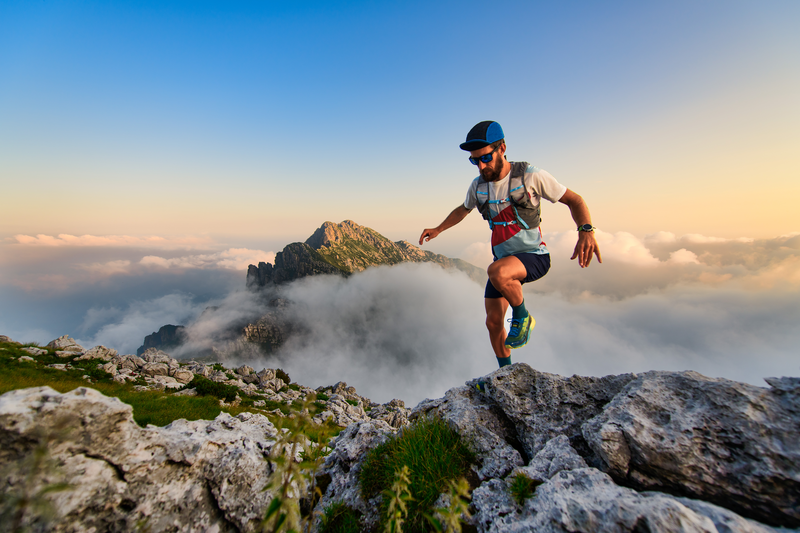
94% of researchers rate our articles as excellent or good
Learn more about the work of our research integrity team to safeguard the quality of each article we publish.
Find out more
MINI REVIEW article
Front. Oncol. , 21 October 2020
Sec. Molecular and Cellular Oncology
Volume 10 - 2020 | https://doi.org/10.3389/fonc.2020.570815
This article is part of the Research Topic Molecular Mechanisms and Treatment of MYCN-driven Tumors View all 14 articles
Asymmetric cell division (ACD) is an important physiological event in the development of various organisms and maintenance of tissue homeostasis. ACD produces two different cells in a single cell division: a stem/progenitor cell and differentiated cell. Although the balance between self-renewal and differentiation is precisely controlled, disruptions to ACD and/or enhancements in the self-renewal division (symmetric cell division: SCD) of stem cells resulted in the formation of tumors in Drosophila neuroblasts. ACD is now regarded as one of the characteristics of human cancer stem cells, and is a driving force for cancer cell heterogeneity. We recently reported that MYCN controls the balance between SCD and ACD in human neuroblastoma cells. In this mini-review, we discuss the mechanisms underlying MYCN-mediated cell division fate.
Neuroblastoma is a common cancer in children and exhibits a broad clinical behavior (1–3). Patients are classified into low-, intermediate-, and high-risk groups based on clinical and biological characteristics (1–3). Minimal treatment may be sufficient for the low-risk group, whereas despite intensive treatment, high-risk patients still present with a dismal outcome. The reasons for this heterogeneity remained unclear until molecular, genetic, and biochemical analyses of tumors provided insights into their different clinical behaviors. Among the many genetic and biochemical features of neuroblastoma, amplification of the MYCN oncogene correlates with an aggressive phenotype and poor prognosis (1–3). Approximately 20% of neuroblastomas show MYCN gene amplification. Recent studies reported that MYCN not only exhibited oncogenic activity, but also played a central role in normal neural stem and progenitor cell self-renewal (4–6).
Neuroblastoma originates from cells of the neural crest, which is a multipotent cell population comprising the embryonic structure (7). The neural crest is composed of migrating cell populations that give rise to diverse cell lineages, including Schwann cells, melanocytes, craniofacial cartilage and bones, smooth muscle, peripheral and intestinal neurons, and glia. Thus, the neural crest acts as pluripotent stem cells that differentiate into mature peripheral nerve tissue. The pluripotent neural crest is suspected to be involved in the tumorigenesis of neuroblastoma due to the abnormal expression of MYCN. Neuroblastoma cells are derived from the pluripotent neural crest that has cancer stem cell-like properties (8). Therefore, human neuroblastoma cultured cells exhibit both proliferative and differentiating abilities, and possess similar characteristics to cancer stem cells (9, 10).
