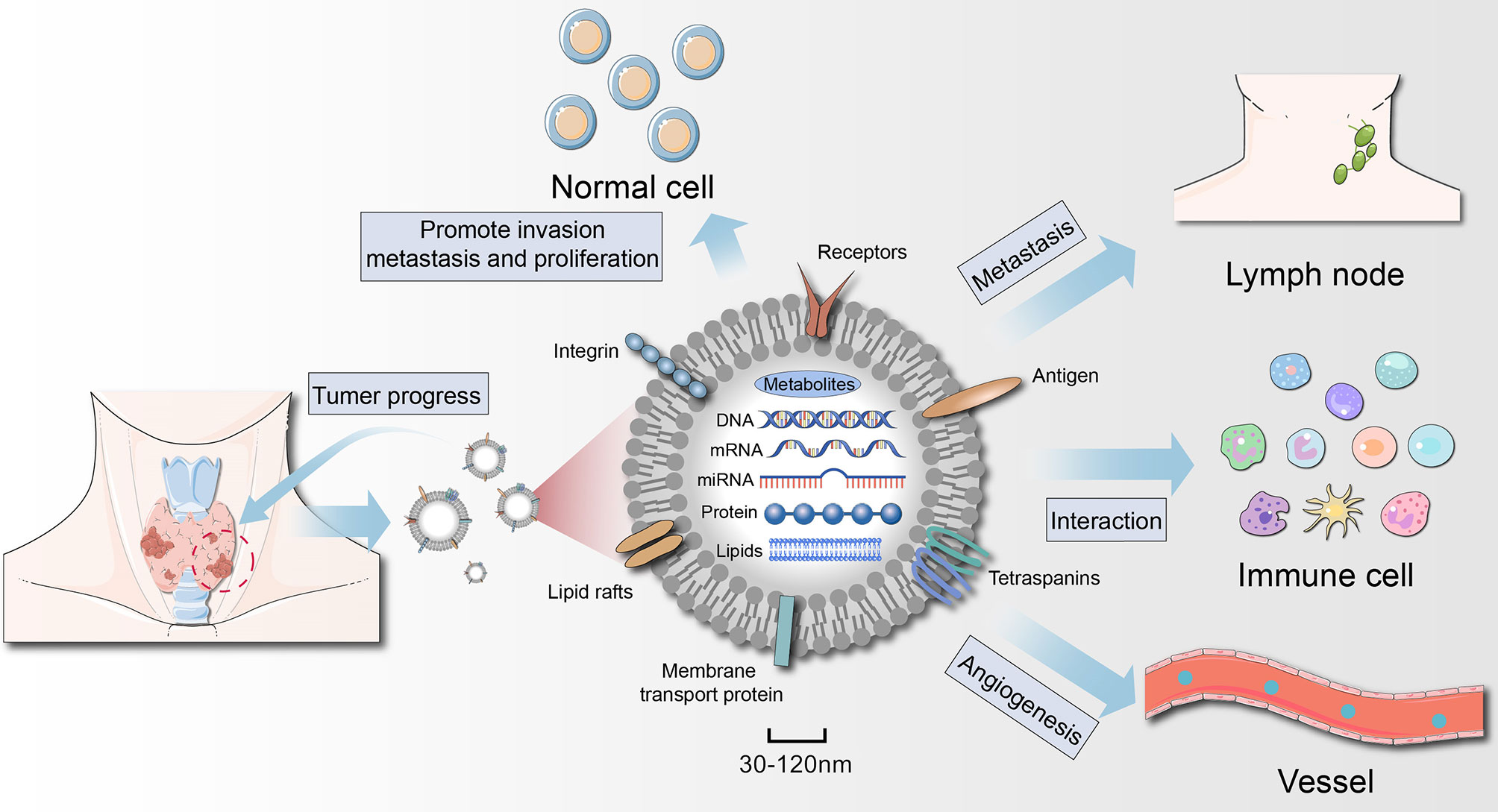
Figure 3 Role of thyroid cancer-derived exosomes in cancer progression. Exosomes act on tumor cells themselves, regulate angiogenesis, induce immunosuppression, and then remodel the tumor microenvironment to achieve tumor growth and metastasis. Exosomes secreted by thyroid cancer (thyroid CSCs) can also promote the oncogenic transformation of normal cells.
The Role of Exosomes in TC Growth and Metastasis
An increasing number of studies have shown that exosomes can not only be used for clinical diagnosis and treatment, but also play an important role in the formation and metastasis of TC. Exosome-mediated intercellular communication plays a critical role in the development of cancer (74). Tumor cells actively produce, release, and utilize exosomes to promote tumor growth (72). As mentioned earlier, miR-146b and miR-222, which are relatively abundant in the exosomes of TPC-1 cells, caused a negative proliferative effect on both TPC-1 and NTHY cells (57). Jiang K et al. observed that exosomal miR-146b-5p and miR-222-3p were not only associated with LNM but also significantly enhanced the migration and invasion abilities of PTC cells (58). The exosome cargo continuously changes, and the quantity depends on the course of disease progression (75). For instance, high expression of exosomal miR-485-3p correlates with tumor size, extrathyroidal extension, the BRAF mutation, LNM and an advanced clinical stage (62). Ye W et al. revealed that miR-423-5p may be involved in the carcinogenic activities of TC. Silencing miR-423-5p in TPC-1 cells inhibited PTC cell migration and invasion owing to reduced miR-423-5p in exosomes, providing a new approach for the treatment of PTC (76). The substances in exosomes play a significant role in the formation and metastasis of TC. Wu G et al. indicated the oncogenic property of circFNDC3B in PTC cells; circFNDC3B was upregulated in serum exosomes from PTC patients compared with healthy patients and could modulate PTC progression by activating the miR-1178/TLR4 pathway (77). In addition, Wu G confirmed that circRASSF2 can exert oncogenic activity in the tumorigenesis of PTC by sponging miR-1178 to upregulate TLR4 expression (66). The metastasis and survival of tumor cells depend on the TME in addition to intrinsic changes in cancer cells. Exosomes released by cancer cells can alter the behavior of localized or recruited stromal cells via external interactions that shift the phenotype of these stromal cells into a metastatic one. This leads to the formation of premetastatic niches that promote tumor growth (71, 78). Cancer-related fibroblasts (CAFs) are the primary stromal cells in the TME and play an essential role in several processes in cancer biology (79). Exosomes released from CAFs contribute to tumor progression and metastasis by transferring substances to cancer cells, and exosomes secreted by cancer cells can promote CAF transformation (20, 80). The crosstalk between TC cells and CAFs has been continuously revealed, and the role of exosomes in their interactions is worthy of further investigation (81). The ongoing revelation of the underlying mechanisms and pathogenesis of TC progression, as well as the influence of exosomes on these mechanisms, may provide more strategies for eventually curing TC.
