- 1Department of Ultrasound, Institute of Ultrasound in Musculoskeletal Sports Medicine, Guangdong Second Provincial General Hospital, Southern Medical University, Guangzhou, China
- 2Key Laboratory of Carcinogenesis and Translational Research (Ministry of Education/Beijing), Department of Ultrasound, Peking University Cancer Hospital & Institute, Beijing, China
Previous studies showed that radiofrequency ablation (RFA) has a favorable treatment efficacy for hepatocellular carcinoma (HCC) or colorectal liver metastases (CRLMs). Palliative RFA (pRFA) resulting from larger HCC or multiple CRLMs further accelerated the progression of potential residual tumor, yet its mechanism was still unknown. This study investigated the influence of myeloid-derived suppressor cells (MDSCs) on T-cell immune responses and tumor recurrence after pRFA. CT26 tumor models were used. The percentage of MDSCs in peripheral blood was analyzed by flow cytometry after pRFA. The level of Th1 and Th2 cytokines were measured by ELISA through different treatments (n = 4/group). The tumor-infiltrating MDSCs, dendritic cells, and intracellular cytokines level were analyzed by IHC staining after different treatments. The functional CD8+ T cells were confirmed by the co-localization immunofluorescence staining. The long-term outcomes were also evaluated through CT26 and 4T1 tumor models. The results showed that tumor models treated with pRFA displayed significant increases in the percentage of MDSCs of peripheral blood and tumor infiltration. The expression level of TGF-β and IL-6 after pRFA was higher than that before pRFA by ELISA and IHC staining. After depleting MDSCs by combining with Abs, the pRFA + Abs group achieved a higher level of Th1 cytokines and greatly enhanced the percentage of tumor-infiltrating functional CD8+ T cells when compared with pRFA alone. The depletion of MDSCs through combination with Abs also resulted in tumor regression. In conclusion, pRFA accelerates the residual tumor progression through increasing tumor-infiltrating MDSCs and reducing T-cell-mediated anti-tumor immune responses, which could provide a potential approach for delaying tumor recurrence caused by pRFA.
Introduction
Radiofrequency ablation (RFA) has attracted increasing attention as a minimally invasive treatment for many tumors, especially hepatocellular carcinoma (HCC) and colorectal liver metastases (CRLM) (1, 2). Radiofrequency ablation has no inferior therapeutic effects when compared with liver resection for small HCC (≤5 cm) or solitary CRLM (3, 4). Previous studies in animal models have demonstrated that in addition to inducing local coagulation necrosis, RFA could also lead to the release of abundant amounts of cell debris, which can serve as the source of tumor antigens to induce anti-tumor immunity mediated by tumor-specific T cells (5–7). However, the antitumor immune response by RFA was insufficient to inhibit tumor recurrence and progression, even palliative RFA (pRFA) resulting from larger HCC or multiple CRLM further accelerated the progression of a potential residual tumor, but the mechanism causing this was unknown.
Recent studies have displayed that an ability to evade immune destruction plays an important role in tumor recurrence and progression (8–10). These studies also demonstrated that myeloid-derived suppressor cells (MDSCs), as immature immune suppressor cells mainly marked by Gr1+CD11b+ in mice, can be recruited and activated in the inflammatory condition and tumor microenvironment (TME) (11). In the TME MDSCs can directly or indirectly impair T cell-mediated immune responses, thus inhibiting the release of several anti-tumor cytokines, such as IFN-γ and TNF-α, and weakening immune destruction for tumors (12). Evidence from clinical and pre-clinical studies also confirmed that the proportion of MDSCs in peripheral blood or tumor tissues was inversely associated with survival, and that inhibiting the expansion and activity of MDSCs can significantly improve anti-tumor therapeutic effects (13–15). However, it is still unclear whether MDSCs are involved in regulating the anti-tumor Tcell immune responses induced by pRFA, leading to the residual tumor recurrence. Therefore, this study aimed to examine the influence of MDSCs on T cell-driven response and tumor recurrence after pRFA.
In this study, we firstly selected the murine CT26 and 4T1 cancer model commonly used in the immune experiment. We analyzed the influence of pRFA on the recruitment and intratumoral infiltration of MDSCs by flow cytometry and immunohistochemical staining. Meanwhile, we evaluated the effect of MDSCs on the T cell-driven response induced by pRFA through combining MDSCs depletion. The successful implementation of the findings of this study will provide an effective potential approach for delaying tumor recurrence after pRFA.
Materials and Methods
The experimental animal protocol was first approved by the Institutional Animal Care and Use Committee of Peking University Cancer Hospital. In this study, the murine CT26 colon cancer model was used in the first two parts below, and the 4T1 and CT26 tumor models were used in the long-term follow-up. This study was employed in three phases to evaluate and analyze the influence of pRFA on the recruitment and intratumoral infiltration of MDSCs and the effect of MDSCs on tumor recurrence after pRFA, the details of RFA and the long-term outcomes from 4T1 tumor models are included in this article’s Supplementary Material.
The Influence of pRFA on T Cells and MDSCs
Flow cytometry was performed to analyze the influence of RFA on the CD4, CD8, MDSCs via mice bearing CT26 colon carcinoma (n = 4). At –1, 3, and 8 days after RFA, 300 μL of blood for each one was harvested by canthus ophthalmic vein and transferred into heparinized tubes. Then the whole blood cells were stained by with FITC-conjugated CD45, PE-conjugated CD8a, APC-conjugated CD4, FITC-conjugated CD11b, PE-conjugated Ly6G/Ly6C (GR1). After 15 min, red blood cells lysate was added and then suspended in flow cytometry buffer. Finally, analysis was carried out by flow cytometry using a FACS flow cytometer (Becton Dickinson).
In order to analyze the percentage of tumor-infiltrating MDSCs, we performed immunohistochemical analysis. Firstly, tumor tissues were fixed in 10% formalin and embedded in paraffin in accordance with standard procedures. Sections were stained with anti-Ly-6G/Ly-6C antibodies (BioLegend, San Diego, CA, United States). The antibody was replaced with non-immune serum to serve as a negative control. After anti-Ly-6G/Ly-6C antibodies staining, observations were performed with a microscope for immunohistochemical staining analysis, and representative digital images were obtained.
The Effect of MDSCs on Anti-Tumor Immune Response After pRFA Plus Abs
According to the manufacturer’s protocols, specific ELISA kits for the level of Th1 cytokines (IFN-γ and TNF-α) and Th2 cytokines (TGF-β and IL-1β) were used to measure cytokine levels in serum and conditioned media for different treatment groups (n = 4/group), including antibody alone (Abs), RFA alone, the combination of RFA with Abs (RFA + Abs).
To identify tumor-infiltrating dendritic cells (DC), immunofluorescence staining was performed by using a polyclonal American hamster PE-labeled CD11c antibody. The antibody was replaced with non-immune serum to serve as a negative control. After CD11c antibody staining, the slides were counterstained and mounted with a medium containing 4, 6-diamidine-2-phenylindole (DAPI). To further determine the function of CD8+ T cells, co-localization immunofluorescence staining was also carried out. After PE-labeled CD8 antibody staining, the slides were counterstained with FITC-labeled IFN-γ antibody. Representative digital images were obtained and analyzed by fluorescence microscope. Images were independently analyzed by two blinded evaluators. Each specimen was divided into 5 fields.
