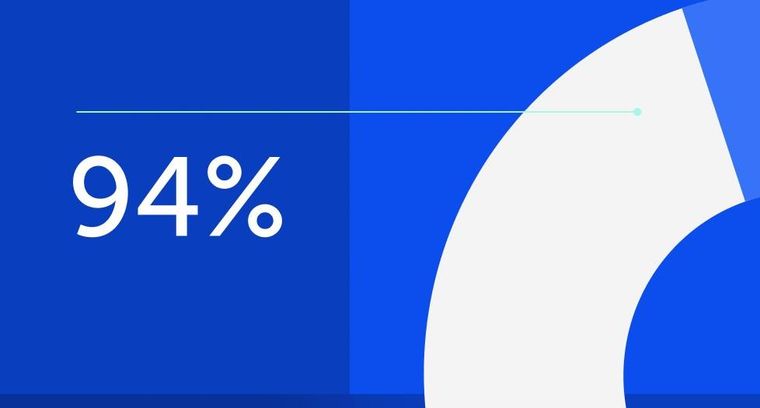
94% of researchers rate our articles as excellent or good
Learn more about the work of our research integrity team to safeguard the quality of each article we publish.
Find out more
MINI REVIEW article
Front. Oncol., 14 October 2020
Sec. Gastrointestinal Cancers
Volume 10 - 2020 | https://doi.org/10.3389/fonc.2020.524205
This article is part of the Research TopicImmunotherapy in Hepatocellular CarcinomaView all 12 articles
Primary liver cancer is one of the leading causes of cancer death worldwide. Surgical and non-surgical treatments are optional for liver cancer therapy based on the cancer stage. Accumulating studies show that the gut–liver axis influences the progression of liver diseases, including liver inflammation, fibrosis, cirrhosis, and cancer. However, the role of gut microbiota and their derived components and metabolites in liver cancer remains to be further clarified. In this review, we discuss the roles of gut microbiota and specific bacterial species in HCC and the strategies to modulate gut microbiota to improve antitumor therapy. Given the limitation of current treatments, gut microbiota-mediated therapy is a potential option for HCC treatment, including fiber diet and vegetable diet, antimicrobials, probiotics, and pharmaceutical inhibitors. Also, gut microbiota can be used as a marker for early diagnosis of HCC. HCC occurs dependent on various environmental and genetic factors, including diet and sex. Furthermore, gut microbiota impacts the immunotherapy of HCC treatment. Therefore, a better understanding of the role of the gut–liver axis in liver cancer is critically important to improve therapeutic efficacy.
Liver cancer is the fourth leading cause of cancer death worldwide (1). In the United States, there will be approximately 42,030 new cases of primary liver cancer and intrahepatic bile duct cancer and 31,780 deaths due to these cancers in 2019, according to the American Cancer Society’s estimate1. Hepatocellular carcinoma (HCC) is the most common type of primary liver cancer (2), and the incidence of HCC is predicted to rise continually in the next decade (3). HCC typically results from chronic liver disease (4), and the main risk factors causing HCC are hepatitis B or C viruses, alcohol abuse, non-alcoholic fatty liver disease (NAFLD), diabetes, and other metabolic and genetic diseases (5, 6). Early diagnosis of HCC in patients is critically important for treatment with good outcomes (7). Unfortunately, the determination of HCC is often made in advanced disease stages, which are frequently accompanied by liver dysfunction or failure (2).
There are multiple options available for HCC treatment, including surgical resection and non-surgical therapies (8). HCC treatment options selectively depend on the stage of the disease, liver function, and cost of treatment. Even though the survival of patients with HCC is prolonged, recurrence remains a major issue for HCC treatment. In the past few years, new molecular targeting agents have been approved for systemic treatment by the United States Food and Drug Administration (FDA) (9, 10). In 2019, the FDA approved cabozantinib (Cabomeyx, Exelixis, Inc.) treatment in HCC patients as the second-line2. Cabozantinib is a multi-tyrosine kinase inhibitor primarily targeting tyrosine-protein kinase Met (c-MET), vascular endothelial growth factor receptor 2 (VEGFR2), and tyrosine kinase receptors AXL and RET, which was initially approved to treat medullary thyroid cancer or advanced renal cell carcinoma (RCC) (11, 12). Given the complex pathogenesis of HCC, current therapies still fail to meet the needs of patients.
Gut microbiota and gut microbiota-derived products have been shown to play important roles in the pathogenesis of HCC and its therapy. For instance, lipoteichoic acid (LTA, a Gram-negative bacterial cell wall component) and deoxycholic acid (DCA, a secondary bile acid produced by bacteria) collaboratively induced the expression of prostaglandin-endoperoxide synthase 2 or cyclooxygenase-2 (COX-2) through Toll-like receptor 2 (TLR-2) in senescent hepatic stellate cells (HSCs) to enlarge prostaglandin E2 (PGE2)-mediated inhibition of antitumor immunity, resulting in HCC progression (13). It has been reported that gut microbiota-derived products can modulate hepatic inflammation and immunity to impact non-alcoholic steatohepatitis (NASH) and virus-induced HCC progression (14). HCC patients who are responsive to anti-programmed cell death protein 1 (PD-1) immunotherapy had higher taxa richness in fecal samples compared to non-responders (15). In addition, Akkermansia muciniphila and Ruminococcaceae spp. are enriched species in responder patients, while Proteobacteria increased in non-responders.
In this review, we first summarize current therapies for liver cancer. Then, we discuss the potential roles of gut microbiota in liver cancer and gut microbiota-mediated treatment and diagnosis for liver cancer, specifically focusing on the shift of gut microbiota in HCC development and treatment.
