- 1Medical Oncology Unit, IRCCS Istituto Tumori “Giovanni Paolo II”, Bari, Italy
- 2Internal Medicine “G. Baccelli”, Department of Biomedical Sciences and Human Oncology, University of Bari, Bari, Italy
- 3Pharmacy Unit, IRCCS Istituto Tumori “Giovanni Paolo II”, Bari, Italy
- 4Rare Tumors and Melanoma Unit, IRCCS Istituto Tumori “Giovanni Paolo II”, Bari, Italy
- 5Radiology Unit, Ricerche Radiologiche “Maggialetti”, Molfetta, Italy
- 6Unit of Hematology and Cell Therapy, Laboratory of Hematological Diagnostics and Cell Characterization, IRCCS Istituto Tumori “Giovanni Paolo II”, Bari, Italy
- 7Pathology Unit, IRCCS Istituto Tumori “Giovanni Paolo II”, Bari, Italy
Recent advances in tumor immunotherapy have made it possible to efficiently unleash immune effectors, reacting against neoplastic cells. Although these approaches primarily aim to eradicate malignancy, immune-related adverse events (irAEs) often influence patients’ prognosis, constituting a new spectrum of side effects. Taking into account the typical microenvironment and the intricate equilibrium between the anti-tumor response and the immune cells, the thymoma constitutes a unicum in the immune-oncology field. We report a fatal immune-mediated adverse events’ storm in a thymoma patient treated with Pembrolizumab, leading to hepatotoxicity accompanied by lymphocytosis, thrombocytopenia, and thyroid dysfunction, unveiling a novel potential pathophysiological effect of immunotherapy. The clinical proficiency of the immune checkpoint inhibitors in thymoma patients warrants timely prevention and management of off-target consequences in order to optimize this promising therapeutic option. This case report describes a unique consequence of irAEs, emerging as a red flag warranting a multidisciplinary approach.
Introduction
Thymic Epithelial Tumors (TETs) are thoracic malignancies with a low incidence in the worldwide population, that present with different clinical features and prognosis (1, 2). From the histopathological standpoint, thymomas have the greater incidence (highest value 0.68/100,000 in the 70–79-year-old age subgroup) compared to the thymic carcinoma (highest value 0.25/100,000 in the 70N74-year-old age subgroup). The 5-year overall survival (OS) rate are 90 and 55%, for thymoma and thymic carcinoma, respectively (1, 2).
Thymoma and thymic carcinoma share the origin in the thymic epithelium. The thymus is a complex immune organ, whose physiological role is to produce T-cells, involved in all processes of immune response comprising non-self reaction (3). Immature T-cells reach the thymus from the bone marrow through the blood stream. The precursors of αβ T-cells are deemed double-negative (DN). In the gland cortex, T-cell receptors (TCRs) CD4 and CD8 expression takes place. Therefore, CD4+CD8+ double positive (DP) T-cells carrying αβ TCRs recognize peptide-major histocompatibility (MHC) complexes, inducing the production of single positive (SP) CD4+ helper T-cells (MHCII) and CD8+ cytotoxic T-cells (MHCI), active in the adaptive immunity (4, 5). Conversely, a negative selection leads to apoptosis of self-reacting T clones (Figure 1).
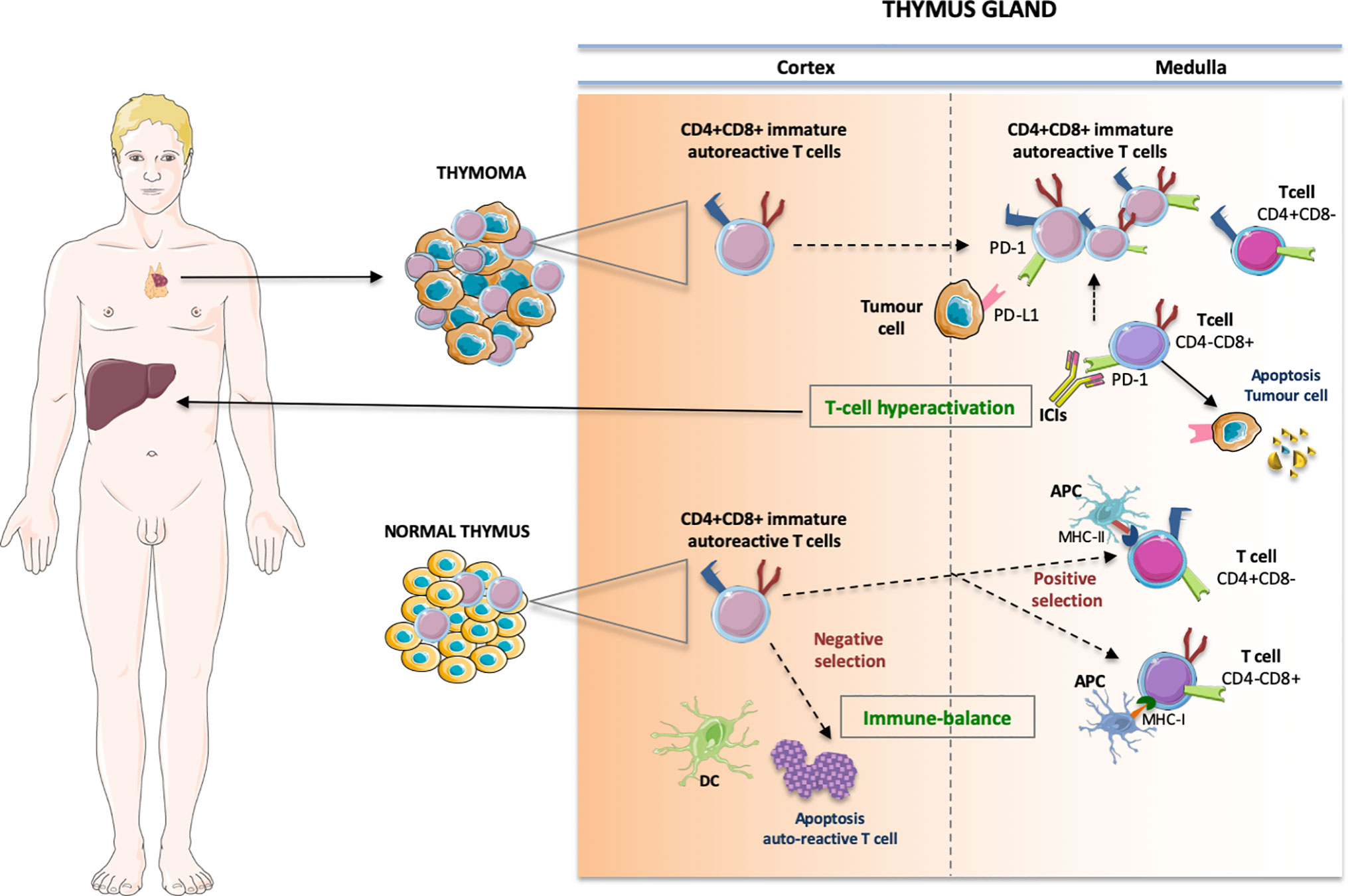
Figure 1 The pathophysiologic process that produces the immune-related storm in thymoma has not yet been fully clarified. Some possible vicious cycles involve enhanced T-cell activity against antigens present in the thymus and in the “innocent” bystander organs, whereby growing amounts of preexisting autoreactive T-cells are unleashed from the medulla, breaking the immune-equilibrium existing in the normal thymus. In particular, the immature CD4+CD8+ double positive cells move to single positive CD4+ helper T-cells (binding MHC class II) and CD8+ cytotoxic T-cells (binding MHC cIass I) involved in the adaptive immunity while a negative selection leads to apoptosis of self-reacting T clones. In thymic neoplasia the immature thymic lymphocytes may escape quality control by DC prompting T-cell hyperactivation and autoimmune manifestations. DC, dentritic cell; APC, antigen presenting cell; MHC, major histocompatibility complex.
