- 1Department of Neurosurgery, Affiliated Hospital of Qingdao University, Qingdao, China
- 2Department of Pharmacology and Toxicology, University of Mississippi Medical Center, Jackson, MS, United States
- 3Department of Nursing, Medical College of Qingdao University, Qingdao, China
Pineoblastoma (PB) is a rare neoplasm of the central nervous system. This analysis aimed to identify factors and establish a predictive model for the prognosis of adult patients with PB. Data for 213 adult patients with PB (Surveillance, Epidemiology, and End Results database) were randomly divided into primary and validation cohorts. A predictive model was established and optimized based on the Akaike Information Criterion and visualized by a nomogram. Its predictive performance (concordance index and receiver operating characteristic curve) and clinical utility (decision curve analyses) were evaluated. We internally and externally validated the model using calibration curves. Multivariate Cox regression analysis identified age, year of diagnosis, therapy, tumor size, and tumor extension as independent predictors of PB. The model exhibited great discriminative ability (concordance index of the nomogram: 0.802; 95% confidence interval: 0.78–0.83; area under the receiver operating characteristic curve: ranging from 0.7 to 0.8). Calibration plots (probability of survival) showed good consistency between the actual observation and the nomogram prediction in both cohorts, and the decision curve analyses demonstrated great clinical utility of the nomogram. The nomogram is a useful and practical tool for evaluating prognosis and determining appropriate therapy strategies.
Introduction
The pineal gland is an endocrine gland in the midline of the brain that secretes melatonin to modulate circadian rhythms. Primary tumors arising from the pineal gland are termed pineal parenchymal tumors (PPTs). These tumors are rare, comprising <1% of all primary central nervous system neoplasms (1). Their occurrence is more commonly found in children [accounting for 3–8% (2) of all intracranial tumors] than in adults [accounting for 0.1–1.0% (3)]. Furthermore, the different histological types of PPTs exhibit varying growth patterns and histological features. According to the World Health Organization (WHO) classification of tumors of the central nervous system published in 2016 (4), PPTs are divided into four major subgroups: pineocytoma [WHO grade I, International Classification of Diseases for Oncology (ICD-O) 9361/1], PPT of intermediate differentiation (WHO grade II/III, ICD-O 9362/3), pineoblastoma (PB) (WHO grade IV, ICD-O 9362/3), and papillary tumor (WHO grade II/III, ICD-O 9395/3). PB, accounting for 25–50% of PPTs, is mostly observed in children, adolescents, and young adults (5–7). The average age of onset is 12.6 years, with a wide range (1–39 years) (8). However, there is a lower incidence of PB in adults vs. children.
Defined as a highly malignant embryonal tumor of the pineal gland, PB is a type of primary supratentorial middle primitive neuroectodermal tumor of the central nervous system (9). Similar to other malignant tumors, PB is traditionally linked to extremely poor prognosis with aggressive clinical behavior. PB often exhibits a high rate of relapse and propensity for seeding throughout the craniospinal axis (10, 11), as well as sporadic metastases to other parts of the body, such as the calvarial bones (12), vertebrae (13), lung (14), peritoneum (15), mandible (16), and pelvis (17). However, due to the rarity of PB, there is a lack of outcome data on patients with PB. At present, the bulk of information regarding overall survival in patients with PB available in the literature is in the form of case reports (18, 19) and single-institution studies comprising very small numbers of patients (5, 20). Although useful information was, to some extent, provided by these investigations, inadequate data and limitations, such as small sample studies, inherent biases, and insufficient statistical power, made it difficult to deduce reliable outcomes for patients with PB. Parikh et al. (21) concluded that pediatric and adult PB behave differently and should therefore be considered separately when analyzing response to surgical and adjuvant therapy. At present, prognostic factors for the survival of adult patients with PB are not clearly defined.
For the above reasons, we used the data of 213 adult patients with PB from the Surveillance, Epidemiology, and End Results (SEER) database to identify independent prognostic factors of adult patients with PB through Cox proportional hazards regression analysis. Moreover, we compared survival outcomes according to patient and tumor characteristics, as well as treatments received. Additionally, we attempted to establish a nomogram model based on the identified independent prognostic factors to provide a more accurate prediction of patient survival.