Currently, there are several treatment options for liver cancer, but the selection is highly dependent on the cancer stage and remaining liver health (16, 17). Surgical resection is one of the major curative treatment options for the primary liver tumor or metastatic liver tumor (18, 19). However, surgical treatment requires to be performed in the early stage of liver cancer with a low potential incidence of metastasis. When surgical resection is not an option, minimally invasive local therapies such as radiofrequency ablation (RFA), microwave ablation (MWA), high-intensity focused ultrasound (HIFU), and irreversible electroporation (IRE) become treatable options for both primary and metastatic liver tumors (20, 21). For widespread liver cancer, chemotherapy, immunotherapy, and targeted therapy may be preferable. For example, sorafenib, a multi-kinase inhibitor with anti-proliferative and anti-angiogenic effects, has represented the primary treatment for advanced HCC for a long time (22). It was the only FDA-approved systemic therapeutic agent for HCC treatment until the recent approval of five new agents. In newly approved agents, lenvatinib is optional in the first-line treatment, while regorafenib, nivolumab, pembrolizumab, and cabozantinib are used as second-line therapies (9). All of these treatment options could be applied according to the stage and size of liver tumor. The treatment options for liver cancer are listed in Table 1.
Cancer recurrence and therapeutic resistance are the main issues that reduce the survival outcomes of cancer patients (23). In this situation, combination therapy, treatment with two or more therapeutic agents or options, is helpful for good outcomes. For example, doxorubicin is a commonly used chemotherapy drug with trans-arterial chemoembolization (TACE) in HCC treatment (24). Tremelimumab, an immune checkpoint blocker, in combination with tumor ablation, is beneficial for patients with advanced HCC and viral infection as it can improve the infiltration of CD8+ T cells and reduce viral load (25).
The liver is directly exposed to gut microbial components and metabolites via the liver portal vein (26). Increasing studies show that the gut–liver axis influences the progression of liver diseases such as liver inflammation, fibrosis, cirrhosis, and cancer (27, 28). For instance, high-alcohol-producing bacterium Klebsiella pneumoniae is implicated in the pathogenesis of NAFLD in human patients, evidenced by oral gavage of a clinically isolated strain causing NAFLD in mice (29). Cirrhotic patients with or without HCC had a higher abundance of genera Lactobacillus and Bacteroides with LDA scores larger than 4.0, whereas healthy controls had a higher abundance of Akkermansia and Methanobrevibacter (30). Additionally, HCC patients possessed relatively greater abundance of Bacteroides and Ruminococcaceae and lower abundance of Bifidobacterium compared with cirrhotic patients without HCC.
Gut microbiota impacts liver cancer by modulating different factors, including bile acids, immune checkpoint inhibitors, and Toll-like receptors (TLRs), among others.
Bile acids (BAs) consist of primary and secondary bile acids. Primary BAs such as cholic acid (CA) and chenodeoxycholic acid (CDCA) are synthesized in hepatocytes from cholesterol, while secondary BAs such as deoxycholic acid (DCA) and lithocholic acid (LCA) are synthesized by the intestinal bacteria using the primary BAs (31, 32). While BAs play pivotal roles in glucose metabolism (33) and vitamin and lipid absorption (34), an overabundance of BAs can cause hepatocyte DNA damage to promote carcinogenesis by promoting the alteration of tumor suppressor genes and oncogenes (34). Ma et al. reported that the conversion of primary to secondary BAs impacted the infiltration of hepatic natural killer T cells (NKT cells), which controlled the progression of liver cancer in mouse (35). The accumulation of hepatic CXCR6+ NKT cells was mediated by the expression of CXCL16 in liver sinusoidal endothelial cells (LSECs). In human samples, the presence of primary bile acid CDCA was positively correlated with CXCL16 expression, with which the expression of secondary bile acid GLCA was inversely correlated (36). The bile acid biotransformation was influenced by gut microbial community (37), such as bacterial species Clostridium (35). These findings indicate that modulating gut microbiota can change the components of BAs to improve antitumor immunity. Furthermore, BA receptors, farnesoid X receptor (FXR), and G protein-coupled bile acid receptor 1 (TGR5) are the potential regulators for BA homeostasis and carcinogenic effects in liver cancer (34).
Immune checkpoint inhibitors are promising treatable options for HCC treatment or applied as an adjunct therapy (38). Cancer development is associated with immune suppression since cancer cells can activate different immune checkpoint pathways to inhibit antitumor therapies (39). Antibodies or inhibitors that block cytotoxic T-lymphocyte-associated antigen 4 (CTLA-4), PD-1, programmed cell death 1 ligand 1 (PD-L1), and CD24 show promising therapeutic effects on cancer treatment (39–41). Tremelimumab, a monoclonal antibody that blocks CTLA-4, was first tested in patients with HCC and hepatitis C virus infection (42, 43). The results indicated that tremelimumab treatment showed not only anti-HCC effect but also enhanced anti-HCV immunity.
Further clinical trials demonstrated the reliable adjunct antitumor effect of tremelimumab with the combination of subtotal RFA or chemoablation in patients with advanced HCC (25). The combination of anti-PD-1/PD-L1 with anti-CTLA-4 antibodies and the synergistic application of immune checkpoint inhibitors with other antitumor therapies are being evaluated at different stages of clinical trials. The results suggest that an anti-PD-1 antibody in combination with locoregional therapy or other targeted therapy is an effective treatment for HCC (44, 45). Immune checkpoint inhibitors have been shown to prolong the survival time in HCC patients (46). Therefore, Nivolumab, a monoclonal antibody that blocks the PD-1 receptor on T cells, was approved by the United States FDA for liver cancer treatment in 2017. Pembrolizumab (Keytruda), another immune checkpoint inhibitor for PD-1, was approved by the United States FDA for HCC treatment in 2018.
Importantly, increasing evidence shows that gut microbiota influences the efficacy of immune checkpoint antibodies, as antibiotic treatment can diminish their effectiveness by depletion of gut microbiome, while the presence of specific gut microbes increases this efficacy (47). Clinical studies have shown that some of the bacterial species enhanced the efficacy of immune checkpoint therapy (48), such as the effect of Bacteroides caccae on anti-CTLA-4 and anti-PD-1 in melanoma (49), and the impact of A. muciniphila on anti-PD-1 in non-small-cell lung carcinoma (NSCLC) and renal cell carcinoma (RCC) (50). Therefore, modulating gut microbial components to improve the antitumor effect of immune checkpoint inhibitors is a potential strategy for HCC treatment.