Long-Term Outcomes
Thirty-two mice bearing CT26 tumors were used in this phase. Tumors measuring 10–12 mm in size were randomized into 4 experimental groups for tumor growth (n = 8/group). These groups included: (a) control (PBS only), (b)Abs, (c) RFA, and (d) RFA + Abs. The mice receiving PBS without RFA or Abs were used as the control. The tumor volume of each mouse was recorded every 3 days for three weeks. After finishing this experiment, tumors were harvested from mice, and then the weight of each tumor was measured.
GR1 Depletion
Systemic GR1 depletion was achieved by intraperitoneal injection of 300 mg of purified monoclonal anti-Gr1 antibody RB6-8C5 (Abs, BioXCell, West Lebanon, NH, United States) at days – 1, 0, 3, 5, and 7 after RFA, and the volume of tumor was followed.
Statistical Analysis
All continuous data were provided as means of standard deviations. The Student’s t-test and ANOVA were used to evaluate the measurement of data. The ANOVA for repeated measurement data was used to compare tumor size and tumor weight at different time courses between different groups. In all cases, P < 0.05 was considered statistically significant. SPSS 21.0 statistical analysis software (SPSS, Chicago, IL, United States) was used.
Results
The Influence of RFA on T Cells and MDSCs
The results from flow cytometry analysis showed that the percentage of MDSCs in peripheral blood at 3 days after RFA was 1.9-fold higher than that before RFA (P < 0.001), and the percentage of MDSCs in peripheral blood had no significant change at 3 days and 8 days after RFA (P > 0.05) (Figures 1A,B). For the CD4/CD8 ratios, the ratio of CD4/CD8 at 3 days after RFA was lower than that at 1 day before RFA (6.15 vs 4.56, P < 0.001), and the ratio of CD4/CD8 at 8 days after RFA continued to decrease compared to that at 3 days after RFA (4.08 vs 4.56, P < 0.01) (Supplementary Figures S1A,B).
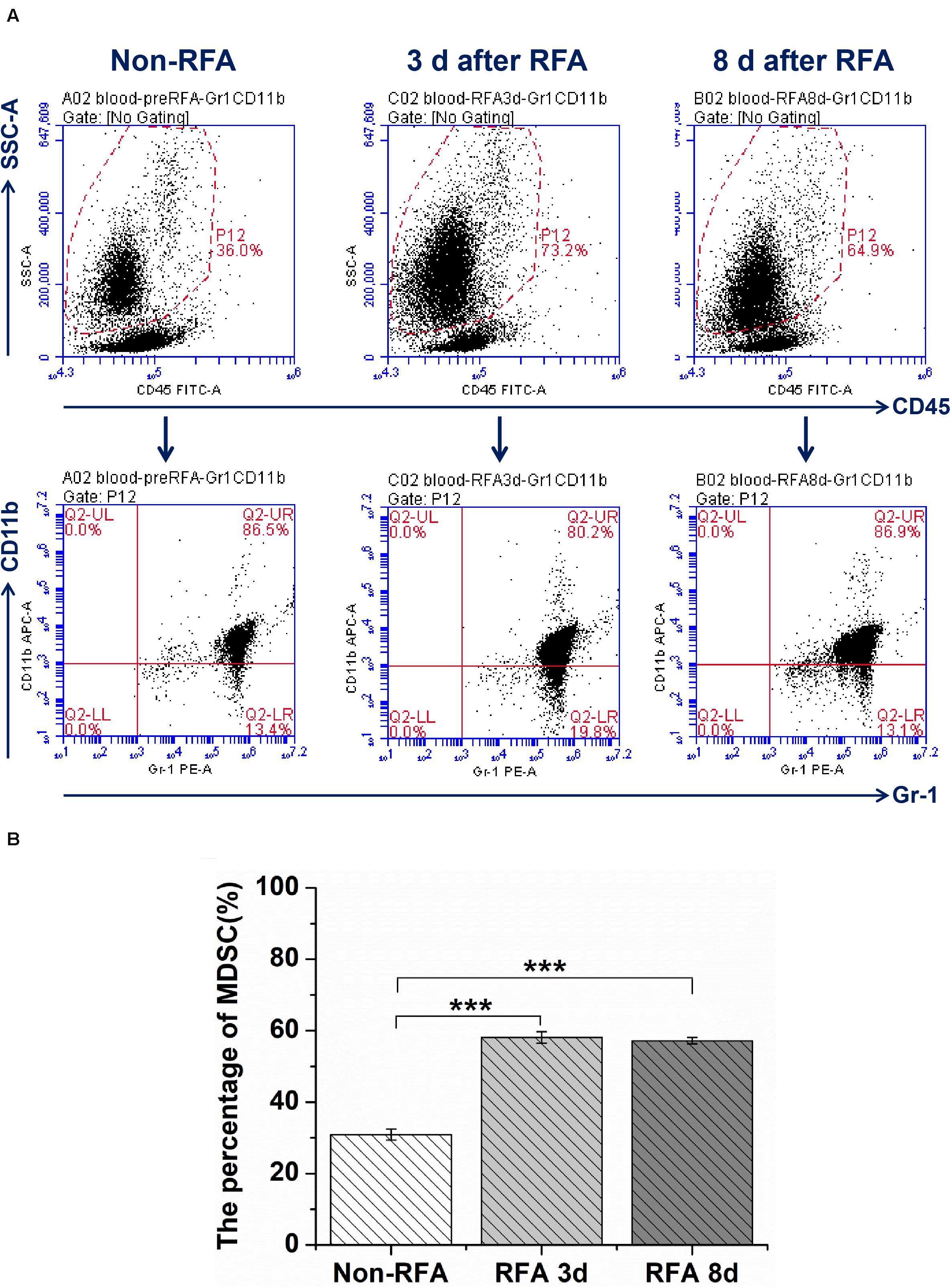
Figure 1. The flow cytometry analysis for MDSCs was performed through whole blood cells from mice bearing tumor treated with different treatment groups at different time points (Non-RFA, 3 days after RFA, 8 days after RFA). (A) the representative images from one experiment show a shift in the MDSCs population by flow cytometry analysis. (B) The percentage of MDSCs from different treatment groups at different time points, ***P < 0.001, **P < 0.01.
To further investigate the influence of RFA on intratumoral MDSCs, immunohistochemical staining was used to analyze the tumor-infiltrating MDSCs by tumor sections from mice treated with different treatments. From the images of immunohistochemical staining, we found that RFA significantly increased the percentage of tumor-infiltrating Gr-1 positive cells (Figure 2A). The quantitative analysis showed that the percentage of Gr-1 positive cells at 8 days after RFA was 10.4-fold higher than that before RFA (P < 0.001) (Figure 2B).
