- 1PET-CT Center, National Cancer Center/National Clinical Research Center for Cancer/Cancer Hospital, Chinese Academy of Medical Sciences and Peking Union Medical College, Beijing, China
- 2Department of Orthopedic Surgery, Peking Union Medical College Hospital, Peking Union Medical College and Chinese Academy of Medical Sciences, Beijing, China
- 3Beijing Key Laboratory for Genetic Research of Skeletal Deformity, Beijing, China
- 4Key Laboratory of Big Data for Spinal Deformities, Chinese Academy of Medical Sciences, Beijing, China
- 5Department of Breast Surgical Oncology, National Cancer Center/National Clinical Research Center for Cancer/Cancer Hospital, Chinese Academy of Medical Sciences and Peking Union Medical College, Beijing, China
- 6Department of Radiology, Peking Union Medical College Hospital, Peking Union Medical College and Chinese Academy of Medical Sciences, Beijing, China
- 7Department of Diagnostic and Interventional Imaging, McGovern Medical School, Health and Science Center at Houston, University of Texas, Houston, TX, United States
- 8Department of Medical Imaging, Second Hospital of Hebei Medical University, Hebei, China
- 9Department of Breast Surgery, The Affiliated Yantai Yuhuangding Hospital of Qingdao University, Yantai, China
- 10Department of Central Laboratory, Peking Union Medical College Hospital, Peking Union Medical College and Chinese Academy of Medical Sciences, Beijing, China
Breast cancer is a major disease with high morbidity and mortality in women worldwide. Increased use of imaging biomarkers has been shown to add more information with clinical utility in the detection and evaluation of breast cancer. To date, numerous studies related to PET-based imaging in breast cancer have been published. Here, we review available studies on the clinical utility of different PET-based molecular imaging methods in breast cancer diagnosis, staging, distant-metastasis detection, therapeutic and prognostic prediction, and evaluation of therapeutic responses. For primary breast cancer, PET/MRI performed similarly to MRI but better than PET/CT. PET/CT and PET/MRI both have higher sensitivity than MRI in the detection of axillary and extra-axillary nodal metastases. For distant metastases, PET/CT has better performance in the detection of lung metastasis, while PET/MRI performs better in the liver and bone. Additionally, PET/CT is superior in terms of monitoring local recurrence. The progress in novel radiotracers and PET radiomics presents opportunities to reclassify tumors by combining their fine anatomical features with molecular characteristics and develop a beneficial pathway from bench to bedside to predict the treatment response and prognosis of breast cancer. However, further investigation is still needed before application of these modalities in clinical practice. In conclusion, PET-based imaging is not suitable for early-stage breast cancer, but it adds value in identifying regional nodal disease and distant metastases as an adjuvant to standard diagnostic imaging. Recent advances in imaging techniques would further widen the comprehensive and convergent applications of PET approaches in the clinical management of breast cancer.
Introduction
Breast cancer is the most common cancer and has the second highest cancer-related morbidity in women worldwide (1). Early diagnosis followed by timely treatment can significantly improve the survival of breast cancer patients. Modern clinical guidelines and standard treatments for breast cancer are based on accurate pathological diagnosis, clinical staging, and molecular subtyping (2). Mammography, ultrasound, and breast magnetic resonance imaging (MRI) are the primary methods of evaluating the local disease extent and stage. Computed tomography (CT) of the chest and abdomen, bone scans, and MRI are used to screen and stage distant metastases. However, the efficacy of clinical methods, including serum antigen protein markers and radiological imaging, is insufficient for breast cancer diagnosis and staging (3, 4). As a useful tool for the in vivo assaying of the metabolic characteristics of breast cancer (5, 6), positron-emission tomography (PET) using radioactive tracer 18F-fluorodeoxyglucose (18F-FDG) has been introduced as an additional imaging modality for these patients, facilitating breast cancer staging, distant-metastasis detection, prognostic prediction, and especially evaluation of the pathological response to treatment (7, 8). Combining PET with traditional radiological methodologies, including CT, MRI, and mammography, has shown a range of clinical utility for appropriate breast cancer patients. Several clinical trials and technical advances were achieved between 1988 and 2018 in testing the clinical utility of PET-based techniques in breast cancer (Figure 1). The application of PET in breast cancer has increased dramatically over the past years, benefiting from the development of commercial equipment and breast-specific imaging approaches.
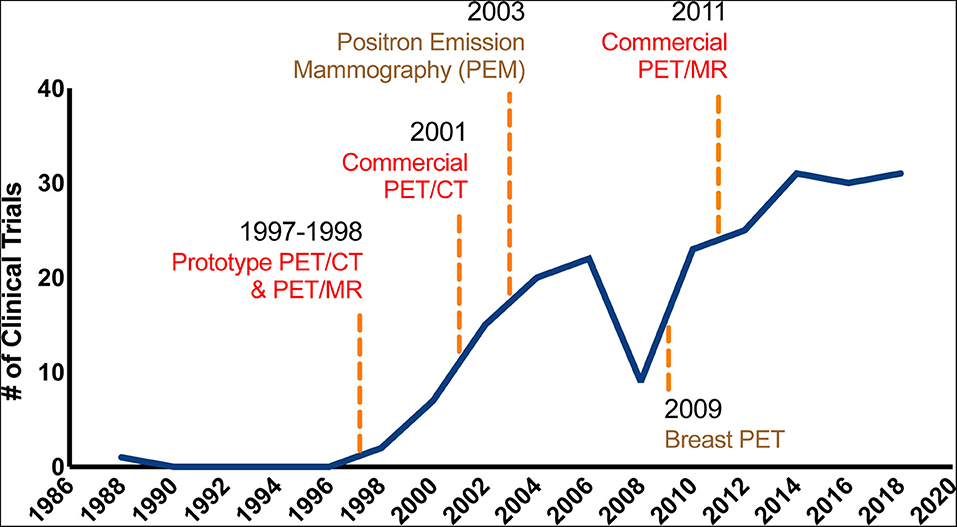
Figure 1. The developing utility of PET-based techniques in breast cancer. The graph represents a timeline of important technological developments in PET-based techniques. The solid line represents the change in the number of PubMed clinical trial publications for PET and breast cancer per year between 1988 and 2018. The earliest models of PET/MR and PET/CT date back to 1987 and 1988, respectively. As shown in the figure, the use of PET in breast cancer has increased dramatically over the past years, benefiting from the development of commercial equipment and breast-specific imaging approaches.
In this review, we first revealed the biological basis of the fluorodeoxyglucose PET imaging in illustrating breast cancer. Then, multiple PET-based imaging techniques, including the breast-specific PET imaging, PET/CT, and PET/MRI, and their clinical applications were described in detail. The clinical applications started with the role in diagnosis and staging, including primary tumor, lymph node, and distant metastases, then local recurrence and therapeutic response evaluation. Furthermore, the clinical applicability of using PET-based imaging in cancer screening in asymptomatic women who are at high risk for breast cancer was also discussed. At last, recent advances in novel PET tracers and PET-based radiomics were demonstrated with the potential prospect in the future.
Fluorodeoxyglucose PET and Tumor Biology
The increased 18F-FDG avidity of malignant cells forms the basis of breast cancer imaging with 18F-FDG-PET (7, 9). However, the uptake of 18F-FDG is also affected by the density of breast tissue. Dense breast tissue highly uptakes 18F-FDG but at amounts still lower than in breast cancer (10, 11). The intensity and pattern of 18F-FDG uptake in breast lesions are directly related to the biological characteristics of the tumor, such as pathological type, aggressiveness, and molecular subtype. Invasive ductal carcinomas (IDCs) exhibit higher avidity for 18F-FDG than noninvasive ductal carcinomas (5, 8, 12, 13), and triple-negative breast cancers display greater 18F-FDG uptake levels than other molecular subtypes (14). In some breast cancer subtypes (luminal A), FDG uptake is physiologically influenced by both the features of the tumor and hormonal fluctuations during the menstrual cycle (15). In addition to the FDG uptake value, advanced imaging features such as histogram of FDG-PET can be used to separate molecular and histological subtypes (16, 17). It has also been reported that FDG-PET radiomics are associated with various tumor aspects (namely, Ki67, pCR after NAC and risk of recurrence) and may potentially become unique pretreatment biomarkers of diagnosis and prognosis (18).
The breast tumor detection rate via 18F-FDG-PET is determined by the tumor size and pathology grade. Although the highest sensitivity of breast cancer detection using 18F-FDG-PET is reported as 96% among all tumor sizes, the sensitivity for small tumors (<1 cm) is reported to be below 60% (12). Because of its low sensitivity, 18F-FDG-PET is not routinely used for early-stage breast cancer patients, but it serves as a powerful complement to other imaging methods for accurate clinical staging in patients with stage III and IV disease. It has been reported that the application of 18F-FDG-PET would cause changes in the planned treatment in one-third of patients with advanced breast cancer by identifying distant-metastasis sites missed by conventional imaging methods (19).
PET-Based Imaging Techniques
Breast-Specific PET Imaging
Since whole-body PET imaging is suboptimal for the detection of breast lesions, it is recommended that a prone position be used to assist in breast-specific PET imaging scans (20). Thus, dedicated PET scanning modalities for the breast, including positron emission mammography and breast PET, have been developed to further improve the spatial resolution (21–23). The PEM system comprises two planar or curved detectors that scan the breasts with mild compression. MacDonald et al. (22) showed that the reconstructed spatial resolution in the PEM system can reach 2.4 mm. The limitation of PEM lies in its acquisition geometry, which may affect the clear imaging of lesions at the edge of the camera (for example, in the chest wall) and introduce structural superimposition (22). The PEM can help reclassify suspicious calcifications detected on standard mammography with largely improved accuracy (95%) (24). Additionally, a PEM-CT system has been introduced to further improve the detection power of the PEM system. The PEM-CT system is able to scan the breast in an uncompressed manner and image suspected lesions in 3 dimensions (25). The other existing prototype, the breast PET system, which has been developed relatively recently (Figure 1), acquires high-resolution breast imaging using multiple rotating planar detectors. We deem that the breast PET approach might help overcome the limitations of PEM.
PET/CT
In most clinical applications, PET is combined with a CT system (PET/CT) to replace PET alone for providing anatomical information useful for accurately interpreting PET signals. The earliest models of PET/CT and PET/MR systems date back to 1987 (Figure 1). Similar to PET, PET/CT is not routinely performed without a previous diagnosis because of its lack of sensitivity, which is considerably low for lobular carcinomas (26) and tumors <1 cm in length (27). According to the guidelines of the National Comprehensive Cancer Network, PET/CT should not be applied for early-stage breast cancer in the absence of symptoms and is not indicated in the staging of clinical stage I or II or operable stage III breast cancer. However, PET/CT is recommended alongside diagnostic CT for identifying unsuspected regional nodal disease and/or distant metastases and in situations where standard staging studies are equivocal or suspicious, especially in the setting of locally advanced or metastatic disease (28). The clinical application of the PET/CT system is described in detail later in the manuscript.