Toll-like receptors are the most well-studied family of pattern recognition receptors (PRRs) (51). TLRs can recognize pathogen-associated molecular patterns (PAMPs) and endogenous damage-associated molecular patterns (DAMPs) like tumor-derived antigens to activate the innate immune responses (52, 53). Gut dysbiosis, the disruption of the balance of gut microbiome, impacts the hepatic immune response through the gut-derived components like LPS and unmethylated CpG DNA, which can activate the TLR-signaling pathway (54). Even though the role of TLRs varies in different cancers (55), a series of studies have shown that targeting TLRs is a promising strategy for cancer immunotherapy (56, 57). In the liver, TLR4 and TLR9 play essential roles in the liver inflammation–fibrosis–cancer axis, as TLR4–/– or TLR9–/– Tak1ΔHep mice experience reduced spontaneous HCC development compared to Tak1ΔHep mice (58). Clinical investigations also show TLR4, the ligand of Gram-negative bacteria membrane component lipopolysaccharide (LPS) that plays a pathogenic role in chronic inflammation, a causative factor in human HCC (59). The expression of TLR9, the ligand of which is unmethylated CpG DNA in bacteria or viruses, has been positively associated with human colorectal cancer and liver metastasis (60). Thus, modulating gut microbiota to change TLR activity may serve as a therapeutic strategy for HCC therapy.
The composition of human gut microbiota can be modulated by various factors such as diet (61), lifestyle (62), antimicrobials (63, 64), environment (65), and diseases (66). Currently, probiotics and Fecal Microbiome Transplantation (FMT) are being investigated in cancer treatment as an adjuvant strategy to increase the efficacy of chemotherapy and immunotherapy (67). There are 80 recruiting or completed microbiota study trials associated with liver diseases on the website ClinicalTrials.gov with the keywords liver disease and microbiota, including NAFLD, NASH, fatty liver disease (FLD), alcoholic liver disease (ALD), HCC, liver encephalopathy, hepatitis, liver transplantation (LT), or resection. The strategies to affect change in the gut microbiota in those trials are summarized in Figure 1.
Figure 1. The strategies to change gut microbiota to prevent or ameliorate liver diseases in clinical trials. There are multiple strategies to restore the balance of gut microbiota such as fecal microbiota transplantation (FMT), drug therapy such as antibiotics (e.g., rifaximin) and proton pump inhibitor, pro/prebiotic, prebiotics or probiotics, change of lifestyle, and others including diet, drink, fatty acids, surgery, and genetic modification.
Overtake of soluble dietary fiber (e.g., Pectin and Fructooligosaccharide) that can be metabolized to short-chain fatty acids (SCFAs) by gut microbiota may cause cholestasis and HCC in mice, specifically with gut overgrowth of fiber-fermenting bacteria like Clostridium cluster XIVa (68). The authors also showed that administration of antibiotic metronidazole reduced butyrate-producing bacteria and the incidence of HCC in TLR5 knockout (KO) mice fed soluble fiber inulin-containing diet. Another study showed that vancomycin could prevent the development of HCC by selectively depleting Gram-positive bacteria Lachnospiraceae (Clostridium cluster XIVa), Ruminococcaceae, and Bifidobacteria, which ferment fiber and generate secondary bile acids (69). Feeding tomato powder (TP) could impede HFD plus diethylnitrosamine (DEN, injected once at 2 weeks of age)-induced HCC development in β-Carotene-15, 15′-oxygenase (BCO1), and β-carotene-9′, 10′-oxygenase (BCO2) double knockout mice (70). In addition, TP feeding altered the richness and diversity of gut microbiota, accompanying a significant decrease in the abundance of genera Clostridium and Mucispirillum. Another study reported that probiotics composed of Lactobacillus rhamnosus GG, viable probiotic Escherichia coli Nissle 1917, and heat-inactivated VSL#3 (1:1:1) could shift the gut microbiota to increase beneficial bacteria such as Prevotella and Oscillibacter, resulting in a reduction of HCC growth and Th17 cell differentiation (71). VSL#3 contains Streptococcus thermophilus, Bifidobacterium breve, Bifidobacterium longum, Bifidobacterium infantis, Lactobacillus acidophilus, Lactobacillus plantarum, Lactobacillus paracasei, and Lactobacillus delbrueckii subsp. Combined (synbiotic) prebiotic B. infantis and probiotic milk oligosaccharide treatment reverses Western diet (WD)-induced NASH in FXR knockout mice (72). Moreover, bariatric surgery, such as Roux-en-Y gastric bypass and laparoscopic sleeve gastrectomy, can induce the shift of gut microbiota to reduce obesity and weight loss (73), showing a promise in NAFLD and NASH (74). Thus, it may be a potent treatment option for early stage of NASH-HCC patients.
Early diagnosis of HCC comes with multiple treatment options and typically leads to good outcomes. Biomarkers including Alpha-fetoprotein (AFP), Lens culinaris agglutinin A-reactive fraction of alpha-fetoprotein (AFP-L3), and des-gamma-carboxy prothrombin (DCP) have been established as HCC-specific tumor markers (75, 76). New potential biomarkers, such as Aldo-keto reductase family 1 member 10 (AKR1B10) (77), are being investigated for the diagnosis and prognosis of HCC. Changes in the gut microbiome may also serve as biomarkers of disease as they have been associated with the progression of liver diseases, from fibrosis/cirrhosis to cancer (78, 79). For example, the abundance of fecal Enterobacteriaceae and Streptococcus is increased in patients with cirrhosis, while the abundance of Akkermansia is reduced. In HCC patients, Bacteroides and Ruminococcaceae were increased, while Bifidobacterium was reduced. Further study showed that Akkermansia and Bifidobacterium were inversely correlated with inflammatory marker calprotectin (30). These results indicated that during the development of HCC, a group of bacteria are associated with different stages of disease and tumor progression. A better understanding of the association of gut microbiota with liver cancer leads to a therapy option. Potent gut microbiota-mediated liver cancer therapies are summarized in Figure 2.