Thymomas are generally associated with autoimmune disorders such as myasthenia gravis, pure red cell aplasia, and hypogammaglobulinemia, often requiring a full workup and multidisciplinary approach, comprising surgery, symptomatic therapy, immune suppression, and immunoglobulin administration (3, 6, 7). From a pathology standpoint, in thymomas T-cells are immature and express terminal deoxynucleotidyl transferase as phenotypic marker; conversely, thymic carcinomas are defined as tumors lacking immature T lymphocytes and never elicit an autoimmune disease. Therefore, the histological features and tumor microenvironment are key elements to distinguish thymomas from thymic carcinomas and, consequently, to tailor the most appropriate histotype-driven treatment (4, 8, 9).
Despite the demonstrated effectiveness of several agents against TETs, these often ultimately relapse, becoming resistant to drugs. Moreover, an optimal chemotherapeutic regimen in platinum-refractory TETs at advanced stages of disease has not yet been identified. Due to the rarity of the disease and the lack of significant randomized controlled trials, the available evidence has been obtained only from phase I to II trials of small and heterogeneous sample size, retrospective studies or published case reports (1).
Different studies showed that PD1/PDL1 inhibitors are effective in advanced TETs and contributed to the approval of Pembrolizumab for thymic carcinoma (10, 11). In the scientific literature, case series of Pembrolizumab or Avelumab also in thymoma subjects have been reported (10, 12). Despite an evident anti-tumor activity, immune-checkpoint inhibitors (ICIs) have displayed a critical immune-mediated toxicity profile; therefore, their safety represents an evolving landscape (10, 12, 13). Specifically, severe systemic ICI-related side effects in patients with thymoma have been described, including myocarditis, hepatitis, and myositis, highlighting the importance of careful patient selection while evaluating immunotherapeutic agents for treatment (Supplementary Table 1) (10, 12, 14, 15).
Given the promising anti-tumor activity and the peculiar thymic physiology within the immune system education, it is of paramount importance to identify potential factors able to predict ICI-related adverse events.
Herein, we report a case of fulminant immune-related hepatitis along with an autoimmune storm, heralded by a marked lymphocytosis, in a refractory patient affected by B2 thymoma treated with Pembrolizumab.
Case Description
A Caucasian 42-year-old male with metastatic thymomas received Pembrolizumab (2 mg/kg q21), after three lines of standard chemotherapy. Four years earlier, the patient had been found to have a massive anterior mediastinum mass and numerous pleural nodules during a workup for progressive dyspnea. Percutaneous pleural biopsy at that timepoint demonstrated CK19+, CK5/6+, EMA− and CK20− versus a CD99+, CD5+, TdT+, CD1a+ pattern in the epithelial and lymphoid components, respectively. Moreover, the tumor-infiltrating lymphocytes’ immunohistochemical phenotype demonstrated an immature T-cell population expressing TdT (presumed CD4, CD8 double positive) and PD-1 at baseline (Figure 2). Therefore, off-label medication with Pembrolizumab was required due to the failure of previous lines of chemotherapy and lack of other standard medications with proven effectiveness. At baseline, laboratory findings were unremarkable, except for a slight increase in absolute lymphocyte count (5,670/μl, normal range, 600 to 3,400/μl) and HBV seroconversion. The patient was admitted to hospital with fever and ecchymosis, 20 days after receiving his second dose of Pembrolizumab. Blood tests performed five days earlier revealed marked lymphocytosis, 11,600/μl; thrombocytopenia, 40,100/μl (normal range, 142,000 to 424,000/μl) and alterations of liver enzymes with increased aspartate amino transferase (AST) levels to 442 U/L (normal range up to 40 U/L) and alanine amino transferase (ALT) levels to 258 U/L (normal range 6−61 U/L). Initial workup upon hospitalization showed a further increase of lymphocytes, 24,310/μl (72% of 33,680/μl total white blood cells); thrombocytopenia, 31,000/μl, grade 4 hepatic toxicity with aspartate amino transferase (AST), 2,103 U/L increasing to 52 times normal range (up to 40 U/L), and ALT 714 U/L (normal range, 8 to 61 U/L); total and direct bilirubin, 18.19 mg/dl and 11.28 mg/dl, respectively (normal range up to 1.2 mg/dl and up to 0.3 mg/dl). Thyroid dysfunction was also shown, with FT4 >7.7 ng/dl (normal range 0.9 to 1.7 ng/dl) and TSH 0.013 mcUI/ml (normal range 0.3 to 4.2 mcUI/ml). Flow cytometric analysis was performed to study lymphocyte subpopulations. T-cells contained a predominant T-cytotoxic lymphocyte (CD3+, CD8+) population, along with low levels of natural killer T (NKT) cells and B-cells (Supplementary Figure 1).