Epithelial-Mesenchymal Transition (EMT) in TC
EMT is a cellular process during which epithelial cells acquire mesenchymal phenotypes and behaviors following the downregulation of proteins that indicate epithelial features (82). EMT arises early during tumor metastasis, playing a key role in mediating the development of an aggressive and invasive tumor phenotype. The main features of EMT include reduced adhesion and increased motility (83). EMT is triggered when a cell receives a signal from the microenvironment (82, 84). Studies have found a close link between EMT and cancer stem-like cells (CSCs), which are subsets of malignant tumor cells with the potential for self-renewal and differentiation and the ability to initiate tumors in vivo (85, 86). There are multiple ways by which EMT and CSCs interplay with each other (84, 87). Hardin et al. found that exosomes from thyroid CSCs transferred the long noncoding RNA (lncRNA) MALAT1, lncRNA ROR, and the EMT marker SLUG and induced EMT in normal thyroid cells (73). PTC-CSCs can also transmit the exosomal lncRNA DOCK9-AS2 to PTC, which can aggravate its malignant activities, including EMT, through the Wnt/β-catenin pathway (88). Critical components such as nucleotides, proteins, and organic intermediates in exosomes can serve as promising EMT regulators (89). Luo D et al. reported the differential expression of several proteins including the overexpression of proteins that are significantly related to cancer cell metastasis are associated with EMT (SRC, TLN1, ITGB2, and CAPNS1), in the exosomes of PTC patients with LNM (90). Some well-characterized miRNAs are important mediators involved in EMT-related signaling pathways induced by exosomes (91). Myriem et al. found that the overexpression of miR-145 in TPC-1 cells inhibited VEGF secretion and N-cadherin expression, which are closely related to the EMT process. Tumors actively excrete miR-145 into the blood via exosomes to reduce its expression in tumor tissue, thus inducing EMT and promoting the growth and metastasis of TC (92). Growing evidence has revealed that tumor cells are often in a different state along the EMT spectrum, as EMT is a continuous process with varying degrees of epithelial and mesenchymal characteristics and is often reversible (93, 94). We hypothesize that exosomes are involved in the dynamic changes between the epithelial and mesenchymal states. Future studies that show the inhibition of EMT-related changes and the reversal of EMT induction of tumors through exosomes will provide evidence of the use of exosomes to mitigate TC invasion and metastasis.
Effect of Exosomes on TC Angiogenesis
The growth and metastasis of malignant tumors depend on the formation and dilation of blood vessels (95). Exosomes from various tumors, such as breast cancer (15), colorectal cancer (96), gastric cancer (97), and lung cancer (80), have been shown to play an important role in promoting angiogenesis. It should be noted that the exact mechanism of exosome-driven angiogenesis is not clear, and the angiogenic characteristics of exosome cargoes from different tumor cells vary widely (98). The thyroid is an important endocrine organ with abundant vascularity (99). In addition, there are more blood vessels in thyroid tumor tissue than in normal thyroid tissue (100). In cancerous tissues, angiogenesis is implicated not only in primary tumors but also in the formation and further outgrowth of metastases (101). Some conditions, such as hypoxia or acidosis, enhance the secretion of exosomes into bodily fluids (102). Tumors are often hypoxic, and this hypoxic state results in advanced but dysfunctional vascularization and can alter different aspects of tumor metabolism (103). Feng W and colleagues revealed that exosomal miR-21-5p secreted by hypoxic PTC cells enhanced the angiogenic activities of human umbilical vein endothelial cells (HUVECs) both in vitro and in vivo. Exosomal miR-21-5p is a potent proangiogenic factor that increases angiogenesis by inhibiting the expression of TGFBI and COL4A1. In addition, it was also found to be elevated in the serum of PTC patients (104). This enhanced angiogenesis and increased vascular permeability in tumors support tumor growth and metastasis by continuously providing sufficient oxygen and nutrients to meet the nutritional needs of subsequent rapid metastatic growth, thereby increasing the proliferation of cancer cells and the formation of a premetastatic niche (105, 106). Currently, some drugs, such as docosahexaenoic acid and curcumin, that can inhibit the angiogenic effects induced by exosomes have been reported (107, 108).
Exosomes Mediate the Immune Regulation of TC
Cancer immune surveillance is considered a significant host protection process to maintain cell homeostasis and inhibit carcinogenesis (109). Cancer cells secrete exosomes to regulate the function and abundance of components in the TME, including immune effector cells and antigen-presenting cells, to escape their surveillance (110). In the microenvironment, exosomes also interact with tumor-associated macrophages (TAMs), which contribute to tumor growth, invasion, angiogenesis, and overall metastasis (105). Cancer-derived exosomes can act as cellular messengers to modulate macrophage polarization to the M2 phenotype, thus providing an immunosuppressive microenvironment for cancer cell survival and invasion (111). Macrophage infiltration is frequently detected in human thyroid tumors (112), and an increase in TAM density is positively correlated with the progression of TC, with TAMs accounting for more than 50% of nucleated cells in cases of ATC (113, 114). We suspect that TC can also affect TAMs through exosomes, but this hypothesis requires further study. Natural killer (NK) cells are recognized as particularly effective immune cells involved in immune surveillance because they are not restricted by the expression of MHC molecules (115). Exosomes from different tumor cells can not only be taken up by NK cells, but also inhibit NK cell cytotoxicity in several cancer types (116, 117). Cancer-derived exosomes induce NK cell dysfunction, inhibit antigen-presenting cells, block T cell activation, and enhance T cell apoptosis to block adaptive immune responses and suppress the antitumor immune response (118, 119). Additionally, the stimulation of NK cells, for example, with cytokines, can improve their tumor-killing effects. Zhu et al. demonstrated that exposure of NK cells to IL-15 results in the increased production of EVs and enhances the immunotherapeutic effects of EVs against several human cancers, including TC (120). Heat shock proteins (HSPs) can mediate immunomodulatory effects and the immune response (121). Caruso et al. found that the levels of Hsp27, Hsp60, and Hsp90 were increased in TPC tissue compared with normal peritumoral tissue and benign goiters. The levels of HSPs in the exosomes of patients with TPC before surgery were significantly higher than those in the exosomes from the same patients after surgery and from patients with benign goiters (122). TC is considered one of the most immunogenic cancers (123); thus, the rapid development of immunotherapy and exosomes as a delivery system brings new opportunities for the treatment of TC.