Figure 2. The development of liver cancer and gut microbiota-mediated therapy. Chronic liver diseases including viral infections, fatty liver disease (FLD), alcoholic liver disease (ALD), non-alcoholic fatty liver disease (NAFLD), non-alcoholic steatohepatitis (NASH), and cirrhosis without effective treatments can lead to liver cancer. Dysbiosis of gut microbiota promotes the progression of this process by leakage of gut microbial products such as deoxycholic acid (DCA), lipopolysaccharide (LPS), and unmethylated CpG DNA. These bacterial products promote liver inflammation, fibrosis, and cirrhosis. Modulation of gut microbiota by applying probiotics, prebiotics, and antibiotics, or using antagonists of bacterial products, can improve gut barrier and reduce the progression of the liver inflammation–fibrosis–cirrhosis–cancer axis. BAs, bile acids; TLR, Toll-like receptor.
Liver cancer is a leading cause of cancer deaths worldwide. Liver resection or transplantation is the curative treatment for HCC, but late diagnosis and lack of donor organs reduce the survival rate. Given these limitations, many non-surgical treatment options are available for advanced stages of HCC. However, the cost for some current treatments like sorafenib is relatively high, which may be associated with adverse or variable effects (80). Modulating gut microbiome is a potential option for liver cancer treatment and diagnosis. HCC occurs about three times more in men than in women (81). Therefore, sex is also another consideration when choosing gut microbiota-mediated treatment. In a streptozotocin–high-fat diet (STZ-HFD)-induced NASH-HCC murine model, male mice possessed a higher abundance of some specific genera than female mice, including Clostridium, Corynebacterium, Bacillus, Desulfovibrio, and Rhodococcus, which were associated with higher HCC incidence (82). Data from prospective cohort studies indicate that intake of vegetables reduces the risk of liver cancer development, especially for men (83). LT can also alter gut microbial profile. The abundance of bacteria, such as Actinobacillus, Escherichia, and Shigella, decreased post-LT compared to pre-LT, whereas the abundance of bacteria, such as Micromonosporaceae, Desulfobacterales, the Sarcina genus of Eubacteriaceae, and Akkermansia increased (84). Furthermore, features of the gut microbiota are also associated with hepatitis virus- and non-hepatitis virus-related HCC, evidenced by the fact that hepatitis B-HCC patients harbor much more pro-inflammatory bacteria such as Escherichia/Shigella and Enterococcus, but less amount of Faecalibacterium, Ruminococcus, and Ruminoclostridium relative to healthy controls (85). Therefore, precise analysis of the change of gut microbiota of each individual in the development of HCC is critically essential for modified treatment. Those recent findings suggest that microbiome-mediated therapeutic options can be applied to treat liver cancer as well as the early stage of chronic liver diseases, which may conquer the drawbacks of current therapies, such as the presence of metastasis and liver dysfunction. However, more clinical trials evaluating gut microbiota-mediated therapies are necessary to improve outcomes of HCC treatment.
CZ and MY conceived and wrote the manuscript. AE critically reviewed and revised the manuscript. All authors contributed to the article and approved the submitted version.
The review was supported by the Postdoctoral Research Grant of University of Missouri, Columbia, Missouri, United States.
The authors declare that the research was conducted in the absence of any commercial or financial relationships that could be construed as a potential conflict of interest.
1. Bray F, Ferlay J, Soerjomataram I, Siegel RL, Torre LA, Jemal A. Global cancer statistics 2018: GLOBOCAN estimates of incidence and mortality worldwide for 36 cancers in 185 countries. CA Cancer J Clin. (2018) 68:394–424. doi: 10.3322/caac.21492
2. Balogh J, Victor D III, Asham EH, Burroughs SG, Boktour M, Saharia A, et al. Hepatocellular carcinoma: a review. J Hepatocell Carcinoma. (2016) 3:41–53. doi: 10.2147/JHC.S61146
3. Mokdad AA, Hester CA, Singal AG, Yopp AC. Management of hepatocellular in the United States. Chin Clin Oncol. (2017) 6:21. doi: 10.21037/cco.2017.04.04
4. Yu L-X, Schwabe RF. The gut microbiome and liver cancer: mechanisms and clinical translation. Nat Rev Gastroenterol Hepatol. (2017) 14:527–39. doi: 10.1038/nrgastro.2017.72
5. Sia D, Villanueva A, Friedman SL, Llovet JM. Liver cancer cell of origin, molecular class, and effects on patient prognosis. Gastroenterology. (2017) 152:745–61. doi: 10.1053/j.gastro.2016.11.048
6. Zhang C, Yang M, Ericsson AC. Antimicrobial peptides: potential application in liver cancer. Front Microbiol. (2019) 10:1257. doi: 10.3389/fmicb.2019.01257
7. Erstad DJ, Tanabe KK. Hepatocellular carcinoma: early-stage management challenges. J Hepatocell Carcinoma. (2017) 4:81–92. doi: 10.2147/jhc.s107370
8. Raza A, Sood GK. Hepatocellular carcinoma review: current treatment, and evidence-based medicine. World J Gastroenterol. (2014) 20:4115–27. doi: 10.3748/wjg.v20.i15.4115
9. Bteich F, Di Bisceglie AM. Current and future systemic therapies for hepatocellular carcinoma. Gastroenterol Hepatol. (2019) 15:266–72.
