- 1Department of Nuclear Medicine, Shanghai Changhai Hospital, Shanghai, China
- 2School of Medical Imaging, Xuzhou Medical University, Xuzhou, China
Primary liver tumor with hepatocellular carcinoma accounting for 75–80% of all such tumors, is one of the global leading causes of cancer-related death, especially in cirrhotic patients. Liver tumors are highly hypervascularized via the hepatic artery, while normal liver tissues are mainly supplied by the portal vein; consequently, intra-arterially delivered treatment, which includes transarterial chemoembolization (TACE) and transarterial radioembolization (TARE), is deemed as a palliative treatment. With the development of nuclear technology and radiochemistry, TARE has become an alternative for patients with hepatic cancer, especially for patients who failed other therapies, or for patients who need tumor downstaging treatment. In practice, some radionuclides have suitable physicochemical characteristics to act as radioactive embolism agents. Among them, 90Y emits β rays only and is suitable for bremsstrahlung single photon emission computed tomography (BS SPECT) and positron emission tomography (PET); meanwhile, some others, such as 131I, 153Sm, 166Ho, 177Lu, 186Re, and 188Re, emit both β and γ rays, enabling embolism beads to play a role in both therapy and single photon emission computed tomography (SPECT) imaging. During TARE, concomitant imaging provide additive diagnostic information and help to guide the course of liver cancer treatment. Therefore, we review the theranostic radionuclides that have been used or could potentially be used in TARE for liver cancer and focus on the clinical benefits of diagnostic applications, including real-time monitoring of embolism beads, evaluating irradiation dose, predicting therapy effects, and corresponding adjustments to TARE.
Introduction
The primary liver tumor often occurs in patients with liver cirrhosis, with hepatitis virus being the most common risk factor for liver cirrhosis (1). As the most common type of liver cancer, hepatocellular carcinoma (HCC) is the fifth most common cancer worldwide and the second leading cause of cancer-related mortality (2).
Nowadays, there are many options for the treatment of liver cancer according to the guidelines in the Barcelona Clinic Liver Cancer (BCLC) algorithm (3). However, because of the underlying liver dysfunction and its often-late diagnosis, only approximately 30% of patients when initially diagnosed are eligible for curative treatments (resection, percutaneous ablation, etc). Additionally, due to locally advanced diseases, poor liver function, or additional comorbidities, recurrence or metastasis are common after major curative treatment. Hence, various palliative treatments are considered for intermediate-advanced stage liver cancer, especially in inoperable cases. Taking advantage of the fact that the tumor is supplied by the hepatic artery while the surrounding normal liver tissue is mainly supplied by the portal system, transarterial radioembolization (TARE) appears as one of the attractive treatment options.
TARE intra-arterially delivers therapeutic radioactive agents to the tumor, which can achieve embolization of the feeding artery and radiation killing effect on tumor cells simultaneously during the treatment of liver cancer. Different from external radiotherapy, TARE limits systemic irradiation and preserves the healthy liver to a maximum extent. Additionally, the incidence of postembolization syndrome in TARE is relatively rare and mild compared to TACE (transarterial chemoembolization). TARE has been demonstrated to be feasible in treating patients at different stages or with postoperative recurrence, and serves as a downstaging strategy prior or as a bridge to transplantation (4). Meanwhile, TARE is an alternative treatment for those may be contraindication to other treatments.
Prior to TARE, structural imaging, such as computed tomography (CT) or magnetic resonance (MR), shows the size and location of the tumor and its relationship with the surrounding tissue. Besides, x-ray angiography is also vital before TARE, which can provide the blood supply of the tumor and the travel, distribution and variation of blood vessels. Single photon emission computed tomography/computed tomography (SPECT/CT) is also common imaging method during TARE. All these imaging technologies are helpful to guide treatment planning (e.g., the method of delivery, suitable treatment dose) and even predict treatment efficacy. After injecting the theranostic radionuclide into the hepatic artery, therapeutic effect is achieved by internal exposure around the tumor. Meanwhile, real-time or post-therapeutic nuclear imaging, such as SPECT/CT, provides information on verification of treatment delivery, the biodistribution of radioconjugates, integral radiation dose at tumor site and organs of interest (lung, liver, thyroid gland, etc) and prediction of treatment outcome. Later post-therapeutic imaging mainly observes the therapeutic effect on the tumor and monitors other organs for complications. Furthermore, positron emission tomography/computed tomography (PET/CT) is also a potential imaging modality for TARE. It realizes functional imaging before and after treatment by showing the glucose metabolism of the tumor and sensitively finding metastasis. Hence, imaging plays a key role in drug development and clinical evaluation, and is conducive to developing adaptive TARE plans according to the metabolic mode.
Theranostic radionuclides integrating imaging and treatment during radioembolization are the most important component of TARE for liver cancer. Based on how TARE works, radionuclides that emit α rays are not suitable because of their pathlengths are relatively short and thus have a limited range in tissue. Radionuclides emiting β rays can provide substantial radiation therapy for liver tumors due to their pathlengths ranging from millimeters to centimeters. Meanwhile, emitted γ rays are helpful in monitoring embolization beads using nuclear imaging. Considering the therapeutic purpose, the half-life of nuclides ranging from one to several days is suitable, allowing the tumor to be exposed to sufficient radiation while avoiding radiation damage in the process of excretion. Thus far, only two 90Y based microspheres (MSs) and 131I-lipiodol are approved for clinical use, while many others are still in the pre-clinical or basic research stage. Recently, the use of additional theranostic nuclides in TARE has been explored. Table 1 summarizes the critical characteristics (nuclear property and imaging type) of theranostic nuclides that have been used and those that have shown potential in TARE for hepatic cancer. At the same time, the theranostic effects, especially the diagnostic performance of those theranostic radionuclides will be summarized by nuclides and presented here. Among them, 90Y is the most important.