The Potential of Exosomes in TC Treatment
The ability of exosomes to transport biomolecules to recipient cells has led to the increased exploration of exosomes as a tool in the field of cancer therapy (23). Elucidation of the role of exosomes in improving drug sensitivity, tumor radiosensitivity, and other responses to treatments will provide ideas for patients with advanced TC. Therapeutic applications of exosomes are promising because they have been shown to be well tolerated and have high bioavailability, low toxicity, and low immunogenicity (124). Notably, employing exosomes as a drug delivery system can easily avoid the first-pass metabolic effect and be effectively delivered to target sites. Compared to liposomes, exosomes can function better at a distance because of their superior systemic retention (125). Encapsulating anticancer drugs within exosomes has shown potential for targeted delivery to tumors in animal models. Gangadaran et al. isolated EVs containing Renilla luciferase in conditioned medium from cultured ATC cells (CAL62) to test the targeting ability of tumor-derived exosomes to their parental tumor in a mouse model. Exosomes injected into ATC model mice were directly internalized into CAL62 tumors and accumulated in the tumor region 30 min after injection (126). Previous studies have shown that cancer cells readily internalize EVs to a greater extent than normal cells. These results support the notion that cancer cell-derived exosomes harbor a specific tropism that can be exploited to deliver drugs into tumor cells. Despite the controversy, the ability of exosomes secreted by stem cells (such as MSCs) to inhibit tumorigenic properties has raised interest in research into their treatment of tumors (127). For instance, bone marrow MSCs can deliver exosomal miR-152 to repress the proliferation and migration of TC cells by targeting DPP4 (128). MSC-derived exosomes have many advantages over stem cells or synthetic nanoparticles, such as availability, ease of manipulation, and preferential access to tumors (24, 129). However, continuous efforts are still needed before exosomes can be applied clinically. Driven by chemical engineering and nanotechnology, in silico exosomes have been fully developed and are predicted to further promote the development of treatments.
The benefits of using exosomes to enhance antitumor immune responses in tumor microenvironment are also emerging. As mentioned above, the IL-15-mediated release of EVs by NK cells has an immunotherapeutic effect on TC (120). More experiments are underway; for instance, engineered macrophage-coated nanoparticles are a promising drug delivery strategy for treating triple-negative breast cancer (130). Exosomes derived from processed dendritic cells showed strong antitumor effects in inducing effective antigen-specific humoral and cellular immune responses (131). Clinical trials of exosomes as cancer vaccines are also underway with the help of immune cells (26). Another benefit of using exosomes therapeutically is that certain environmental niches may allow only exosomes to pass through (24). With the joint efforts of researchers from different disciplines, these nanoparticles will translate from the laboratory to the clinic faster and more efficiently (69).
Conclusions and Perspectives
Exosomes promote the growth and development of TC via various strategies. The critical role of exosomes and their cargoes in TC has led to many insightful studies (Table 1). As discussed, tumor-derived exosomes can be used as a potentially valuable noninvasive diagnostic tool for TC in the field of liquid biopsy because they provide a broad diagnostic window for TC detection and monitoring, and future studies will aim to clinically validate promising treatments that may benefit more TC patients. In addition, work aimed at the clinical validation of promising treatments is ongoing. Although the field of exosome research has been deepened and enriched in recent years, the mechanistic understanding and clinical application of exosomes in TC are still lacking. Tumor immunity, drug resistance, radiotherapy sensitivity, and other aspects of TC are the primary difficulties associated with treating advanced TC, however, studies on the involvement of exosomes in these processes are rare. In addition to research on the cancer-promoting mechanism of tumor exosomes, for most TC patients with a good prognosis, the role of exosomes in tumor inhibition may provide ideas for the treatment of other tumors. Further research on exosomes will contribute to a more comprehensive and multidimensional understanding of TC. We also recognize that there is still a long way to go before exosomes are ready for clinical use, as the underlying mechanisms relating to exosome biogenesis, selective cargo loading, transport and function need to be clarified, and the best practices for isolating and identifying large numbers of high-quality vesicles for clinical use remain problematic (26). Considering the complexity of exosomes, more research is needed to deepen the understanding of exosomes and develop new diagnostic and therapeutic strategies for TC.
Author Contributions
DY conceived the idea and provided guidance. KF wrote the manuscript, GD and DN completed the figures, and RM and LZ carefully reviewed the manuscript. HL and YT made critical revisions to the manuscript. All authors contributed to the article and approved the submitted version.
Funding
This study was supported by the National Natural Science Foundation of China (81372863), the University Scientific and Technological Innovation Team Project of Henan Province (19IRTSTHN002), the Thousand Talents Science and Technology Innovation Leading Talents Subsidy Project of Central Plains (194200510011) and the Major Scientific Research Projects of Traditional Chinese Medicine in Henan Province (No.20-21ZYZD14).
Conflict of Interest