PET/MRI
Dynamic contrast-enhanced magnetic resonance imaging (DCE-MRI) is both a morphological and functional imaging tool and is the most sensitive technique to date for breast cancer screening (29). DCE-MRI is frequently used in breast cancer staging and treatment monitoring (30). Integrated PET/MRI, which can acquire both metabolic data and high-contrast morphological images in a single exam, has recently been used more often in breast cancer patients. PET/MRI has been suggested as a means to improve diagnostic accuracy and decrease false-positive rates over those obtained with MR (31, 32). It has also demonstrated increased axillary nodal metastasis sensitivity (33, 34). Bitencourt et al. (35) reported that 100% sensitivity and 55% specificity could be achieved in breast cancer diagnosis using multiparametric PET/MRI evaluation (including DCE, diffusion-weighted imaging [DWI], and 3D 1H-MRSI). Pinker et al. (36) also reported that in their study of 78 indeterminate or suspicious lesions, half of the unnecessary biopsies would be performed with PET/MRI compared with conducting MRI alone. Furthermore, Jena et al. (37) indicated that by adding acquisition time into the pharmacokinetic parameters of PET/MRI, an accuracy of 94.50% can be achieved. The pharmacokinetic DCE-MRI parameters can be further correlated with metastatic status, Ki67 expression (38), and prognosis (39, 40). Catalano et al. demonstrated that 18F-FDG PET/MRI could distinguish between molecular subtypes in 13/21 breast cancer patients (41).
Clinical Application of PET-Based Imaging Techniques
Imaging the Primary Breast Cancer
Breast cancer detection, including assessment of tumor size and multifocality, is mainly based on mammography, ultrasound, and MRI. Several studies have reported that FDG-PET displays low sensitivity and relatively high specificity and accuracy in the detection of primary breast cancer (7, 12, 42, 43). Figure 2 shows multiple images of PET/CT, MRI, and mammography in a female patient with right breast cancer. Compared with MRI, PET/CT has been shown to be less sensitive but more specific in breast cancer detection (44). Jung et al. (26) compared the accuracy of PET/CT and MRI among 105 patients with biopsy-proven breast cancers, illustrating that MRI identified all 105 (100%) primary tumors, whereas PET/CT correctly detected 85 (81.0%) primary tumors. In the detection of multifocal breast cancers, Ergul et al. (45) reported that the sensitivity of PET/CT and MRI was 67 vs. 78%, while the specificity was 100 vs. 53%, respectively.
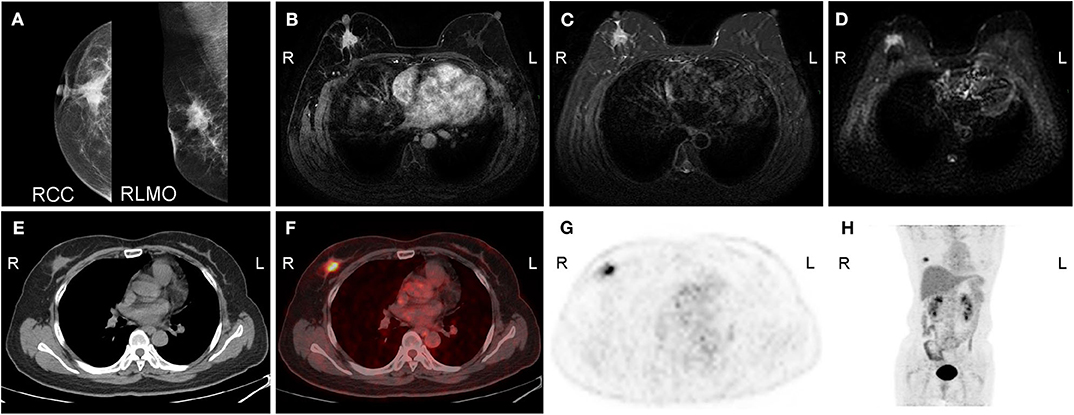
Figure 2. A 50-year-old woman with carcinoma of the right breast. (A) Molybdenum target mammography shows a mass in the upper quadrant of the right breast with an irregular shape, fuzzy boundary, speculation, linear calcification, and a retracted nipple. (B) Enhanced MRI shows that the right breast mass was significantly enhanced during the arterial phase, with a high signal on (C) T2WI/FS and (D) DWI. Axial (E) CT, (F) fused image, (G) FDG-PET, and (H) MIP (maximal intensity projection) image show irregular nodules with intense uptake on the upper outer quadrant of the right breast. R, right; L, left; RCC, right craniocaudal; RMLO, right mediolateral oblique.
In addition, PET/MRI performs better in primary breast cancer detection than PET/CT does, but compared with the detection with MRI, the improvement is limited. In a study comparing MRI, PET/CT, and PET/MRI in 50 breast cancer patients, PET/MRI and MRI showed higher accuracy in identifying the tumor size than PET/CT [41/50 (82%) by using PET/MRI, 41/50 (82%) by using MRI, and 34/50 (68%) by using PET/CT; P < 0.05] (34). Additionally, PET/MRI and MRI also showed higher accuracy in identifying multifocal or multicentric breast cancer than PET/CT [8/9 (89%) by using both PET/MRI and MRI vs. 5/9 (56%) by using PET/CT; P < 0.05] (34). Thus, FDG-PET is not recommended in breast cancer screening and is utilized in primary tumor detection only when standard procedures deliver a suspicious result.
Imaging the Lymph-Node Metastases
Although sentinel lymph node (SLN) mapping and biopsy are routinely applied to evaluate lymph-node metastases of breast cancer, imaging techniques are utilized as additional modalities in nodal assessment. Most studies have indicated that PET-based imaging is more sensitive in detecting axillary metastases than MRI (30). Figure 3 shows an example of the imaging lymph-node metastasis in a 66-year-old female patient with right breast cancer by PET/CT and MRI. Ergul et al. (45) reported that the sensitivity of PET/CT in detecting axillary metastasis was 67%, while that obtained from MRI was 47% (N = 15). The specificity of PET/CT for detecting axillary nodal involvement was also higher than that of MRI (89% by PET/CT vs. 78% by MRI, respectively). In a study of 128 patients with IDC, the c-statistic of predictive axillary lymph node (ALN) metastasis achieved a score of 0.791 when using a PET/CT-based model (46). It is worth noting that the CT portion of PET/CT appears to be critical for the sensitivity of axillary state detection. It has been reported that the sensitivity of PET alone for axillary metastases is 60%, while that of MRI alone is 93.3%, and the specificity for axillary metastases is 91% for both PET alone and MRI alone (47). In a study of 49 breast cancer patients (18 with axillary metastases), the sensitivity for axillary lymph-node status was 78% for PET/CT, 78% for PET/MR, and 67% for MRI, while PET/CT also demonstrate a slightly superior specificity of 94% for axillary metastases, compared with 90% from PET/MRI and 87% from MRI (34). In a feasibility study, van Nijnatten et al. (48) reported a dedicated axillary FDG PET/MRI that improved sensitivity to 100% (N = 12) in axillary nodal staging for clinically positive patients. Thus, compared with MRI, PET/CT and PET/MRI both show largely advanced accuracy in ALN detection.
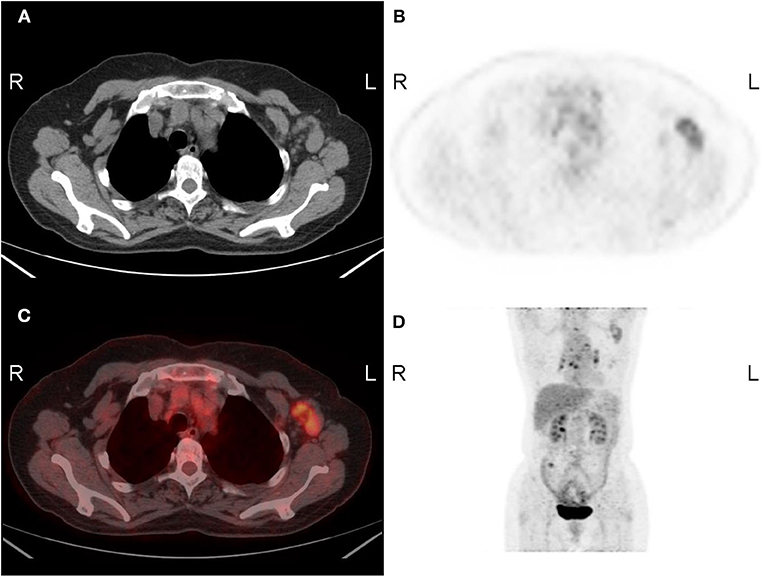
Figure 3. A 66-year-old woman with lymph-node metastasis of the left breast. Axial (A) CT, (B) FDG-PET, (C) fused image, and (D) MIP image show enlarged lymph nodes with intense uptake in the left axilla. The SUVmax was 3.4. R, right; L, left.
Generally, 18F-FDG PET/CT is not recommended for axillary staging, especially in patients with small tumors, as PET/CT entails the delivery of a relatively high radiation dose (49). 18F-FDG-PET displays moderate sensitivity for axillary metastases that is insufficient for eliminating the necessity for sentinel lymph-node biopsy (50). The high positive predictive value of PET-based techniques allows surgeons to submit axillary node-positive patients directly to axillary lymph-node dissection (ALND) (51). In a study conducted in the Netherlands, 159 patients underwent combined FDG-PET/CT and MARI (marking axillary lymph nodes with radioactive iodine seeds) procedures for axillary staging. According to the results, most of the patients received direct locoregional radiotherapy without ALND. Subsequently, the authors reported an 82% reduction of ALND for node-positive breast cancer patients (52).
On the other hand, FDG-PET is helpful in identifying unsuspected lymph nodes in addition to ALNs that cannot be effectively assessed during SLN biopsy. Studies have consistently shown that 18F-FDG-PET is superior to CT in detecting internal mammary (IM) and mediastinal lymph-node metastases (53). A study of 58 breast cancer patients compared the performance of PET/MRI and MRI alone in the detection of axillary, internal mammary, and supraclavicular lymph nodes. For IM and supraclavicular lymph-node detection, the difference between PET/MRI and MRI was not statistically significant (31). Jochelson et al. (54) also found a similar detection rate of IM adenopathy by MRI and PET/CT (14/90 [16%] for MRI, 13/90 [14%] for PET/CT, P = 0.317). In general, PET/CT, MRI, and PET/MRI hitherto appear equivalent in the detection lymph-node metastases located in less common sites.