Yttrium-90
Yttrium-90, which has favorable physicochemical characteristics, is presently the most widely used nuclide for TARE of liver cancer clinically. Yttrium-90 is a pure β emitter and has a half-life of 64.2 h. The average energy of β emission is 0.937 MeV with a mean tissue penetration of 2.5 mm. High energy, short tissue penetration distance and relatively short half-life make 90Y a suitable nuclide in TARE. Compared with other radionuclide compounds, 90Y-labeled MSs (90Y-MSs) are already clinically available for liver cancer in some countries. The two currently commercially available 90Y-MS products are glass MSs (TheraSphere) and resin MSs (SIR-Spheres). Because of the different physical and chemical properties, the treatment dose calculation for 90Y-MS TARE differs significantly according to the MS type (5).
Blood supply and hepatic collateral circulation of tumor are essential for the efficacy and safety of treatment. Current clinical 90Y-MS BS SPECT (bremsstrahlung single photon emission computed tomography) is hindered by image quality, while 90Y-MS PET is extremely challenging due to low activity concentrations in the lungs and the low positron yield of 90Y. Hence, 99mTc-MAA (Technetium-99m macroaggregated albumin) is a surrogate of 90Y for pre-treatment imaging. Intra-arterially delivery of 99mTc-MAA is able to simulate the distribution of therapeutic drugs in the liver, by measuring the radiation intensity of cancerous and non-cancerous liver tissue respectively, the ratio of tumor/non-tumor (T/NT) can be calculated more accurately (6). That provides a quantitative index for evaluating the abundance of tumor blood supply and the ability of cancer tissue to absorb therapeutic drugs through hepatic artery. Patients should undergo 99mTc-MAA examination before 90Y-MS TARE, which offers important preprocedural information. In 99mTc-MAA SPECT/CT, 99mTc-MAA (4–5 mCi) is injected into the hepatic artery to assess extra-hepatic depositions and lung shunting, and can provide the calculation of the lung shunt absorbed dose, which guides dosimetry to avoid radiation pneumonitis. Moreover, accurate superselective angiography should be performed to evaluate the targeted vascular territory and anatomic variants or extra-hepatic vessels should accept prophylactic embolization, which can avoid ectopic embolization of non-targeted organs. However, the concordance between 99mTc-MAA and 90Y-MSs remains controversial. Many studies have reported both similarity (7) and differences (8) between 99mTc-MAA and 90Y-MSs uptake. The inconsistent concordance may be due to several factors (the different size, shape, and number of MAA relative to microspheres, flow dynamics during delivery, etc), for which the post-therapy images of 90Y-activity distribution is considered appropriate to determine the tumor dose-response. Apart from SPECT/CT, CT or MR imaging are also be used to assess the volume, location, and relationship with surrounding hepatic tissues of the tumor before TARE.
99mTc-MAA scanning and superselective angiography are often performed before 90Y-MS TARE to guide dosimetry, although accumulating evidence has improved the safety and efficacy of using radioembolization with 90Y-labeled resin or glass MSs for the management of hepatic tumors (9). A study of 1,652 patients (1,124 TACE, 528 90Y-TARE) showed that 90Y-MS TARE increased the 2-year overall survival (OS) rates relative to the observational subgroup and resulted in better objective response (OR) rates than treatment with TACE. Furthermore, a lower risk of adverse events was observed in 90Y-TARE than in TACE (10). Many studies have demonstrated that 90Y-MS TARE is an effective choice and results in significantly fewer severe adverse events when treating HCC patients with portal vein thrombosis (PVT), which is a contraindication for most traditional therapies (11).
For post-treatment imaging, compared with the majority of the radionuclides used in TARE, 90Y is a pure β emitter and lacks discrete-energy photon emissions, such as γ rays and/or characteristic fluorescence x-rays (12). The β particles emitted by 90Y produce secondary bremsstrahlung radiation, which may be imaged to locate the 90Y-MSs. Commonly, BS SPECT is used after radioembolization to exclude extra-hepatic activity deposition and to assess intra-hepatic MSs distribution. Ahmadzadehfar et al. performed BS SPECT/CT imaging on 188 patients who accepted 90Y-MS TARE 24 h prior, to assess the capability of BS SPECT/CT in detecting and positioning of extrahepatic accumulation (gastrointestinal complications). Gastroduodenoscopy served as reference standards. The results showed that the positive predictive values, sensitivity, specificity and the accuracy of BS SPECT/CT were 100, 87, 100, and 99%, respectively (13). Furthermore, BS SPECT/CT after radioembolization is beneficial to evaluate tumor dose-response characteristics and predict treatment response for the management of patients (14). Potrebko et al. demonstrated that voxel-based dosimetry for 90Y microsphere therapy allows for quantitative quality assurance of the delivered treatment using deformable image registration, calculated isodose distributions, and dose-volume histograms (DVHs). Since β‐radiation emitted by 90Y interacts with body tissues resulting in bremsstrahlung radiation, SPECT/CT has traditionally been regarded as the gold‐standard modality to image the biodistribution of this radionuclide. However, the limitations of the BS SPECT image are poor spatial resolution (11–15 mm) and limited quantitative accuracy because of the low photon yield and continuous nature of the BS X-ray spectrum. The absence of some correction techniques leads to a further reduction in the image contrast. Many efforts have been made to improve BS SPECT/CT imaging qualitatively and quantitatively, in which Monte Carlo simulation is frequently accepted. However, it is not commercially available and cannot be easily implemented clinically (15).