10. Kudo M. Targeted and immune therapies for hepatocellular carcinoma: predictions for 2019 and beyond. World J Gastroenterol. (2019) 25:789–807. doi: 10.3748/wjg.v25.i7.789
11. Lyseng-Williamson KA. Cabozantinib as first-line treatment in advanced renal cell carcinoma: a profile of its use. Drugs Ther Perspect. (2018) 34:457–65. doi: 10.1007/s40267-018-0547-6
12. Ml BP, Miksad RA. Cabozantinib in the treatment of hepatocellular carcinoma. Future Oncol. (2017) 13:1915–29. doi: 10.2217/fon-2017-0169
13. Loo TM, Kamachi F, Watanabe Y, Yoshimoto S, Kanda H, Arai Y, et al. Gut microbiota promotes obesity-associated liver cancer through PGE(2)-mediated suppression of antitumor immunity. Cancer Discov. (2017) 7:522–38. doi: 10.1158/2159-8290.Cd-16-0932
14. Schwabe RF, Greten TF. Gut microbiome in HCC – mechanisms, diagnosis and therapy. J Hepatol. (2020) 72:230–8. doi: 10.1016/j.jhep.2019.08.016
15. Zheng Y, Wang T, Tu X, Huang Y, Zhang H, Tan D, et al. Gut microbiome affects the response to anti-PD-1 immunotherapy in patients with hepatocellular carcinoma. J Immunother Cancer. (2019) 7:193. doi: 10.1186/s40425-019-0650-9
16. Bruix J, Han KH, Gores G, Llovet JM, Mazzaferro V. Liver cancer: approaching a personalized care. J Hepatol. (2015) 62(1 Suppl.):S144–56. doi: 10.1016/j.jhep.2015.02.007
17. Kumari R, Sahu MK, Tripathy A, Uthansingh K, Behera M. Hepatocellular carcinoma treatment: hurdles, advances and prospects. Hepat Oncol. (2018) 5:He08. doi: 10.2217/hep-2018-0002
18. Dhir M, Sasson AR. Surgical management of liver metastases from colorectal cancer. J Oncol Pract. (2016) 12:33–9. doi: 10.1200/jop.2015.009407
19. Orcutt ST, Anaya DA. Liver resection and surgical strategies for management of primary liver cancer. Cancer Control. (2018) 25:1073274817744621. doi: 10.1177/1073274817744621
20. Li D, Kang J, Golas BJ, Yeung VW, Madoff DC. Minimally invasive local therapies for liver cancer. Cancer Biol Med. (2014) 11:217–36. doi: 10.7497/j.issn.2095-3941.2014.04.001
21. Dodd GD III, Soulen MC, Kane RA, Livraghi T, Lees WR, Yamashita Y, et al. Minimally invasive treatment of malignant hepatic tumors: at the threshold of a major breakthrough. Radiographics. (2000) 20:9–27. doi: 10.1148/radiographics.20.1.g00ja019
22. Colagrande S, Regini F, Taliani GG, Nardi C, Inghilesi AL. Advanced hepatocellular carcinoma and sorafenib: diagnosis, indications, clinical and radiological follow-up. World J Hepatol. (2015) 7:1041–53. doi: 10.4254/wjh.v7.i8.1041
23. Cidon EU. Systemic treatment of hepatocellular carcinoma: past, present and future. World J Hepatol. (2017) 9:797–807. doi: 10.4254/wjh.v9.i18.797
24. Greten TF, Mauda-Havakuk M, Heinrich B, Korangy F, Wood BJ. Combined locoregional-immunotherapy for liver cancer. J Hepatol. (2019) 70:999–1007. doi: 10.1016/j.jhep.2019.01.027
25. Duffy AG, Ulahannan SV, Makorova-Rusher O, Rahma O, Wedemeyer H, Pratt D, et al. Tremelimumab in combination with ablation in patients with advanced hepatocellular carcinoma. J Hepatol. (2017) 66:545–51. doi: 10.1016/j.jhep.2016.10.029
26. Tripathi A, Debelius J, Brenner DA, Karin M, Loomba R, Schnabl B, et al. The gut-liver axis and the intersection with the microbiome. Nat Rev Gastroenterol Hepatol. (2018) 15:397–411. doi: 10.1038/s41575-018-0011-z
27. Ohtani N, Kawada N. Role of the Gut-liver axis in liver inflammation, fibrosis, and cancer: a special focus on the Gut microbiota relationship. Hepatol Commun. (2019) 3:456–70. doi: 10.1002/hep4.1331
28. Wang L, Wan Y-JY. The role of gut microbiota in liver disease development and treatment. Liver Res. (2019) 3:3–18. doi: 10.1016/j.livres.2019.02.001
29. Yuan J, Chen C, Cui J, Lu J, Yan C, Wei X, et al. Fatty liver disease caused by high-alcohol-producing Klebsiella pneumoniae. Cell Metab. (2019) 30:1172. doi: 10.1016/j.cmet.2019.11.006
30. Ponziani FR, Bhoori S, Castelli C, Putignani L, Rivoltini L, Del Chierico F, et al. Hepatocellular carcinoma is associated with Gut microbiota profile and inflammation in nonalcoholic fatty liver disease. Hepatology. (2019) 69:107–20. doi: 10.1002/hep.30036
31. Šarenac TM, Mikov M. Bile acid synthesis: from nature to the chemical modification and synthesis and their applications as drugs and nutrients. Front Pharmacol. (2018) 9:939. doi: 10.3389/fphar.2018.00939
32. Ridlon JM, Kang DJ, Hylemon PB. Bile salt biotransformations by human intestinal bacteria. J Lipid Res. (2006) 47:241–59. doi: 10.1194/jlr.R500013-JLR200
33. Shapiro H, Kolodziejczyk AA, Halstuch D, Elinav E. Bile acids in glucose metabolism in health and disease. J Exp Med. (2018) 215:383. doi: 10.1084/jem.20171965
34. Wang X, Fu X, Van Ness C, Meng Z, Ma X, Huang W. Bile acid receptors and liver cancer. Curr Pathobiol Rep. (2013) 1:29–35. doi: 10.1007/s40139-012-0003-6
35. Ma C, Han M, Heinrich B, Fu Q, Zhang Q, Sandhu M, et al. Gut microbiome-mediated bile acid metabolism regulates liver cancer via NKT cells. Science. (2018) 360:eaan5931. doi: 10.1126/science.aan5931
36. Jia B. Commentary: gut microbiome-mediated bile acid metabolism regulates liver cancer via NKT cells. Front Immunol. (2019) 10:282. doi: 10.3389/fimmu.2019.00282
37. Staley C, Weingarden AR, Khoruts A, Sadowsky MJ. Interaction of gut microbiota with bile acid metabolism and its influence on disease states. Appl Microbiol Biotechnol. (2017) 101:47–64. doi: 10.1007/s00253-016-8006-6
38. Liu X, Qin S. Immune checkpoint inhibitors in hepatocellular carcinoma: opportunities and challenges. Oncologist. (2019) 24(Suppl. 1):S3–10. doi: 10.1634/theoncologist.2019-IO-S1-s01
39. Darvin P, Toor SM, Sasidharan Nair V, Elkord E. Immune checkpoint inhibitors: recent progress and potential biomarkers. Exp Mol Med. (2018) 50:165. doi: 10.1038/s12276-018-0191-1
40. Azoury SC, Straughan DM, Shukla V. Immune checkpoint inhibitors for cancer therapy: clinical efficacy and safety. Curr Cancer Drug Targets. (2015) 15:452–62.