Materials and Methods
Data Resource
We acquired the data of patients with PB from the SEER database of the American National Cancer Institute using the latest SEER*Sat version 8.3.6 (https://seer.cancer.gov/), which contained the SEER 18 registries research data.
Inclusion and Exclusion Criteria
The aim of this study is to analyze the prognostic factors of adult patients with PB in a large sample dataset. In order to meet the research needs, inclusion and exclusion criteria were set up as follows. Inclusion criteria: (1)patients were diagnosed as PB between 1975 and 2016 [according to the ICD-O, Third Edition (ICD-O-3) issued by the WHO, ICD-O-3 code 9362/3]; (2) adult patients with PB who were older than 20 years old and younger than 80 at diagnosis; (3) cases with clear treatment information. Exclusion criteria: (1) other types of cancer were excluded according to the ICD-O-3 and Conversion of Neoplasms by Topography and Morphology from the ICD-O-2 to ICD-O-3 edited by the SEER Program National Cancer Institute; (2) patients aged <20 years; (3) patients without clear treatment information (unknown); (4) dead of other causes; (5) there was only one case in some treatment regimens (including the intraoperative beam radiotherapy [RT], RT prior to surgery, RT before and after surgery as well as sequence unknown, but both were given). Finally, 213 adult patients with PB were identified and selected for this analysis (Figure 1).
Variables Selection
The following variables were included: age, sex, race, year of diagnosis, laterality (not including a paired site, right-origin of primary, left-origin of primary, and paired site, but no information concerning laterality), therapy [including RT; chemotherapy [CT]; surgical treatment; only biopsy; RT and CT, sequence unknown; RT after surgery; RT after surgery and CT, sequence unknown; and RT after non-primary surgical procedure and CT, sequence unknown], tumor extension (including invasive tumor confined to gland of origin, localized, not otherwise specified, adjacent connective tissue, adjacent organs/structures, and further contiguous extension; http://web2.facs.org/cstage0205/intracranialgland/IntracranialGland_bfy.html), and tumor size. The time from diagnosis to death or last follow-up was defined as survival time, and those cases lost to follow-up were censored by the SEER. Non-survival included death caused by PB. Furthermore, there were some missing data regarding variable tumor extension and size. Multiple imputation of missing data was performed using the R software (version 3.6.0; http://www.r-project.org/) to ensure a sufficient sample size and boost statistical power (22).
Statistical Analysis
All cases were randomly divided into a primary cohort and a validation cohort at a ratio of 7:3 (23). The predictive model and nomogram were established using the data from the primary cohort; validation of the model was performed through the data of the validation cohort. The procedure for the construction of the predictive model and nomogram was as follows. First, a univariate Cox regression analysis was conducted to explore the possible prognostic risk factors; factors with a p < 0.1 in the univariate analysis were identified as possible risk factors. Second, all possible risk factors and those with a p < 0.05 in the multivariate analysis were identified as independent risk factors. Third, by selecting the factors with a p < 0.1 and given the limitation of the sample size in this study, we established the predictive model based on all risk factors and selected the optimal predictive model according to the results of the Akaike Information Criterion in a stepwise manner (24). Finally, the model was visualized by the nomogram.
The nomogram was validated internally in the primary cohort and externally in the validation cohort, as well as cross-validation in primary and validation cohorts by bootstrapping. The concordance index (C-index) and the time-dependent receiver operating characteristic (ROC) curve were applied to evaluate the accuracy and discriminative ability (25, 26). The association between the actual outcomes and the predicted probability was compared through calibration curves (27). Both discrimination and calibration were evaluated using bootstrapping with 1,000 resamples. Decision curve analysis (DCA) was employed to access the clinical utility of the nomogram (28).
The X-title software (version 3.61; Yale University, New Haven, CT, USA) was used to explore the best cutoff point of the continuous variables (including age, year of diagnosis, and tumor size), based on the log-rank test statistics (29). The optimal cutoff value was defined as the point reflecting the most significant split among the survival distributions of those factors with different categorical ages and tumor sizes. Lastly, the new categorical variables were generated based on the optimal cutoff point and used as predictors in the survival analysis.