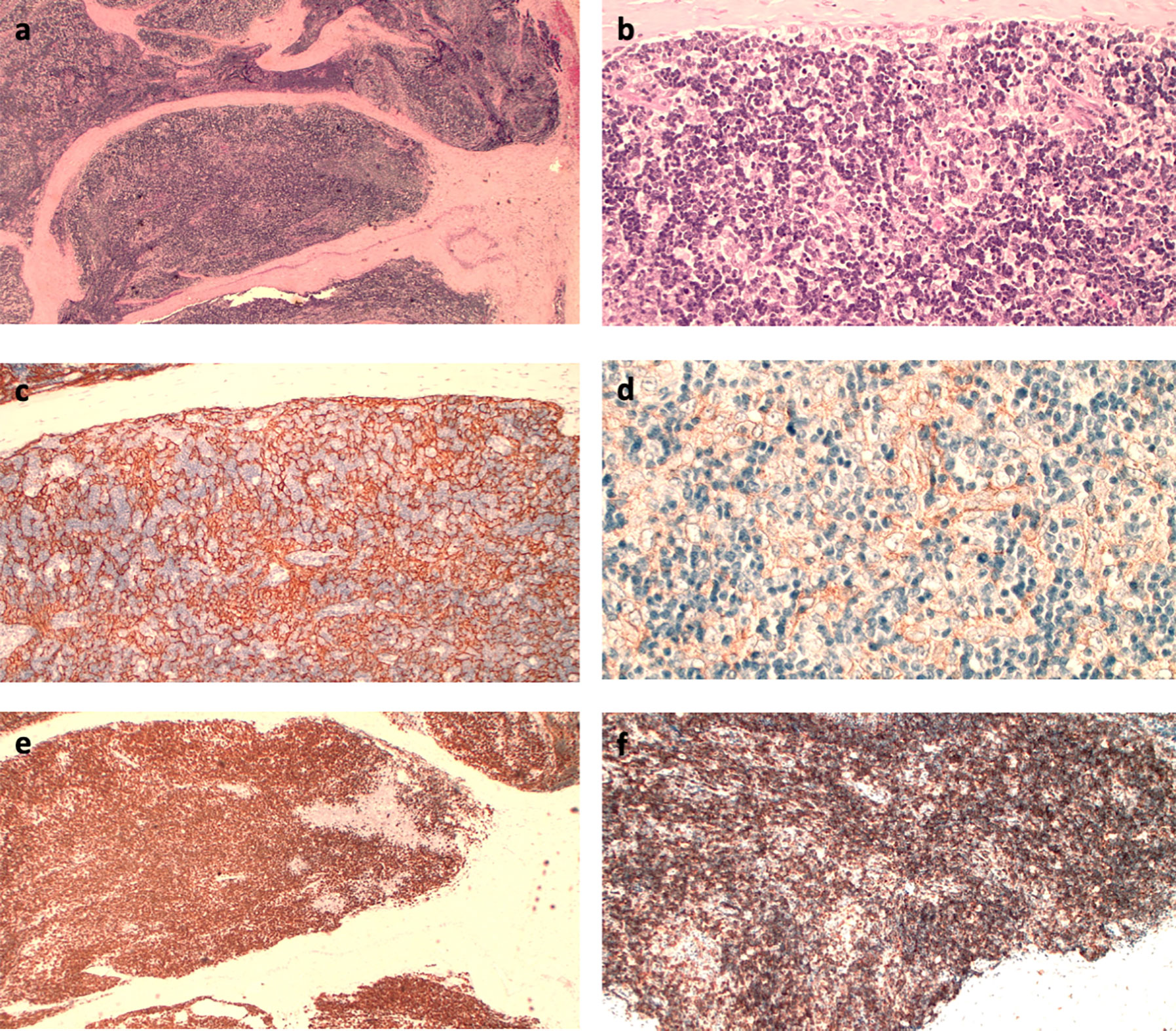
Figure 2 (A) Hematoxylin–eosin (HE) immunostaining (IHC): fibrous septa within the tumor separate the lymphoepithelial elements (4×); (B) HE IHC: aggregates of large epithelial cells with a clear cytoplasm interspersed between lymphoid cells (20×); (C) CK 19 IHC: dense epithelial cell network (10×); (D) PD-L1 IHC (Clone 22 C3): Membranous positivity of the epithelial cells (20×); (E) TdT IHC: lymphocytes consist predominantly of immature T-cells; (F) CD8 IHC: immune-positivity of CD8 on immature lymphocytes (10×).
Biochemical and laboratory findings paralleled the clinically evident liver failure course over time characterized by bruising, progressive, jaundice, changes in personality, aggressive behavior, sleeping disorders, and hepatic encephalopathy. Remarkably, early increased lymphocyte counts paralleled the exponential liver enzymes increase (Figure 3). Computed tomography scan revealed a partial response according to RECIST criteria, with a decrease of the mediastinum mass and pleural nodules (Figure 4).