41. Barkal AA, Brewer RE, Markovic M, Kowarsky M, Barkal SA, Zaro BW, et al. CD24 signalling through macrophage Siglec-10 is a target for cancer immunotherapy. Nature. (2019) 572:392–6. doi: 10.1038/s41586-019-1456-0
42. Sangro B, Gomez-Martin C, de la Mata M, Iñarrairaegui M, Garralda E, Barrera P, et al. A clinical trial of CTLA-4 blockade with tremelimumab in patients with hepatocellular carcinoma and chronic hepatitis C. J Hepatol. (2013) 59:81–8. doi: 10.1016/j.jhep.2013.02.022
43. Seidel JA, Otsuka A, Kabashima K. Anti-PD-1 and Anti-CTLA-4 therapies in cancer: mechanisms of action, efficacy, and limitations. Front Oncol. (2018) 8:86. doi: 10.3389/fonc.2018.00086
44. Greten TF, Lai CW, Li G, Staveley-O’Carroll KF. Targeted and immune-based therapies for hepatocellular carcinoma. Gastroenterology. (2019) 156:510–24. doi: 10.1053/j.gastro.2018.09.051
45. Xu F, Jin T, Zhu Y, Dai C. Immune checkpoint therapy in liver cancer. J Exp Clin Cancer Res. (2018) 37:110. doi: 10.1186/s13046-018-0777-4
46. Mahipal A, Tella SH, Kommalapati A, Lim A, Kim R. Immunotherapy in hepatocellular carcinoma: is there a light at the end of the tunnel? Cancers. (2019) 11:1078. doi: 10.3390/cancers11081078
47. Zitvogel L, Ma Y, Raoult D, Kroemer G, Gajewski TF. The microbiome in cancer immunotherapy: diagnostic tools and therapeutic strategies. Science. (2018) 359:1366–70. doi: 10.1126/science.aar6918
48. Frankel AE, Deshmukh S, Reddy A, Lightcap J, Hayes M, McClellan S, et al. Cancer immune checkpoint inhibitor therapy and the gut microbiota. Integr Cancer Ther. (2019) 18:1534735419846379. doi: 10.1177/1534735419846379
49. Frankel AE, Coughlin LA, Kim J, Froehlich TW, Xie Y, Frenkel EP, et al. Metagenomic shotgun sequencing and unbiased metabolomic profiling identify specific human gut microbiota and metabolites associated with immune checkpoint therapy efficacy in melanoma patients. Neoplasia. (2017) 19:848–55. doi: 10.1016/j.neo.2017.08.004
50. Routy B, Le Chatelier E, Derosa L, Duong CPM, Alou MT, Daillere R, et al. Gut microbiome influences efficacy of PD-1-based immunotherapy against epithelial tumors. Science. (2018) 359:91–7. doi: 10.1126/science.aan3706
51. Mogensen TH. Pathogen recognition and inflammatory signaling in innate immune defenses. Clin Microbiol Rev. (2009) 22:240–73. doi: 10.1128/CMR.00046-08
52. Nie L, Cai S-Y, Shao J-Z, Chen J. Toll-like receptors, associated biological roles, and signaling networks in non-mammals. Front Immunol. (2018) 9:1523. doi: 10.3389/fimmu.2018.01523
53. Kawai T, Akira S. The role of pattern-recognition receptors in innate immunity: update on Toll-like receptors. Nat Immunol. (2010) 11:373–84. doi: 10.1038/ni.1863
54. Konturek PC, Harsch IA, Konturek K, Schink M, Konturek T, Neurath MF, et al. Gut–liver axis: how do gut bacteria influence the liver? Med Sci. (2018) 6:79. doi: 10.3390/medsci6030079
55. Zou H, Wang WK, Liu YL, Braddock M, Zheng MH, Huang DS. Toll-like receptors in hepatocellular carcinoma: potential novel targets for pharmacological intervention. Expert Opin Ther Targets. (2016) 20:1127–35. doi: 10.1517/14728222.2016.1168809
56. Mikulandra M, Pavelic J, Glavan TM. Recent findings on the application of toll-like receptors agonists in cancer therapy. Curr Med Chem. (2017) 24:2011–32. doi: 10.2174/0929867324666170320114359
57. Shi M, Chen X, Ye K, Yao Y, Li Y. Application potential of toll-like receptors in cancer immunotherapy: systematic review. Medicine. (2016) 95:e3951. doi: 10.1097/MD.0000000000003951
58. Song IJ, Yang YM, Inokuchi-Shimizu S, Roh YS, Yang L, Seki E. The contribution of toll-like receptor signaling to the development of liver fibrosis and cancer in hepatocyte-specific TAK1-deleted mice. Int J Cancer. (2018) 142:81–91. doi: 10.1002/ijc.31029
59. Sepehri Z, Kiani Z, Kohan F, Alavian SM, Ghavami S. Toll like receptor 4 and hepatocellular carcinoma; a systematic review. Life Sci. (2017) 179:80–7. doi: 10.1016/j.lfs.2017.04.025
60. Gao C, Qiao T, Zhang B, Yuan S, Zhuang X, Luo Y. TLR9 signaling activation at different stages in colorectal cancer and NF-kappaB expression. OncoTargets Ther. (2018) 11:5963–71. doi: 10.2147/OTT.S174274
61. Singh RK, Chang H-W, Yan D, Lee KM, Ucmak D, Wong K, et al. Influence of diet on the gut microbiome and implications for human health. J Transl Med. (2017) 15:73. doi: 10.1186/s12967-017-1175-y