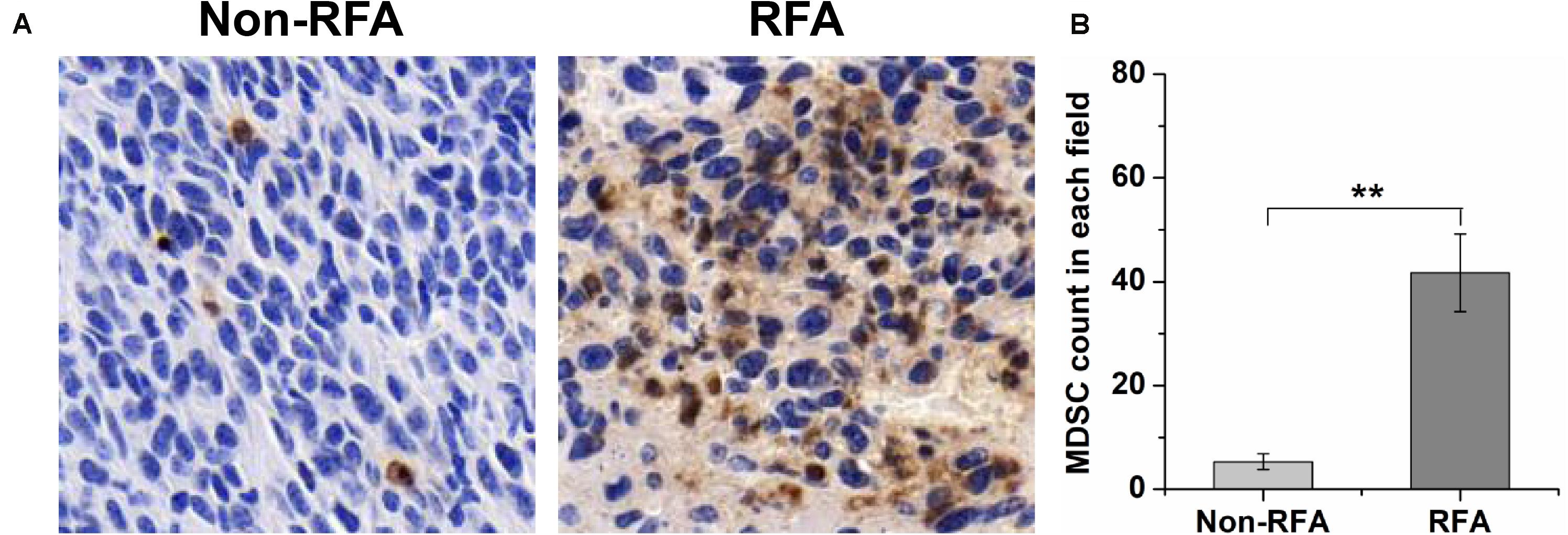
Figure 2. At 8 days after RFA, tumors were taken out and sectioned, and the Gr-1 staining was performed to analyze MDSC infiltration. (A) the representative plots about tumor-infiltrating MDSCs before or after RFA. (B) The quantitative analysis showed that the percentage of Gr-1 positive cells at 8 days after RFA was 10.4-fold higher than that before RFA (***P < 0.01).
The Effect of Anti-Tumor Immune Response After pRFA Plus Abs
The ELISA assays were used to evaluate systemic immune responses by measuring the level of cytokine in vivo in different treatments. The data showed that the level of IFN-γ increased in the first 3 days after RFA but decreased from 3 to 8 days after RFA alone. Meanwhile, the level of IFN-γ was on the rise within 8 days after RFA + Abs, and there were significant differences in the level of IFN-γ before RFA when comparing with that at 3 days and 8 days after RFA + Abs (P < 0.001) (Figure 3A). Moreover, the change in TNF-α (Figure 3B) was similar to that in IFN-γ for RFA alone group and RFA + Abs group. For TGF-β, the level of TGF-β was on the rise within 8 days after RFA alone, the level of TGF-β at 8 days after RFA alone was 1.23-fold higher than that before RFA (P < 0.001). However, in the RFA + Abs group, the level of TGF-β was declining within 8 days, and the level of TGF-β at 8 days after RFA + Abs was 1.63-fold lower than that before RFA alone (P < 0.001) (Figure 3C). For IL-6, the level of IL-6 was increasing within 8 days after RFA alone, and the level of IL-6 at 8 days after RFA alone was 6.55-fold higher than that before RFA (P < 0.001). When combining with Abs, the level of IL-6 at 8 days after RFA + Abs had no significant difference when compared with that before treatment (P > 0.01), but the level of IL-6 at 3 days after RFA + Abs had a certain increase when compared with that before treatment (P < 0.001) (Figure 3D).
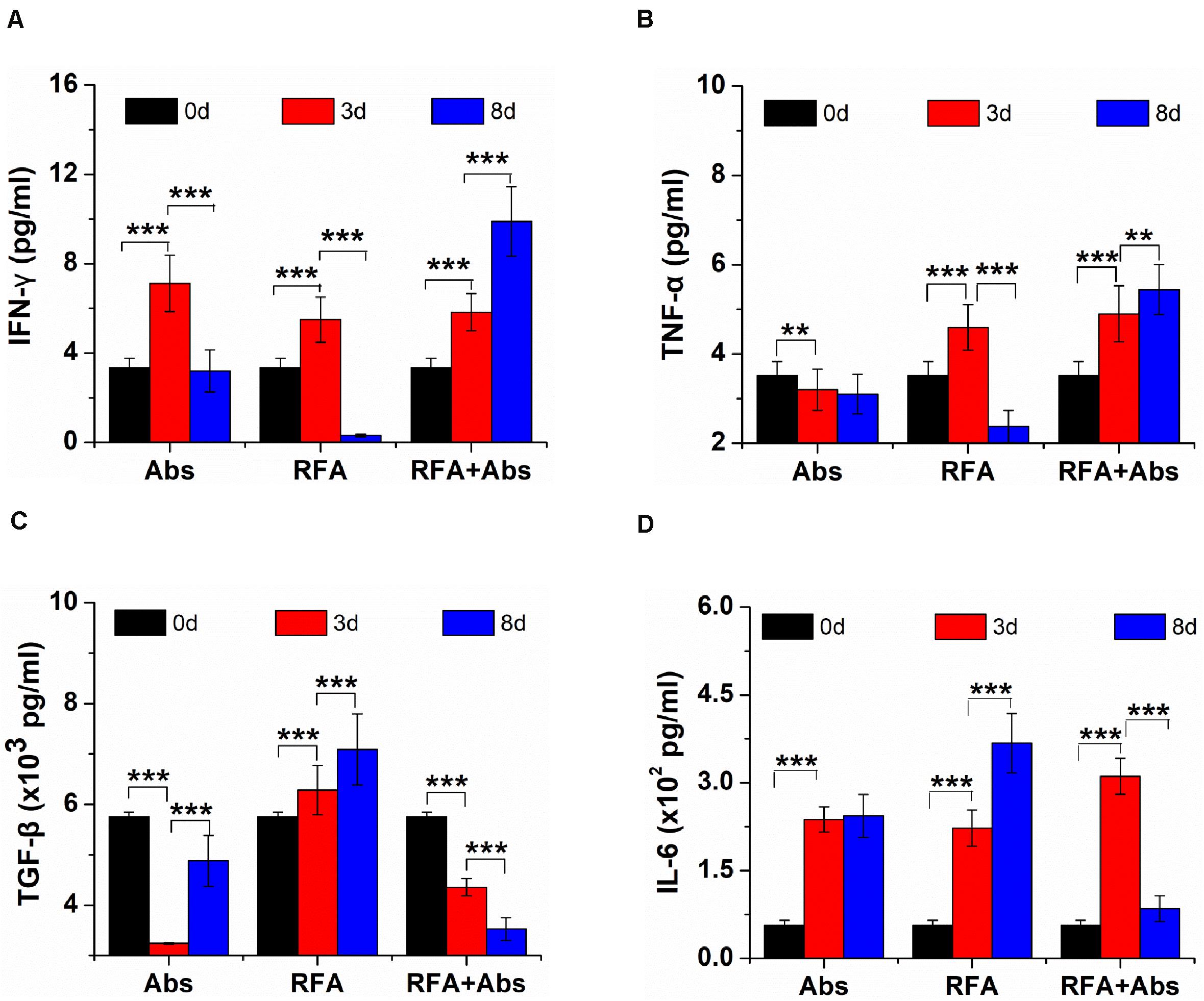
Figure 3. The ELISA assays were used to evaluate systemic immune responses by measuring the level of cytokine in vivo from different treatments (Abs, RFA, and Abs + RFA) at different time points (before RFA, 3 days after RFA, 8 days after RFA). (A) The ELISA assays of IFN-γ from different groups at different time points. (B) The ELISA assays of TNF-α from different groups at different time points. (C) The ELISA assays of TGF-β from different groups at different times. (D) The ELISA assays of IL-6 from different groups at different time points. ***P < 0.001, **P < 0.01.