In brief, emerging studies have reported the fine detection power of PET-based techniques in lymph-node staging, but improvement and further studies are still necessary with regard to clinical utility. We deem that PET/MRI might become favored, especially in identifying unsuspected lymph nodes, due to its low radiation dose.
Imaging Distant Metastases
Although PET-based imaging is not a standard procedure in staging early-stage breast cancer, it plays an important role in systemic staging. Groheux et al. (55) showed that PET/CT detected unsuspected metastases in up to 47.1% of patients with untreated stage III breast cancer. The upstaging rates reported for each stage were similar among subtypes of estrogen receptor [ER]+/HER2– and HER2+ breast cancer (56). The bone, liver, and lung are the most common sites of the distant metastasis of breast cancer (57). Generally, PET/MRI exhibits higher sensitivity than PET/CT in detecting metastatic lesions, especially in the liver and bones, but it is unable to detect lung metastasis (58–60). Moreover, PET/CT is thought to have low sensitivity for brain (61, 62) and liver (63) metastases.
Compared with whole-body CT and bone scintigraphy, 18F-FDG-PET can detect early bone marrow metastases before the signs of bone abnormalities appear on CT or bone scintigraphy (64). CT and bone scintigraphy cannot separate active or therapy-burnout lesions, so the prognosis from these techniques can be ambiguous. For example, bone metastases initially presenting as osteolytic lesions on CT could appear as osteosclerosis as a result of the repair process and become morphologically abnormal after successful chemotherapy or the introduction of bisphosphonates. On the other hand, increased 18F-FDG uptake indicates active bone metastasis regardless of the morphological appearance of the bone and can be accurately detected with PET-based imaging (65, 66). Furthermore, PET/CT can be helpful in predicting subsequent pathological fracture in metastatic breast cancer patients (67). Using F-FDG/F-NaF cocktail tracers, Roop et al. (68) reported significant advancement in bone metastasis detection by PET/CT. Despite the advantages of PET/CT in bone metastasis detection, PET/MRI demonstrated an improved performance (69). Catalano et al. (70) reported that PET/MRI could identify significantly more bone metastases than PET/CT in breast cancers (N = 25, P < 0.001).
In the liver, PET/MRI imaging depicted increased sensitivity compared with PET/CT (80–100% by PET/MRI vs. 70–75% by PET/CT, P < 0.001), which might be due to the application of DWI. Regarding pulmonary metastases, in contrast to bone and liver metastasis, PET/CT was reported to be slightly superior to PET/MRI in a study of 242 distant-metastatic lesions (59). Nevertheless, most of the lung lesions omitted by PET/MRI were small (<1 cm) or determined on follow-up, and thus, the clinical importance is unclear (71).
Imaging Local Recurrence
PET-based imaging is of great use in the detection of tumor recurrence and unexpected remote metastasis in patients with local recurrence (72). In a study of 23 female patients with suspected breast cancer recurrence undergoing both PET/CT and whole-body MRI, PET/CT demonstrated higher specificity and sensitivity in the assessment of nodal and distant lesions than MR/DWI but lower sensitivity in the detection of local breast lesions (73). The overall sensitivity, specificity, and accuracy of PET/CT were 84.8, 86.3, and 85.4%, respectively, vs. 82.1, 78.0, and 80.5% for MRI (73). Additionally, PET/CT appeared to show an advantage over CT and PET alone in the diagnosis of breast cancer recurrence (74). Impressively, dual time-point PET/CT yielded an area under the ROC curve of 0.99 for recurrence detection (n = 100) (75). Imaging features of lymph nodes, rather than those of primary tumors, were shown to be even better predictors of recurrence (76). When combined with percutaneous FDG-avid target biopsies, PET/CT achieved a sensitivity of 99% for local recurrence surveillance (77). Compared with standard practice, PET/CT may offer improved diagnostic accuracy in breast cancer recurrence. It is also recommended to perform FDG-PET/CT at the same time as diagnostic CT in the prediction of breast cancer recurrence.
Whole-body PET/MRI is comparatively unfavored for detecting local recurrence. In a study of 94 primary breast cancer patients, all primary breast tumors were identified by prone breast/MRI, while whole-body PET-MRI missed primary breast cancers in 7/94 (7.4%) patients (78). Regarding small tumors, Kong et al. (27) demonstrated that only 4/10 (40%) breast cancer tumors <1 cm in length were seen on whole-body PET/MRI. Additionally, the contrast-enhanced MRI at 3T was demonstrated more sensitive in detecting small lesions (<10 mm) (79). Thus, PET/MRI is considerably sensitive to lesions in other parts of the body, and breast MRI or prone breast PET/MRI might be superior to supine whole-body PET/MRI in detecting local recurrences.
Imaging the Therapeutic Response
Predicting the therapeutic response to chemotherapy in breast cancer is another important application of PET-based techniques. High-dimensional features in PET, CT, and MR images can be extracted with radiomics (80). Serial PET imaging after treatment is helpful for therapy response monitoring (81, 82). Changes in 18F-FDG uptake induced by different therapies might be valuable signs in the separation of responsive and non-responsive patients in an early phase (83). The therapeutic response is also deemed a powerful prognostic stratification indicator (for both disease-free survival and overall survival) in either locally advanced breast cancer or metastatic breast cancer (84, 85).
NAC has been proven to be substantially effective in primary tumor pre-surgery downstaging and is now increasingly utilized in stage II and III breast cancer (86). Abundant studies have confirmed the efficacy of PET/CT in the early assessment of the response to NAC. However, this method of assessment has not been introduced in routine clinical practice. In a study of 23 patients with locally advanced breast cancer, Kumar et al. (87) reviewed that the sensitivity, specificity, and accuracy of PET/CT in response detection were 93, 75, and 87%, respectively. Tian et al. (88) conducted a meta-analysis encompassing a total of 22 studies including 1,119 patients, and the pooled data showed that the sensitivity and specificity were 81.9 and 79.3%, respectively, for PET/CT in predicting the pathological response to NAC in breast cancer patients. In another meta-analysis of 19 studies involving 920 pathologically confirmed patients (89), the pooled sensitivity and specificity of PET in the prediction of the histopathological response in primary breast lesions were 84 and 66%, respectively. Additionally, in regional lymph nodes, the sensitivity of PET was 92% (89). Based on PET/CT parameters, the concept of complete metabolic response (CMR) has been introduced as a promising outcome predictor during the mid-course of NACs (90).
The treatment response in metastatic breast cancer could also be predicted by a quantitative assay based on changes in tracer uptake throughout the treatment. Groheux et al. reported that changes in 18F-FDG uptake (defined as ΔSUVmax) are highly associated with the pathological complete response (pCR) after NAC (P = 0.0001) (91). Reported by two studies, a high SUV decrease after the first cycle of chemotherapy was able to predict the clinical response at the end of the treatment (92, 93). Couturier et al. (94) also reported that the changes in SUVmax after three cycles of chemotherapy could predict not only the clinical response but also the overall survival.
In a comparison of the performance of PET and MR imaging in predicting the pathological complete response (pCR), PET appears more sensitive, and MR imaging is more specific (88). Integrated PET/MRI metric data and clinical features can help improve accuracy. PET/MRI imaging characteristics can include changes in SUVmax, total lesion glycolysis (TLG), signal enhancement ratio (SER), and peak enhancement ratio (PER) in pilot studies. Clinical data, namely, patient age, and breast cancer subtype, have also been taken into consideration (95–97). Additionally, MRI-derived anatomical data such as DWI and DCE sequences can also improve the specificity and negative predictive value of PET in response prediction (98).
The diagnostic performance and favorable factors of PET/CT and PET/MRI in breast cancer are compared in Table 1. For primary breast cancer, PET/MRI performed similarly to MRI but better than PET/CT. PET/CT and PET/MRI have higher sensitivity than MRI in the detection of axillary lymph-node metastases. However, in the detection of sentinel lymph nodes (SLNs), the sensitivity of the PET-based imaging methods was lower. PET-based imaging has shown its value in detecting unsuspected extra-axillary nodal metastases. For distant metastasis, PET/CT performs better in the detection of lung metastasis, while PET/MRI performs better in the liver and bone. Additionally, PET/CT is superior in terms of monitoring local recurrence. However, only a few cancer centers are equipped with a PET/MRI device, which severely limits the clinical application of the technique.
Recent Advances in PET-Based Strategies
Special Populations
Despite the medical concern for women who are at high risk for breast cancer, PET-based techniques have scarcely been used in cancer screening in this population due to the lack of solid evidence (99). Although genetic high-risk features can also be investigated using PET imaging as a result of the various changes in normal tissues (8), it is relatively difficult to assess the true utility of the technique. BRCA1 mutation carriers have a higher risk of developing triple-negative breast cancer (100), which might appear with significantly increased 18F-FDG avidity (14). Therefore, compared to standard screening techniques, PET breast cancer screening might show improved sensitivity and a better chance of clinical utility in patients with BRCA1 mutations, but further investigations are needed.
Novel Radiotracers
Various new radiotracers are being developed and applied for the in vivo measurement of different aspects of breast cancer, such as proliferation, metastasis, hypoxia, receptor status, tumor antigen levels, and therapeutic response. Herein, we summarized some of the newly developed radiotracers (Table 2). As a cellular proliferation marker, 18F-fluorothymidine (18F-FLT) allows the in vivo investigation and quantification of tumor growth and metastases and is also utilized in identifying the treatment response (101, 102). 18F-Fluoromisonidazole (FMISO), which is a hypoxia biomarker, has also been used in breast cancer imaging since the degree of hypoxia in a tumor is associated with its biological features, such as aggressiveness and therapeutic resistance (6). Integrin αvβ3 overexpression appears in endothelial cells activated by tumor-induced angiogenesis and has been reported to be a marker of local advancement and metastasis of breast cancer (6, 125, 126). The 18F-galacto-arginine-glycine-aspartic acid tripeptide, which can bind to integrin αvβ3, has been used as a PET tracer to measure αvβ3 expression in both primary tumors and metastatic lesions (125). 68Ga-PSMA, which is a tumor-specific antigen that was first used in prostate cancer staging, has been repeatedly utilized and is rapidly becoming a complementary tracer for breast cancers with low FDG avidities. High PSMA uptake has been exhibited in invasive ductal carcinoma, triple-negative breast cancer, and rare pathological subtypes such as Signet-ring cell breast adenocarcinoma (110–112, 127). Like 18F-FDG, tracers based on cellular metabolism are continuously being studied. 18F-Fluciclovine, which is a leucine analog, has been shown to be strongly correlated with the tumor response after NAC therapy (106). 18F-Choline PET/CT has been reported to be efficacious in leptomeningeal metastasis detection (113).