The authors declare that the research was conducted in the absence of any commercial or financial relationships that could be construed as a potential conflict of interests.
References
1. Cramer JD, Fu P, Harth KC, Margevicius S, Wilhelm SM. Analysis of the rising incidence of thyroid cancer using the Surveillance, Epidemiology and End Results national cancer data registry. Surgery (2010) 148(6):1147–52; discussion 52-3. doi: 10.1016/j.surg.2010.10.016
2. Carling T. Udelsman RJArom. Thyroid Cancer (2014) 65:125–37. doi: 10.1146/annurev-med-061512-105739
3. Miller K, Fidler-Benaoudia M, Keegan T, Hipp H, Jemal A, Siegel RJ. Cancer statistics for adolescents and young adults, 2020. Cacjfc (2020). doi: 10.3322/caac.21637
4. Xing M. Molecular pathogenesis and mechanisms of thyroid cancer. Cancer Nr (2013) 13(3):184–99. doi: 10.1038/nrc3431
5. Xu B, Ghossein R. Genomic Landscape of poorly Differentiated and Anaplastic Thyroid Carcinoma. Endocr Pathol (2016) 27(3):205–12. doi: 10.1007/s12022-016-9445-4
6. Luster M, Aktolun C, Amendoeira I, Barczynski M, Bible KC, Duntas LH, et al. European Perspective on 2015 American Thyroid Association Management Guidelines for Adult Patients with Thyroid Nodules and Differentiated Thyroid Cancer: Proceedings of an Interactive International Symposium. Thyroid (2019) 29(1):7–26. doi: 10.1089/thy.2017.0129
7. Naoum GE, Morkos M, Kim B, Arafat W. Novel targeted therapies and immunotherapy for advanced thyroid cancers. Mol Cancer (2018) 17(1):51. doi: 10.1186/s12943-018-0786-0
8. Kim WB, Jeon MJ, Kim WG, Kim TY, Shong YK. Unmet Clinical Needs in the Treatment of Patients with Thyroid Cancer. Endocrinol Metab (Seoul) (2020) 35(1):14–25. doi: 10.3803/EnM.2020.35.1.14
9. Qiu B, Li H, Chang Q, Yin D. Nomograms Predict Survival in Patients with Anaplastic Thyroid Carcinoma. smimjoe Res c (2019) 25:8447–56. doi: 10.12659/msm.918245
10. Salehian B, Liem SY, Mojazi Amiri H, Maghami E. Clinical Trials in Management of Anaplastic Thyroid Carcinoma; Progressions and Set Backs: A Systematic Review. Int J Endocrinol Metab (2019) 17(1):e67759. doi: 10.5812/ijem.67759
11. Möller A, Lobb RJ. The evolving translational potential of small extracellular vesicles in cancer. NrC (2020). doi: 10.1038/s41568-020-00299-w
12. Jabbari N, Karimipour M, Khaksar M, Akbariazar E, Heidarzadeh M, Mojarad B, et al. Tumor-derived extracellular vesicles: insights into bystander effects of exosomes after irradiation. Lasers Med Sci (2020) 35(3):531–45. doi: 10.1007/s10103-019-02880-8
13. Thery C, Zitvogel L, Amigorena S. Exosomes: composition, biogenesis and function. Nat Rev Immunol (2002) 2(8):569–79. doi: 10.1038/nri855
14. Théry C, Witwer K, Aikawa E, Alcaraz M, Anderson J, Andriantsitohaina R, et al. Minimal information for studies of extracellular vesicles 2018 (MISEV2018): a position statement of the International Society for Extracellular Vesicles and update of the MISEV2014 guidelines. J Extracell Vesicles (2018) 7(1):1535750. doi: 10.1080/20013078.2018.1535750
15. Jabbari N, Akbariazar E, Feqhhi M, Rahbarghazi R, Rezaie JJ. Breast cancer-derived exosomes: Tumor progression and therapeutic agents. Jocp (2020) 235(10):6345–56. doi: 10.1002/jcp.29668
16. Han Q, Zhao H, Jiang Y, Yin C, Zhang J. HCC-Derived Exosomes: Critical Player and Target for Cancer Immune Escape. Cells (2019) 8(6). doi: 10.3390/cells8060558
17. Wu M, Wang G, Hu W, Yao Y, Yu XF. Emerging roles and therapeutic value of exosomes in cancer metastasis. Mol Cancer (2019) 18(1):53. doi: 10.1186/s12943-019-0964-8
18. de Araujo Farias V, O’Valle F, Serrano-Saenz S, Anderson P, Andres E, Lopez-Penalver J, et al. Exosomes derived from mesenchymal stem cells enhance radiotherapy-induced cell death in tumor and metastatic tumor foci. Mol Cancer (2018) 17(1):122. doi: 10.1186/s12943-018-0867-0
19. Munteanu R, Onaciu A, Moldovan C, Zimta AA, Gulei D, Paradiso AV, et al. Adipocyte-Based Cell Therapy in Oncology: The Role of Cancer-Associated Adipocytes and Their Reinterpretation as Delivery Platforms. Pharmaceutics (2020) 12(5). doi: 10.3390/pharmaceutics12050402
20. Kalluri R, LeBleu VS. The biology, function, and biomedical applications of exosomes. Science (2020) 367(6478). doi: 10.1126/science.aau6977
21. Wolf P. The nature and significance of platelet products in human plasma. Br J Haematol (1967) 13(3):269–88. doi: 10.1111/j.1365-2141.1967.tb08741.x
22. Pan BT, Teng K, Wu C, Adam M, Johnstone RM. Electron microscopic evidence for externalization of the transferrin receptor in vesicular form in sheep reticulocytes. J Cell Biol (1985) 101(3):942–8. doi: 10.1083/jcb.101.3.942
23. Srivastava A, Amreddy N, Pareek V, Chinnappan M, Ahmed R, Mehta M, et al. Progress in extracellular vesicle biology and their application in cancer medicine. Wiley Interdiscip Rev Nanomed Nanobiotechnol (2020) 12(4):e1621. doi: 10.1002/wnan.1621
24. Madhusoodanan J. The therapeutic potential of exosomes. Nature (2020) 581(7807):S10–S1. doi: 10.1038/d41586-020-01375-9
25. Pathan M, Fonseka P, Chitti SV, Kang T, Sanwlani R, Van Deun J, et al. Vesiclepedia 2019: a compendium of RNA, proteins, lipids and metabolites in extracellular vesicles. Nucleic Acids Res (2019) 47(D1):D516–D9. doi: 10.1093/nar/gky1029
26. O’Brien K, Breyne K, Ughetto S, Laurent LC, Breakefield XO. RNA delivery by extracellular vesicles in mammalian cells and its applications. Nat Rev Mol Cell Biol (2020) 22(10):586–606. doi: 10.1038/s41580-020-0251-y
27. Pegtel DM, Gould SJ. Exosomes. Annu Rev Biochem (2019) 88:487–514. doi: 10.1146/annurev-biochem-013118-111902
28. Zhang J, Cui X, Guo J, Cao C, Zhang Z, Wang B, et al. Small but significant: Insights and new perspectives of exosomes in cardiovascular disease. J Cell Mol Med (2020) 24(15):8291–303. doi: 10.1111/jcmm.15492
29. Peng C, Trojanowski JQ, Lee VM. Protein transmission in neurodegenerative disease. Nat Rev Neurol (2020) 16(4):199–212. doi: 10.1038/s41582-020-0333-7