Then the intratumoral expression level of TGF-β (Supplementary Figures S2A,B) and IL-6 (Supplementary Figures S3A,B) was analyzed by immunohistochemical staining to evaluate systemic immune responses in vivo through different treatment. The results from immunohistochemical staining showed that the expression level of TGF-β and IL-6 after RFA alone had a generally higher result than that in other groups, and the other three groups showed similar expression levels. The quantitative analysis also confirmed that there was a significant difference between the RFA alone group and the other three groups (P < 0.001).
Meanwhile, the results also displayed that both the RFA alone group and the RFA + Abs group had an enhanced percentage of tumor-infiltrating DCs. Further analysis showed that there was no significant difference between RFA + Abs and RFA alone (P > 0.05). Then, the results found that the injection of antibody alone did not increase the percentage of tumor-infiltrating DCs, and there was no significant difference between the Abs alone group and the control group (P > 0.05) (Figures 4A,B).
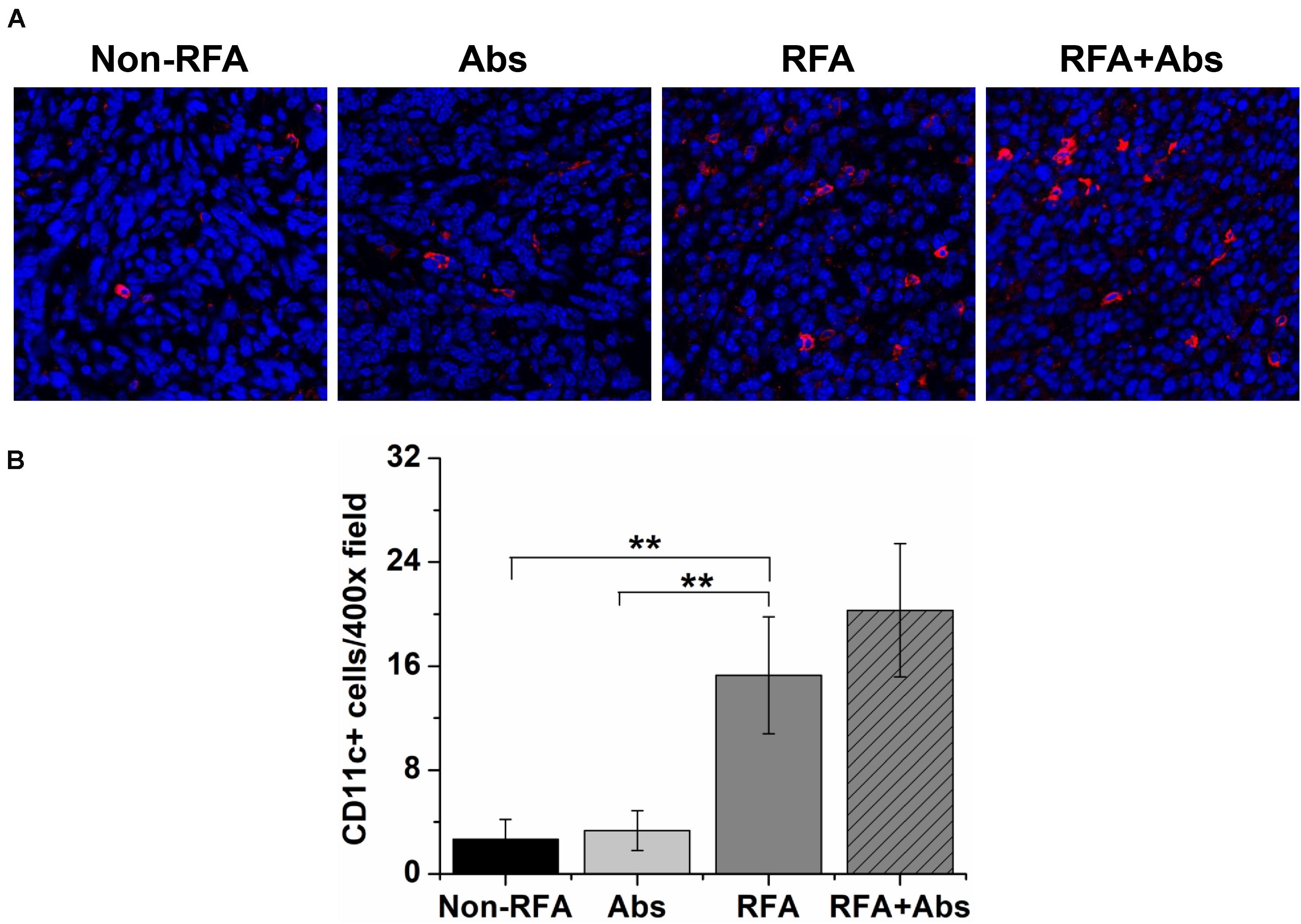
Figure 4. Immunofluorescence staining was performed by using a polyclonal American hamster PE-labeled CD11c antibody to identify tumor-infiltrating dendritic cells (DCs) after different treatment groups (Non-RFA, Abs, RFA, and RFA + Abs). (A) The representative images of immunofluorescence staining for DCs from different treatment groups, the red fluorescence indicates CD11c + cells, and the blue fluorescence indicates a cell nucleus; (B) the quantitative analysis of DCs from different treatment groups, the results displayed that both RFA alone group and RFA + Abs group enhanced the percentage of tumor-infiltrating DCs when compared with Abs alone group and control group (**P < 0.01).
To determine the tumor-infiltrating functional lymphocytes (TILs), double immunofluorescence staining was performed to analyze the percentage of IFN-γ+ CD8+ T cells. The results indicated that the percentage of IFN-γ+ CD8+ T cells was significantly increased in the RFA + Abs group when compared with that in the RFA group (Figures 5A,B).