Radiotracers targeting hormone receptors and HER2 are also being investigated and may eventually help in the development of new drugs and in the evaluation of their efficacy (128–132). 18F-16α-Fluoroestradiol (FES) is a substrate of estrogen receptors. It has been used as a tracer for specific receptors in breast cancer and has been proven to be significantly associated with ER expression (133). Studies have indicated that FES avidity can be a pharmacodynamic biomarker for ER-directed therapy (121, 122). Another novel tracer, 68Ga-NOTA-RM26, was found to be correlated with both ER expression and menstrual status and to improve the sensitivity and specificity of breast cancer diagnosis to 100 and 90.9%, respectively, in proliferating phase patients (103). HER2 expression status plays an important role in prognosis and chemotherapy in breast cancer; trastuzumab and pertuzumab are two HER2 antagonists currently in use. In clinical practice, HER2 status is determined by immunohistochemical or fluorescence in situ hybridization testing of biopsy samples. Nevertheless, the test results might be affected by tumor heterogeneity and expression discordance between the primary tumor and metastasis sites (134). Therefore, new PET tracers such as 89Zr-trastuzumab and 89Zr-pertuzumab were developed for measuring the HER2 expression of the primary tumor and metastases simultaneously in a non-invasive manner and have shown promising results in clinical studies; nevertheless, false-positive foci still remain a challenge to their effective utility (116, 135, 136). In addition, 68Ga-labeled affibody molecules were shown to accurately measure HER2 expression in a phase I/II clinical trial of advanced breast cancers (137).
PET Radiomics
Radiomics has the potential to uncover disease characteristics by extracting numerous parameters/features from tomographic images within a region of interest using mathematical algorithms (80). Non-invasive image-derived biomarkers can also be generated from PET radiomics according to the intensity of the pixels, their associated parameters, and their positions (138). Based on the clinical application of PET-based techniques, several studies have focused on the clinical and technical feasibility of applying PET radiomics to diagnosis (139, 140), staging (141–143), pathological characterization (17, 18), NAC response (18, 144–146), and outcome prediction (147, 148) in breast cancer. However, the difficulty in detecting the edges of breast lesions using CT limits the PET/CT in identifying the breast tumor and the alignment of the imaging modalities (141). As MRI has shown high sensitivity in breast cancer detection, combining PET- and MRI-derived radiomics features would further widen the spectrum of features and enable the building of more powerful predictive models (138, 142). However, most radiomics-based predictive models or classifiers have been found to be of insufficient quality because of the lack of complete information for model development (149), and they should be further validated with independent cohorts from external sources before application in clinical practice.
Summary
To date, the development and clinical application of PET/CT and PET/MRI have shown their individual usefulness in breast cancer. This progress also presents opportunities to reclassify tumors by combining their fine anatomical features with metabolic characteristics and develop a beneficial pathway from bench to bedside to better understand the biological and pathophysiological bases of breast cancer. According to current clinical guidelines, PET-based imaging is not suitable for early-stage breast cancer in the absence of symptoms and is not indicated in the staging of clinical stage I or II or operable stage III breast cancer. However, PET/CT and PET/MRI add value in identifying regional nodal disease and distant metastases as an adjuvant to standard diagnostic imaging techniques. Nevertheless, further investigations are still necessary to optimize the detection power, efficiency, and predictability of PET/CT and PET/MRI. Although some studies have investigated the utility of PET-based strategies in high-risk women, current evidence does not support the use of PET as a screening technology. Moreover, novel radiotracers based on tumor biological behavior, hormone receptors, and HER2 status have emerged and will hopefully promote the precise detection of breast cancer. Recent advances in PET radiomics could further widen the spectrum of features, especially when combined with MRI-derived radiomics. Similar to other radiomics-based predictive models, independent validation of PET-based radiomics models in multiple centers are still needed before application in clinical practice. We conclude that comprehensive and convergent applications of PET approaches will become a future trend in the clinical management of breast cancer. With the expansion of tumor information provided by advanced examination techniques, the treatment of breast cancer can further develop via greater individualization and precision.
Author Contributions
All authors listed have made a substantial, direct and intellectual contribution to the work, and approved it for publication.
Funding
This research was funded in part by the National Natural Science Foundation of China (No. 81802669 to JL), the PUMC Youth Fund & the Fundamental Research Funds for the Central Universities (No. 3332019052 to YM), Non-profit Central Research Institute Fund of Chinese Academy of Medical Sciences (No. 2019PT320025), CAMS Initiative Fund for Medical Sciences (Nos. 2016-I2M-3-003 to NW, 2016-I2M-1-001 to XW, 2016-I2M-2-006, and 2017-I2M-2-001 to ZW), and the National Key Research and Development Program of China (No. 2018YFC0910506 to NW and ZW).
Conflict of Interest
The authors declare that the research was conducted in the absence of any commercial or financial relationships that could be construed as a potential conflict of interest.
References
1. Siegel RL, Miller KD, Jemal A. Cancer statistics, 2018. CA Cancer J Clin. (2018) 68:7–30. doi: 10.3322/caac.21442
2. Gradishar WJ, Anderson BO, Balassanian R, Blair SL, Burstein HJ, Cyr A, et al. Breast cancer, version 4.2017, NCCN clinical practice guidelines in oncology. J Natl Compr Canc Netw. (2018) 16:310–20. doi: 10.6004/jnccn.2018.0012
3. Pisano ED, Gatsonis C, Hendrick E, Yaffe M, Baum JK, Acharyya S, et al. Diagnostic performance of digital versus film mammography for breast-cancer screening. N Engl J Med. (2005) 353:1773–83. doi: 10.1056/NEJMoa052911
4. Tang S, Wei L, Sun Y, Zhou F, Zhu S, Yang R, et al. CA153 in breast secretions as a potential molecular marker for diagnosing breast cancer: a meta analysis. PLoS ONE. (2016) 11:e0163030. doi: 10.1371/journal.pone.0163030
5. Lee JH, Rosen EL, Mankoff DA. The role of radiotracer imaging in the diagnosis and management of patients with breast cancer: part 1–overview, detection, and staging. J Nucl Med. (2009) 50:569–81. doi: 10.2967/jnumed.108.053512
6. Mankoff DA. Molecular imaging as a tool for translating breast cancer science. Breast Cancer Res. (2008) 10(Suppl. 1):S3. doi: 10.1186/bcr2126
7. Quon A, Gambhir SS. FDG-PET and beyond: molecular breast cancer imaging. J Clin Oncol. (2005) 23:1664–73. doi: 10.1200/JCO.2005.11.024
8. Buck AK, Schirrmeister H, Mattfeldt T, Reske SN. Biological characterisation of breast cancer by means of PET. Eur J Nucl Med Mol Imaging. (2004) 31(Suppl. 1):S80–7. doi: 10.1007/s00259-004-1529-6
9. Eubank WB, Mankoff DA. Evolving role of positron emission tomography in breast cancer imaging. Semin Nucl Med. (2005) 35:84–99. doi: 10.1053/j.semnuclmed.2004.11.001
10. Vranjesevic D, Schiepers C, Silverman DH, Quon A, Villalpando J, Dahlbom M, et al. Relationship between 18F-FDG uptake and breast density in women with normal breast tissue. J Nucl Med. (2003) 44:1238–1242.
11. Lakhani P, Maidment AD, Weinstein SP, Kung JW, Alavi A. Correlation between quantified breast densities from digital mammography and 18F-FDG PET uptake. Breast J. (2009) 15:339–47. doi: 10.1111/j.1524-4741.2009.00737.x
12. Avril N, Rose CA, Schelling M, Dose J, Kuhn W, Bense S, et al. Breast imaging with positron emission tomography and fluorine-18 fluorodeoxyglucose: use and limitations. J Clin Oncol. (2000) 18:3495–502. doi: 10.1200/JCO.2000.18.20.3495
13. Avril N, Menzel M, Dose J, Schelling M, Weber W, Janicke F, et al. Glucose metabolism of breast cancer assessed by 18F-FDG PET: histologic and immunohistochemical tissue analysis. J Nucl Med. (2001) 42:9–16.