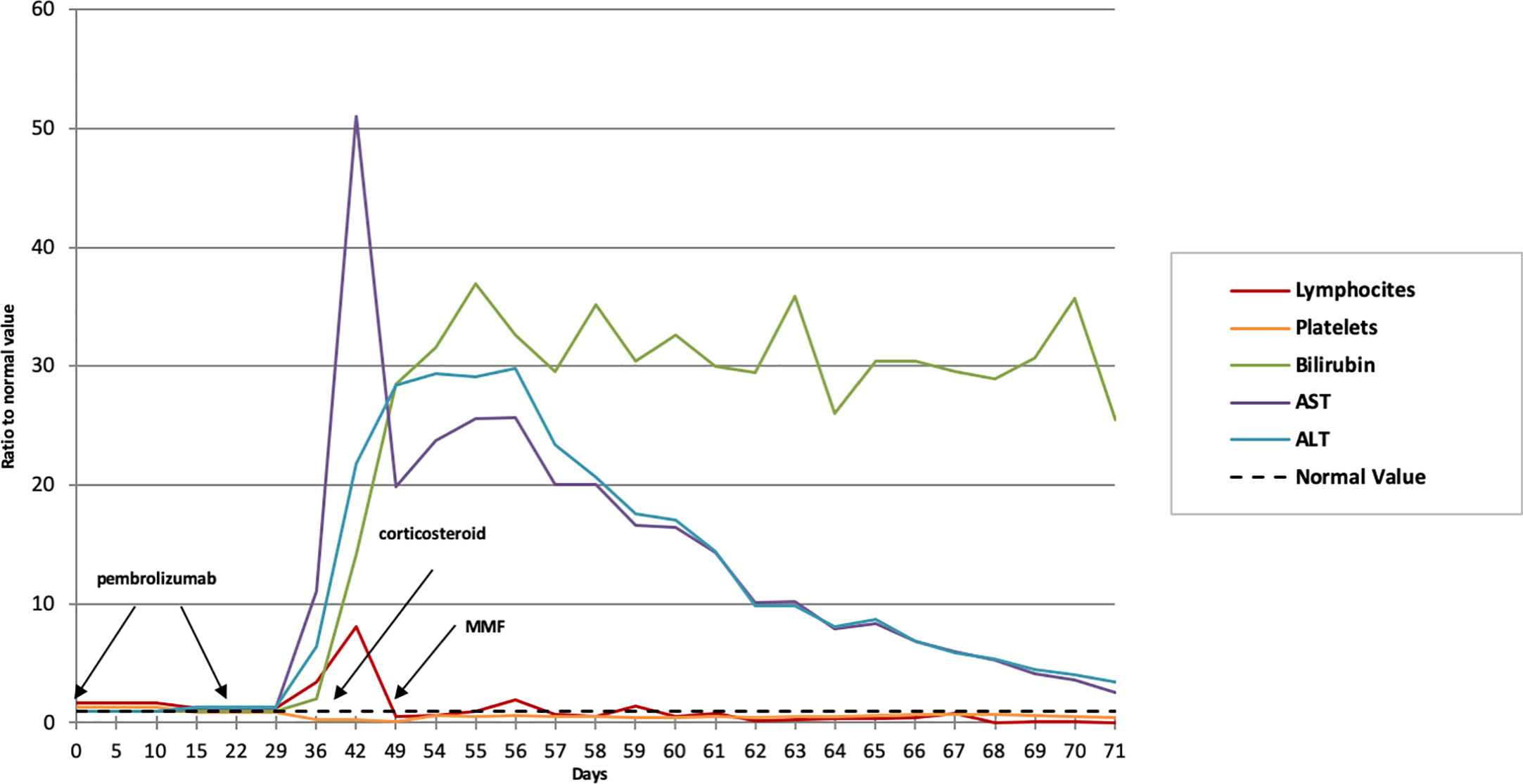
Figure 3 Biochemical and laboratory findings trend over time. The normal value (dotted line) refers to the unit, representative of the referral value to whom each fold change is referring to. MMF, mycophenolate mofetil.
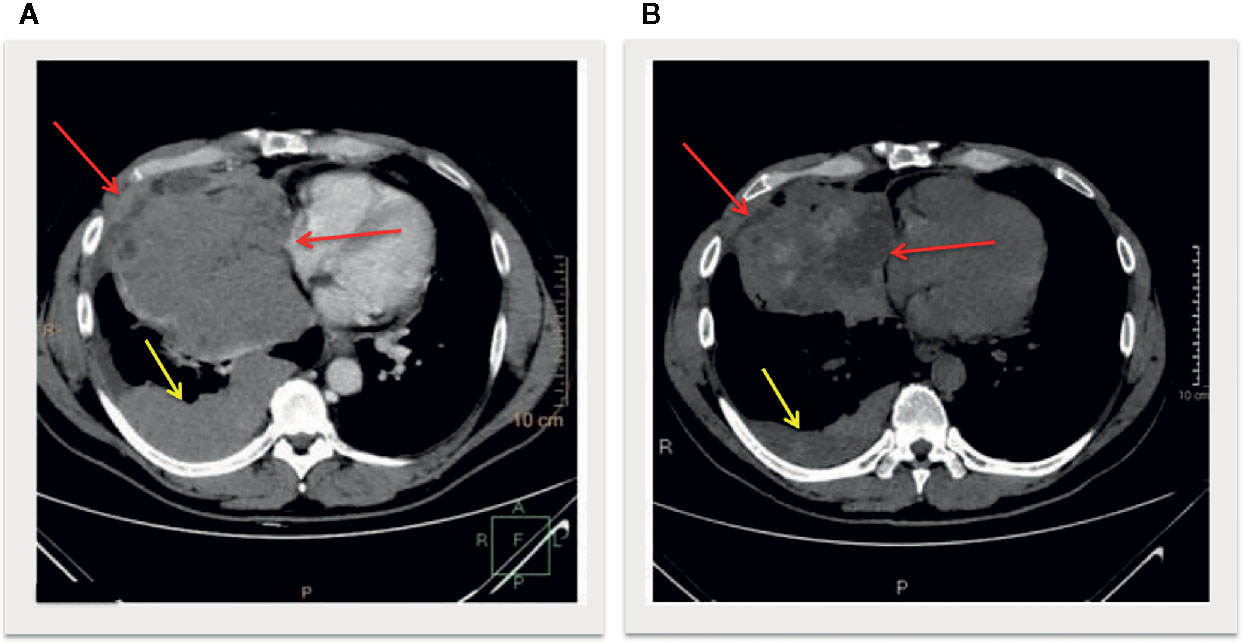
Figure 4 Longitudinal slices of computed tomography scans at baseline (A) and post treatment (B) showed a decrease of the mediastinum mass with intralesional hypondensity (red arrows) and pleural nodules (yellow arrows), defined as partial response according to iRECIST criteria.
On the assumption of an immune-related toxicity, a high-dose glucocorticoid regimen was administered (intravenous methylprednisolone 1 mg/kg/day, escalated up to 2 mg after 24 hours given the unsatisfactory laboratory improvement). Neither serologic evidence of acute active viral hepatitis nor ultrasound liver parenchyma alterations were detected, notwithstanding the fact that the patient harbored a seroconverted HBV positivity. Due to the severe thrombocytopenia, a liver biopsy could not be safely performed. Nonetheless, given the virologic history, entecavir 0.5 mg/die was administered. At 72 h after beginning steroid treatment, he started mycophenolate mofetil at 1,000 mg bis in die for progressive clinical deterioration. Selective bilirubin apheresis was required for worsening total bilirubin levels (44.36 mg/dl) and the appearance of psychomotor agitation. Despite these interventions, the patient developed a refractory hepatorenal syndrome and progressive fatal multisystem organ failure.