Cancer stem cells are considered to undergo asymmetric cell division (ACD), a physiological event resulting in tumor cell heterogeneity (11, 12). ACD is a strategy that maintains the correct number of self-renewing stem cells and differentiated cells in a single division. Therefore, ACD balances the stem cell pool with its progenitor pool. Recent studies revealed that the misregulation of this balance between self-renewal and differentiation by ACD led to the emergence of abnormal stem cells, resulting in tumorigenesis in Drosophila neuroblast populations (13). Therefore, cancer stem cells may use ACD as a strategy to generate more cancer stem cells in addition to many differentiated cancer cells. We herein investigated the mechanisms underlying ACD using a series of human neuroblastoma cultured cells as a model system (14–16).
Asymmetric cell division studies were originally conducted using model organisms, such as nematode embryos (17, 18), Drosophila neuroblasts (13), and Drosophila germ stem cells (19). The findings of these genetic studies revealed that the mechanism of ACD is highly conserved. Previous studies demonstrated the ACD of stem cells in muscle (20), skin (21), the gut (22), mammary glands (23), the hematopoietic system (24), and the developing mouse brain (25, 26). Comparisons of ACD studies using these organisms and model systems revealed ACD in neuroblastoma cells in an evolutionarily conserved manner (14). The magnitude of MYCN gene expression influences the regulation of cell division fate. The overexpression of MYCN induces symmetric cell division (SCD) (self-renewal division), and the decreased expression of MYCN causes ACD (14). Furthermore, the transcriptional activity of MYCN is important for inducing SCD in human neuroblastoma cells (14). Although the specific transcriptional target(s) of MYCN currently remain unclear, except the high mobility group A1 (HMGA1) oncogene, several key molecular pathways involved in MYCN-mediated cell division fate have been identified (Figure 1).
Figure 1. Molecular pathways of MYCN-mediated cell division fate. MYCN protein stability depends on the interaction partners. The receptor type-tyrosine kinase, ALK, activates downstream targets, such as PI-3K, and eventually activates AKT. Activated AKT stabilizes the MYCN protein by inhibiting GSK-3β. NCYM also stabilizes MYCN for inhibition of the GSK3β-MYCN interaction. MYCN induces the expression of NCYM and HMGA1. OCT4 and MYCN or ALDH18A1 and MYCN form a positive feedback loop for their transcriptional expression. HMGA1 inhibits the expression of NUMB and p53. On the other hand, LIN28B inhibits the microRNA, let-7, and contributes to the stability of the MYCN and AURKA (Aurora-A) protein. AURKA and PLK1 also stabilize the MYCN protein to inhibit Fbxw7-dependent MYCN ubiquitination. Thus, many oncogenic proteins contribute to the stability of MYCN. As a result, MYCN-dependent tumor cells display symmetric cell division (SCD) and the degradation of MYCN causes asymmetric cell division (ACD).
Tripartite motif-containing 32 (TRIM32) was identified as an ACD inducer in human neuroblastoma cells (15). Previous studies established TRIM32, an ortholog of Drosophila melanogaster, Brat, which participates in ACD as a neural determinant and inhibits Drosophila MYC (dMYC) function in the neuroblasts of fly (27). In addition, mouse TRIM32 was shown to exhibit ubiquitin ligase activity, and facilitated the degradation of the c-MYC oncoprotein in neurogenesis (28). However, the functions of TRIM32 in human cancers remain largely unknown. We recently reported that TRIM32 promoted the proteasomal degradation of MYCN at spindle poles during cell division, while TRIM32 overexpression induced ACD in human neuroblastoma cells (Figures 1, 2) (15). TRIM3, another ortholog of D. melanogaster, Brat, is frequently deleted in human glioblastoma (29). Moreover, TRIM3 has been shown to facilitate the degradation of c-MYC and regulate ACD in human glioma cells (29). Thus, TRIM32/TRIM3 may not only induce ACD, but also function as a tumor suppressor in human tumors.
Figure 2. Molecular mechanism underlying TRIM32-mediated asymmetric cell division. During metaphase, TRIM32 is recruited to spindle poles (mitotic centrosomes) by CDK1/cyclin B signaling. In parallel, MYCN accumulates at spindle poles through GSK-3β signaling. Thus, TRIM32 associates with MYCN at spindle poles during metaphase. TRIM32 then facilitates the ubiquitination and degradation of MYCN by a proteasome complex at spindle poles. During anaphase, TRIM32 asymmetrically localizes to the one of the daughter cells without the NuMA crescent.