14. Basu S, Chen W, Tchou J, Mavi A, Cermik T, Czerniecki B, et al. Comparison of triple-negative and estrogen receptor-positive/progesterone receptor-positive/HER2-negative breast carcinoma using quantitative fluorine-18 fluorodeoxyglucose/positron emission tomography imaging parameters: a potentially useful method for disease characterization. Cancer. (2008) 112:995–1000. doi: 10.1002/cncr.23226
15. Miyake KK, Nakamoto Y, Saji S, Sugie T, Kurihara K, Kanao S, et al. Impact of physiological hormonal fluctuations on (18)F-fluorodeoxyglucose uptake in breast cancer. Breast Cancer Res Treat. (2018) 169:437–46. doi: 10.1007/s10549-018-4711-4
16. Antunovic L, Gallivanone F, Sollini M, Sagona A, Invento A, Manfrinato G, et al. [F]FDG PET/CT features for the molecular characterization of primary breast tumors. Eur J Nuclear Med Mol Imaging. (2017) 44:1945–54. doi: 10.1007/s00259-017-3770-9
17. Lemarignier C, Martineau A, Teixeira L, Vercellino L, Espié M, Merlet P, et al. Correlation between tumour characteristics, SUV measurements, metabolic tumour volume, TLG and textural features assessed with F-FDG PET in a large cohort of oestrogen receptor-positive breast cancer patients. Eur J Nuclear Med Mol Imaging. (2017) 44:1145–54. doi: 10.1007/s00259-017-3641-4
18. Ha S, Park S, Bang JI, Kim EK, Lee HY. Metabolic radiomics for pretreatment F-FDG PET/CT to characterize locally advanced breast cancer: histopathologic characteristics, response to neoadjuvant chemotherapy, and prognosis. Sci Rep. (2017) 7:1556. doi: 10.1038/s41598-017-01524-7
19. Port ER, Yeung H, Gonen M, Liberman L, Caravelli J, Borgen P, et al. 18F-2-fluoro-2-deoxy-D-glucose positron emission tomography scanning affects surgical management in selected patients with high-risk, operable breast carcinoma. Ann Surg Oncol. (2006) 13:677–84. doi: 10.1245/ASO.2006.03.035
20. Heusner TA, Kuemmel S, Umutlu L, Koeninger A, Freudenberg LS, Hauth EA, et al. Breast cancer staging in a single session: whole-body PET/CT mammography. J Nucl Med. (2008) 49:1215–22. doi: 10.2967/jnumed.108.052050
21. Rosen EL, Turkington TG, Soo MS, Baker JA, Coleman RE. Detection of primary breast carcinoma with a dedicated, large-field-of-view FDG PET mammography device: initial experience. Radiology. (2005) 234:527–34. doi: 10.1148/radiol.2342040654
22. MacDonald L, Edwards J, Lewellen T, Haseley D, Rogers J, Kinahan P. Clinical imaging characteristics of the positron emission mammography camera: PEM flex solo II. J Nucl Med. (2009) 50:1666–75. doi: 10.2967/jnumed.109.064345
23. Levine EA, Freimanis RI, Perrier ND, Morton K, Lesko NM, Bergman S, et al. Positron emission mammography: initial clinical results. Ann Surg Oncol. (2003) 10:86–91. doi: 10.1245/ASO.2003.03.047
24. Bitencourt AG, Lima EN, Macedo BR, Conrado JL, Marques EF, Chojniak R. Can positron emission mammography help to identify clinically significant breast cancer in women with suspicious calcifications on mammography? Eur Radiol. (2017) 27:1893–900. doi: 10.1007/s00330-016-4576-z
25. Bowen SL, Wu Y, Chaudhari AJ, Fu L, Packard NJ, Burkett GW, et al. Initial characterization of a dedicated breast PET/CT scanner during human imaging. J Nucl Med. (2009) 50:1401–8. doi: 10.2967/jnumed.109.064428
26. Jung NY, Kim SH, Kim SH, Seo YY, Oh JK, Choi HS, et al. Effectiveness of breast MRI and (18)F-FDG PET/CT for the preoperative staging of invasive lobular carcinoma versus ductal carcinoma. J Breast Cancer. (2015) 18:63–72. doi: 10.4048/jbc.2015.18.1.63
27. Kong EJ, Chun KA, Bom HS, Lee J, Lee SJ, Cho IH. Initial experience of integrated PET/MR mammography in patients with invasive ductal carcinoma. Hell J Nucl Med. (2014) 17:171–6. doi: 10.1967/s002449910142
28. National Comprehensive Cancer Network (NCCN). NCCN clinical practice guidelines in oncology: breast cancer—version 2.2020. NCCN website. Available online at: www.nccn.org (accessed March 30, 2020).
29. Cho N, Han W, Han BK, Bae MS, Ko ES, Nam SJ, et al. Breast cancer screening with mammography plus ultrasonography or magnetic resonance imaging in women 50 years or younger at diagnosis and treated with breast conservation therapy. JAMA Oncol. (2017) 3:1495–502. doi: 10.1001/jamaoncol.2017.1256
30. Pujara AC, Kim E, Axelrod D, Melsaether AN. PET/MRI in breast cancer. J Magn Reson Imaging. (2019) 49:328–42. doi: 10.1002/jmri.26298
31. Botsikas D, Kalovidouri A, Becker M, Copercini M, Djema DA, Bodmer A, et al. Clinical utility of 18F-FDG-PET/MR for preoperative breast cancer staging. Eur Radiol. (2016) 26:2297–307. doi: 10.1007/s00330-015-4054-z
32. Heusner TA, Hahn S, Jonkmanns C, Kuemmel S, Otterbach F, Hamami ME, et al. Diagnostic accuracy of fused positron emission tomography/magnetic resonance mammography: initial results. Br J Radiol. (2011) 84:126–35. doi: 10.1259/bjr/93330765
33. Garcia-Velloso MJ, Ribelles MJ, Rodriguez M, Fernandez-Montero A, Sancho L, Prieto E, et al. MRI fused with prone FDG PET/CT improves the primary tumour staging of patients with breast cancer. Eur Radiol. (2017) 27:3190–8. doi: 10.1007/s00330-016-4685-8
34. Grueneisen J, Nagarajah J, Buchbender C, Hoffmann O, Schaarschmidt BM, Poeppel T, et al. Positron emission tomography/magnetic resonance imaging for local tumor staging in patients with primary breast cancer: a comparison with positron emission tomography/computed tomography and magnetic resonance imaging. Invest Radiol. (2015) 50:505–13. doi: 10.1097/RLI.0000000000000197
35. Bitencourt AG, Lima EN, Chojniak R, Marques EF, Souza JA, Andrade WP, et al. Multiparametric evaluation of breast lesions using PET-MRI: initial results and future perspectives. Medicine. (2014) 93:e115. doi: 10.1097/MD.0000000000000115
36. Pinker K, Bogner W, Baltzer P, Karanikas G, Magometschnigg H, Brader P, et al. Improved differentiation of benign and malignant breast tumors with multiparametric 18fluorodeoxyglucose positron emission tomography magnetic resonance imaging: a feasibility study. Clin Cancer Res. (2014) 20:3540–9. doi: 10.1158/1078-0432.CCR-13-2810
37. Jena A, Taneja S, Singh A, Negi P, Mehta SB, Sarin R. Role of pharmacokinetic parameters derived with high temporal resolution DCE MRI using simultaneous PET/MRI system in breast cancer: a feasibility study. Eur J Radiol. (2017) 86:261–6. doi: 10.1016/j.ejrad.2016.11.029
38. Margolis NE, Moy L, Sigmund EE, Freed M, McKellop J, Melsaether AN, et al. Assessment of aggressiveness of breast cancer using simultaneous 18F-FDG-PET and DCE-MRI: preliminary observation. Clin Nucl Med. (2016) 41:e355–61. doi: 10.1097/RLU.0000000000001254
39. Kim TH, Yoon JK, Kang DK, Lee SJ, Jung YS, Yim H, et al. Correlation between F-18 fluorodeoxyglucose positron emission tomography metabolic parameters and dynamic contrast-enhanced MRI-derived perfusion data in patients with invasive ductal breast carcinoma. Ann Surg Oncol. (2015) 22:3866–72. doi: 10.1245/s10434-015-4526-z
40. Ghandhari H, Tari HV, Ameri E, Safari MB, Fouladi DF. Vertebral, rib, and intraspinal anomalies in congenital scoliosis: a study on 202 Caucasians. Eur Spine J. (2015) 24:1510–21. doi: 10.1007/s00586-015-3833-1
41. Catalano OA, Horn GL, Signore A, Iannace C, Lepore M, Vangel M, et al. PET/MR in invasive ductal breast cancer: correlation between imaging markers and histological phenotype. Br J Cancer. (2017) 116:893–902. doi: 10.1038/bjc.2017.26
42. Wu D, Gambhir SS. Positron emission tomography in diagnosis and management of invasive breast cancer: current status and future perspectives. Clin Breast Cancer. (2003) 4(Suppl. 1):S55–63. doi: 10.3816/CBC.2003.s.016
43. Suarez M, Perez-Castejon MJ, Jimenez A, Domper M, Ruiz G, Montz R, et al. Early diagnosis of recurrent breast cancer with FDG-PET in patients with progressive elevation of serum tumor markers. Q J Nucl Med. (2002) 46:113–21.
44. Brix G, Lechel U, Glatting G, Ziegler SI, Munzing W, Muller SP, et al. Radiation exposure of patients undergoing whole-body dual-modality 18F-FDG PET/CT examinations. J Nucl Med. (2005) 46:608–13.
45. Ergul N, Kadioglu H, Yildiz S, Yucel SB, Gucin Z, Erdogan EB, et al. Assessment of multifocality and axillary nodal involvement in early-stage breast cancer patients using 18F-FDG PET/CT compared to contrast-enhanced and diffusion-weighted magnetic resonance imaging and sentinel node biopsy. Acta Radiol. (2015) 56:917–23. doi: 10.1177/0284185114539786
46. Song BI, Kim HW, Won KS. Predictive value of F-FDG PET/CT for axillary lymph node metastasis in invasive ductal breast cancer. Ann Surg Oncol. (2017) 24:2174–81. doi: 10.1245/s10434-017-5860-0
47. Taneja S, Jena A, Goel R, Sarin R, Kaul S. Simultaneous whole-body (1)(8)F-FDG PET-MRI in primary staging of breast cancer: a pilot study. Eur J Radiol. (2014) 83:2231–9. doi: 10.1016/j.ejrad.2014.09.008
48. van Nijnatten TJA, Goorts B, Vöö S, de Boer M, Kooreman LFS, Heuts EM, et al. Added value of dedicated axillary hybrid 18F-FDG PET/MRI for improved axillary nodal staging in clinically node-positive breast cancer patients: a feasibility study. Eur J Nucl Med Mol Imaging. (2018) 45:179–86. doi: 10.1007/s00259-017-3823-0
49. Huang B, Law MW, Khong PL. Whole-body PET/CT scanning: estimation of radiation dose and cancer risk. Radiology. (2009) 251:166–74. doi: 10.1148/radiol.2511081300
50. Kutluturk K, Simsek A, Comak A, Gonultas F, Unal B, Kekilli E. Factors affecting the accuracy of (18)F-FDG PET/CT in evaluating axillary metastases in invasive breast cancer. Niger J Clin Pract. (2019) 22:63–8. doi: 10.4103/njcp.njcp_198_18
51. Wahl RL, Siegel BA, Coleman RE, Gatsonis CG, Group PETS. Prospective multicenter study of axillary nodal staging by positron emission tomography in breast cancer: a report of the staging breast cancer with PET study group. J Clin Oncol. (2004) 22:277–85. doi: 10.1200/JCO.2004.04.148
52. van der Noordaa MEM, van Duijnhoven FH, Straver ME, Groen EJ, Stokkel M, Loo CE, et al. Major reduction in axillary lymph node dissections after neoadjuvant systemic therapy for node-positive breast cancer by combining PET/CT and the MARI procedure. Ann Surg Oncol. (2018) 25:1512–20. doi: 10.1245/s10434-018-6404-y
53. Eubank WB, Mankoff DA, Takasugi J, Vesselle H, Eary JF, Shanley TJ, et al. 18fluorodeoxyglucose positron emission tomography to detect mediastinal or internal mammary metastases in breast cancer. J Clin Oncol. (2001) 19:3516–23. doi: 10.1200/JCO.2001.19.15.3516
54. Jochelson MS, Lebron L, Jacobs SS, Zheng J, Moskowitz CS, Powell SN, et al. Detection of internal mammary adenopathy in patients with breast cancer by PET/CT and MRI. AJR Am J Roentgenol. (2015) 205:899–904. doi: 10.2214/AJR.14.13804
55. Groheux D, Hindie E, Delord M, Giacchetti S, Hamy AS, de Bazelaire C, et al. Prognostic impact of (18)FDG-PET-CT findings in clinical stage III and IIB breast cancer. J Natl Cancer Inst. (2012) 104:1879–87. doi: 10.1093/jnci/djs451
56. Ulaner GA, Castillo R, Wills J, Gönen M, Goldman DA. F-FDG-PET/CT for systemic staging of patients with newly diagnosed ER-positive and HER2-positive breast cancer. Eur J Nuclear Med Mol Imaging. (2017) 44:1420–7. doi: 10.1007/s00259-017-3709-1
57. Borst MJ, Ingold JA. Metastatic patterns of invasive lobular versus invasive ductal carcinoma of the breast. Surgery. (1993) 114:637–41.