All statistics analyses were performed using the R software (version 3.6.0; http://www.r-project.org/), X-title software (version 3.61; Yale University, New Haven, CT, USA), and SPSS (version 25.0; IBM Corporation, Armonk, NY, USA). A p < 0.1 was chosen as the criterion for excluding a variable from the multivariate Cox proportional hazards model, and a p < 0.05 denoted statistical significance for all other tests.
Results
Clinicopathological Characteristics of Patients
A total of 213 patients with PB were finally selected and analyzed in this study. The primary and validation cohorts consisted of 152 and 61 patients, respectively. Baseline information on the patients included in this study is shown in Table 1. Overall, the male-to-female ratio in this analysis was ~1:1. The majority of patients were white (n = 159, 74.6%) and aged 20–50 years (n = 154, 72.3%). In addition, a large proportion of the patients had laterality characteristics without a paired site (n = 207, 97%), and tumor extension characteristics confined to the gland of origin and localized, not otherwise specified (n = 135, 63.3%). About 80% of patients were diagnosed in the period from 2001 to 2016 (n = 169, 79.3%). Importantly, the ratio of cases with confirmed diagnosis by the positive histology were up to 97% (n = 207, 97%).
Univariate Analysis
As shown in Figure 2, the results of the univariate analysis revealed that age, year of diagnosis, therapy (RT after surgery and RT after surgery combined with CT), tumor size, and extent of tumor extension were significantly associated with patient survival. Other factors did not show statistically significant differences. However, taking into account the limitation of the sample size, those factors were not excluded from the multivariate analysis.
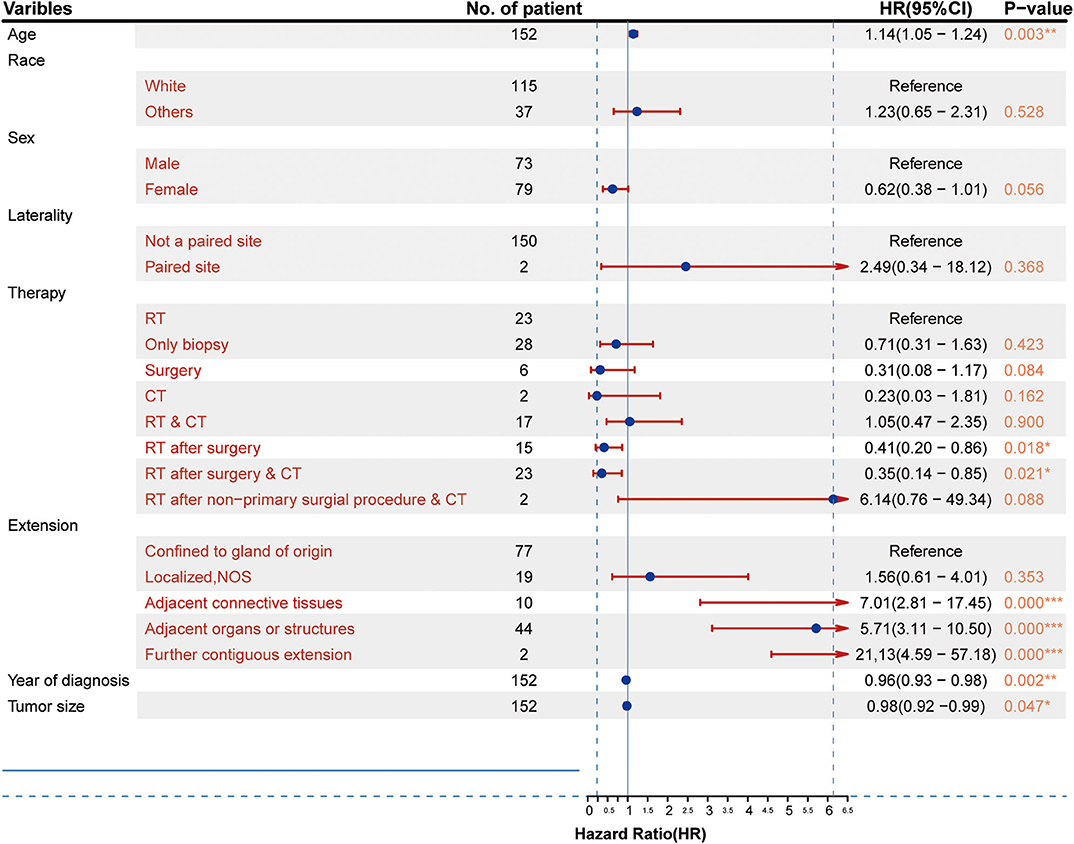
Figure 2. Clinicopathological characteristics of the patients and results of the univariate Cox proportional hazards analysis (HR, 95% confidence interval). *p < 0.05, **p < 0.01, ***p < 0.001.