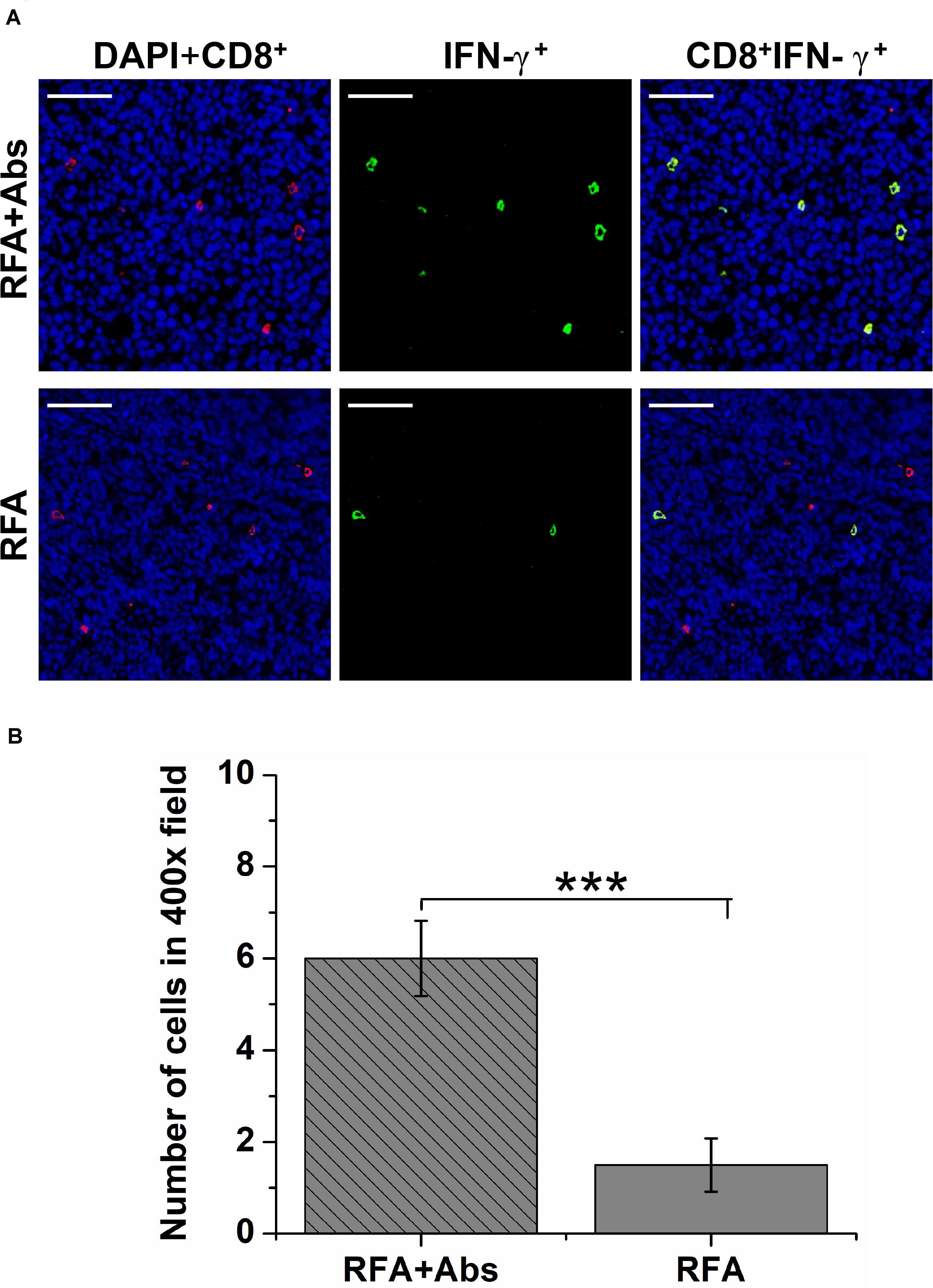
Figure 5. The double immunofluorescence staining was performed to analyze the percentage of IFN-γ+ CD8+ T cells to determine the functional tumor-infiltrating lymphocytes (TILs). (A) The representative images of double immunofluorescence staining for IFN-γ + CD8 + T cells from different treatment groups (RFA and RFA + Abs), the red fluorescence indicates CD8+ cells, and the green fluorescence indicates IFN-γ+ TILs; (B) the quantitative analysis of IFN-γ+ CD8+ T cells from different treatment groups, the results indicated that the percentage of IFN-γ + CD8 + T cells was significantly increased in the RFA + Abs group when compared with that in the RFA group (***P < 0.001).
Long-Term Outcomes
As shown by the tumor growth curves, the tumor growth rate in the RFA + Abs group was lowest when compared to the RFA alone group (P < 0.01) and Abs alone group (P < 0.01). There was no significant difference between the Abs alone group and the control group (P > 0.05) (Figure 6A). After finishing this experiment, the weight of the tumor was measured, and the results showed that the tumor weight in the RFA + Abs group was much lower than that in the RFA group (P < 0.001) (Figure 6B and Supplementary Figures S4A,B).
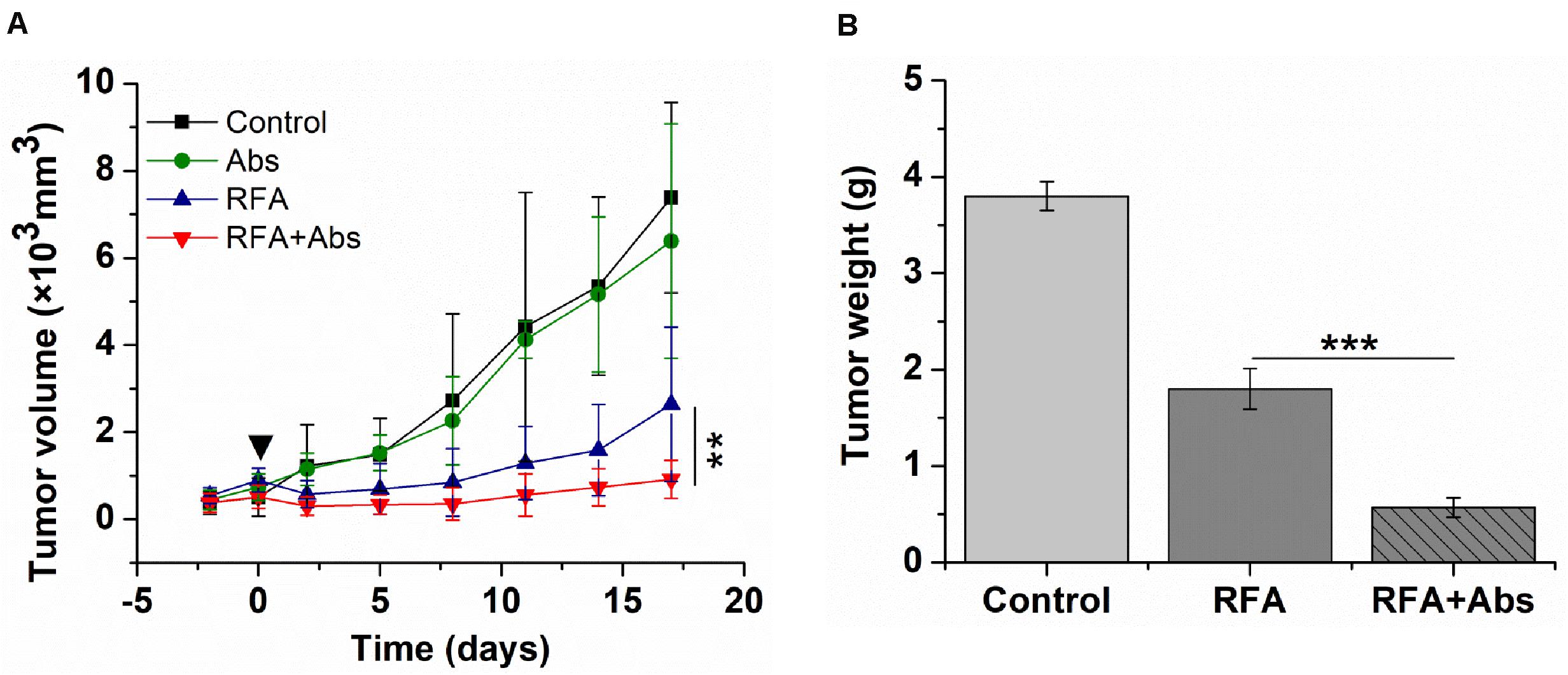
Figure 6. Long-term outcomes after different treatments in the CT26 tumor model. (A) the tumor growth rate in the RFA + Abs group was lowest when compared to RFA alone group (P < 0.01) and Abs alone group, and there was no significant difference between Abs alone group and the control group, (B) the tumor weight in RFA + Abs group was 3.0-fold lower than that in RFA group. ***P < 0.001, **P < 0.01.