Interestingly, the 90Y decay process is of a very small branching to the excited state of stable 90Zr. The internal pair production (positron) generated during the decay scheme of 90Y allows quantitative monitoring on itself via PET (16, 17). Compared with BS SPECT, 90Y PET imaging provides better image quality in contrast and resolution (4–5 mm). Elschot et al. quantitatively demonstrated that the image quality of PET was superior to that of BS SPECT for the assessment of 90Y-MS distribution after radioembolization in a study of estimated liver dose distributions in 5 patients (18) (Figure 1B). The Figure 1A showed that the PET-based CDVH (cumulative dose-volume histograms) of the spherical region-of-interest followed the true CDVH more closely than the SPECT-based CDVH. Moreover, calculating the tumor dose on imaging may predict the response to the treatment. A study published in 2013 demonstrated that tumor and non-target tissue absorbed dose quantification using 90Y PET was accurate and yielded radiobiologically meaningful dose-response information to guide adjuvant or mitigative action (23). The true definitions of the minimal effective tumor dose and maximum tolerated non-tumor dose remain challenges for each tumor and MS type, and 90Y PET may be a potential solution.
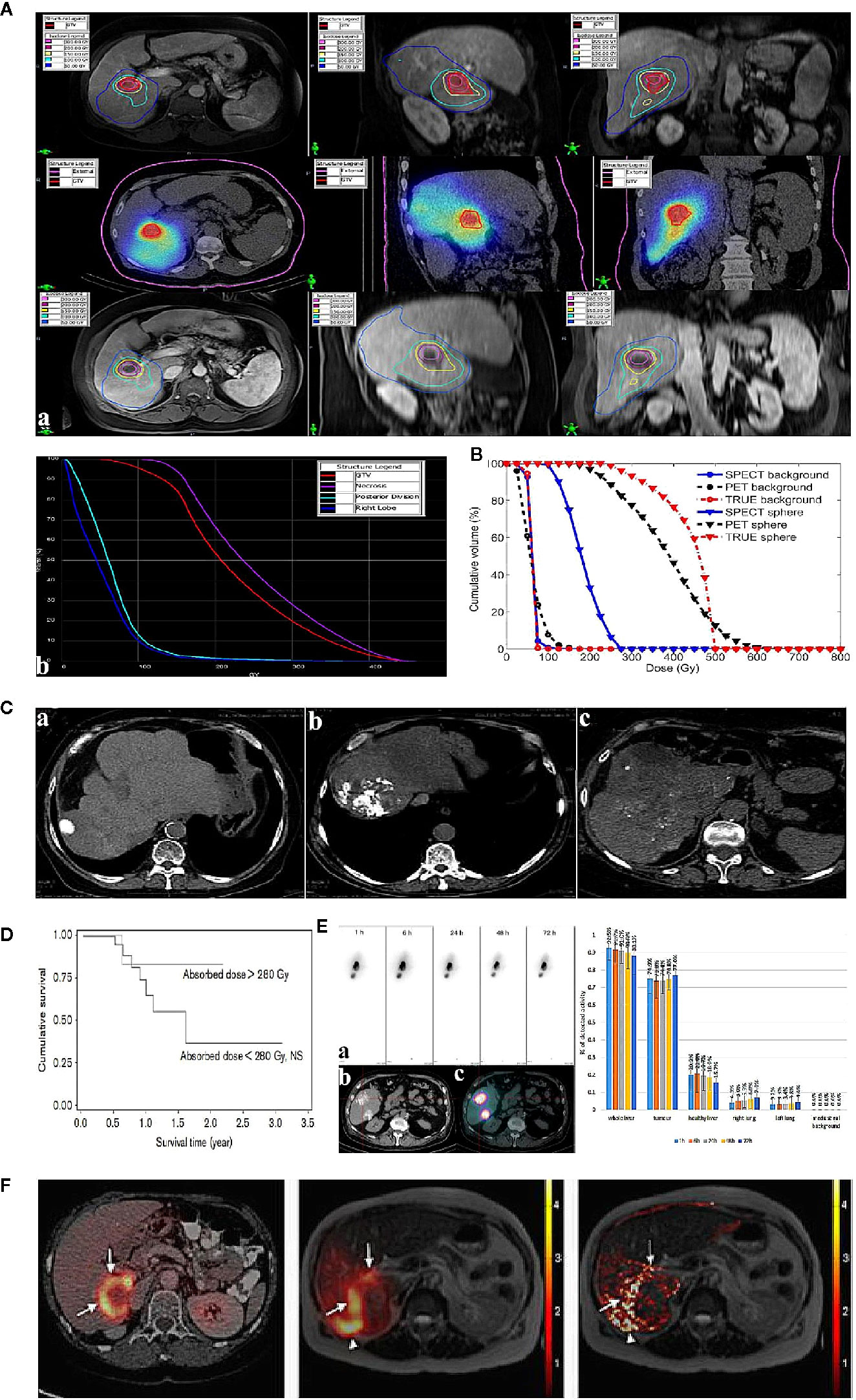
Figure 1 Examples of post-therapeutic imaging of liver tumor. (A) The dose of each ROI (regions of interest) in pre-treatment diagnostic MR (top), post-treatment SPECT/CT (middle), and 5-week follow-up MR (bottom) for one patient with HCC (a). Dose-volume histograms of the GTV (gross target volume), necrotic region, posterior division, and right lobe (on the right) (b). Adapted from Potrebko et al. (14). (B) CDVH of the phantom (a method of image reconstruction) background ROI and the ROI of the 37-mm diameter sphere. Adapted from Elschot et al. (18). (C) Lipiodol tumor accretion at 1 month after intra-arterial injection: complete accretion (49 patients): (a) complete accumulation in 5 patients (10%), (b) partial defect in 15 (31%), (c) faint accumulation in 29 patients (59%). Adapted from Gaultier et al. (19). (D) Survival in terms of tumoural absorbed dose (threshold=280 Gy) calculated in tomographic mode. Adapted from Becker et al. (20). (E) Intra-arterial injection of 188Re-SSS Lipiodol on hepatocellular carcinoma patients. Adapted from Delaunay et al. (21). The left shows whole-body scintigraphic studies (mean geometric) of patient by (a) SPECT, (b) CT, (c) fused SPECT/CT. The right is the average biodistribution profile of patients treated 188Re-SSS lipiodol by SPECT/CT image. (F) The picture shows intrahepatic biodistribution of 166Ho-microspheres on liver tumor patient by 18F-FDG PET/CT, SPECT and MR imaging (from left to right). Adapted from Smits et al. (22).