Independent Prognostic Factors in the Primary Cohort
The results from the multivariate analysis are shown in Figure 3. The results demonstrated that the independent prognostic factors were age [hazard ratio (HR) = 1.15, P < 0.01], therapy strategies (RT after surgery: HR = 0.43, P < 0.05; RT after surgery combined with CT: HR = 0.38, P < 0.05), the scope of tumor extension (extension adjacent tissues: HR = 3.70, P < 0.05; extension adjacent organs and structures: HR = 4.74, P < 0.001; tumor further contiguous extension: HR = 23.31, P < 0.01), tumor size (HR = 0.96, P < 0.01), and year of diagnosis (HR = 0.96, P < 0.05). Sex (female: HR = 0.97, P > 0.1), race (others: HR = 1.14, P > 0.1), and laterality (paired site: HR = 5.44, P > 0.1) were not identified as independent prognostic factors.
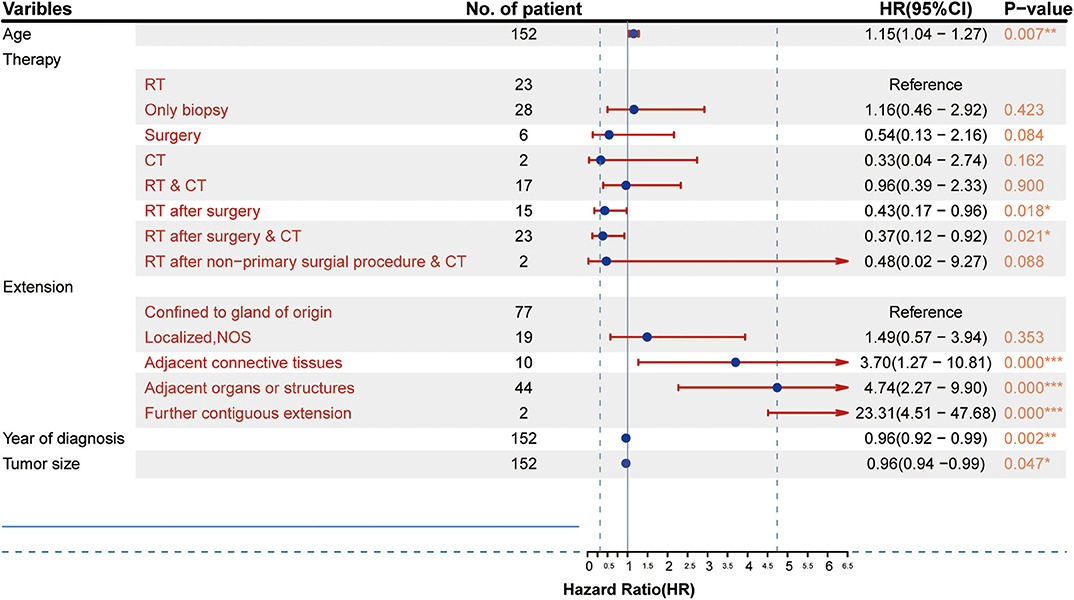
Figure 3. Results of the multivariate analysis of different factors (HR, 95% confidence interval). *p < 0.05, **p < 0.01, ***p < 0.001.
In addition, the effects of all dependent prognostic factors on survival of patients were assessed by survival analysis and shown by the Kaplan–Meier curves (Figure 4).