Discussion
The results of this study demonstrated that RFA promoted the migration of MDSCs to peripheral blood, and the proportion of MDSCs in peripheral blood at 3 days after RFA was 1.9-fold higher than that before RFA. Moreover, the ratio of CD4/CD8 was declining within 8 days after RFA, indicating the dysfunction of the effector T cells to some extent (16–19). These findings are similar to those of previous studies examining the relationship between tumor-associated antigen (TAA)-specific T cells and the frequency of MDSCs. For example, a report from Shuichi Kaneko et al. indicates that the number of TAA-specific T cells after RFA was inversely correlated with the frequency of CD14+HLA-DR–/low MDSCs in peripheral blood (20). These previous studies were confined to investigating the peripheral immune response rather than the TME. The results from this study go well beyond previous findings, as current data showed that RFA further promoted the infiltration of MDSC in TME. The phenomenon may be due to heat-induced injury, which can lead to the release of a large amount of pro-inflammatory factors.
As the key pro-inflammatory factor, the level of IL-6 was analyzed in our study, and the results showed that the level of IL-6 was increasing within 8 days after RFA alone, when compared with the control group. This data was consistent with the result of cytoplasmic staining that the expression level of IL-6 in RFA alone was higher than that in other groups. Previous studies have shown that several factors, such as IL-6, trigger different signaling pathways in MDSCs that mainly involve the signal transducer and activator of transcription 3 (STAT3). Signal transducer and activator of transcription 3 regulates the expansion of MDSCs by stimulating myelopoiesis and inhibiting myeloid-cell differentiation, and it also promotes MDSCs survival by inducing the expression of Myc, cyclin D1, and B-cell lymphoma XL (BCL-XL), eventually inducing the expansion of MDSCs in TEM (11, 21). Similar studies also demonstrated that IL-6, a downstream mediator of the IL-1β, induced expansion of MDSCs, and IL-1 receptor (IL-1R)-deficient mice have a delayed accumulation of MDSCs and delayed primary and metastatic tumor progression (22–24).
In the present study, the roles of MDSCs in tumor progression after pRFA was further evaluated by the targeted depletion of MDSCs via the Gr-1 (RB6-8C5) antibody. Results showed that the combination of the antibody with pRFA decreased the accumulation of MDSCs in TEM after pRFA. The mechanism for Gr-1 antibody-mediated depletion could include the killing of MDSCs by complement-dependent and -independent cytotoxicity (25). Additionally, previous studies also demonstrated that the Gr-1 antibody induces signals leading to myelopoiesis, macrophage differentiation, neutrophil apoptosis, and abrogation of MDSC activity (26, 27). Meanwhile, the tumor growth was also observed in different treatment groups, and the results found that the combination of the antibody with pRFA delayed tumor progression in this study. This may be associated with reversing immunosuppression via the targeted depletion of MDSCs, which was to some extent confirmed by the ELISA of cytokines in this study. The results from the ELISA assay showed that the level of TGF-β had been increasing within 8 days in the RFA group, and the level of TGF-β at 8 days after RFA alone was 1.23-fold higher than that before RFA. However, in the RFA + Abs group, the level of TGF-β had been declining within 8 days, and the level of TGF-β at 8 days after RFA + Abs was 1.63-fold lower than that before RFA. The cytoplasmic staining of TGF-β also displayed that the expression level of TGF-β in the RFA + Abs group was much weaker than that in the RFA alone group. These findings were consistent with the results from Berzofsky et al. that TGF-β derived mainly from CD11b+Gr-1+ cells (MDSCs) in tumor-bearing mice induced the down-regulation of tumor immunosurveillance and tumor recurrence (28).
Additionally, as the potent antigen-presenting cells, DCs in the TEM was also equally important (29), so tumor-infiltrating DCs before and after RFA or RFA + Abs was analyzed in this study. The results showed that RFA alone increased tumor-infiltrating DCs when compared with the control group, which was consistent with previous findings, however, the tumor progression in the RFA group could not be inhibited. Previous studies have demonstrated that total RFA treatment resulted in enhanced systemic antitumor T-cell immune responses and tumor regression that was associated with increased DC infiltration (7). The difference may be related to the heterogeneity in immune responses, which firstly should be considered due to the difference of tumor mutation burden (TMB) in different tumor models in both experiments (30–32). Additionally, take an example in this study, the boundary of recurrent tumor after pRFA alone was more irregular in 4T1 tumor models than that in CT26 tumor models, which displayed worse biology behavior after pRFA in 4T1 tumor models (data not shown); and the hepatic metastasis was showed after pRFA alone in 4T1 tumor models (Supplementary Figure S5). Moreover, the pre-RFA tumor burden may have certain effects on the prognosis of treatment in these two experiments, and the pre-RFA tumor size in this study (the average diameter was 12 mm) was larger than that in the previous report (the average diameter was 7.8 mm). Accumulating evidence showed that the relationship between the intensity of the immune response and pretreatment tumor burden was vital for achieving effective immunotherapy (33). John Wherry et al. have demonstrated that immunotherapy failure or success in many patients was not solely due to the ability to induce immune reinvigoration, rather it resulted from the balance between T-cell reinvigoration and tumor burden. They also confirmed a calibration of immune responses to antigen burden and raise the possibility that even robust CD8 T-cell reinvigoration by immunotherapy may be clinically ineffective if the tumor burden is high.