Undoubtedly, 90Y is the most suitable theranostic radionuclide for TARE of liver cancer so far. 90Y, as a pure β emitter, has high energy and relatively small radiation range, which can effectively kill tumor cells while avoiding damage to surrounding healthy tissue, and no requiring subsequent isolation of patients is needed. Although BS SPECT and PET can match the imaging requirements, there are still many unsolved technological issues restricting the accuracy of images and widespread clinical use. For example, low true-coincidence count-rate for positron emission results in relatively longer acquisition times for 90Y PET imaging. Besides, standardization and uniform implementation of reconstruction and correction techniques are urgently needed. The necessity of specialized nuclear reactors and relatively long transportation times to each hospital limit the use of 90Y. Importantly, 90Y is still not be approved and available in many countries, such as in China. Serious complications caused by ectopic embolization of 90Y-MS may also occur, which needs high attention in clinical application.
Iodine-131
The nuclide 131I (T1/2 = 8.04d) emits both β and γ rays. Its β particles have a maximum energy of 0.6 MeV and a maximum path length in tissue of 2.3 mm. Utilizing the characteristics of iodine lipiodol, which selectively deposits in nodules of hepatocellular carcinoma, radioactive lipiodol accumulates in cancer tissues and decreases irradiation damage to non-cancer tissues. Hence, 131I-lipiodol was often studied for TARE of liver cancer before. In a representative clinical study of 50 patients, the median survival of patients treated with 131I-lipiodol was 24 weeks longer than that of the untreated group and no radiotoxic effect was found, especially interstitial pneumonia (19) (Figure 1C).
In addition to lipiodol, 131I can also be used to label embolism beads, normally in the form of MSs, which are often prepared in stepwise procedures for multiple theranostic applications. Liu et al. incorporated hollow CuS nanoparticles into PLGA (poly lactic-co-glycolic acid) MSs to produce HCuSNPs-loaded MSs, which were then labeled with 131I to form 131I-HCuSNPs-MSs (24). After the intra-arterial embolization with 131I-HCuSNPs-MSs delivered into the hepatic artery of liver tumor-bearing rats, SPECT imaging confirmed that MSs were mainly located in liver tumor and only minimal accumulation was detected in other organs, particularly in the lungs. SPECT imaging findings qualitatively manifested the success of TARE operation and avoidance of damage potentially resulting from the leakage of embolism beads. The follow-up SPECT scan proved that effective embolization lasted for up to 48 h. The predictive results aided understanding of the entire treatment process and prognosis, which was more timely to figure out the primary outcome than traditional follow-up diagnosis, such as blood tests and CT.
Regarding diagnostic performance, 131I-based imaging provides qualitative information on whole-body distribution, including tumor accumulation, and even permits semi-quantitative analysis (25). Becker et al. reported that 7 days after the injection, 61% of 131I-lipiodol was distributed in the liver tumor, 23% was distributed in non-tumoral liver, and 16% was distributed in the lungs, which highlighted that the clinical toxicities of 131I-lipiodol were more commonly affected the lungs (20). More importantly, tumor radioactivity dosimetry helps to define an absorbed dose threshold, especially when the accumulation of the radioactive embolism agent was recorded dynamically (Figure 1D). That would predict a therapeutic effect, thus providing guidance for the subsequent therapeutic approach and optimizing the activity to be injected.
There are still some deficiencies in the TARE transformation of 131I. Compared to 90Y, possible extra managements are needed for the patient’s excreta (such as urine) due to the longer half-life of 131I. Besides, non-therapeutic rays (γ rays) with high energy and long path length probably damage the surrounding normal tissue. In addition, too many scattered rays produced by 131I lead to relatively low contrast and resolution of SPECT image that are not conducive to quantitative analysis and barely suitable for qualitative analysis. Moreover, defects in terms of in vivo stability still exist in 131I compounds produced using iodized oil replacement or labeling methods.