NCYM (MYCNOS) is a de novo evolutionary cis-antisense gene for MYCN that encodes a 109-amino acid small protein and only exists in humans and chimpanzees (30). NCYM induces the expression of not only MYCN, LIN28B, NANOG, and SOX2, but also OCT4, a MYCN-mediated core reprogramming factor (31). MYCN and OCT4 form a positive feedback loop (Figure 1) (31). A previous study reported that NCYM promoted malignant transformation and metastasis in NCYM/MYCN double transgenic mice (30). These findings indicated that MYCN cooperates with NCYM to promote the malignant transformation of neuroblastoma and its stemness. NCYM was shown to suppress the degradative activity of GSK3β against MYCN and facilitated the induction of SCD, while the knockdown of NCYM destabilized the MYCN protein and caused ACD (Figure 1) (31). On the other hand, NCYM also functions as a non-coding RNA and cooperates with CTCF to promote the progression of neuroblastoma by facilitating the expression of MYCN (32). Since the NCYM protein has some homology with the OCT4 protein (Figure 3), NCYM may function as a transcription factor in addition to non-coding RNA.
Figure 3. The NCYM protein has weak homology with the OCT4 protein. (A) Amino acid comparisons between the NCYM and OCT4 proteins. Identities are 24% and positives are 40%. (B) Molecular structure of the OCT4 protein. The OCT4 protein consists of four domains: the N-terminal transactivation domain (N-TAD), C-terminal transactivation domain (C-TAD), POU transcription factor-specific domain (POUs), and POU transcription factor homeodomain (POUHD). OCT4 shares weak homology with NCYM around the N-TAD and POUs domains.
Aldehyde dehydrogenase family 18 member A1 (ALDH18A1) was originally identified as the key enzyme for the synthesis of proline from glutamate, which catalyzes the coupled phosphorylation and reduction conversion of glutamate to β-pyrroline-5-carboxylate (P5C) and plays a critical role in regulating glutamine metabolism (33). A recent study revealed that ALDH18A1 formed a positive feedback loop with MYCN and was involved in the malignant transformation of neuroblastoma cells (Figure 1) (34). These findings demonstrated that the overexpression of ALDH18A1 decreased the rate of ACD and induced SCD, whereas the knockdown of ALDH18A1 increased the rate of ACD (34). Furthermore, molecular docking was applied to screen ALDH18A1 inhibitors, and the findings obtained showed that one compound, termed YG1702, from the approximately >200,000 compounds tested specifically inhibited the function of ALDH18A1 (34). Therefore, YG1702 has potential as a therapeutic drug that induces ACD and reduces the malignant transformation of MYCN-amplified neuroblastoma cells.
The important transcriptional target of MYCN in neuroblastoma is the high mobility group A1 (HMGA1) oncogene (35) (Figure 1). The HMGA1 protein is an architectural chromatin protein that is abundantly expressed during embryonic development and in most cancer tissues, but is weakly expressed or absent in normal adult tissues. It is important as an additional potential mechanism by which MYCN may induce the SCD of neuroblastoma stem cells. HMGA1 has been shown to induce the SCD of cancer stem cells by negatively regulating the expression of NUMB (36) or p53 (37) (Figure 1). NUMB is a cell fate determinant, and its expression is the basis for achieving the ACD of stem cells and its expression is either lost or reduced in many tumors (38). In Drosophila neuroblasts, NUMB mutations induce the formation of tumors (13). The NUMB protein contributes to the stabilization of p53 by suppressing the effects of HDM2, which is an E3-ubiquitin ligase (39). The deletion of p53 in mammary stem cells was shown to abolish NUMB asymmetry during cell division (23). These findings indicate that p53 and NUMB work in concert with ACD; however, the underlying mechanisms are not yet known in neuroblastoma cells.
Mitotic kinases, such as Aurora kinase A (AURKA) and Polo-like kinase 1 (PLK1), are reported to stabilize MYCN by inhibiting the Fbxw7-mediated degradation of the MYCN protein (40–42) and may promote SCD in MYCN-amplified neuroblastoma cells (Figure 1). AURKA and PLK1 are up-regulated by MYCN and are frequently overexpressed in MYCN-amplified neuroblastomas (40, 43). On the other hand, AURKA and Polo (Drosophila ortholog of PLK1) were shown to be necessary for asymmetric protein localization during mitosis in model organisms, such as a Drosophila external sensory organ (44), and functioned as tumor suppressors in Drosophila neuroblasts (45, 46). Therefore, AURKA- and PLK1-mediated cell division fates (ACD or SCD) may be context-dependent.