30. Hassanpour M, Rezaie J, Nouri M, Panahi YJI. genetics, epidemiology ejom, diseases egii. The role of extracellular vesicles in COVID-19 virus infection. Infect Genet Evol (2020) 85:104422. doi: 10.1016/j.meegid.2020.104422
31. Ocansey DKW, Zhang L, Wang Y, Yan Y, Qian H, Zhang X, et al. Exosome-mediated effects and applications in inflammatory bowel disease. Biol Rev Camb Philos Soc (2020) 95(5):1287–307. doi: 10.1111/brv.12608
32. van Niel G, D’Angelo G, Raposo G. Shedding light on the cell biology of extracellular vesicles. Nat Rev Mol Cell Biol (2018) 19(4):213–28. doi: 10.1038/nrm.2017.125
33. Keller MD, Ching KL, Liang FX, Dhabaria A, Tam K, Ueberheide BM, et al. Decoy exosomes provide protection against bacterial toxins. Nature (2020) 579(7798):260–4. doi: 10.1038/s41586-020-2066-6
34. Du Toit A. Distracting your enemy with bubbles. Nat Rev Microbiol (2020) 18(5):263. doi: 10.1038/s41579-020-0355-6
35. Edo N, Kawakami K, Fujita Y, Morita K, Uno K, Tsukamoto K, et al. Exosomes Expressing Thyrotropin Receptor Attenuate Autoantibody-Mediated Stimulation of Cyclic Adenosine Monophosphate Production. Thyroid (2019) 29(7):1012–7. doi: 10.1089/thy.2018.0772
36. Ludwig A, BJTijob G. biology c. Exosomes: small vesicles participating intercellular communication (2012) 44(1):11–5. doi: 10.1016/j.biocel.2011.10.005
37. Mathieu M, Martin-Jaular L, Lavieu G, Thery C. Specificities of secretion and uptake of exosomes and other extracellular vesicles for cell-to-cell communication. Nat Cell Biol (2019) 21(1):9–17. doi: 10.1038/s41556-018-0250-9
38. Vietri M, Radulovic M, Stenmark H. The many functions of ESCRTs. Nat Rev Mol Cell Biol (2020) 21(1):25–42. doi: 10.1038/s41580-019-0177-4
39. Bissig C, Gruenberg J. ALIX and the multivesicular endosome: ALIX in Wonderland. JTicb (2014) 24(1):19–25. doi: 10.1016/j.tcb.2013.10.009
40. Tang S, Buchkovich N, Henne W, Banjade S, Kim Y, Emr SJE. ESCRT-III activation by parallel action of ESCRT-I/II and ESCRT-0/Bro1 during MVB biogenesis. Elife (2016). doi: 10.7554/eLife.15507
41. Wei D, Zhan W, Gao Y, Huang L, Gong R, Wang W, et al. RAB31 marks and controls an ESCRT-independent exosome pathway. Cell Res (2020). doi: 10.1038/s41422-020-00409-1
42. Fais S, O’Driscoll L, Borras FE, Buzas E, Camussi G, Cappello F, et al. Evidence-Based Clinical Use of Nanoscale Extracellular Vesicles in Nanomedicine. ACS Nano (2016) 10(4):3886–99. doi: 10.1021/acsnano.5b08015
43. Yang D, Zhang W, Zhang H, Zhang F, Chen L, Ma L, et al. Progress, opportunity, and perspective on exosome isolation - efforts for efficient exosome-based theranostics. Theranostics (2020) 10(8):3684–707. doi: 10.7150/thno.41580
44. Ludwig N, Whiteside TL, Reichert TE. Challenges in Exosome Isolation and Analysis in Health and Disease. Int J Mol Sci (2019) 20(19). doi: 10.3390/ijms20194684
45. Gardiner C, Di Vizio D, Sahoo S, Thery C, Witwer KW, Wauben M, et al. Techniques used for the isolation and characterization of extracellular vesicles: results of a worldwide survey. J Extracell Vesicles (2016) 5:32945. doi: 10.3402/jev.v5.32945
46. Konoshenko MY, Lekchnov EA, Vlassov AV, Laktionov PP. Isolation of Extracellular Vesicles: General Methodologies and Latest Trends. BioMed Res Int (2018) 2018:8545347. doi: 10.1155/2018/8545347
47. Théry C, Witwer KW, Aikawa E, Alcaraz MJ, Anderson JD, Andriantsitohaina R, et al. Minimal information for studies of extracellular vesicles 2018 (MISEV2018): a position statement of the International Society for Extracellular Vesicles and update of the MISEV2014 guidelines. J Extracell Vesicles (2018) 7(1):1535750. doi: 10.1080/20013078.2018.1535750
48. Duick DS, Klopper JP, Diggans JC, Friedman L, Kennedy GC, Lanman RB, et al. The impact of benign gene expression classifier test results on the endocrinologist-patient decision to operate on patients with thyroid nodules with indeterminate fine-needle aspiration cytopathology. Thyroid (2012) 22(10):996–1001. doi: 10.1089/thy.2012.0180
49. Wong CKH, Liu X, Lang BHH. Cost-effectiveness of fine-needle aspiration cytology (FNAC) and watchful observation for incidental thyroid nodules. J Endocrinol Invest (2020) 43(11):1645–54. doi: 10.1007/s40618-020-01254-0
50. Kulasinghe A, Kapeleris J, Kimberley R, Mattarollo S, Thompson E, Thiery J, et al. The prognostic significance of circulating tumor cells in head and neck and non-small-cell lung cancer. Cancer Med (2018) 7(12):5910–9. doi: 10.1002/cam4.1832
51. Li W, Wang H, Zhao Z, Gao H, Liu C, Zhu L, et al. Emerging Nanotechnologies for Liquid Biopsy: The Detection of Circulating Tumor Cells and Extracellular Vesicles. Adv Mater (2019) 31(45):e1805344. doi: 10.1002/adma.201805344
52. Khatami F, Tavangar SM. Liquid Biopsy in Thyroid Cancer: New Insight. Int J Hematol Oncol Stem Cell Res (2018) 12(3):235–48.
53. Rappa G, Puglisi C, Santos MF, Forte S, Memeo L, Lorico A. Extracellular Vesicles from Thyroid Carcinoma: The New Frontier of Liquid Biopsy. Int J Mol Sci (2019) 20(5). doi: 10.3390/ijms20051114
54. Allenson K, Castillo J, San Lucas F, Scelo G, Kim D, Bernard V, et al. High prevalence of mutant KRAS in circulating exosome-derived DNA from early-stage pancreatic cancer patients. Ann Onco (2017) 28(4):741–7. doi: 10.1093/annonc/mdx004
55. Pan Q, Zhao J, Li M, Liu X, Xu Y, Li W, et al. Exosomal miRNAs are potential diagnostic biomarkers between malignant and benign thyroid nodules based on next-generation sequencing. Carcinogenesis (2020) 41(1):18–24. doi: 10.1093/carcin/bgz160
56. Hu C, Meiners S, Lukas C, Stathopoulos GT, Chen J. Role of exosomal microRNAs in lung cancer biology and clinical applications. Cell Prolif (2020) 53(6):e12828. doi: 10.1111/cpr.12828
57. Lee JC, Zhao JT, Gundara J, Serpell J, Bach LA, Sidhu S. Papillary thyroid cancer-derived exosomes contain miRNA-146b and miRNA-222. J Surg Res (2015) 196(1):39–48. doi: 10.1016/j.jss.2015.02.027