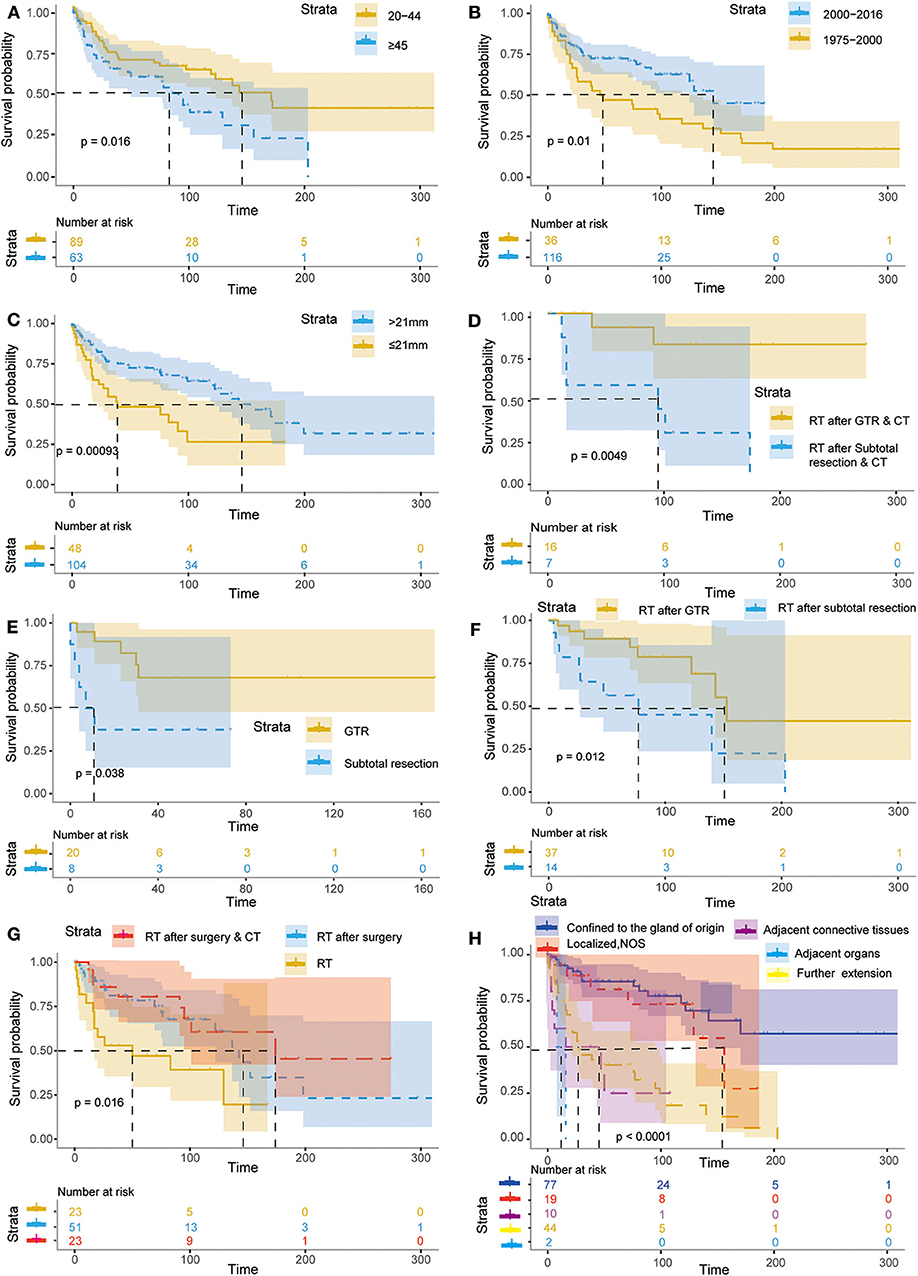
Figure 4. Kaplan–Meier curves for patients with PB according to different independent prognostic factors. The Kaplan–Meier curves for patients with primary PB according to age (A), year of diagnosis (B), tumor size (C), RT after GTR & CT or RT after subtotal resection & CT (D), GTR and subtotal resection (E), RT after GTR or subtotal resection (F), RT, RT after surgery & RT after surgery & CT (G), extent of tumor extension (H).