Discussion
By re-educating the immune response against tumors, outstanding outcomes have been achieved in challenging oncologic scenarios; nonetheless, the clinical efficacy has often paralleled an uncontrolled immune activation, directed against off-target body functions and districts (16−17).
Herein, we report an early and fatal immune-mediated of high-grade adverse events in a thymoma patient treated with Pembrolizumab, characterized by dramatic lymphocytosis (CD8+ T-cell predominant), thrombocytopenia, hepatitis, and thyroiditis paralleling the manifest anti-tumor activity.
Recently, the US Food and Drug Administration (FDA) approved the use of Pembrolizumab in pre-treated thymic carcinoma, based on efficacy (overall response rate 22.5%) and safety data from a phase II study (11). Six patients out of 41 (15%) developed high grade irAEs, including four patients with hepatitis, three patients with polymyositis, and two patients with myocarditis. No deaths were reported (11). Pembrolizumab approval was restricted to thymic carcinoma subtype, due to pilot case series reporting immune-related toxicity in thymoma patients (Supplementary Table 2) (4).
Indeed, a phase II clinical trial by Cho et al. reported seven patients with thymoma (four pts B2, one pt B2/B3, two pts B3) treated with intravenous Pembrolizumab at a fixed dose of 200 mg every 3 weeks (10). The disease control rate (DCR) was 100%, with 28.6% of partial responses; the median PFS was 6.1 months (4.3 to 7.9 months), with a median number of 8 cycles (1–25). The major reason for treatment discontinuation was grade 3 or 4 irAEs occurring in five patients (71.4%), four of them within the first two administrations. Remarkably, two patients with toxicity and preexisting myasthenia obtained a partial response after only one cycle of treatment. The most frequently observed severe irAEs (G3-4) were autoimmune myocarditis (three patients); hepatitis (two patients); nephritis, colitis, thyroiditis, and conjunctivitis (one patient each). Six patients recovered from irAEs with high-dose corticosteroids, intravenous immunoglobulin, and other immunosuppressive agents. One death occurred due to cytomegalovirus superinfection during immunosuppressive treatment for severe hepatitis and colitis (10).
Similarly, Rajan et al. reported safety and efficacy of PD-L1 inhibition in a phase I clinical trial (12). Seven patients with pretreated thymoma (one pt B1, three pts B2 and three pts B3) received intravenous Avelumab at a dose of 10 to 20 mg/kg every two weeks. DCR was 86%. Partial response was observed in four patients (57%), most of whom developed irAEs after just one cycle of immunotherapy. Three of seven patients (43%) with thymoma suffered grade 3–4 irAEs, including shortness of breath, myositis, diarrhea, hyperkalemia, and asymptomatic elevated levels of liver transaminases. The irAEs were treated with corticosteroid therapy, achieving a rapid improvement of symptoms (12).
Literature data include sporadic additional case reports describing thymoma patients treated with ICIs and fatal multi-organ failure including myocarditis and myasthenia gravis, highlighting a critical scenario in terms of efficacy and toxicity (14, 15). Indeed, the anti-tumoral effect of ICIs often mirrors the onset of severe irAEs due to still-unexplored reasons.
Notably, in their case series Rajan et al. reported a correlation between the absolute lymphocyte count at baseline and the ICI response (12). Our report confirms the previous evidence, showing lymphocytes at baseline twofold higher than the normal limits. To our knowledge the case presented, with clinical and laboratory evidence offers the first description on an overt lymphocytosis within an immune-storm of adverse events and tumor shrinkage. This outcome warrants further statistically powered investigation in order to better define the real impact of increased lymphocyte absolute numbers in predicting irAEs and clinical response in thymoma patients.
These findings are substantially extended, providing a deeper insight into the pivotal role played by immune phenotyping in thymoma patients who are candidates for ICI.
As a paradigmatic condition for cancer development within a composite immune ecosystem, the thymic gland deserves a peculiar pathophysiological consideration as the soil that nurses malignant cells (3, 19). This emblematic crosstalk might justify the hazardous equilibrium between immune-tolerance and autoimmune brakes and suffice to explain the clinically explosive side effects with multi-system involvement (20).
Indeed, this storm of immune-mediated toxicity is a biological likelihood. The thymus is a lymphatic organ with a key role in the regulation of the immunological balance. Maintaining self-tolerance passes through the control of the differentiation and positive/negative selection of immature T-cells (21, 22). In physiological conditions, immature T lymphocytes migrate from the cortex to the medullary region, where the maturation process is completed following an accurate selection process. Thus, immature CD4−CD8− (double negative) is transformed into CD4+CD8+ (double positive) cells in the cortex region and can mature into either CD4+ or CD8+ single positive in the thymic medulla (23). The ravaged structure in thymic neoplasia hinders negative selection to eliminate autoreactive T-cells, and immature thymic lymphocytes may escape quality control by dendritic cells. Moreover, in neoplastic thymic epithelium, the production of regulatory T-cells is impaired, as well as the expression of auto-immune regulators (AIREs), a crucial issue in immune tolerance (24). Thus, in thymoma patients the function of self-tolerance is lost, prompting autoimmune manifestations. Moreover, the compromised expression of HLA-DR molecules in neoplastic thymocytes increases levels of HLA-A24 and HLA-B8, which are associated with an enhanced likelihood of autoimmune manifestations (25–27). Remarkably, the emerging correlation between a given HLA and irAEs highlights the potential relevance of the genetic background in predicting both immune side effects (28) and clinical efficacy (29).
In this precarious balance, PD-1/PD-L1 blockade upsets the immunological scenario. Blocking PD-1/PD-L1 reverts cancer immunotolerance, inducing disinhibition of effector T-cells and breakdown of peripheral tolerance against self-cells. Indeed, in physiological conditions, PD-1 restrains TCR-mediated positive selection through the PD-1/PD-L1 crosstalk (30, 31). Moreover, blocking PD1/PD-L1 signaling fuels the loss of inhibition of effector T-cells (Teff). Therefore, Teff can, in turn, better induce epithelial and endothelial thymic apoptosis and necrosis, whilst disabling immunological self-tolerance (32–35).