58. Jiang K, Li G, Chen W, Song L, Wei T, Li Z, et al. Plasma Exosomal miR-146b-5p and miR-222-3p are Potential Biomarkers for Lymph Node Metastasis in Papillary Thyroid Carcinomas. Onco Targets Ther (2020) 13:1311–9. doi: 10.2147/OTT.S231361
59. Jee HG, Kim BA, Kim M, Yu HW, Choi JY, Kim SJ, et al. Expression of SLC5A5 in Circulating Tumor Cells May Distinguish Follicular Thyroid Carcinomas from Adenomas: Implications for Blood-Based Preoperative Diagnosis. J Clin Med (2019) 8(2). doi: 10.3390/jcm8020257
60. Samsonov R, Burdakov V, Shtam T, Radzhabovsmall A CZ, Vasilyev D, Tsyrlina E, et al. Plasma exosomal miR-21 and miR-181a differentiates follicular from papillary thyroid cancer. Tumour Biol (2016) 37(9):12011–21. doi: 10.1007/s13277-016-5065-3
61. Wang Z, Lv J, Zou X, Huang Z, Zhang H, Liu Q, et al. A three plasma microRNA signature for papillary thyroid carcinoma diagnosis in Chinese patients. Gene (2019) 693:37–45. doi: 10.1016/j.gene.2019.01.016
62. Dai D, Tan Y, Guo L, Tang A, Zhao Y. Identification of exosomal miRNA biomarkers for diagnosis of papillary thyroid cancer by small RNA sequencing. Eur J Endocrinol (2020) 182(1):111–21. doi: 10.1530/EJE-19-0524
63. Liang M, Yu S, Tang S, Bai L, Cheng J, Gu Y, et al. A Panel of Plasma Exosomal miRNAs as Potential Biomarkers for Differential Diagnosis of Thyroid Nodules. Front Genet (2020) 11:449. doi: 10.3389/fgene.2020.00449
64. Zhang L, Hou C, Chen C, Guo Y, Yuan W, Yin D, et al. The role of N-methyladenosine (mA) modification in the regulation of circRNAs. Mol Cancer (2020) 19(1):105. doi: 10.1186/s12943-020-01224-3
65. Huang A, Zheng H, Wu Z, Chen M, Huang Y. Circular RNA-protein interactions: functions, mechanisms, and identification. Theranostics (2020) 10(8):3503–17. doi: 10.7150/thno.42174
66. Wu G, Zhou W, Lin X, Sun Y, Li J, Xu H, et al. circRASSF2 Acts as ceRNA and Promotes Papillary Thyroid Carcinoma Progression through miR-1178/TLR4 Signaling Pathway. Mol Ther Nucleic Acids (2020) 19:1153–63. doi: 10.1016/j.omtn.2019.11.037
67. Yang C, Wei Y, Yu L, Xiao Y. Identification of Altered Circular RNA Expression in Serum Exosomes from Patients with Papillary Thyroid Carcinoma by High-Throughput Sequencing. Med Sci Monit (2019) 25:2785–91. doi: 10.12659/MSM.915658
68. Huang T, Wang C, Chen K, Huang L. Urinary Exosomal Thyroglobulin in Thyroid Cancer Patients With Post-ablative Therapy: A New Biomarker in. Thyroid Cancer (2020) 11:382. doi: 10.3389/fendo.2020.00382
69. Tran PHL, Xiang D, Tran TTD, Yin W, Zhang Y, Kong L. Exosomes and Nanoengineering: A Match Made for Precision Therapeutics. Adv Mater (2020) 32(18):e1904040. doi: 10.1002/adma.201904040
70. Tan S, Xia L, Yi P, Han Y, Tang L, Pan Q, et al. Exosomal miRNAs in tumor microenvironment. J Exp Clin Cancer Res (2020) 39(1):67. doi: 10.1186/s13046-020-01570-6
71. Bebelman MP, Smit MJ, Pegtel DM, Baglio SR. Biogenesis and function of extracellular vesicles in cancer. Pharmacol Ther (2018) 188:1–11. doi: 10.1016/j.pharmthera.2018.02.013
72. Whiteside TL. Tumor-Derived Exosomes and Their Role in Cancer Progression. Adv Clin Chem (2016) 74:103–41. doi: 10.1016/bs.acc.2015.12.005
73. Hardin H, Helein H, Meyer K, Robertson S, Zhang R, Zhong W, et al. Thyroid cancer stem-like cell exosomes: regulation of EMT via transfer of lncRNAs. Lab Invest (2018) 98(9):1133–42. doi: 10.1038/s41374-018-0065-0
74. Tai YL, Chu PY, Lee BH, Chen KC, Yang CY, Kuo WH, et al. Basics and applications of tumor-derived extracellular vesicles. J BioMed Sci (2019) 26(1):35. doi: 10.1186/s12929-019-0533-x
75. Wang Y, Xu F, Zhong JY, Lin X, Shan SK, Guo B, et al. Exosomes as Mediators of Cell-to-Cell Communication in Thyroid Disease. Int J Endocrinol (2020) 2020:4378345. doi: 10.1155/2020/4378345
76. Ye W, Deng X, Fan Y. Exosomal miRNA423-5p mediated oncogene activity in papillary thyroid carcinoma: a potential diagnostic and biological target for cancer therapy. Neoplasma (2019) 66(4):516–23. doi: 10.4149/neo_2018_180824N643
77. Wu G, Zhou W, Pan X, Sun Z, Sun Y, Xu H, et al. Circular RNA Profiling Reveals Exosomal circ_0006156 as a Novel Biomarker in Papillary Thyroid Cancer. Mol Ther Nucleic Acids (2020) 19:1134–44. doi: 10.1016/j.omtn.2019.12.025
78. Biswas AK, Acharyya S. Understanding cachexia in the context of metastatic progression. Nat Rev Cancer (2020) 20(5):274–84. doi: 10.1038/s41568-020-0251-4
79. Sun LP, Xu K, Cui J, Yuan DY, Zou B, Li J, et al. Cancerassociated fibroblastderived exosomal miR3825p promotes the migration and invasion of oral squamous cell carcinoma. Oncol Rep (2019) 42(4):1319–28. doi: 10.3892/or.2019.7255
80. Fan J, Xu G, Chang Z, Zhu L, Yao J. miR-210 transferred by lung cancer cell-derived exosomes may act as proangiogenic factor in cancer-associated fibroblasts by modulating JAK2/STAT3 pathway. Clin Sci (Lond) (2020) 134(7):807–25. doi: 10.1042/CS20200039
81. Minna E, Brich S, Todoerti K, Pilotti S, Collini P, Bonaldi E, et al. Cancer Associated Fibroblasts and Senescent Thyroid Cells in the Invasive Front of Thyroid Carcinoma. Cancers (Basel) (2020) 12(1). doi: 10.3390/cancers12010112
82. Yang J, Antin P, Berx G, Blanpain C, Brabletz T, Bronner M, et al. Guidelines and definitions for research on epithelial-mesenchymal transition. Nat Rev Mol Cell Biol (2020) 21(6):341–52. doi: 10.1038/s41580-020-0237-9
83. Baum B, Settleman J, Quinlan MP. Transitions between epithelial and mesenchymal states in development and disease. Semin Cell Dev Biol (2008) 19(3):294–308. doi: 10.1016/j.semcdb.2008.02.001
84. Yu C, Liu Q, Chen C, Wang J. Quantification of the Underlying Mechanisms and Relationships Among Cancer, Metastasis, and Differentiation and Development. Front Genet (2019) 10:1388:1388. doi: 10.3389/fgene.2019.01388
85. Jimenez-Pascual A, Hale JS, Kordowski A, Pugh J, Silver DJ, Bayik D, et al. ADAMDEC1 Maintains a Growth Factor Signaling Loop in Cancer Stem Cells. Cancer Discov (2019) 9(11):1574–89. doi: 10.1158/2159-8290.CD-18-1308
86. Magee JA, Piskounova E, Morrison SJ. Cancer stem cells: impact, heterogeneity, and uncertainty. Cancer Cell (2012) 21(3):283–96. doi: 10.1016/j.ccr.2012.03.003