Other Potential Theranostic Radionuclides for TARE
Rhenium-188
188Re is obtained from 188W/188Re generators in a convenient and comparatively cheap way, and its radioactivity yield can recover to 80% at 40 h postelution. The product of 188Re is 188Os by β decay, with a half-life of 16.9 h. 188Re with a shorter half-life and similar energy of β rays compared 90Y, is a promising radionuclide proposed for TARE of hepatic tumors. Similar to 131I, the γ ray emission of 188Re allows post-therapeutic SPECT imaging.
Early available 188Re embolism agents were the lipiodol conjugates as well. 188Re-chelator mixed with lipiodol, such as 188Re-HDD/lipiodol, 188Re-DEDC/lipiodol, and 188Re-SSS/lipiodol, has been tested in humans to date. Some studies showed that TARE with 188Re-HDD/lipiodol is a safe, effective, and promising method. Meanwhile, 188Re was also used to label MSs as embolism beads. A study of 10 patients (3 patients with unresectable colorectal liver metastases and 7 patients with hepatocellular cancer), who received treatment with 188Re-human serum albumin MSs, displayed considerable survival rates and tolerable toxicity (26). Other reported 188Re-MSs include 188Re-PLGA (27), 188Re-poly(L-lactic acid) (PLLA) (28), 188Re-glass-MSs, and 188Re-resin-MSs.
Regarding diagnostic performance, embolization evaluation and related dosimetry in nuclear imaging are helpful for the treatment plan. In a study of 6 patients with unresectable HCC who accepted intra-arterial 188Re-SSS/lipiodol, whole-body planar scintigraphy and SPECT were used during therapy (21) and revealed curative hepatic uptake; 90.7 ± 1.6% of radioactivity was detected in the liver with 74.9 ± 1.8% of detected radioactivity in the tumor and 9.3 ± 1.6% of radioactivity was detected in the lungs (Figure 1E). The average T/NT uptake ratio was 42.7 ± 7.8 on SPECT. These quantitative data provided a definite diagnosis to conclude that 188Re-SSS/lipiodol had satisfactory embolization characteristics, guaranteeing in vivo therapeutic application.
Because of its short half-life, much more activities are needed for 188Re than 90Y to achieve a comparable therapeutic effect, which may produce acute radiation damage to normal tissue. Notably, 188Re labeling relies on a relatively complicated reaction, which partially restricts the synthesis of the 188Re embolism agents and clinical transformation.
Holmium-166
166Ho emits high-energy β rays, with a comparatively short half-life (1.1 days) (29). Due to its short half-life, 166Ho is suitable for patients in urgent need of downstaging treatment compared to the longer radiation therapy time of 90Y. 166Ho can be produced by two methods, in a nuclear reactor or by neutron activation of 164Dy; hence its production is both fast and relatively cheap. Additionally, its outstanding advantage over 90Y, 131I, and 188Re is that 166Ho is paramagnetic and emits low-energy γ radiation, which enables the dual MR and SPECT imaging.
166Ho-labeled lipiodol has rarely been reported. Das et al. prepared radiolabeled lipiodol by dispersing 166Ho-oxine complex in lipiodol (30). For in vivo evaluation, the imaging studies of 166Ho-lipiodol revealed satisfactory hepatic retention and insignificant uptake was detected in other major organs or tissues except skeleton. 166Ho-MSs were extensively studied. Several 166Ho-MSs have been prepared so far, including glass, resin, phosphate, and polymer MSs, and all of them have shown good prospects for TARE, especially polymer MSs, due to the advantage of near-plasma density, biodegradability, and biocompatibility (31). In a phase I trial including 15 patients with an unresectable and chemorefractory liver tumor, 166Ho-PLLA-MS TARE was implemented in four cohorts of three to six patients, injecting 20 Gy, 40 Gy, 60 Gy, and 80 Gy, respectively. At 6 weeks, treatment response was one patient with partial response, seven with stable disease and seven with disease progression (32). Similarly, 166Ho-chitosan MSs and 166Ho-alginate MSs (33) that were prepared for radioembolization behaved feasibly and safely.
What is noteworthy is that, due to favorable nuclear imaging characteristics of 166Ho, a scout dose (250 MBq) of 166Ho is used as an alternative before 166Ho-MS TARE, which is superior in calculating the lung shunt fraction compared to 99mTc MAA (34). Considering controversy over the concordance of 99mTc MAA and 90Y-MSs, 166Ho itself can be used in pre-treatment imaging, for which pre-treatment imaging planning dosimetry could potentially be more accurate. Regarding diagnostic performance, taking advantage of both SPECT and MR imaging, the estimated radiation absorbed dose could be evaluated more precisely in 166Ho-complex liver radioembolization. In 2013, Smits et al. performed a phase I clinical trial to investigate the feasibility of quantitative imaging of 166Ho radioembolization in 15 patients with unresectable liver cancer based on SPECT and MR imaging (22). The gross comparison yielded a strong correlation between MR and SPECT imaging and moderate agreement between the absorbed dose in each segment as evaluated based on MR and SPECT imaging (Figure 1F). The median overall T/NT ratio was 1.4 based on SPECT (range, 0.9–2.8) and 1.4 based on MR imaging (range, 1.1–3.1). In 40% patients (6/15), all T/NT ratios ≥1, indicating that dosimetry based on 166Ho-MS SPECT and MR correlated well for the dose in liver segments and tumor tissue. There is no doubt that the use of two imaging modalities allows more informed decision making regarding treatment.
Due to the shorter half-life and lower energy of β rays of 166Ho compared to 90Y, similar to 188Re, a larger administered doses are needed to achieve the dosimetry equivalent to that of 90Y for treatment, which potentially cause acute damage to the surrounding normal tissue.