62. Conlon MA, Bird AR. The impact of diet and lifestyle on gut microbiota and human health. Nutrients. (2015) 7:17–44.
63. Jernberg C, Lofmark S, Edlund C, Jansson JK. Long-term impacts of antibiotic exposure on the human intestinal microbiota. Microbiology. (2010) 156(Pt 11):3216–23. doi: 10.1099/mic.0.040618-0
64. Zhang C, Yang M. The role and potential application of antimicrobial peptides in autoimmune diseases. Front Immunol. (2020) 11:859. doi: 10.3389/fimmu.2020.00859
65. Rothschild D, Weissbrod O, Barkan E, Kurilshikov A, Korem T, Zeevi D, et al. Environment dominates over host genetics in shaping human gut microbiota. Nature. (2018) 555:210. doi: 10.1038/nature25973
66. Thursby E, Juge N. Introduction to the human gut microbiota. Biochem J. (2017) 474:1823. doi: 10.1042/BCJ20160510
67. Vivarelli S, Salemi R, Candido S, Falzone L, Santagati M, Stefani S, et al. Gut microbiota and cancer: from pathogenesis to therapy. Cancers. (2019) 11:38. doi: 10.3390/cancers11010038
68. Singh V, Yeoh BS, Chassaing B, Xiao X, Saha P, Aguilera Olvera R, et al. Dysregulated microbial fermentation of soluble fiber induces cholestatic liver cancer. Cell. (2018) 175:679–94.e22. doi: 10.1016/j.cell.2018.09.004
69. Singh V, Yeoh BS, Abokor AA, Golonka RM, Tian Y, Patterson AD, et al. Vancomycin prevents fermentable fiber-induced liver cancer in mice with dysbiotic gut microbiota. Gut Microbes. (2020) 11:1077–91. doi: 10.1080/19490976.2020.1743492
70. Xia H, Liu C, Li CC, Fu M, Takahashi S, Hu KQ, et al. Dietary tomato powder inhibits high-fat diet-promoted hepatocellular carcinoma with alteration of gut microbiota in mice lacking carotenoid cleavage enzymes. Cancer Prev Res. (2018) 11:797–810. doi: 10.1158/1940-6207.Capr-18-0188
71. Li J, Sung CY, Lee N, Ni Y, Pihlajamäki J, Panagiotou G, et al. Probiotics modulated gut microbiota suppresses hepatocellular carcinoma growth in mice. Proc Natl Acad Sci USA. (2016) 113:E1306–15. doi: 10.1073/pnas.1518189113
72. Jena PK, Sheng L, Nagar N, Wu C, Barile D, Mills DA, et al. Synbiotics Bifidobacterium infantis and milk oligosaccharides are effective in reversing cancer-prone nonalcoholic steatohepatitis using western diet-fed FXR knockout mouse models. J Nutr Biochem. (2018) 57:246–54. doi: 10.1016/j.jnutbio.2018.04.007
73. Palmisano S, Campisciano G, Silvestri M, Guerra M, Giuricin M, Casagranda B, et al. Changes in Gut microbiota composition after bariatric surgery: a new balance to decode. J Gastrointest Surg. (2019) 24:1736–46. doi: 10.1007/s11605-019-04321-x
74. Taitano AA, Markow M, Finan JE, Wheeler DE, Gonzalvo JP, Murr MM. Bariatric surgery improves histological features of nonalcoholic fatty liver disease and liver fibrosis. J Gastrointest Surg. (2015) 19:429–36; discussion 436–7. doi: 10.1007/s11605-014-2678-y
75. Toyoda H, Kumada T, Tada T, Sone Y, Kaneoka Y, Maeda A. Tumor markers for hepatocellular carcinoma: simple and significant predictors of outcome in patients with HCC. Liver Cancer. (2015) 4:126–36. doi: 10.1159/000367735
76. Zhao Y-J, Ju Q, Li G-C. Tumor markers for hepatocellular carcinoma. Mol Clin Oncol. (2013) 1:593–8. doi: 10.3892/mco.2013.119
77. DiStefano JK, Davis B. Diagnostic and prognostic potential of AKR1B10 in human hepatocellular carcinoma. Cancers. (2019) 11:486. doi: 10.3390/cancers11040486
78. Meng X, Li S, Li Y, Gan R-Y, Li H-B. Gut microbiota’s relationship with liver disease and role in hepatoprotection by dietary natural products and probiotics. Nutrients. (2018) 10:1457. doi: 10.3390/nu10101457
79. Ponziani FR, Nicoletti A, Gasbarrini A, Pompili M. Diagnostic and therapeutic potential of the gut microbiota in patients with early hepatocellular carcinoma. Ther Adv Med Oncol. (2019) 11:1758835919848184. doi: 10.1177/1758835919848184
80. Palmer DH, Hussain SA, Smith AJ, Hargreaves S, Ma YT, Hull D, et al. Sorafenib for advanced hepatocellular carcinoma (HCC): impact of rationing in the United Kingdom. Br J Cancer. (2013) 109:888–90. doi: 10.1038/bjc.2013.410
81. Wu EM, Wong LL, Hernandez BY, Ji J-F, Jia W, Kwee SA, et al. Gender differences in hepatocellular cancer: disparities in nonalcoholic fatty liver disease/steatohepatitis and liver transplantation. Hepatoma Res. (2018) 4:66. doi: 10.20517/2394-5079.2018.87
82. Xie G, Wang X, Zhao A, Yan J, Chen W, Jiang R, et al. Sex-dependent effects on gut microbiota regulate hepatic carcinogenic outcomes. Sci Rep. (2017) 7:45232. doi: 10.1038/srep45232
83. Guo XF, Shao XF, Li JM, Li S, Li KL, Li D. Fruit and vegetable intake and liver cancer risk: a meta-analysis of prospective cohort studies. Food Funct. (2019) 10:4478–85. doi: 10.1039/c9fo00804g
84. Sun L-Y, Yang Y-S, Qu W, Zhu Z-J, Wei L, Ye Z-S, et al. Gut microbiota of liver transplantation recipients. Sci Rep. (2017) 7:3762. doi: 10.1038/s41598-017-03476-4
85. Liu Q, Li F, Zhuang Y, Xu J, Wang J, Mao X, et al. Alteration in gut microbiota associated with hepatitis B and non-hepatitis virus related hepatocellular carcinoma. Gut Pathog. (2019) 11:1. doi: 10.1186/s13099-018-0281-6