Thus, it is reasonable to envisage a vicious cycle feeding a bidirectional behavior of ICI-mediated effects that drive the on-tumor efficacy as well as the off-target side effects (Figure 1).
Consistent with previous findings, the detection of an immune tolerogenic microenvironment early on when starting thymoma patients on ICI-based treatment holds the potential to gain enhanced tumor shrinkage. However, an immune-mediated storm might also take place, mirroring the ICI off-target effects while hindering the PD-1 dependent immune-brake.
In this patient, the subclinical HBV seroconversion might have played an additional role in mediating an enhanced off-target irAE affecting the liver parenchyma. Notoriously, chronic HBV-viral infection induces a complex repertoire shaping the immune-infiltrate, which could have predisposed our patient to experience an increased immune flare mediated by the hepatic CD4+CD8+PD1+ milieu (36–38). Specifically, in HBV infected patients the increased regulatory T-cell (Treg) levels within the liver halt the effector function of CD4+ and CD8+ lymphocytes, sustaining an immune-suppressive milieu. Since PD1 is a crucial inhibitory receptor, its blockade might revert T-cell exhaustion, unleash the immune response and potentially lead to the hepatic damage (36–38). Despite the lack of clear guidelines driving the clinical approach to HBV seroconverted patients with thymoma, it is reasonable to retrospectively consider antiviral prophylaxis as a reasonable approach for ICI-treatment candidates (39–41). Indeed, the lesson learned from extensively investigated HBV reactivation in alternative clinical scenarios (42, 43) can be translated to the ICI-based approach, warranting a careful risk assessment at baseline and taking into consideration anti-viral prophylaxis.
Overall, clinicians should be vigilant against irAE in thymoma patients treated with ICIs, due to its prompt onset and fulminant progression. Other evidence has already shown thyroid immune-mediated damage to be an early side effect preceding and paralleling the autoimmune phenomena (44, 45). We confirm the above-mentioned clinical behavior. It is therefore tempting to speculate about the role of laboratory evaluation with full blood cell count, liver function assessment, and a full endocrine panel in making repeated indirect snapshots in order to avert the dynamic immune-related toxicity onset (46, 47). Thus, it is mandatory to better refine the druggable focuses, decreasing the incidence of undesired effects exerted by immune-system modulation and novel bullets striking the non-cancerous neighborhood. The rarity and heterogeneity of thymoma subtypes pose an objective difficulty in developing robust clinical trials to investigate efficacy and safety in this subset of TETs. In this framework, promising phase I–II clinical trials evaluating the safety and efficacy of ICIs in thymoma patients (NTC03076554, NCT03134118, NCT03295227) are ongoing.
Ethics Statement
Written informed consent was obtained from the individual(s) for the publication of any potentially identifiable images or data included in this article.
Author Contributions
AA, AS, and MG conceptualized the study. AA, ML, VU, AN, SF, SS, DP, and AZ contributed to the data curation. AS acquired the funding. MG supervised the study. AA, AS, ML, and VU wrote the original draft of the manuscript. MG wrote, reviewed, and edited the manuscript. All authors contributed to the article and approved the submitted version.
Funding
This research project was also supported in part by the Apulian Regional Project “Medicina di Precisione” to AS.
Conflict of Interest
The authors declare that the research was conducted in the absence of any commercial or financial relationships that could be construed as a potential conflict of interest.
Acknowledgments
The authors thank Mary Victoria Pragnell, BA, at the School of Medicine and Surgery at the University of Bari Aldo Moro, for linguistic revision. The authors acknowledge the Smart Servier Medical Art (http://smart.servier.com/) for providing comprehensive medical and biological figures and datasets that are fruitful for the international scientific community.
Supplementary Material
The Supplementary Material for this article can be found online at: https://www.frontiersin.org/articles/10.3389/fonc.2020.583781/full#supplementary-material
Supplementary Figure 1 | Immunophenotypic characterization of lymphocytes in the peripheral blood. The lymphocyte population is identified as low SSC and bright CD45 (region “LYMPHOCYTES”) in the CD45 vs SSC dot plot. The percentages refer to the “LYMPHOCYTES” gated population. CD3+, T lymphocytes; CD19+, B lymphocytes; CD56+ CD16+ CD3−, NK lymphocytes; CD3+ CD56+, NKT lymphocytes; CD3+ CD4+, T helper lymphocytes; CD3+ CD8+, T cytotoxic lymphocytes. NK, natural killer.