58. Plecha DM, Faulhaber P. PET/MRI of the breast. Eur J Radiol. (2017) 94:A26–A34. doi: 10.1016/j.ejrad.2017.05.006
59. Melsaether AN, Raad RA, Pujara AC, Ponzo FD, Pysarenko KM, Jhaveri K, et al. Comparison of whole-body (18)F FDG PET/MR imaging and whole-body (18)F FDG PET/CT in terms of lesion detection and radiation dose in patients with breast cancer. Radiology. (2016) 281:193–202. doi: 10.1148/radiol.2016151155
60. Catalano OA, Daye D, Signore A, Iannace C, Vangel M, Luongo A, et al. Staging performance of whole-body DWI, PET/CT and PET/MRI in invasive ductal carcinoma of the breast. Int J Oncol. (2017) 51:281–8. doi: 10.3892/ijo.2017.4012
61. Griffeth LK, Rich KM, Dehdashti F, Simpson JR, Fusselman MJ, McGuire AH, et al. Brain metastases from non-central nervous system tumors: evaluation with PET. Radiology. (1993) 186:37–44. doi: 10.1148/radiology.186.1.8416584
62. Kitajima K, Nakamoto Y, Okizuka H, Onishi Y, Senda M, Suganuma N, et al. Accuracy of whole-body FDG-PET/CT for detecting brain metastases from non-central nervous system tumors. Ann Nucl Med. (2008) 22:595–602. doi: 10.1007/s12149-008-0145-0
63. Rappeport ED, Loft A, Berthelsen AK, von der Recke P, Larsen PN, Mogensen AM, et al. Contrast-enhanced FDG-PET/CT vs. SPIO-enhanced MRI vs. FDG-PET vs. CT in patients with liver metastases from colorectal cancer: a prospective study with intraoperative confirmation. Acta Radiol. (2007) 48:369–78. doi: 10.1080/02841850701294560
64. Even-Sapir E. Imaging of malignant bone involvement by morphologic, scintigraphic, and hybrid modalities. J Nucl Med. (2005) 46:1356–67.
65. Du Y, Cullum I, Illidge TM, Ell PJ. Fusion of metabolic function and morphology: sequential [18F]fluorodeoxyglucose positron-emission tomography/computed tomography studies yield new insights into the natural history of bone metastases in breast cancer. J Clin Oncol. (2007) 25:3440–7. doi: 10.1200/JCO.2007.11.2854
66. Tateishi U, Gamez C, Dawood S, Yeung HW, Cristofanilli M, Macapinlac HA. Bone metastases in patients with metastatic breast cancer: morphologic and metabolic monitoring of response to systemic therapy with integrated PET/CT. Radiology. (2008) 247:189–96. doi: 10.1148/radiol.2471070567
67. Ulaner GA, Zindman AM, Zheng J, Kim TW, Healey JH. FDG PET/CT assesses the risk of femoral pathological fractures in patients with metastatic breast cancer. Clin Nucl Med. (2017) 42:264–70. doi: 10.1097/RLU.0000000000001580
68. Roop MJ, Singh B, Singh H, Watts A, Kohli PS, Mittal BR, et al. Incremental value of cocktail 18F-FDG and 18F-NaF PET/CT over 18F-FDG PET/CT alone for characterization of skeletal metastases in breast cancer. Clin Nucl Med. (2017) 42:335–40. doi: 10.1097/RLU.0000000000001615
69. Sawicki LM, Grueneisen J, Schaarschmidt BM, Buchbender C, Nagarajah J, Umutlu L, et al. Evaluation of (1)(8)F-FDG PET/MRI, (1)(8)F-FDG PET/CT, MRI, and CT in whole-body staging of recurrent breast cancer. Eur J Radiol. (2016) 85:459–65. doi: 10.1016/j.ejrad.2015.12.010
70. Catalano OA, Nicolai E, Rosen BR, Luongo A, Catalano M, Iannace C, et al. Comparison of CE-FDG-PET/CT with CE-FDG-PET/MR in the evaluation of osseous metastases in breast cancer patients. Br J Cancer. (2015) 112:1452–60. doi: 10.1038/bjc.2015.112
71. Raad RA, Friedman KP, Heacock L, Ponzo F, Melsaether A, Chandarana H. Outcome of small lung nodules missed on hybrid PET/MRI in patients with primary malignancy. J Magn Reson Imaging. (2016) 43:504–11. doi: 10.1002/jmri.25005
72. van Oost FJ, van der Hoeven JJ, Hoekstra OS, Voogd AC, Coebergh JW, van de Poll-Franse LV. Staging in patients with locoregionally recurrent breast cancer: current practice and prospects for positron emission tomography. Eur J Cancer. (2004) 40:1545–53. doi: 10.1016/j.ejca.2004.03.012
73. Rezk M, Nasr I, Ali I, Abdelhamed H. Comparative study between (18)F FDG-PET/CT and whole body MRI DWIBS in assessment of recurrent breast cancer (prospective, comparative, cross-sectional study design). Indian J Nucl Med. (2019) 34:1–9. doi: 10.4103/ijnm.IJNM_121_18
74. Pennant M, Takwoingi Y, Pennant L, Davenport C, Fry-Smith A, Eisinga A, et al. A systematic review of positron emission tomography (PET) and positron emission tomography/computed tomography (PET/CT) for the diagnosis of breast cancer recurrence. Health Technol Assess. (2010) 14:1–103. doi: 10.3310/hta14500
75. Hildebrandt MG, Gerke O, Baun C, Falch K, Hansen JA, Farahani ZA, et al. [18F]Fluorodeoxyglucose (FDG)-Positron Emission Tomography (PET)/Computed Tomography (CT) in suspected recurrent breast cancer: a prospective comparative study of dual-time-point FDG-PET/CT, Contrast-Enhanced CT, and bone scintigraphy. J Clin Oncol. (2016) 34:1889–97. doi: 10.1200/JCO.2015.63.5185
76. Kim YI, Kim YJ, Paeng JC, Cheon GJ, Lee DS, Chung JK, et al. Prediction of breast cancer recurrence using lymph node metabolic and volumetric parameters from F-FDG PET/CT in operable triple-negative breast cancer. Eur J Nucl Med Mol Imaging. (2017) 44:1787–95. doi: 10.1007/s00259-017-3748-7
77. Guo W, Hao B, Luo N, Ruan D, Guo X, Chen HJ, et al. Early re-staging and molecular subtype shift surveillance of locally recurrent or metastatic breast cancer: a new PET/CT integrated precise algorithm. Cancer Lett. (2018) 418:221–9. doi: 10.1016/j.canlet.2018.01.019
78. Riola-Parada C, Garcia-Canamaque L, Perez-Duenas V, Garcerant-Tafur M, Carreras-Delgado JL. Simultaneous PET/MRI vs PET/CT in oncology. a systematic review. Rev Esp Med Nucl Imagen Mol. (2016) 35:306–12. doi: 10.1016/j.remnie.2016.06.006
79. Magometschnigg HF, Baltzer PA, Fueger B, Helbich TH, Karanikas G, Dubsky P, et al. Diagnostic accuracy of (18)F-FDG PET/CT compared with that of contrast-enhanced MRI of the breast at 3 T. Eur J Nucl Med Mol Imaging. (2015) 42:1656–65. doi: 10.1007/s00259-015-3099-1
80. Gillies RJ, Kinahan PE, Hricak H. Radiomics: images are more than pictures, they are data. Radiology. (2016) 278:563–77. doi: 10.1148/radiol.2015151169
81. Mankoff DA, Dunnwald LK, Gralow JR, Ellis GK, Charlop A, Lawton TJ, et al. Blood flow and metabolism in locally advanced breast cancer: relationship to response to therapy. J Nucl Med. (2002) 43:500–9.
82. Schelling M, Avril N, Nahrig J, Kuhn W, Romer W, Sattler D, et al. Positron emission tomography using [(18)F]Fluorodeoxyglucose for monitoring primary chemotherapy in breast cancer. J Clin Oncol. (2000) 18:1689–95. doi: 10.1200/JCO.2000.18.8.1689
83. Rousseau C, Devillers A, Sagan C, Ferrer L, Bridji B, Campion L, et al. Monitoring of early response to neoadjuvant chemotherapy in stage II and III breast cancer by [18F]fluorodeoxyglucose positron emission tomography. J Clin Oncol. (2006) 24:5366–72. doi: 10.1200/JCO.2006.05.7406
84. Cachin F, Prince HM, Hogg A, Ware RE, Hicks RJ. Powerful prognostic stratification by [18F]fluorodeoxyglucose positron emission tomography in patients with metastatic breast cancer treated with high-dose chemotherapy. J Clin Oncol. (2006) 24:3026–31. doi: 10.1200/JCO.2005.04.6326
85. Dunnwald LK, Gralow JR, Ellis GK, Livingston RB, Linden HM, Specht JM, et al. Tumor metabolism and blood flow changes by positron emission tomography: relation to survival in patients treated with neoadjuvant chemotherapy for locally advanced breast cancer. J Clin Oncol. (2008) 26:4449–57. doi: 10.1200/JCO.2007.15.4385
86. Wang HC, Lo SS. Future prospects of neoadjuvant chemotherapy in treatment of primary breast cancer. Semin Surg Oncol. (1996) 12:59–66. doi: 10.1002/(SICI)1098-2388(199601/02)12:1<59::AID-SSU9>3.0.CO;2-5
87. Kumar A, Kumar R, Seenu V, Gupta SD, Chawla M, Malhotra A, et al. The role of 18F-FDG PET/CT in evaluation of early response to neoadjuvant chemotherapy in patients with locally advanced breast cancer. Eur Radiol. (2009) 19:1347–57. doi: 10.1007/s00330-009-1303-z
88. Tian F, Shen G, Deng Y, Diao W, Jia Z. The accuracy of 18F-FDG PET/CT in predicting the pathological response to neoadjuvant chemotherapy in patients with breast cancer: a meta-analysis and systematic review. Eur Radiol. (2017) 27:4786–96. doi: 10.1007/s00330-017-4831-y
89. Wang Y, Zhang C, Liu J, Huang G. Is 18F-FDG PET accurate to predict neoadjuvant therapy response in breast cancer? a meta-analysis. Breast Cancer Res Treat. (2012) 131:357–69. doi: 10.1007/s10549-011-1780-z
90. Chen S, Ibrahim NK, Yan Y, Wong ST, Wang H, Wong FC. Complete metabolic response on interim F-Fluorodeoxyglucose positron emission tomography/computed tomography to predict long-term survival in patients with breast cancer undergoing neoadjuvant chemotherapy. Oncologist. (2017) 22:526–34. doi: 10.1634/theoncologist.2016-0334
91. Groheux D, Biard L, Lehmann-Che J, Teixeira L, Bouhidel FA, Poirot B, et al. Tumor metabolism assessed by FDG-PET/CT and tumor proliferation assessed by genomic grade index to predict response to neoadjuvant chemotherapy in triple negative breast cancer. Eur J Nucl Med Mol Imaging. (2018) 45:1279–88. doi: 10.1007/s00259-018-3998-z
92. Dose Schwarz J, Bader M, Jenicke L, Hemminger G, Janicke F, Avril N. Early prediction of response to chemotherapy in metastatic breast cancer using sequential 18F-FDG PET. J Nucl Med. (2005) 46:1144–50.