The LIN28B gene encodes a developmentally regulated RNA binding protein and is a key repressor of the let-7 family of miRNAs, which act as potent tumor suppressors by post-transcriptionally repressing multiple oncogenic targets, including MYCN (Figure 1) (47). In neuroblastoma cells, LIN28B promotes AURKA expression (48) and increases MYCN expression by repressing let-7 miRNAs (47). Since LIN28B is involved in SCD in Drosophila intestine stem cells (49) and Caenorhabditis elegans embryos (50), it may control the cell division fate in neuroblastoma cells in an evolutionarily conserved manner.
Why do neuroblastoma cultured cells exhibit ACD? As discussed above, many cultured neuroblastoma cells show the unique characteristics of proliferation and differentiation capabilities (9, 10). Previous studies demonstrated that human neuroblastoma cell lines may be classified into three distinct cellular phenotypes with different differentiation potentials: the sympathoadrenal neuroblast type (N-type), substrate adherent type (S-type), and intermediate type (I-type) (9, 10). Based on its morphological and growth characteristics, the I-type was recognized as a neuroblastoma stem cell by its unique differentiation and malignant potentials (9, 10). In addition, neuroblastoma is a typical childhood cancer that may arise at the fetal development stage when a large number of stem cells exhibit ACD.
In this mini-review, we discussed a group of molecules that are involved in ACD and SCD through the regulation of the MYCN protein. Since the major function of MYCN is as a transcription factor, further studies are needed to clarify whether HMGA1, a target of MYCN, is involved in the cell division fate of human neuroblastoma cells.
Since this mini-review mainly described the intrinsic factors of ACD, further studies are needed on extrinsic factors including the tumor microenvironment. The findings obtained may contribute to direct applications for therapeutic strategies.
Therefore, human neuroblastoma cultured cells have potential as a very useful model system for providing insights into the mechanisms underlying ACD.
HI performed a literature review and submitted the manuscript to the journal. HI, YK, and AN wrote the manuscript. All authors contributed to the article and approved the submitted version.
This article was financially supported by the Japan Society for the Promotion of Science (18K07223), Takeda Research Support, Japan, and Gold Ribbon Network, Japan.
The authors declare that the research was conducted in the absence of any commercial or financial relationships that could be construed as a potential conflict of interest.
ACD, asymmetric cell division; ALDH18A1, Aldehyde dehydrogenase family 18 member A1; ALK, Anaplastic lymphoma kinase; AURKA, Aurora kinase A; Brat, brain tumor; CDK1, cyclin dependent kinase 1; C-TAD, C-terminal transactivation domain; CTCF, CCCTC-binding factor; Fbxw7, F-box and WD repeat domain-containing 7; GSK3β, glycogen synthase kinase 3 β; HMGA1, high mobility group A1; MYCNOS, MYCN opposite strand; N-TAD, N-terminal transactivation domain; NuMA, Nuclear mitotic apparatus; OCT4, Octamer-binding transcription factor 4; PI-3K, Phosphoinositide 3-kinase; PLK1, Polo-like kinase 1; POUHD, POU transcription factor homeodomain; POUs, POU transcription factor-specific domain; SCD, symmetric cell division; TRIM3, tripartite motif-containing 3; TRIM32, tripartite motif-containing 32.
1. Brodeur GM. Neuroblastoma: biological insights into a clinical enigma. Nat Rev Cancer. (2003) 3:203–16. doi: 10.1038/nrc1014
2. Matthay KK, Maris JM, Schleiermacher G, Nakagawara A, Mackall CL, Diller L, et al. Neuroblastoma. Nat Rev Dis Primers. (2016) 2:16078.
3. Nakagawara A, Li Y, Izumi H, Muramori K, Inada H, Nishi M. Neuroblastoma. Jpn J Clin Oncol. (2018) 48:214–41.
4. Kerosuo L, Neppala P, Hsin J, Mohlin S, Vieceli FM, Torok Z, et al. Enhanced expression of MycN/CIP2A drives neural crest toward a neural stem cell-like fate: implications for priming of neuroblastoma. Proc Natl Acad Sci USA. (2018) 115:E7351–60.