Lutecium-177
177Lu, a popular theranostic nuclide in clinical studies and recently applied in many contexts, may also be the promising radionuclide in TARE for liver cancer treatment. 177Lu has a similar half-life to 90Y but emits relatively low energy β rays, which limits the radiation exposure to surrounding tissues. Regarding diagnostic performance, emitted γ rays are convenient for positioning observation and radiation dosimetry. As a potential theranostic radionuclide, 177Lu has shown to be advantageous in peptide receptor radionuclide therapy (PRRT) of neuroendocrine tumors and peptide radioligand therapy (PRLT) of prostate cancers (35). As a source for TARE, 177Lu still has many issues that need to be addressed in future studies. 177Lu is produced using reactor irradiation, but few sources of supply and relatively high cost limit its broad application now.
Samarium-153
153Sm emits both β and γ rays, meeting the requirements for theranostic radionuclides. Recent studies have shown that the absorbed doses of 153Sm-labeled MSs in all organs can be controlled below 1 Gy and are safe for surrounding healthy tissues (36). Due to limited reports, its therapeutic potential in cancer treatment has not been widely studied, and its diagnostic performance is still not clear.
Rhenium-186
186Re has an appropriate half-life and emits both β and γ rays. At present, 186Re is clinically used to treat bone metastases caused by prostate cancer, rarely used in TARE for liver cancer. Due to its shared chemical properties and referential experience in embolism agent development with 188Re, 186Re is likely to be an ideal nuclide for TARE.
Conclusion
The incidence of liver cancer is increasing worldwide; nevertheless, effective therapeutic options are limited, and recurrence is common after preferred suitable treatment. Although TARE is not included in the BCLC staging system guidelines, current ongoing randomized clinical trials suggest that TARE is a safe, feasible, and palliative treatment for liver cancer, especially when other conventional treatments have failed. In some cases, where there may be contraindications for other treatments, TARE will be a safe and effective choice. It can achieve a similar or higher overall survival rate and slow down the progression of the disease compared to other routine treatments in the reported literature. Theranostic radionuclides in TARE allow for controlled high radiation doses to be supplied to hepatic tumors while the adjacent liver is relatively spared. In the meantime, diagnostic performance is attractive in the course of treatment. The qualitative or quantitative detection of effective embolization promotes the development of radioactive embolic agents, and assessment of the accumulated dose can guide the course of treatment using post-treatment partial or full body scan. In fact, due to the differences in the supply of nuclides around the world, the current status of clinical application of each radionuclide is not the same. In future, technical improvements and clinical studies are needed to promote the use of radionuclides in TARE. In terms of technology, the technique of radionuclide labeling, efficiency and technology of the acquisition of β and γ rays, and technology of image reconstruction all could be improved and developed for better diagnostic and therapeutic effect. In addition, besides 90Y, other potential nuclides (166Ho, 177Lu, etc) still lack adequate clinical studies and applications for TARE.
Furthermore, except for theranostic radionuclides, embolism agents are also being widely developed and studied. Various new drug-eluting beads (such as, HepaSpheres, CalliSpheres, TANDEM, etc) have obtained certain verification in clinical trials. Natural biological materials, silk fibroin, is proposed to become a potential embolism agent for the treatment of liver cancer and still in basic research stage. In a word, future efforts should be aimed at personalizing TARE therapy depending on the characteristics of different theranostic radionuclides and embolism agents, which making TARE a more effective and safe treatment for liver cancer.
Author Contributions
RL wrote the manuscript. RL, DL and GJ were responsible for searching related literature. XL, GS, and CZ were involved in revisions and proof-reading. All authors contributed to the article and approved the submitted version.
Funding
This work was funded by the National Natural Science Foundation of China (81471714 and 81871390) and National Natural Science Foundation Youth Project (81701761).
Conflict of Interest
The authors declare that the research was conducted in the absence of any commercial or financial relationships that could be construed as a potential conflict of interest.