93. Gennari A, Donati S, Salvadori B, Giorgetti A, Salvadori PA, Sorace O, et al. Role of 2-[18F]-fluorodeoxyglucose (FDG) positron emission tomography (PET) in the early assessment of response to chemotherapy in metastatic breast cancer patients. Clin Breast Cancer. (2000) 1:156–61. doi: 10.3816/CBC.2000.n.014
94. Couturier O, Jerusalem G, N'Guyen JM, Hustinx R. Sequential positron emission tomography using [18F]fluorodeoxyglucose for monitoring response to chemotherapy in metastatic breast cancer. Clin Cancer Res. (2006) 12:6437–43. doi: 10.1158/1078-0432.CCR-06-0383
95. Cho N, Im SA, Cheon GJ, Park IA, Lee KH, Kim TY, et al. Integrated (18)F-FDG PET/MRI in breast cancer: early prediction of response to neoadjuvant chemotherapy. Eur J Nucl Med Mol Imaging. (2018) 45:328–39. doi: 10.1007/s00259-017-3849-3
96. Wang J, Shih TT, Yen RF. Multiparametric evaluation of treatment response to neoadjuvant chemotherapy in breast cancer using integrated PET/MR. Clin Nucl Med. (2017) 42:506–13. doi: 10.1097/RLU.0000000000001684
97. Pengel KE, Koolen BB, Loo CE, Vogel WV, Wesseling J, Lips EH, et al. Combined use of (1)(8)F-FDG PET/CT and MRI for response monitoring of breast cancer during neoadjuvant chemotherapy. Eur J Nucl Med Mol Imaging. (2014) 41:1515–24. doi: 10.1007/s00259-014-2770-2
98. An YY, Kim SH, Kang BJ, Lee AW. Treatment response evaluation of breast cancer after neoadjuvant chemotherapy and usefulness of the imaging parameters of MRI and PET/CT. J Korean Med Sci. (2015) 30:808–15. doi: 10.3346/jkms.2015.30.6.808
99. Even-Sapir E, Inbar M. PET in women with high risk for breast or ovarian cancer. Lancet Oncol. (2010) 11:899–905. doi: 10.1016/S1470-2045(10)70022-X
100. Sun J, Meng H, Yao L, Lv M, Bai J, Zhang J, et al. Germline mutations in cancer susceptibility genes in a large series of unselected breast cancer patients. Clin Cancer Res. (2017) 23:6113–9. doi: 10.1158/1078-0432.CCR-16-3227
101. Smyczek-Gargya B, Fersis N, Dittmann H, Vogel U, Reischl G, Machulla HJ, et al. PET with [18F]fluorothymidine for imaging of primary breast cancer: a pilot study. Eur J Nucl Med Mol Imaging. (2004) 31:720–4. doi: 10.1007/s00259-004-1462-8
102. Kenny L, Coombes RC, Vigushin DM, Al-Nahhas A, Shousha S, Aboagye EO. Imaging early changes in proliferation at 1 week post chemotherapy: a pilot study in breast cancer patients with 3'-deoxy-3'-[18F]fluorothymidine positron emission tomography. Eur J Nucl Med Mol Imaging. (2007) 34:1339–47. doi: 10.1007/s00259-007-0379-4
103. Zang J, Mao F, Wang H, Zhang J, Liu Q, Peng L, et al. 68Ga-NOTA-RM26 PET/CT in the evaluation of breast cancer: a pilot prospective study. Clin Nucl Med. (2018) 43:663–9. doi: 10.1097/RLU.0000000000002209
104. Zhang J, Mao F, Niu G, Peng L, Lang L, Li F, et al. Ga-BBN-RGD PET/CT for GRPR and integrin αβ imaging in patients with breast cancer. Theranostics. (2018) 8:1121–30. doi: 10.7150/thno.22601
105. Kim YI, Yoon HJ, Paeng JC, Cheon GJ, Lee DS, Chung JK, et al. Prognostic value of 68Ga-NOTA-RGD PET/CT for predicting disease-free survival for patients with breast cancer undergoing neoadjuvant chemotherapy and surgery: a comparison study with dynamic contrast enhanced MRI. Clin Nucl Med. (2016) 41:614–20. doi: 10.1097/RLU.0000000000001274
106. Ulaner GA, Goldman DA, Corben A, Lyashchenko SK, Gönen M, Lewis JS, et al. Prospective clinical trial of F-Fluciclovine PET/CT for determining the response to neoadjuvant therapy in invasive ductal and invasive lobular breast cancers. J Nucl Med. (2017) 58:1037–42. doi: 10.2967/jnumed.116.183335
107. Gill HS, Tade F, Greenwald DT, Yonover PM, Savir-Baruch B. Metastatic male breast cancer with increased uptake on 18F-Fluciclovine PET/CT scan. Clin Nucl Med. (2018) 43:23–4. doi: 10.1097/RLU.0000000000001893
108. Tade FI, Cohen MA, Styblo TM, Odewole OA, Holbrook AI, Newell MS, et al. Anti-3-18F-FACBC (18F-Fluciclovine) PET/CT of breast cancer: an exploratory study. J Nucl Med. (2016) 57:1357–63. doi: 10.2967/jnumed.115.171389
109. Ulaner GA, Goldman DA, Gönen M, Pham H, Castillo R, Lyashchenko SK, et al. Initial results of a prospective clinical trial of 18F-fluciclovine PET/CT in newly diagnosed invasive ductal and invasive lobular breast cancers. J Nucl Med. (2016) 57:1350–6. doi: 10.2967/jnumed.115.170456
110. Malik D, Kumar R, Mittal BR, Singh H, Bhattacharya A, Singh SK. 68Ga-labeled PSMA uptake in nonprostatic malignancies: has the time come to remove “PS” From PSMA? Clin Nucl Med. (2018) 43:529–32. doi: 10.1097/RLU.0000000000002103
111. Passah A, Arora S, Damle NA, Tripathi M, Bal C, Subudhi TK, et al. 68Ga-prostate-specific membrane antigen PET/CT in triple-negative breast cancer. Clin Nucl Med. (2018) 43:460–1. doi: 10.1097/RLU.0000000000002071
112. Kumar R, Mittal BR, Bhattacharya A, Singh H, Singh SK. Synchronous detection of male breast cancer and prostatic cancer in a patient with suspected prostatic carcinoma on 68Ga-PSMA PET/CT imaging. Clin Nucl Med. (2018) 43:431–2. doi: 10.1097/RLU.0000000000002063
113. Cuppari L, Reccia P, Burei M, Cervino AR, Evangelista L. 18F-Choline PET/CT in leptomeningeal breast cancer metastases. Clin Nucl Med. (2019) 44:e96–7. doi: 10.1097/RLU.0000000000002407
114. Hugentobler A, Gilbeau L, Talbot JN, Gauthé M. 18F-fluorocholine PET/CT of incidental male breast cancer. Clin Nucl Med. (2017) 42:e56–7. doi: 10.1097/RLU.0000000000001429
115. Passah A, Tripathi M, Ballal S, Yadav MP, Kumar R, Roesch F, et al. Evaluation of bone-seeking novel radiotracer Ga-NO2AP-Bisphosphonate for the detection of skeletal metastases in carcinoma breast. Eur J Nucl Med Mol Imaging. (2017) 44:41–9. doi: 10.1007/s00259-016-3469-3
116. Ulaner GA, Hyman DM, Lyashchenko SK, Lewis JS, Carrasquillo JA. 89Zr-Trastuzumab PET/CT for detection of human epidermal growth factor receptor 2-positive metastases in patients with human epidermal growth factor receptor 2-negative primary breast cancer. Clin Nucl Med. (2017) 42:912–7. doi: 10.1097/RLU.0000000000001820
117. Mortimer JE, Bading JR, Park JM, Frankel PH, Carroll MI, Tran TT, et al. Tumor uptake of Cu-DOTA-trastuzumab in patients with metastatic breast cancer. J Nucl Med. (2018) 59:38–43. doi: 10.2967/jnumed.117.193888
118. Sasada S, Kurihara H, Kinoshita T, Yoshida M, Honda N, Shimoi T, et al. 64Cu-DOTA-trastuzumab PET imaging for HER2-specific primary lesions of breast cancer. Ann Oncol. (2017) 28:2028–9. doi: 10.1093/annonc/mdx227
119. Ulaner GA, Lyashchenko SK, Riedl C, Ruan S, Zanzonico PB, Lake D, et al. First-in-Human human epidermal growth factor receptor 2-targeted imaging using Zr-pertuzumab PET/CT: dosimetry and clinical application in patients with breast cancer. J Nucl Med. (2018) 59:900–6. doi: 10.2967/jnumed.117.202010
120. Sandberg D, Tolmachev V, Velikyan I, Olofsson H, Wennborg A, Feldwisch J, et al. Intra-image referencing for simplified assessment of HER2-expression in breast cancer metastases using the Affibody molecule ABY-025 with PET and SPECT. Eur J Nucl Med Mol Imaging. (2017) 44:1337–46. doi: 10.1007/s00259-017-3650-3
121. Chae SY, Kim SB, Ahn SH, Kim HO, Yoon DH, Ahn JH, et al. A randomized feasibility study of F-Fluoroestradiol PET to predict pathologic response to neoadjuvant therapy in estrogen receptor-rich postmenopausal breast cancer. J Nucl Med. (2017) 58:563–8. doi: 10.2967/jnumed.116.178368
122. Lin FI, Gonzalez EM, Kummar S, Do K, Shih J, Adler S, et al. Utility of F-fluoroestradiol (F-FES) PET/CT imaging as a pharmacodynamic marker in patients with refractory estrogen receptor-positive solid tumors receiving Z-endoxifen therapy. Eur J Nucl Med Mol Imaging. (2017) 44:500–8. doi: 10.1007/s00259-016-3561-8