5. Zhang JT, Weng ZH, Tsang KS, Tsang LL, Chan HC, Jiang XH. MycN is critical for the maintenance of human embryonic stem cell-derived neural crest stem cells. PLoS One. (2016) 11:e0148062. doi: 10.1371/journal.pone.0148062
6. Huang M, Weiss WA. Neuroblastoma and MYCN. Cold Spring Harb Perspect Med. (2013) 3:a014415. doi: 10.1101/cshperspect.a014415
7. De Preter K, Vandesompele J, Heimann P, Yigit N, Beckman S, Schramm A, et al. Human fetal neuroblast and neuroblastoma transcriptome analysis confirms neuroblast origin and highlights neuroblastoma candidate genes. Genome Biol. (2006) 7:R84. doi: 10.1186/gb-2006-7-9-r84
8. Cotterman R, Knoepfler PS. N-Myc regulates expression of pluripotency genes in neuroblastoma including lif, klf2, klf4, and lin28b. PLoS One. (2009) 4:e5799. doi: 10.1371/journal.pone.0005799
9. Ross RA, Spengler BA. Human neuroblastoma stem cells. Semin Cancer Biol. (2007) 17:241–7. doi: 10.1016/j.semcancer.2006.04.006
11. Morrison SJ, Kimble J. Asymmetric and symmetric stem-cell divisions in development and cancer. Nature. (2006) 441:1068–74. doi: 10.1038/nature04956
12. Meacham CE, Morrison SJ. Tumour heterogeneity and cancer cell plasticity. Nature. (2013) 501:328–37. doi: 10.1038/nature12624
13. Caussinus E, Gonzalez C. Induction of tumor growth by altered stem-cell asymmetric division in Drosophila melanogaster. Nat Genet. (2005) 37:1125–9. doi: 10.1038/ng1632
14. Izumi H, Kaneko Y. Evidence of asymmetric cell division and centrosome inheritance in human neuroblastoma cells. Proc Natl Acad Sci USA. (2012) 109:18048–53. doi: 10.1073/pnas.1205525109
15. Izumi H, Kaneko Y. Trim32 facilitates degradation of MYCN on spindle poles and induces asymmetric cell division in human neuroblastoma cells. Cancer Res. (2014) 74:5620–30. doi: 10.1158/0008-5472.CAN-14-0169
16. Izumi H, Kaneko Y. Symmetry breaking in human neuroblastoma cells. Mol Cell Oncol. (2014) 1:e968510. doi: 10.4161/23723548.2014.968510
17. Gonczy P. Mechanisms of asymmetric cell division: flies and worms pave the way. Nat Rev Mol Cell Biol. (2008) 9:355–66. doi: 10.1038/nrm2388
18. Sawa H. Control of cell polarity and asymmetric division in C. elegans. Curr Top Dev Biol. (2012) 101:55–76. doi: 10.1016/B978-0-12-394592-1.00003-X
19. Yamashita YM, Mahowald AP, Perlin JR, Fuller MT. Asymmetric inheritance of mother versus daughter centrosome in stem cell division. Science. (2007) 315:518–21. doi: 10.1126/science.1134910
20. Shinin V, Gayraud-Morel B, Gomes D, Tajbakhsh S. Asymmetric division and cosegregation of template DNA strands in adult muscle satellite cells. Nat Cell Biol. (2006) 8:677–87. doi: 10.1038/ncb1425
21. Lechler T, Fuchs E. Asymmetric cell divisions promote stratification and differentiation of mammalian skin. Nature. (2005) 437:275–80. doi: 10.1038/nature03922
22. Quyn AJ, Appleton PL, Carey FA, Steele RJ, Barker N, Clevers H, et al. Spindle orientation bias in gut epithelial stem cell compartments is lost in precancerous tissue. Cell Stem Cell. (2010) 6:175–81. doi: 10.1016/j.stem.2009.12.007
23. Cicalese A, Bonizzi G, Pasi CE, Faretta M, Ronzoni S, Giulini B, et al. The tumor suppressor p53 regulates polarity of self-renewing divisions in mammary stem cells. Cell. (2009) 138:1083–95. doi: 10.1016/j.cell.2009.06.048
24. Wu M, Kwon HY, Rattis F, Blum J, Zhao C, Ashkenazi R, et al. Imaging hematopoietic precursor division in real time. Cell Stem Cell. (2007) 1:541–54. doi: 10.1016/j.stem.2007.08.009