References
1. Gomaa AI, Khan SA, Toledano MB, Waked I, Taylor-Robinson SD. Hepatocellular carcinoma: epidemiology, risk factors and pathogenesis. World J Gastroenterol (2008) 14(27):4300–8. doi: 10.3748/wjg.14.4300
2. Ferlay J, Soerjomataram I, Dikshit R, Eser S, Mathers C, Rebelo M, et al. Cancer incidence and mortality worldwide: sources, methods and major patterns in GLOBOCAN 2012. Int J Cancer (2015) 136(5):E359–86. doi: 10.1002/ijc.29210
3. Bruix J, Sherman M. Management of hepatocellular carcinoma: an update. Hepatology (2011) 53(3):1020–2. doi: 10.1002/hep.24199
4. Woodall CE, Scoggins CR, Ellis SF, Tatum CM, Hahl MJ, Ravindraet KV, et al. Is selective internal radioembolization safe and effective for patients with inoperable hepatocellular carcinoma and venous thrombosis? J Am Coll Surg (2009) 208(3):375–82. doi: 10.1016/j.jamcollsurg.2008.12.009
5. Saini A, Wallace A, Alzubaidi S, Knuttinen MG, Naidu S, Sheth R, et al. History and Evolution of Yttrium-90 Radioembolization for Hepatocellular Carcinoma. J Clin Med (2019) 8(1):55. doi: 10.3390/jcm8010055
6. Camacho JC, Kokabi N, Xing M, Schuster DM, Kim HS. PET Response Criteria for Solid Tumors Predict Survival at Three Months After Intra-Arterial Resin-Based 90Yttrium Radioembolization Therapy for Unresectable Intrahepatic Cholangiocarcinoma. Clin Nucl Med (2014) 39:944–50. doi: 10.1097/RLU.0000000000000557
7. Chiesa C, Mira M, Maccauro M, Spreafico C, Romito R, Morosi C, et al. Radioembolization of hepatocarcinoma with 90Y glass microspheres: development of an individualized treatment planning strategy based on dosimetry and radiobiology. Eur J Nucl Med Mol Imaging (2015) 42(11):1718–38. doi: 10.1007/s00259-015-3068-8
8. Haste P, Tann M, Persohn S, LaRoche T, Aaron V, Mauxion T, et al. Correlation of Technetium-99m Macroaggregated Albumin and Yttrium-90 Glass Microsphere Biodistribution in Hepatocellular Carcinoma: A Retrospective Review of Pretreatment Single Photon Emission CT and Posttreatment Positron Emission Tomography/CT. J Vasc Interv Radiol (2017) 28(5):722–30. doi: 10.1016/j.jvir.2016.12.1221
9. Raoul JL, Boucher E, Rolland Y, Garin E. Treatment of hepatocellular carcinoma with intra-arterial injection of radionuclides. Nat Rev Gastro Hepat (2010) 7(1):41–9. doi: 10.1038/nrgastro.2009.202
10. Yang Y, Si T. Yttrium-90 transarterial radioembolization versus conventional transarterial chemoembolization for patients with hepatocellular carcinoma: a systematic review and meta-analysis. Cancer Biol Med (2018) 15(3):299–310. doi: 10.20892/j.issn.2095-3941.2017.0177
11. Cho YY, Lee M, Kim HC, Chung JW, Kim YH, Gwak GY, et al. Radioembolization Is a Safe and Effective Treatment for Hepatocellular Carcinoma with Portal Vein Thrombosis: A Propensity Score Analysis. PloS One (2016) 11(5):e0154986. doi: 10.1371/journal.pone.0154986
12. Siman W, Mikell JK, Kappadath SC. Practical reconstruction protocol for quantitative 90Y bremsstrahlung SPECT/CT. Med Phys (2016) 43(9):5093–103. doi: 10.1118/1.4960629
13. Ahmadzadehfar H, Muckle M, Sabet A, Wilhelm K, Kuhl C, Biermann K, et al. The significance of bremsstrahlung SPECT/CT after yttrium-90 radioembolization treatment in the prediction of extrahepatic side effects. Eur J Nucl Med Mol Imaging (2012) 39:309–15. doi: 10.1007/s00259-011-1940-8
14. Potrebko PS, Shridhar R, Biagioli MC, Sensakovic WF, Andl G, Poleszczuk J, et al. SPECT/CT image-based dosimetry for Yttrium-90 radionuclide therapy: Application to treatment response. J Appl Clin Med Phys (2018) 19(5):435–43. doi: 10.1002/acm2.12400
15. Du XRY, Ljungberg M, Rault E, Vandenberghe S, Frey EC. Development and evaluation of an improved quantitative 90Y bremsstrahlung SPECT method. Med Phys (2012) 39(5):2346–58. doi: 10.1118/1.3700174
16. Kallini JR, Gabr A, Salem R, Lewandowski RJ. Transarterial Radioembolization with Yttrium-90 for the Treatment of Hepatocellular Carcinoma. Adv Ther (2016) 33(5):699–714. doi: 10.1007/s12325-016-0324-7
17. Kao YH, Tan EH, Lim KY, Ng CE, Goh SW. Yttrium-90 internal pair production imaging using first generation PET/CT provides high-resolution images for qualitative diagnostic purposes. Br J Radiol (1015) 2012) 85:1018–9. doi: 10.1259/bjr/33524085
18. Elschot M, Vermolen BJ, Lam MG, de Keizer B, van den Bosch MAAJ, de Jong HWAM. Quantitative Comparison of PET and Bremsstrahlung SPECT for Imaging the In Vivo Yttrium-90 Microsphere Distribution after Liver Radioembolization. PloS One (2013) 8(2):e55742. doi: 10.1371/journal.pone.0055742
19. Gaultier AL, Perret C, Ansquer C, Euge`nec T, Bode´re FK, Frampas E. Intra-arterial injection of 131I-labeled Lipiodol for advanced hepatocellular carcinoma: a 7 years’ experience. Nucl Med Commun (2013) 34(7):674–81. doi: 10.1097/MNM.0b013e32836141a0
20. Becker S, Laffonta S, Vitry F, Rolland Y, Lecloirec J, Boucher E, et al. Dosimetric evaluation and therapeutic response to internal radiation therapy of hepatocarcinomas using iodine-131-labelled lipiodol. Nucl Med Commun (2008) 29(9):815–25. doi: 10.1097/MNM.0b013e32830439c6