References
1. Girard N, Ruffini E, Marx A, Faivre-Finn C, Peters S. Thymic epithelial tumours: ESMO Clinical Practice Guidelines for diagnosis, treatment and follow-up. Ann Oncol (2015) 26:v40–55. doi: 10.1093/annonc/mdv277
2. Hsu C-H, Chan JK, Yin C-H, Lee C-C, Chern C-U, Liao C-I. Trends in the incidence of thymoma, thymic carcinoma, and thymic neuroendocrine tumor in the United States. PLoS One (2019) 14:e0227197. doi: 10.1371/journal.pone.0227197
3. Shelly S, Agmon-Levin N, Altman A, Shoenfeld Y. Thymoma and autoimmunity. Cell Mol Immunol (2011) 8:199–202. doi: 10.1038/cmi.2010.74
4. Yokoyama S, Miyoshi H. Thymic tumors and immune checkpoint inhibitors. J Thorac Dis (2018) 10:S1509–15. doi: 10.21037/jtd.2017.10.157
5. Hoffacker V, Schultz A, Tiesinga JJ, Gold R, Schalke B, Nix W, et al. Thymomas alter the T-cell subset composition in the blood: a potential mechanism for thymoma-associated autoimmune disease. Blood (2000) 96:3872–9. doi: 10.1182/blood.V96.12.3872.h8003872_3872_3879
6. Tachdjian R, Keller JJ, Pfeffer M. A Bad Case of Good’s Syndrome. Infect Dis Ther (2014) 3:333–7. doi: 10.1007/s40121-014-0045-7
7. Vacca A, Melaccio A, Sportelli A, Solimando AG, Dammacco F, Ria R. Subcutaneous immunoglobulins in patients with multiple myeloma and secondary hypogammaglobulinemia: a randomized trial. Clin Immunol (Orlando Fla) (2018) 191:110–5. doi: 10.1016/j.clim.2017.11.014
8. Kondo K, Monden Y. Therapy for thymic epithelial tumors: a clinical study of 1,320 patients from Japan. Ann Thorac Surg (2003) 76:878–84. doi: 10.1016/S0003-4975(03)00555-1
9. Radovich M, Pickering CR, Felau I, Ha G, Zhang H, Jo H, et al. The Integrated Genomic Landscape of Thymic Epithelial Tumors. Cancer Cell (2018) 33:244–58.e10. doi: 10.1016/j.ccell.2018.01.003
10. Cho J, Kim HS, Ku BM, Choi Y-L, Cristescu R, Han J, et al. Pembrolizumab for Patients With Refractory or Relapsed Thymic Epithelial Tumor: An Open-Label Phase II Trial. J Clin Oncol (2019) 37:2162–70. doi: 10.1200/JCO.2017.77.3184
11. Giaccone G, Kim C, Thompson J, McGuire C, Kallakury B, Chahine JJ, et al. Pembrolizumab in patients with thymic carcinoma: a single-arm, single-centre, phase 2 study. Lancet Oncol (2018) 19:347–55. doi: 10.1016/S1470-2045(18)30062-7
12. Rajan A, Heery CR, Thomas A, Mammen AL, Perry S, O’Sullivan Coyne G, et al. Efficacy and tolerability of anti-programmed death-ligand 1 (PD-L1) antibody (Avelumab) treatment in advanced thymoma. J Immunother Cancer (2019) 7:269. doi: 10.1186/s40425-019-0723-9
13. Szuchan C, Elson L, Alley E, Leung K, Camargo AL, Elimimian E, et al. Checkpoint inhibitor-induced myocarditis and myasthenia gravis in a recurrent/metastatic thymic carcinoma patient: a case report. Eur Heart J Case Rep (2020) 4:1–8. doi: 10.1093/ehjcr/ytaa051
14. Konstantina T, Konstantinos R, Anastasios K, Anastasia M, Eleni L, Ioannis S, et al. Fatal adverse events in two thymoma patients treated with anti-PD-1 immune check point inhibitor and literature review. Lung Cancer (2019) 135:29–32. doi: 10.1016/j.lungcan.2019.06.015
15. Hyun J-W, Kim GS, Kim S-H, Cho J-Y, Kim HJ, Lee GK, et al. Fatal Simultaneous Multi-organ Failure Following Pembrolizumab Treatment for Refractory Thymoma. Clin Lung Cancer (2020) 21:e74–7. doi: 10.1016/j.cllc.2019.10.008
16. Postow MA, Sidlow R, Hellmann MD. Immune-Related Adverse Events Associated with Immune Checkpoint Blockade. N Engl J Med (2018) 378:158–68. doi: 10.1056/NEJMra1703481
17. Wang DY, Salem J-E, Cohen JV, Chandra S, Menzer C, Ye F, et al. Fatal Toxic Effects Associated With Immune Checkpoint Inhibitors: A Systematic Review and Meta-analysis. JAMA Oncol (2018) 4:1721. doi: 10.1001/jamaoncol.2018.3923
18. Khoja L, Day D, Wei-Wu Chen T, Siu LL, Hansen AR. Tumour- and class-specific patterns of immune-related adverse events of immune checkpoint inhibitors: a systematic review. Ann Oncol (2017) 28:2377–85. doi: 10.1093/annonc/mdx286
19. Weksler B, Lu B. Alterations of the Immune System in Thymic Malignancies. J Thorac Oncol (2014) 9:S137–42. doi: 10.1097/JTO.0000000000000299
20. Zhao C, Rajan A. Immune checkpoint inhibitors for treatment of thymic epithelial tumors: how to maximize benefit and optimize risk? Mediastinum Hong Kong China (2019) 3:35. doi: 10.21037/med.2019.08.02
21. Thapa P, Farber DL. The Role of the Thymus in the Immune Response. Thorac Surg Clin (2019) 29:123–31. doi: 10.1016/j.thorsurg.2018.12.001
22. Takahama Y, Ohigashi I, Baik S, Anderson G. Generation of diversity in thymic epithelial cells. Nat Rev Immunol (2017) 17:295–305. doi: 10.1038/nri.2017.12
23. Germain RN. T-cell development and the CD4-CD8 lineage decision. Nat Rev Immunol (2002) 2:309–22. doi: 10.1038/nri798
24. Cheng M, Anderson MS. Thymic tolerance as a key brake on autoimmunity. Nat Immunol (2018) 19:659–64. doi: 10.1038/s41590-018-0128-9
25. Yang H, Hao J, Peng X, Simard AR, Zhang M, Xie Y, et al. The association of HLA-DQA1*0401 and DQB1*0604 with thymomatous myasthenia gravis in northern Chinese patients. J Neurol Sci (2012) 312(1-2):57–61. doi: 10.1016/j.jns.2011.08.023
26. Massa R, Greco G, Testi M, Rastelli E, Terracciano C, Frezza E, et al. Thymomatous myasthenia gravis: novel association with HLA DQB1*05:01 and strengthened evidence of high clinical and serological severity. J Neurol (2019) 266(4):982–9. doi: 10.1007/s00415-019-09225-z
27. Deitiker PR, Oshima M, Smith RG, Mosier D, Atassi MZ. Association with HLA DQ of early onset myasthenia gravis in Southeast Texas region of the United States. Int J Immunogenet (2011) 38(1):55–62. doi: 10.1111/j.1744-313X.2010.00979.x
28. Hasan Ali O, Berner F, Bomze D, Fässler M, Diem S, Cozzio A, et al. Human leukocyte antigen variation is associated with adverse events of checkpoint inhibitors. Eur J Cancer Oxf Engl 1990 (2019) 107:8–14. doi: 10.1016/j.ejca.2018.11.009