123. Gong C, Yang Z, Sun Y, Zhang J, Zheng C, Wang L, et al. A preliminary study of F-FES PET/CT in predicting metastatic breast cancer in patients receiving docetaxel or fulvestrant with docetaxel. Sci Rep. (2017) 7:6584. doi: 10.1038/s41598-017-06903-8
124. Papadakis GZ, Millo C, Sadowski SM, Karantanas AH, Bagci U, Patronas NJ. Breast fibroadenoma with increased activity on 68Ga DOTATATE PET/CT. Clin Nucl Med. (2017) 42:145–6. doi: 10.1097/RLU.0000000000001463
125. Beer AJ, Niemeyer M, Carlsen J, Sarbia M, Nahrig J, Watzlowik P, et al. Patterns of alphavbeta3 expression in primary and metastatic human breast cancer as shown by 18F-Galacto-RGD PET. J Nucl Med. (2008) 49:255–9. doi: 10.2967/jnumed.107.045526
126. Hood JD, Cheresh DA. Role of integrins in cell invasion and migration. Nat Rev Cancer. (2002) 2:91–100. doi: 10.1038/nrc727
127. Parihar AS, Mittal BR, Sood A, Basher RK, Singh G. 68Ga-prostate-specific membrane antigen PET/CT and 18F-FDG PET/CT of primary signet ring cell breast adenocarcinoma. Clin Nucl Med. (2018) 43:e414–6. doi: 10.1097/RLU.0000000000002265
128. Dijkers EC, Oude Munnink TH, Kosterink JG, Brouwers AH, Jager PL, de Jong JR, et al. Biodistribution of 89Zr-trastuzumab and PET imaging of HER2-positive lesions in patients with metastatic breast cancer. Clin Pharmacol Ther. (2010) 87:586–92. doi: 10.1038/clpt.2010.12
129. Gaykema SB, de Jong JR, Perik PJ, Brouwers AH, Schroder CP, Oude Munnink TH, et al. (111)In-trastuzumab scintigraphy in HER2-positive metastatic breast cancer patients remains feasible during trastuzumab treatment. Mol Imaging. (2014) 13:11. doi: 10.2310/7290.2014.00011
130. Gaykema SB, Schroder CP, Vitfell-Rasmussen J, Chua S, Oude Munnink TH, Brouwers AH, et al. 89Zr-trastuzumab and 89Zr-bevacizumab PET to evaluate the effect of the HSP90 inhibitor NVP-AUY922 in metastatic breast cancer patients. Clin Cancer Res. (2014) 20:3945–54. doi: 10.1158/1078-0432.CCR-14-0491
131. Gemignani ML, Patil S, Seshan VE, Sampson M, Humm JL, Lewis JS, et al. Feasibility and predictability of perioperative PET and estrogen receptor ligand in patients with invasive breast cancer. J Nucl Med. (2013) 54:1697–702. doi: 10.2967/jnumed.112.113373
132. Katzenellenbogen JA. Designing steroid receptor-based radiotracers to image breast and prostate tumors. J Nucl Med. (1995) 36(Suppl. 6):8S−13.
133. Peterson LM, Mankoff DA, Lawton T, Yagle K, Schubert EK, Stekhova S, et al. Quantitative imaging of estrogen receptor expression in breast cancer with PET and 18F-fluoroestradiol. J Nucl Med. (2008) 49:367–74. doi: 10.2967/jnumed.107.047506
134. Perez EA, Suman VJ, Davidson NE, Martino S, Kaufman PA, Lingle WL, et al. HER2 testing by local, central, and reference laboratories in specimens from the North Central cancer treatment group N9831 intergroup adjuvant trial. J Clin Oncol. (2006) 24:3032–8. doi: 10.1200/JCO.2005.03.4744
135. Dijkers EC, Kosterink JG, Rademaker AP, Perk LR, van Dongen GA, Bart J, et al. Development and characterization of clinical-grade 89Zr-trastuzumab for HER2/neu immunoPET imaging. J Nucl Med. (2009) 50:974–81. doi: 10.2967/jnumed.108.060392
136. Ahlgren S, Wallberg H, Tran TA, Widstrom C, Hjertman M, Abrahmsen L, et al. Targeting of HER2-expressing tumors with a site-specifically 99mTc-labeled recombinant affibody molecule, ZHER2:2395, with C-terminally engineered cysteine. J Nucl Med. (2009) 50:781–9. doi: 10.2967/jnumed.108.056929
137. Tolmachev V, Orlova A. Affibody molecules as targeting vectors for PET imaging. Cancers. (2020) 12:651. doi: 10.3390/cancers12030651
138. Sollini M, Cozzi L, Ninatti G, Antunovic L, Cavinato L, Chiti A, et al. PET/CT radiomics in breast cancer: mind the step. Methods. (in press) doi: 10.1016/j.ymeth.2020.01.007
139. Ou X, Wang J, Zhou R, Zhu S, Pang F, Zhou Y, et al. Ability of (18)F-FDG PET/CT radiomic features to distinguish breast carcinoma from breast lymphoma. Contrast Media Mol Imaging. (2019) 2019:4507694. doi: 10.1155/2019/4507694
140. Vogl WD, Pinker K, Helbich TH, Bickel H, Grabner G, Bogner W, et al. Automatic segmentation and classification of breast lesions through identification of informative multiparametric PET/MRI features. Eur Radiol Exp. (2019) 3:18. doi: 10.1186/s41747-019-0096-3
141. Acar E, Turgut B, Yigit S, Kaya G. Comparison of the volumetric and radiomics findings of 18F-FDG PET/CT images with immunohistochemical prognostic factors in local/locally advanced breast cancer. Nucl Med Commun. (2019) 40:764–72. doi: 10.1097/MNM.0000000000001019
142. Huang SY, Franc BL, Harnish RJ, Liu G, Mitra D, Copeland TP, et al. Exploration of PET and MRI radiomic features for decoding breast cancer phenotypes and prognosis. NPJ Breast Cancer. (2018) 4:24. doi: 10.1038/s41523-018-0078-2
143. Moscoso A, Ruibal A, Dominguez-Prado I, Fernandez-Ferreiro A, Herranz M, Albaina L, et al. Texture analysis of high-resolution dedicated breast (18) F-FDG PET images correlates with immunohistochemical factors and subtype of breast cancer. Eur J Nucl Med Mol Imaging. (2018) 45:196–206. doi: 10.1007/s00259-017-3830-1
144. Antunovic L, De Sanctis R, Cozzi L, Kirienko M, Sagona A, Torrisi R, et al. PET/CT radiomics in breast cancer: promising tool for prediction of pathological response to neoadjuvant chemotherapy. Eur J Nucl Med Mol Imaging. (2019) 46:1468–77. doi: 10.1007/s00259-019-04313-8
145. Lee H, Lee DE, Park S, Kim TS, Jung SY, Lee S, et al. Predicting response to neoadjuvant chemotherapy in patients with breast cancer: combined statistical modeling using clinicopathological factors and FDG PET/CT texture parameters. Clin Nucl Med. (2019) 44:21–9. doi: 10.1097/RLU.0000000000002348
146. von Minckwitz G, Untch M, Blohmer JU, Costa SD, Eidtmann H, Fasching PA, et al. Definition and impact of pathologic complete response on prognosis after neoadjuvant chemotherapy in various intrinsic breast cancer subtypes. J Clin Oncol. (2012) 30:1796–804. doi: 10.1200/JCO.2011.38.8595
147. Molina-Garcia D, Garcia-Vicente AM, Perez-Beteta J, Amo-Salas M, Martinez-Gonzalez A, Tello-Galan MJ, et al. Intratumoral heterogeneity in (18)F-FDG PET/CT by textural analysis in breast cancer as a predictive and prognostic subrogate. Ann Nucl Med. (2018) 32:379–88. doi: 10.1007/s12149-018-1253-0
148. Yoon HJ, Kim Y, Chung J, Kim BS. Predicting neo-adjuvant chemotherapy response and progression-free survival of locally advanced breast cancer using textural features of intratumoral heterogeneity on F-18 FDG PET/CT and diffusion-weighted MR imaging. Breast J. (2019) 25:373–80. doi: 10.1111/tbj.13032
Keywords: breast cancer, PET, PET/CT, PET/MR, molecular imaging
Citation: Ming Y, Wu N, Qian T, Li X, Wan DQ, Li C, Li Y, Wu Z, Wang X, Liu J and Wu N (2020) Progress and Future Trends in PET/CT and PET/MRI Molecular Imaging Approaches for Breast Cancer. Front. Oncol. 10:1301. doi: 10.3389/fonc.2020.01301
Received: 28 October 2019; Accepted: 23 June 2020;
Published: 12 August 2020.
Edited by:
Georgios S. Limouris, National and Kapodistrian University of Athens, GreeceReviewed by:
Laurence Gluch, The Strathfield Breast Centre, AustraliaMin-Ying Su, University of California, Irvine, United States
Athanasios G. Zafeirakis, Army Share Fund Hospital (NIMTS), Greece
Copyright © 2020 Ming, Wu, Qian, Li, Wan, Li, Li, Wu, Wang, Liu and Wu. This is an open-access article distributed under the terms of the Creative Commons Attribution License (CC BY). The use, distribution or reproduction in other forums is permitted, provided the original author(s) and the copyright owner(s) are credited and that the original publication in this journal is cited, in accordance with accepted academic practice. No use, distribution or reproduction is permitted which does not comply with these terms.
*Correspondence: Jiaqi Liu, ai5saXVAY2ljYW1zLmFjLmNu; Ning Wu, Y2pyLnd1bmluZ0B2aXAuMTYzLmNvbQ==
†These authors have contributed equally to this work