21. Delaunay K, Edeline J, Rolland Y, Lepareur N, Laffont S, Palard X, et al. Preliminary results of the Phase 1 Lip-Re I clinical trial: biodistribution and dosimetry assessments in hepatocellular carcinoma patients treated with 188Re-SSS Lipiodol radioembolization. Eur J Nucl Med Mol I (2019) 46(7):1506–17. doi: 10.1007/s00259-019-04277-9
22. Smits ML, Elschot M, van den Bosch MA, van de Maat GH, van het Schip AD, Zonnenberg BA, et al. In Vivo Dosimetry Based on SPECT and MR Imaging of 166Ho-Microspheres for Treatment of Liver Malignancies. J Nucl Med (2013) 54(12):2093–100. doi: 10.2967/j.113.119768
23. Kao YH, Steinberg JD, Tay YS, Lim GK, Yan J, Townsend DW, et al. Post-radioembolization yttrium-90 PET/CT-part 2: dose-response and tumor predictive dosimetry for resin microspheres. EJNMMI Res (2013) 3(1):57. doi: 10.1186/2191-219X-3-57
24. Liu QF, Qian YY, Li PL, Zhang SH, Liu JJ, Sun XG, et al. 131I-labeled copper sulfide-loaded microspheres to treat hepatic tumors via hepatic artery embolization. Theranostics (2018) 8(3):785–99. doi: 10.7150/thno.21491
25. Lambert B, Van de Wiele C. Treatment of hepatocellular carcinoma by means of radiopharmaceuticals. Eur J Nucl Med Mol Imaging (2005) 32(8):980–9. doi: 10.1007/s00259-005-1859-z
26. Liepe, Brogsitter C, Leonhard J, Wunderlich G, Hliscs R, Pinkert J, et al. Feasibility of High Activity Rhenium-188-Microsphere in Hepatic Radioembolization. JPN J Clin Oncol (2007) 37(12):942–50. doi: 10.1093/jjco/hym137
27. Vega JCDL, Esquinas PL, Rodríguez-Rodríguez C, Bokharaei M, Moskalev I, Liu D, et al. Radioembolization of Hepatocellular Carcinoma with Built-In Dosimetry: First in vivo Results with Uniformly-Sized, Biodegradable Microspheres Labeled with 188Re. Theranostics (2019) 9(3):868–83. doi: 10.7150/thno.29381
28. Jamre M, Shamsaei M, Erfani M, Sadjadi S, Ghannadi Maragheh M. Preparation and evaluation of 188Re sulfide colloidal nanoparticles loaded biodegradable poly (L-lactic acid) microspheres for radio-embolization therapy. J Labelled Compd Rad (2018) 61(8):586–94. doi: 10.1002/jlcr.3627
29. Reinders MTM, Smits MLJ, Roekel CV, Braat AJAT. Holmium-166 Microsphere Radioembolization of Hepatic Malignancies. Semin Nucl Med (2019) 49(3):237–43. doi: 10.1053/j.semnuclmed.2019.01.008
30. Das T, Chakraborty S, Sarma HD, Venkatesh M, Banerjee S. Preparation of 166Ho-oxine-lipiodol and its preliminary bioevaluation for the potential application in therapy of liver cancer. Nucl Med Commun (2009) 30(5):362–7. doi: 10.1097/MNM.0b013e328329981a
31. Bouvry C, Palard X, Edeline J, Ardisson V, Loyer P, Garin E, et al. Transarterial Radioembolization (TARE) Agents beyond 90Y-Microspheres. BioMed Res Int (2018) 2018:1–14. doi: 10.1155/2018/1435302
32. Smits ML, Nijsen JF, van den Bosch MA, Lam MG, Vente MD, Huijbregts JE, et al. Holmium-166 radioembolization for the treatment of patients with liver metastases: design of the phase I HEPAR trial. J Exp Clin Canc Res (2010) 29(1):70. doi: 10.1186/1756-9966-29-70
33. Zielhuis SW, Seppenwoolde JH, Bakker CJ, Jahnz U, Zonnenberg BA, van het Schip AD, et al. Characterization of holmium loaded alginate microspheres for multimodality imaging and therapeutic applications. J BioMed Mater Res (2007) 82A(4):892–8. doi: 10.1002/jbm.a.31183
34. Prince JF, Rooij RV, Bol GH, de Jong HW, van den Bosch MAJ, Lam MEH. Safety of a Scout Dose Preceding Hepatic Radioembolization with 166Ho Microspheres. J Nucl Med (2015) 56:817–23. doi: 10.2967/jnumed.115.155564
35. Ceci F, Castellucci P, Cerci JJ, Fanti S. New Aspects of Molecular Imaging in Prostate Cancer. Methods (2017) 130:36–41. doi: 10.1016/j.ymeth.2017.07.009
Keywords: liver cancer, diagnostic performance, theranostic radionuclides, transarterial radioembolization, nuclear imaging
Citation: Li R, Li D, Jia G, Li X, Sun G and Zuo C (2021) Diagnostic Performance of Theranostic Radionuclides Used in Transarterial Radioembolization for Liver Cancer. Front. Oncol. 10:551622. doi: 10.3389/fonc.2020.551622
Received: 14 April 2020; Accepted: 07 December 2020;
Published: 25 January 2021.
Edited by:
An Liu, City of Hope National Medical Center, United StatesReviewed by:
Bilgin Kadri Aribas, Bülent Ecevit University, TurkeyNing Wen, Henry Ford Health System, United States
Copyright © 2021 Li, Li, Jia, Li, Sun and Zuo. This is an open-access article distributed under the terms of the Creative Commons Attribution License (CC BY). The use, distribution or reproduction in other forums is permitted, provided the original author(s) and the copyright owner(s) are credited and that the original publication in this journal is cited, in accordance with accepted academic practice. No use, distribution or reproduction is permitted which does not comply with these terms.
*Correspondence: Gaofeng Sun, c2dmMTI1NkAxNjMuY29t; Changjing Zuo, Y2hhbmdqaW5nLnp1b0BxcS5jb20=