29. Chowell D, Morris LGT, Grigg CM, Weber JK, Samstein RM, Makarov V, et al. Patient HLA class I genotype influences cancer response to checkpoint blockade immunotherapy. Science (2018) 359:582–7. doi: 10.1126/science.aao4572
30. Nishimura H, Honjo T, Minato N. Facilitation of beta selection and modification of positive selection in the thymus of PD-1-deficient mice. J Exp Med (2000) 191:891–8. doi: 10.1084/jem.191.5.891
31. Keir ME, Latchman YE, Freeman GJ, Sharpe AH. Programmed death-1 (PD-1):PD-ligand 1 interactions inhibit TCR-mediated positive selection of thymocytes. J Immunol Baltimore Md 1950 (2005) 175:7372–9. doi: 10.4049/jimmunol.175.11.7372
32. Amarnath S, Mangus CW, Wang JCM, Wei F, He A, Kapoor V, et al. The PDL1-PD1 axis converts human TH1 cells into regulatory T cells. Sci Transl Med (2011) 3:111ra120. doi: 10.1126/scitranslmed.3003130
33. Anderson G, Takahama Y. Thymic epithelial cells: working class heroes for T cell development and repertoire selection. Trends Immunol (2012) 33:256–63. doi: 10.1016/j.it.2012.03.005
34. Schaaf MB, Garg AD, Agostinis P. Defining the role of the tumor vasculature in antitumor immunity and immunotherapy. Cell Death Dis (2018) 9:115. doi: 10.1038/s41419-017-0061-0
35. Yan Y, Kumar AB, Finnes H, Markovic SN, Park S, Dronca RS, et al. Combining Immune Checkpoint Inhibitors With Conventional Cancer Therapy. Front Immunol (2018) 9:1739. doi: 10.3389/fimmu.2018.01739
36. Stoop JN, van der Molen RG, Baan CC, van der Laan LJW, Kuipers EJ, Kusters JG, et al. Regulatory T cells contribute to the impaired immune response in patients with chronic hepatitis B virus infection. Hepatology (Baltimore Md) (2005) 41:771–8. doi: 10.1002/hep.20649
37. Boni C, Fisicaro P, Valdatta C, Amadei B, Di Vincenzo P, Giuberti T, et al. Characterization of hepatitis B virus (HBV)-specific T-cell dysfunction in chronic HBV infection. J Virol (2007) 81:4215–25. doi: 10.1128/JVI.02844-06
38. Pu D, Yin L, Zhou Y, Li W, Huang L, Cai L, et al. Safety and efficacy of immune checkpoint inhibitors in patients with HBV/HCV infection and advanced-stage cancer: A systematic review. Med (Baltimore) (2020) 99:e19013. doi: 10.1097/MD.0000000000019013
39. Lombardi A, Mondelli MU. Review article: immune checkpoint inhibitors and the liver, from therapeutic efficacy to side effects. Aliment Pharmacol Ther (2019) 50:872–84. doi: 10.1111/apt.15449
40. Lampertico P, Agarwal K, Berg T, Buti M, Janssen HLA, Papatheodoridis G, et al. EASL 2017 Clinical Practice Guidelines on the management of hepatitis B virus infection. J Hepatol (2017) 67:370–98. doi: 10.1016/j.jhep.2017.03.021
41. Terrault NA, Lok ASF, McMahon BJ, Chang K-M, Hwang JP, Jonas MM, et al. Update on prevention, diagnosis, and treatment of chronic hepatitis B: AASLD 2018 hepatitis B guidance. Hepatology (2018) 67:1560–99. doi: 10.1002/hep.29800
42. Evens AM, Jovanovic BD, Su Y-C, Raisch DW, Ganger D, Belknap SM, et al. Rituximab-associated hepatitis B virus (HBV) reactivation in lymphoproliferative diseases: meta-analysis and examination of FDA safety reports. Ann Oncol (2011) 22:1170–80. doi: 10.1093/annonc/mdq583
43. Solimando AG, Ribatti D, Vacca A, Einsele H. Targeting B-cell non Hodgkin lymphoma: New and old tricks. Leuk Res (2016) 42:93–104. doi: 10.1016/j.leukres.2015.11.001
44. Sakakida T, Ishikawa T, Uchino J, Chihara Y, Komori S, Asai J, et al. Clinical features of immune-related thyroid dysfunction and its association with outcomes in patients with advanced malignancies treated by PD-1 blockade. Oncol Lett (2019) 18:2140–7. doi: 10.3892/ol.2019.10466
45. Barroso-Sousa R, Barry WT, Garrido-Castro AC, Hodi FS, Min L, Krop IE, et al. Incidence of Endocrine Dysfunction Following the Use of Different Immune Checkpoint Inhibitor Regimens: A Systematic Review and Meta-analysis. JAMA Oncol (2018) 4:173–82. doi: 10.1001/jamaoncol.2017.3064
46. von Itzstein MS, Khan S, Gerber DE. Investigational Biomarkers for Checkpoint Inhibitor Immune-Related Adverse Event Prediction and Diagnosis. Clin Chem (2020) 66:779–93. doi: 10.1093/clinchem/hvaa081
Keywords: thymoma, immune-microenvironment, immunotherapy, pembrolizumab, lymphocytosis, hepatitis, immune related adverse events
Citation: Argentiero A, Solimando AG, Ungaro V, Laforgia M, Strippoli S, Pinto D, Negri A, Ferraiuolo S, Zito A and Guida M (2020) Case Report: Lymphocytosis Associated With Fatal Hepatitis in a Thymoma Patient Treated With Anti-PD1: New Insight Into the Immune-Related Storm. Front. Oncol. 10:583781. doi: 10.3389/fonc.2020.583781
Received: 23 July 2020; Accepted: 04 November 2020;
Published: 14 December 2020.
Edited by:
Valli De Re, Aviano Oncology Reference Center (IRCCS), ItalyReviewed by:
Anne Bass, Hospital for Special Surgery, United StatesMaria Lina Tornesello, Istituto Nazionale Tumori Fondazione G. Pascale (IRCCS), Italy
Copyright © 2020 Argentiero, Solimando, Ungaro, Laforgia, Strippoli, Pinto, Negri, Ferraiuolo, Zito and Guida. This is an open-access article distributed under the terms of the Creative Commons Attribution License (CC BY). The use, distribution or reproduction in other forums is permitted, provided the original author(s) and the copyright owner(s) are credited and that the original publication in this journal is cited, in accordance with accepted academic practice. No use, distribution or reproduction is permitted which does not comply with these terms.
*Correspondence: Antonio Giovanni Solimando, antonio.solimando@uniba.it; Antonella Argentiero, argentieroantonella@gmail.com
†These authors have contributed equally to this work