In addition, T cell exhaustion as a state of T cell dysfunction that arises during tumor progression, should not be ignored. Past studies have demonstrated that naive T cells are primed by antigen, costimulation, and inflammation, and then differentiate into effector T cells during the initial inflammatory response. However, T cells are accompanied by a progressive increase in the amount and diversity of inhibitory receptors expressed, such as PD-1/PD-L1, LAG-3. T cells could progress through stages of dysfunction or be completely eliminated, leading to the loss of T-cell responses when inflammatory response or antigens persist (34). Concerning the relationship between T-cell responses and RFA treatment, recent reports have showed that RFA induced a marked decrease of the functional TILs in CT26 tumor tissues at 8 days after RFA, which may be associated with the persistent intracellular necrotic debris (as an effective in situ antigen) from thermal ablation (35). In the current study, similar results also showed that the amount of IFN-γ+ CD8+ TILs was at a low level at 8 days after RFA alone, which may be attributed to the persistence of in situ antigens and the elevated extracellular potassium (34, 36). Yet, the amount of IFN-γ+ CD8+ TIL increased significantly after RFA, when combined with the GR-1 antibody, which may be due to the activation of T cell and the inhibition of T cell exhaustion because of the depletion of MDSCs. The results from long-term follow-up also showed that RFA treatment further delayed the tumor progression at different degrees when combined with the depletion of MDSCs in CT26 and 4T1 tumor models. Taken together, tumor-infiltrating MDSCs could be associated with tumor recurrence after RFA and recruiting MDSCs after pRFA could have an influence on the function of DC and induce T cell exhaustion.
While these observations are compelling, it must be acknowledged that there were several limitations to this study. Firstly, although murine CT26 and 4T1 tumor models are the classic tumor model for immunologic studies, only two tumor cell lines were used in this study. Future studies will explore whether similar observations after RFA would occur in other tumor models. Secondly, GR-1 antibodies play an important role in targeted depletion of MDSCs in mice. However, the phenotype of MDSCs is complex in humans. In other words, the depletion of MDSCs by GR-1 antibodies could not be achieved in humans. Therefore, further studies will focus on exploring how to clear MDSCs in humans from different perspectives as a way of achieving better therapeutic efficacy after RFA.
In conclusion, the current study found that pRFA accelerates residual tumor progression through increasing tumor-infiltrating MDSCs and reducing T-cell-mediated anti-tumor immune responses. This study also indicated that the standardization of RFA techniques is essential to achieving complete ablation of a tumor and in preventing tumor progression. When taken together, the findings of this study could provide a potential approach for delaying tumor recurrence caused by pRFA and shows the importance of the standardization of RFA techniques.
Data Availability Statement
All datasets presented in this study are included in the article/Supplementary Material.
Ethics Statement
The animal study was reviewed and approved by the Institutional Animal Care and Use Committee of Peking University Cancer Hospital.
Author Contributions
HW, WY, and HL designed the study, analyzed the data, and wrote the manuscript. HW, SL, A-NJ, MZ, YH, and SW conducted the experiments. HL and WY read and approved the final manuscript. HW and SL were equally responsible for all parts of the manuscript. All authors contributed to the article and approved the submitted version.
Funding
This research was supported by the National Natural Science Foundation of China (Grant Nos. 81773286, 81571674, and 81771853), Nature Science Foundation of Guangdong Province (Grant No. 2018A030313824), Medical Science and Technology Research Foundation of Guangdong Province (Grant No. B2019090), Youth Research Foundation of Guangdong Second Provincial General Hospital (Grant No. YQ2018-004), and Talent Research Foundation of Guangdong Second Provincial General Hospital (Grant No. YN-2018-002).
Conflict of Interest
The authors declare that the research was conducted in the absence of any commercial or financial relationships that could be construed as a potential conflict of interest.
Supplementary Material
The Supplementary Material for this article can be found online at: https://www.frontiersin.org/articles/10.3389/fonc.2020.01308/full#supplementary-material
References
1. Goldberg SN, Charboneau JW, Dodd GD III, Dupuy DE, Gervais DA, Gillams AR, et al. Image-guided tumor ablation: proposal for standardization of terms and reporting criteria. Radiology. (2003) 228:335–45. doi: 10.1148/radiol.2282021787
2. Ahmed M, Brace CL, Lee FT Jr., Goldberg SN. Principles of and advances in percutaneous ablation. Radiology. (2011) 258:351–69. doi: 10.1148/radiol.10081634
3. Chen MS, Li JQ, Zheng Y, Guo RP, Liang HH, Zhang YQ, et al. A prospective randomized trial comparing percutaneous local ablative therapy and partial hepatectomy for small hepatocellular carcinoma. Ann Surg. (2006) 243:321–8. doi: 10.1097/01.sla.0000201480.65519.b8
4. Oshowo A, Gillams A, Harrison E, Lees WR, Taylor I. Comparison of resection and radiofrequency ablation for treatment of solitary colorectal liver metastases. Br J Surg. (2003) 90:1240–3. doi: 10.1002/bjs.4264
5. Wissniowski TT, Hansler J, Neureiter D, Frieser M, Schaber S, Esslinger B, et al. Activation of tumor-specific T lymphocytes by radio-frequency ablation of the VX2 hepatoma in rabbits. Cancer Res. (2003) 63:6496–500.
6. den Brok MH, Sutmuller RP, van der Voort R, Bennink EJ, Figdor CG, Ruers TJ, et al. In situ tumor ablation creates an antigen source for the generation of antitumor immunity. Cancer Res. (2004) 64:4024–9. doi: 10.1158/0008-5472.CAN-03-3949
7. Dromi SA, Walsh MP, Herby S, Traughber B, Xie J, Sharma KV, et al. Radiofrequency ablation induces antigen-presenting cell infiltration and amplification of weak tumor-induced immunity. Radiology. (2009) 251:58–66. doi: 10.1148/radiol.2511072175
8. Hanahan D, Weinberg RA. Hallmarks of cancer: the next generation. Cell. (2011) 144:646–74. doi: 10.1016/j.cell.2011.02.013
9. O’Donnell JS, Teng MWL, Smyth MJ. Cancer immunoediting and resistance to T cell-based immunotherapy. Nat Rev Clin Oncol. (2019) 16:151–67. doi: 10.1038/s41571-018-0142-8
10. Smyth MJ, Ngiow SF, Ribas A, Teng MW. Combination cancer immunotherapies tailored to the tumour microenvironment. Nat Rev Clin Oncol. (2016) 13:143–58. doi: 10.1038/nrclinonc.2015.209