87. Kalluri R, Weinberg RA. The basics of epithelial-mesenchymal transition. J Clin Invest (2009) 119(6):1420–8. doi: 10.1172/JCI39104
88. Dai W, Jin X, Han L, Huang H, Ji Z, Xu X, et al. Exosomal lncRNA DOCK9-AS2 derived from cancer stem cell-like cells activated Wnt/β-catenin pathway to aggravate stemness, proliferation, migration, and invasion in papillary thyroid carcinoma. Cell Death Dis (2020) 11(9):743. doi: 10.1038/s41419-020-02827-w
89. Kim H, Lee S, Shin E, Seong KM, Jin YW, Youn H, et al. The Emerging Roles of Exosomes as EMT Regulators in Cancer. Cells (2020) 9(4). doi: 10.3390/cells9040861
90. Luo D, Zhan S, Xia W, Huang L, Ge W, Wang T. Proteomics study of serum exosomes from papillary thyroid cancer patients. Endocr Relat Cancer (2018) 25(10):879–91. doi: 10.1530/ERC-17-0547
91. Mannavola F, Pezzicoli G, Tucci M. DLC-1 down-regulation via exosomal miR-106b-3p exchange promotes CRC metastasis by the epithelial-to-mesenchymal transition. Clin Sci (Lond) (2020) 134(8):955–9. doi: 10.1042/CS20200181
92. Boufraqech M, Zhang L, Jain M, Patel D, Ellis R, Xiong Y, et al. miR-145 suppresses thyroid cancer growth and metastasis and targets AKT3. Endocr Relat Cancer (2014) 21(4):517–31. doi: 10.1530/ERC-14-0077
93. Nieto MA, Huang RY, Jackson RA, Thiery JP. Emt: 2016. Cell (2016) 166(1):21–45. doi: 10.1016/j.cell.2016.06.028
94. Liao TT, Yang MH. Hybrid Epithelial/Mesenchymal State in Cancer Metastasis: Clinical Significance and Regulatory Mechanisms. Cells (2020) 9(3). doi: 10.3390/cells9030623
95. Nishida N, Yano H, Nishida T, Kamura T, Kojiro M. Angiogenesis in cancer. Vasc Health Risk Manag (2006) 2(3):213–9. doi: 10.2147/vhrm.2006.2.3.213
96. Shang A, Wang X, Gu C, Liu W, Sun J, Zeng B, et al. Exosomal miR-183-5p promotes angiogenesis in colorectal cancer by regulation of FOXO1. Aging (Albany NY) (2020) 12(9):8352–71. doi: 10.18632/aging.103145
97. Du J, Liang Y, Li J, Zhao JM, Wang ZN, Lin XY. Gastric Cancer Cell-Derived Exosomal microRNA-23a Promotes Angiogenesis by Targeting PTEN. Front Oncol (2020) 10:326. doi: 10.3389/fonc.2020.00326
98. Ahmadi M, Rezaie J. Tumor cells derived-exosomes as angiogenenic agents: possible therapeutic implications. J Trans Med (2020) 18(1):249. doi: 10.1186/s12967-020-02426-5
99. Rajabi S, Dehghan MH, Dastmalchi R, Jalali Mashayekhi F, Salami S, Hedayati M. The roles and role-players in thyroid cancer angiogenesis. Endocr J (2019) 66(4):277–93. doi: 10.1507/endocrj.EJ18-0537
100. Akslen LA, Livolsi VA. Increased angiogenesis in papillary thyroid carcinoma but lack of prognostic importance. Hum Pathol (2000) 31(4):439–42. doi: 10.1053/1-ip.2000.6548
101. Hanahan D, Weinberg RA. Hallmarks of cancer: the next generation. Cell (2011) 144(5):646–74. doi: 10.1016/j.cell.2011.02.013
102. Mashouri L, Yousefi H, Aref AR, Ahadi AM, Molaei F, Alahari SK. Exosomes: composition, biogenesis, and mechanisms in cancer metastasis and drug resistance. Mol Cancer (2019) 18(1):75. doi: 10.1186/s12943-019-0991-5
103. Muz B, de la Puente P, Azab F, Azab AK. The role of hypoxia in cancer progression, angiogenesis, metastasis, and resistance to therapy. Hypoxia (Auckl) (2015) 3:83–92. doi: 10.2147/HP.S93413
104. Wu F, Li F, Lin X, Xu F, Cui RR, Zhong JY, et al. Exosomes increased angiogenesis in papillary thyroid cancer microenvironment. Endocr Relat Cancer (2019) 26(5):525–38. doi: 10.1530/ERC-19-0008
105. Feng W, Dean DC, Hornicek FJ, Shi H, Duan Z. Exosomes promote pre-metastatic niche formation in ovarian cancer. Mol Cancer (2019) 18(1):124. doi: 10.1186/s12943-019-1049-4
106. Zhou W, Fong MY, Min Y, Somlo G, Liu L, Palomares MR, et al. Cancer-secreted miR-105 destroys vascular endothelial barriers to promote metastasis. Cancer Cell (2014) 25(4):501–15. doi: 10.1016/j.ccr.2014.03.007
107. Hannafon B, Carpenter K, Berry W, Janknecht R, Dooley W, Ding WJMC. Exosome-mediated microRNA signaling from breast cancer cells is altered by the anti-angiogenesis agent docosahexaenoic acid (DHA). Mol Cancer (2015) 14:133. doi: 10.1186/s12943-015-0400-7
108. Taverna S, Fontana S, Monteleone F, Pucci M, Saieva L, De Caro V, et al. Curcumin modulates chronic myelogenous leukemia exosomes composition and affects angiogenic phenotype via exosomal miR-21. Oncotarget (2016) 7(21):30420–39. doi: 10.18632/oncotarget.8483
109. Kim R, Emi M, Tanabe K. Cancer immunoediting from immune surveillance to immune escape. Immunology (2007) 121(1):1–14. doi: 10.1111/j.1365-2567.2007.02587.x
110. Yi M, Xu L, Jiao Y, Luo S, Li A, Wu K. The role of cancer-derived microRNAs in cancer immune escape. J Hematol Oncol (2020) 13(1):25. doi: 10.1186/s13045-020-00848-8
111. Baig MS, Roy A, Rajpoot S, Liu D, Savai R, Banerjee S, et al. Tumor-derived exosomes in the regulation of macrophage polarization. Inflamm Res (2020) 69(5):435–51. doi: 10.1007/s00011-020-01318-0
112. Mazzoni M, Mauro G, Erreni M, Romeo P, Minna E, Vizioli MG, et al. Senescent thyrocytes and thyroid tumor cells induce M2-like macrophage polarization of human monocytes via a PGE2-dependent mechanism. J Exp Clin Cancer Res (2019) 38(1):208. doi: 10.1186/s13046-019-1198-8
113. Caillou B, Talbot M, Weyemi U, Pioche-Durieu C, Al Ghuzlan A, Bidart JM, et al. Tumor-associated macrophages (TAMs) form an interconnected cellular supportive network in anaplastic thyroid carcinoma. PLoS One (2011) 6(7):e22567. doi: 10.1371/journal.pone.0022567
114. Huang FJ, Zhou XY, Ye L, Fei XC, Wang S, Wang W, et al. Follicular thyroid carcinoma but not adenoma recruits tumor-associated macrophages by releasing CCL15. BMC Cancer (2016) 16:98. doi: 10.1186/s12885-016-2114-7
115. Caligiuri MA. Human natural killer cells. Blood (2008) 112(3):461–9. doi: 10.1182/blood-2007-09-077438
116. Huyan T, Du Y, Huang Q, Huang Q, Li Q. Uptake Characterization of Tumor Cell-derived Exosomes by Natural Killer Cells. Iran J Public Health (2018) 47(6):803–13.