86. Nathan H, Schulick RD, Choti MA, Pawlik TM. Predictors of survival after resection of early hepatocellular carcinoma. Ann Surg. (2009) 249:799–805. doi: 10.1097/SLA.0b013e3181a38eb5
87. Tsim NC, Frampton AE, Habib NA, Jiao LR. Surgical treatment for liver cancer. World J Gastroenterol. (2010) 16:927–33. doi: 10.3748/wjg.v16.i8.927
88. Madkhali AA, Fadel ZT, Aljiffry MM, Hassanain MM. Surgical treatment for hepatocellular carcinoma. Saudi J Gastroenterol. (2015) 21:11–7. doi: 10.4103/1319-3767.151216
89. Attallah AM, El-Far M, Zahran F, Shiha GE, Farid K, Omran MM, et al. Interferon-gamma is associated with hepatic dysfunction in fibrosis, cirrhosis, and hepatocellular carcinoma. J Immunoassay Immunochem. (2016) 37:597–610. doi: 10.1080/15321819.2016.1179646
90. Bala MM, Riemsma RP, Wolff R, Pedziwiatr M, Mitus JW, Storman D, et al. Cryotherapy for liver metastases. Cochrane Database Syst Rev. (2019) 7:Cd009058. doi: 10.1002/14651858.CD009058.pub3
91. Ryan MJ, Willatt J, Majdalany BS, Kielar AZ, Chong S, Ruma JA, et al. Ablation techniques for primary and metastatic liver tumors. World J Hepatol. (2016) 8:191–9. doi: 10.4254/wjh.v8.i3.191
92. Rammohan A, Sathyanesan J, Ramaswami S, Lakshmanan A, Senthil-Kumar P, Srinivasan UP, et al. Embolization of liver tumors: past, present and future. World J Radiol. (2012) 4:405–12. doi: 10.4329/wjr.v4.i9.405
93. Wáng Y-XJ, Baere T. De, Idée J-M, Ballet S. Transcatheter embolization therapy in liver cancer: an update of clinical evidences. Chin J Cancer Res. (2015) 27:96–121. doi: 10.3978/j.issn.1000-9604.2015.03.03
94. Katsanos K, Kitrou P, Spiliopoulos S, Maroulis I, Petsas T, Karnabatidis D. Comparative effectiveness of different transarterial embolization therapies alone or in combination with local ablative or adjuvant systemic treatments for unresectable hepatocellular carcinoma: a network meta-analysis of randomized controlled trials. PLoS One. (2017) 12:e0184597. doi: 10.1371/journal.pone.0184597
95. Fukumitsu N, Okumura T, Sakurai H. Radiotherapy for liver cancer. J Gen Fam Med. (2017) 18:126–30. doi: 10.1002/jgf2.19
96. Sundram FX, Buscombe JR. Selective internal radiation therapy for liver tumours. Clin Med. (2017) 17:449–53. doi: 10.7861/clinmedicine.17-5-449
97. Chen CP. Role of radiotherapy in the treatment of hepatocellular carcinoma. J Clin Transl Hepatol. (2019) 7:183–90. doi: 10.14218/jcth.2018.00060
98. Kudo M. Targeted therapy for liver cancer: updated review in 2012. Curr Cancer Drug Targets. (2012) 12:1062–72.
99. Chua CWL, Choo SP. Targeted therapy in hepatocellular carcinoma. Int J Hepatol. (2011) 2011:348297. doi: 10.4061/2011/348297
100. Greten TF, Sangro B. Targets for immunotherapy of liver cancer. J Hepatol. (2017) 68:157–66. doi: 10.1016/j.jhep.2017.09.007
101. Cariani E, Missale G. Immune landscape of hepatocellular carcinoma microenvironment: implications for prognosis and therapeutic applications. Liver Int. (2019) 39:1608–21. doi: 10.1111/liv.14192
102. Chu H, Duan Y, Yang L, Schnabl B. Small metabolites, possible big changes: a microbiota-centered view of non-alcoholic fatty liver disease. Gut. (2019) 68:359. doi: 10.1136/gutjnl-2018-316307
103. Le Grazie M, Biagini MR, Tarocchi M, Polvani S, Galli A. Chemotherapy for hepatocellular carcinoma: the present and the future. World J Hepatol. (2017) 9:907–20. doi: 10.4254/wjh.v9.i21.907
Keywords: liver cancer, treatments, sex, gut microbiota, clinical trials
Citation: Zhang C, Yang M and Ericsson AC (2020) The Potential Gut Microbiota-Mediated Treatment Options for Liver Cancer. Front. Oncol. 10:524205. doi: 10.3389/fonc.2020.524205
Received: 28 April 2020; Accepted: 21 September 2020;
Published: 14 October 2020.
Edited by:
Weijia Fang, Zhejiang University, ChinaCopyright © 2020 Zhang, Yang and Ericsson. This is an open-access article distributed under the terms of the Creative Commons Attribution License (CC BY). The use, distribution or reproduction in other forums is permitted, provided the original author(s) and the copyright owner(s) are credited and that the original publication in this journal is cited, in accordance with accepted academic practice. No use, distribution or reproduction is permitted which does not comply with these terms.
*Correspondence: Aaron C. Ericsson, ZXJpY3Nzb25hQG1pc3NvdXJpLmVkdQ==; Ming Yang, eWFuZ21pbkBoZWFsdGgubWlzc291cmkuZWR1
Disclaimer: All claims expressed in this article are solely those of the authors and do not necessarily represent those of their affiliated organizations, or those of the publisher, the editors and the reviewers. Any product that may be evaluated in this article or claim that may be made by its manufacturer is not guaranteed or endorsed by the publisher.
Research integrity at Frontiers
Learn more about the work of our research integrity team to safeguard the quality of each article we publish.