25. Wang X, Tsai JW, Imai JH, Lian WN, Vallee RB, Shi SH. Asymmetric centrosome inheritance maintains neural progenitors in the neocortex. Nature. (2009) 461:947–55. doi: 10.1038/nature08435
26. Costa MR, Wen G, Lepier A, Schroeder T, Gotz M. Par-complex proteins promote proliferative progenitor divisions in the developing mouse cerebral cortex. Development. (2008) 135:11–22. doi: 10.1242/dev.009951
27. Betschinger J, Mechtler K, Knoblich JA. Asymmetric segregation of the tumor suppressor brat regulates self-renewal in Drosophila neural stem cells. Cell. (2006) 124:1241–53. doi: 10.1016/j.cell.2006.01.038
28. Schwamborn JC, Berezikov E, Knoblich JA. The TRIM-NHL protein TRIM32 activates microRNAs and prevents self-renewal in mouse neural progenitors. Cell. (2009) 136:913–25. doi: 10.1016/j.cell.2008.12.024
29. Chen G, Kong J, Tucker-Burden C, Anand M, Rong Y, Rahman F, et al. Human Brat ortholog TRIM3 is a tumor suppressor that regulates asymmetric cell division in glioblastoma. Cancer Res. (2014) 74:4536–48. doi: 10.1158/0008-5472.CAN-13-3703
30. Suenaga Y, Islam SM, Alagu J, Kaneko Y, Kato M, Tanaka Y, et al. NCYM, a Cis-antisense gene of MYCN, encodes a de novo evolved protein that inhibits GSK3beta resulting in the stabilization of MYCN in human neuroblastomas. PLoS Genet. (2014) 10:e1003996. doi: 10.1371/journal.pgen.1003996
31. Kaneko Y, Suenaga Y, Islam SM, Matsumoto D, Nakamura Y, Ohira M, et al. Functional interplay between MYCN, NCYM, and OCT4 promotes aggressiveness of human neuroblastomas. Cancer Sci. (2015) 106:840–7. doi: 10.1111/cas.12677
32. Zhao X, Li D, Pu J, Mei H, Yang D, Xiang X, et al. CTCF cooperates with noncoding RNA MYCNOS to promote neuroblastoma progression through facilitating MYCN expression. Oncogene. (2016) 35:3565–76. doi: 10.1038/onc.2015.422
33. Hu CA, Khalil S, Zhaorigetu S, Liu Z, Tyler M, Wan G, et al. Human Delta1-pyrroline-5-carboxylate synthase: function and regulation. Amino Acids. (2008) 35:665–72. doi: 10.1007/s00726-008-0075-0
34. Guo YF, Duan JJ, Wang J, Li L, Wang D, Liu XZ, et al. Inhibition of the ALDH18A1-MYCN positive feedback loop attenuates MYCN-amplified neuroblastoma growth. Sci Transl Med. (2020) 12:eaax8694. doi: 10.1126/scitranslmed.aax8694
35. Giannini G, Cerignoli F, Mellone M, Massimi I, Ambrosi C, Rinaldi C, et al. High mobility group A1 is a molecular target for MYCN in human neuroblastoma. Cancer Res. (2005) 65:8308–16. doi: 10.1158/0008-5472.CAN-05-0607
36. Puca F, Tosti N, Federico A, Kuzay Y, Pepe A, Morlando S, et al. HMGA1 negatively regulates NUMB expression at transcriptional and post transcriptional level in glioblastoma stem cells. Cell Cycle. (2019) 18:1446–57. doi: 10.1080/15384101.2019.1618541
37. Puca F, Colamaio M, Federico A, Gemei M, Tosti N, Bastos AU, et al. HMGA1 silencing restores normal stem cell characteristics in colon cancer stem cells by increasing p53 levels. Oncotarget. (2014) 5:3234–45. doi: 10.18632/oncotarget.1914
38. Pece S, Confalonieri S, Romano PR, Di Fiore P. P. NUMB-ing down cancer by more than just a NOTCH. Biochim Biophys Acta. (2011) 1815:26–43. doi: 10.1016/j.bbcan.2010.10.001
39. Colaluca IN, Tosoni D, Nuciforo P, Senic-Matuglia F, Galimberti V, Viale G, et al. NUMB controls p53 tumour suppressor activity. Nature. (2008) 451:76–80. doi: 10.1038/nature06412
40. Otto T, Horn S, Brockmann M, Eilers U, Schuttrumpf L, Popov N, et al. Stabilization of N-Myc is a critical function of Aurora A in human neuroblastoma. Cancer Cell. (2009) 15:67–78. doi: 10.1016/j.ccr.2008.12.005