117. Hedlund M, Nagaeva O, Kargl D, Baranov V, Mincheva-Nilsson L. Thermal- and oxidative stress causes enhanced release of NKG2D ligand-bearing immunosuppressive exosomes in leukemia/lymphoma T and B cells. PLoS One (2011) 6(2):e16899. doi: 10.1371/journal.pone.0016899
118. Czernek L, Duchler M. Functions of Cancer-Derived Extracellular Vesicles in Immunosuppression. Arch Immunol Ther Exp (Warsz) (2017) 65(4):311–23. doi: 10.1007/s00005-016-0453-3
119. Guo Y, Ji X, Liu J, Fan D, Zhou Q, Chen C, et al. Effects of exosomes on pre-metastatic niche formation in tumors. Mol Cancer (2019) 18(1):39. doi: 10.1186/s12943-019-0995-1
120. Zhu L, Gangadaran P, Kalimuthu S, Oh JM, Baek SH, Jeong SY, et al. Novel alternatives to extracellular vesicle-based immunotherapy - exosome mimetics derived from natural killer cells. Artif Cells Nanomed Biotechnol (2018) 46(sup3):S166–S79. doi: 10.1080/21691401.2018.1489824
121. Taha EA, Ono K, Eguchi T. Roles of Extracellular HSPs as Biomarkers in Immune Surveillance and Immune Evasion. Int J Mol Sci (2019) 20(18). doi: 10.3390/ijms20184588
122. Caruso Bavisotto C, Cipolla C, Graceffa G, Barone R, Bucchieri F, Bulone D, et al. Immunomorphological Pattern of Molecular Chaperones in Normal and Pathological Thyroid Tissues and Circulating Exosomes: Potential Use in Clinics. Int J Mol Sci (2019) 20(18). doi: 10.3390/ijms20184496
123. Chakladar J, Li WT, Bouvet M, Chang EY, Wang-Rodriguez J, Ongkeko WM. Papillary Thyroid Carcinoma Variants are Characterized by Co-dysregulation of Immune and Cancer Associated Genes. Cancers (Basel) (2019) 11(8). doi: 10.3390/cancers11081179
124. Kamerkar S, LeBleu VS, Sugimoto H, Yang S, Ruivo CF, Melo SA, et al. Exosomes facilitate therapeutic targeting of oncogenic KRAS in pancreatic cancer. Nature (2017) 546(7659):498–503. doi: 10.1038/nature22341
125. Lim W, Kim HS. Exosomes as Therapeutic Vehicles for Cancer. Tissue Eng Regener Med (2019) 16(3):213–23. doi: 10.1007/s13770-019-00190-2
126. Gangadaran P, Li XJ, Kalimuthu SK, Min OJ, Hong CM, Rajendran RL, et al. New Optical Imaging Reporter-labeled Anaplastic Thyroid Cancer-Derived Extracellular Vesicles as a Platform for In Vivo Tumor Targeting in a Mouse Model. Sci Rep (2018) 8(1):13509. doi: 10.1038/s41598-018-31998-y
127. Shojaei S, Hashemi SM, Ghanbarian H, Salehi M, Mohammadi-Yeganeh S. Effect of mesenchymal stem cells-derived exosomes on tumor microenvironment: Tumor progression versus tumor suppression. J Cell Physiol (2019) 234(4):3394–409. doi: 10.1002/jcp.27326
128. Tang M, Wang Q, Wang K, Wang F. Mesenchymal stem cells-originated exosomal microRNA-152 impairs proliferation, invasion and migration of thyroid carcinoma cells by interacting with DPP4. J Endocrinol Invest (2020) 43(12):1787–96. doi: 10.1007/s40618-020-01406-2
129. Galland S, Stamenkovic I. Mesenchymal stromal cells in cancer: a review of their immunomodulatory functions and dual effects on tumor progression. J Pathol (2020) 250(5):555–72. doi: 10.1002/path.5357
130. Li S, Wu Y, Ding F, Yang J, Li J, Gao X, et al. Engineering macrophage-derived exosomes for targeted chemotherapy of triple-negative breast cancer. Nanoscale (2020) 12(19):10854–62. doi: 10.1039/d0nr00523a
Keywords: extracellular vesicles, exosome, thyroid cancer, biomarker, oncology treatment
Citation: Feng K, Ma R, Zhang L, Li H, Tang Y, Du G, Niu D and Yin D (2020) The Role of Exosomes in Thyroid Cancer and Their Potential Clinical Application. Front. Oncol. 10:596132. doi: 10.3389/fonc.2020.596132
Received: 18 August 2020; Accepted: 30 October 2020;
Published: 01 December 2020.
Edited by:
Qiang Shen, Louisiana State University, United StatesReviewed by:
Jafar Rezaie, Urmia University of Medical Sciences, IranYu Wang, Tianjin Medical University Cancer Institute and Hospital, China
Copyright © 2020 Feng, Ma, Zhang, Li, Tang, Du, Niu and Yin. This is an open-access article distributed under the terms of the Creative Commons Attribution License (CC BY). The use, distribution or reproduction in other forums is permitted, provided the original author(s) and the copyright owner(s) are credited and that the original publication in this journal is cited, in accordance with accepted academic practice. No use, distribution or reproduction is permitted which does not comply with these terms.
*Correspondence: Detao Yin, ZGV0YW95aW5Aenp1LmVkdS5jbg==