11. Gabrilovich DI, Nagaraj S. Myeloid-derived suppressor cells as regulators of the immune system. Nat Rev Immunol. (2009) 9:162–74. doi: 10.1038/nri2506
12. Marvel D, Gabrilovich DI. Myeloid-derived suppressor cells in the tumor microenvironment: expect the unexpected. J Clin Invest. (2015) 125:3356–64. doi: 10.1172/JCI80005
13. Draghiciu O, Lubbers J, Nijman HW, Daemen T. Myeloid derived suppressor cells-an overview of combat strategies to increase immunotherapy efficacy. Oncoimmunology. (2015) 4:e954829. doi: 10.4161/21624011.2014.954829
14. Horikawa N, Abiko K, Matsumura N, Hamanishi J, Baba T, Yamaguchi K, et al. Expression of vascular endothelial growth factor in ovarian cancer inhibits tumor immunity through the accumulation of myeloid-derived suppressor cells. Clin Cancer Res. (2016) 23:587–99. doi: 10.1158/1078-0432.CCR-16-0387
15. Wang PF, Song SY, Wang TJ, Ji WJ, Li SW, Liu N, et al. Prognostic role of pretreatment circulating MDSCs in patients with solid malignancies: a meta-analysis of 40 studies. Oncoimmunology. (2018) 7:e1494113. doi: 10.1080/2162402X.2018.1494113
16. Yi JS, Du M, Zajac AJ. A vital role for interleukin-21 in the control of a chronic viral infection. Science. (2009) 324:1572–6. doi: 10.1126/science.1175194
17. Frohlich A, Kisielow J, Schmitz I, Freigang S, Shamshiev AT, Weber J, et al. IL-21R on T cells is critical for sustained functionality and control of chronic viral infection. Science. (2009) 324:1576–80. doi: 10.1126/science.1172815
18. Elsaesser H, Sauer K, Brooks DG. IL-21 is required to control chronic viral infection. Science. (2009) 324:1569–72. doi: 10.1126/science.1174182
19. Sheu BC, Hsu SM, Ho HN, Lin RH, Torng PL, Huang SC. Reversed CD4/CD8 ratios of tumor-infiltrating lymphocytes are correlated with the progression of human cervical carcinoma. Cancer. (1999) 86:1537–43. doi: 10.1002/(sici)1097-0142(19991015)86:8<1537::aid-cncr21>3.0.co;2-d
20. Mizukoshi E, Yamashita T, Arai K, Sunagozaka H, Ueda T, Arihara F, et al. Enhancement of tumor-associated antigen-specific T cell responses by radiofrequency ablation of hepatocellular carcinoma. Hepatology. (2013) 57:1448–57. doi: 10.1002/hep.26153
21. Bunt SK, Sinha P, Clements VK, Leips J, Ostrand-Rosenberg S. Inflammation induces myeloid-derived suppressor cells that facilitate tumor progression. J Immunol. (2006) 176:284–90. doi: 10.4049/jimmunol.176.1.284
22. Tu S, Bhagat G, Cui G, Takaishi S, Kurt-Jones EA, Rickman B, et al. Overexpression of interleukin-1beta induces gastric inflammation and cancer and mobilizes myeloid-derived suppressor cells in mice. Cancer Cell. (2008) 14:408–19. doi: 10.1016/j.ccr.2008.10.011
23. Bunt SK, Yang L, Sinha P, Clements VK, Leips J, Ostrand-Rosenberg S. Reduced inflammation in the tumor microenvironment delays the accumulation of myeloid-derived suppressor cells and limits tumor progression. Cancer Res. (2007) 67:10019–26. doi: 10.1158/0008-5472.CAN-07-2354
24. Song X, Krelin Y, Dvorkin T, Bjorkdahl O, Segal S, Dinarello CA, et al. CD11b+/Gr-1+ immature myeloid cells mediate suppression of T cells in mice bearing tumors of IL-1beta-secreting cells. J Immunol. (2005) 175:8200–8. doi: 10.4049/jimmunol.175.12.8200
25. Abbitt KB, Cotter MJ, Ridger VC, Crossman DC, Hellewell PG, Norman KE. Antibody ligation of murine Ly-6G induces neutropenia, blood flow cessation, and death via complement-dependent and independent mechanisms. J Leukoc Biol. (2009) 85:55–63. doi: 10.1189/jlb.0507305
26. Ribechini E, Leenen PJ, Lutz MB. Gr-1 antibody induces STAT signaling, macrophage marker expression and abrogation of myeloid-derived suppressor cell activity in BM cells. Eur J Immunol. (2009) 39:3538–51. doi: 10.1002/eji.200939530
27. Daley JM, Thomay AA, Connolly MD, Reichner JS, Albina JE. Use of Ly6G-specific monoclonal antibody to deplete neutrophils in mice. J Leukoc Biol. (2008) 83:64–70. doi: 10.1189/jlb.0407247
28. Terabe M, Matsui S, Park JM, Mamura M, Noben-Trauth N, Donaldson DD, et al. Transforming growth factor-beta production and myeloid cells are an effector mechanism through which CD1d-restricted T cells block cytotoxic T lymphocyte-mediated tumor immunosurveillance: abrogation prevents tumor recurrence. J Exp Med. (2003) 198:1741–52. doi: 10.1084/jem.20022227
29. Figdor CG, de Vries IJ, Lesterhuis WJ, Melief CJ. Dendritic cell immunotherapy: mapping the way. Nat Med. (2004) 10:475–80. doi: 10.1038/nm1039
30. Swanton C. Intratumor heterogeneity: evolution through space and time. Cancer Res. (2012) 72:4875–82. doi: 10.1158/0008-5472.CAN-12-2217
31. Van Allen EM, Miao D, Schilling B, Shukla SA, Blank C, Zimmer L, et al. Genomic correlates of response to CTLA-4 blockade in metastatic melanoma. Science. (2015) 350:207–11. doi: 10.1126/science.aad0095
32. Rizvi NA, Hellmann MD, Snyder A, Kvistborg P, Makarov V, Havel JJ, et al. Cancer immunology. Mutational landscape determines sensitivity to PD-1 blockade in non-small cell lung cancer. Science. (2015) 348:124–8. doi: 10.1126/science.aaa1348
33. Huang AC, Postow MA, Orlowski RJ, Mick R, Bengsch B, Manne S, et al. T-cell invigoration to tumour burden ratio associated with anti-PD-1 response. Nature. (2017) 545:60–5. doi: 10.1038/nature22079
35. Shi L, Chen L, Wu C, Zhu Y, Xu B, Zheng X, et al. PD-1 blockade boosts radiofrequency ablation-elicited adaptive immune responses against tumor. Clin Cancer Res. (2016) 22:1173–84. doi: 10.1158/1078-0432.CCR-15-1352
Keywords: palliative radiofrequency ablation, myeloid-derived suppressor cells, tumor recurrence, immune response, residual tumor
Citation: Wu H, Li S, Zhou M, Jiang A-N, He Y, Wang S, Yang W and Liu H (2020) Palliative Radiofrequency Ablation Accelerates the Residual Tumor Progression Through Increasing Tumor-Infiltrating MDSCs and Reducing T-Cell-Mediated Anti-Tumor Immune Responses in Animal Model. Front. Oncol. 10:1308. doi: 10.3389/fonc.2020.01308
Received: 17 April 2020; Accepted: 23 June 2020;
Published: 04 September 2020.
Edited by:
Gene A. Cardarelli, Brown University, United StatesReviewed by:
Michael Wayne Epperly, University of Pittsburgh, United StatesJames William Jacobberger, Case Western Reserve University, United States
Copyright © 2020 Wu, Li, Zhou, Jiang, He, Wang, Yang and Liu. This is an open-access article distributed under the terms of the Creative Commons Attribution License (CC BY). The use, distribution or reproduction in other forums is permitted, provided the original author(s) and the copyright owner(s) are credited and that the original publication in this journal is cited, in accordance with accepted academic practice. No use, distribution or reproduction is permitted which does not comply with these terms.
*Correspondence: Wei Yang, MTM2ODE0MDgxODNAMTYzLmNvbQ==; Hongmei Liu, aG9uZ21laWxpdTNAMTYzLmNvbQ==
†These authors have contributed equally to this work