41. Brockmann M, Poon E, Berry T, Carstensen A, Deubzer HE, Rycak L, et al. Small molecule inhibitors of aurora-a induce proteasomal degradation of N-myc in childhood neuroblastoma. Cancer Cell. (2013) 24:75–89. doi: 10.1016/j.ccr.2013.05.005
42. Xiao D, Yue M, Su H, Ren P, Jiang J, Li F, et al. Polo-like Kinase-1 regulates Myc stabilization and activates a feedforward circuit promoting tumor cell survival. Mol Cell. (2016) 64:493–506. doi: 10.1016/j.molcel.2016.09.016
43. Ackermann S, Goeser F, Schulte JH, Schramm A, Ehemann V, Hero B, et al. Polo-like kinase 1 is a therapeutic target in high-risk neuroblastoma. Clin Cancer Res. (2011) 17:731–41. doi: 10.1158/1078-0432.CCR-10-1129
44. Berdnik D, Knoblich JA. Drosophila Aurora-A is required for centrosome maturation and actin-dependent asymmetric protein localization during mitosis. Curr Biol. (2002) 12:640–7. doi: 10.1016/s0960-9822(02)00766-2
45. Wang H, Somers GW, Bashirullah A, Heberlein U, Yu F, Chia W. Aurora-A acts as a tumor suppressor and regulates self-renewal of Drosophila neuroblasts. Genes Dev. (2006) 20:3453–63. doi: 10.1101/gad.1487506
46. Wang H, Ouyang Y, Somers WG, Chia W, Lu B. Polo inhibits progenitor self-renewal and regulates Numb asymmetry by phosphorylating Pon. Nature. (2007) 449:96–100. doi: 10.1038/nature06056
47. Molenaar JJ, Domingo-Fernandez R, Ebus ME, Lindner S, Koster J, Drabek K, et al. LIN28B induces neuroblastoma and enhances MYCN levels via let-7 suppression. Nat Genet. (2012) 44:1199–206. doi: 10.1038/ng.2436
48. Schnepp RW, Khurana P, Attiyeh EF, Raman P, Chodosh SE, Oldridge DA, et al. A LIN28B-RAN-AURKA signaling network promotes neuroblastoma tumorigenesis. Cancer Cell. (2015) 28:599–609. doi: 10.1016/j.ccell.2015.09.012
49. Chen CH, Luhur A, Sokol N. Lin-28 promotes symmetric stem cell division and drives adaptive growth in the adult Drosophila intestine. Development. (2015) 142:3478–87. doi: 10.1242/dev.127951
Keywords: MYCN, TRIM32, NCYM, ALDH18A1, asymmetric cell division, neuroblastoma
Citation: Izumi H, Kaneko Y and Nakagawara A (2020) The Role of MYCN in Symmetric vs. Asymmetric Cell Division of Human Neuroblastoma Cells. Front. Oncol. 10:570815. doi: 10.3389/fonc.2020.570815
Received: 09 June 2020; Accepted: 21 September 2020;
Published: 21 October 2020.
Edited by:
Christer Einvik, UiT The Arctic University of Norway, NorwayReviewed by:
Monica Fedele, Consiglio Nazionale delle Ricerche (CNR), ItalyCopyright © 2020 Izumi, Kaneko and Nakagawara. This is an open-access article distributed under the terms of the Creative Commons Attribution License (CC BY). The use, distribution or reproduction in other forums is permitted, provided the original author(s) and the copyright owner(s) are credited and that the original publication in this journal is cited, in accordance with accepted academic practice. No use, distribution or reproduction is permitted which does not comply with these terms.
*Correspondence: Hideki Izumi, aXp1bWktaGlkZWtpQGtvc2Vpa2FuLmpw; orcid.org/0000-0003-1484-934X
†These authors have contributed equally to this work
Disclaimer: All claims expressed in this article are solely those of the authors and do not necessarily represent those of their affiliated organizations, or those of the publisher, the editors and the reviewers. Any product that may be evaluated in this article or claim that may be made by its manufacturer is not guaranteed or endorsed by the publisher.
Research integrity at Frontiers
Learn more about the work of our research integrity team to safeguard the quality of each article we publish.