Prognostic Nomogram of Overall Survival
The prognostic nomogram, shown in Figure 5, was constructed based on the optimization results of the Akaike Information Criterion protocol in the primary cohort. As illustrated in Figure 5, the scores assigned on the points scale could match each level of every variable on the nomogram. Therefore, a total score could be obtained by adding the score from various variables or their levels. Finally, the 36-, 60-, and 120-month cancer-specific survival for each individual patient could be estimated and predicted according to the patient's total score on the nomogram. Of note, in the nomogram, the proportion of extent of disease extension is the largest, while that of treatment is the smallest. These findings revealed that current treatment regimens played a limited role in improving the prognosis of patients with PB, and the extent of tumor extension was still a key factor to the prognosis.
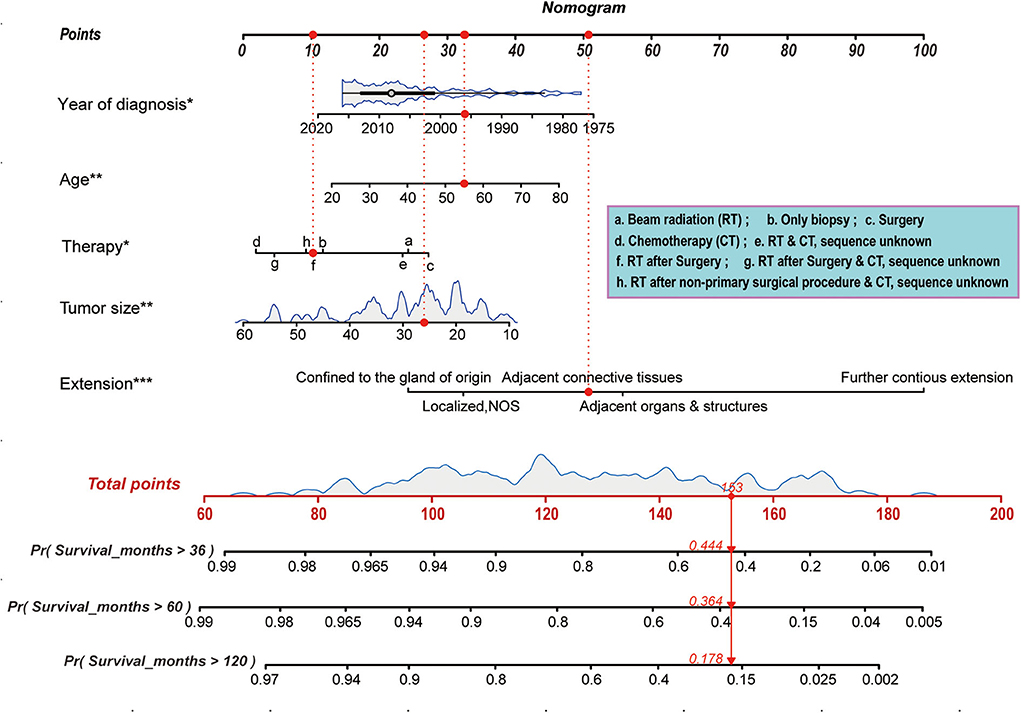
Figure 5. Nomogram predicting 36-, 60-, and 120-month cancer-specific survival for patients with PB. Prognostic factors including age, race, tumor extension, tumor size, and therapy, and the scores assigned on the points scale could match each level of every variable on the nomogram. Thus, a total score was obtained by adding the score from various variables or their levels. Finally, the 36-, 60-, and 120-month cancer-specific survival for each individual patient could be estimated on the basis of the total score. *p < 0.05, **p < 0.01, ***p < 0.001.
Validation and Calibration of the Nomogram
Based on the data of 61 patients in the validation cohort, the C-index of the nomogram for predicting overall survival was 0.802 (95% CI: 0.78–0.83). Additionally, calibration plots of the nomogram showed good consistency between observation and prediction both in the primary (Figures 6A–C) and validation (Figures 6D–F) cohorts for the probability of 36-, 60-, and 120-month survival, as well as the cross-validation in primary and validation cohorts (Figure 6G). Besides, the time-dependent ROC curve was applied to evaluate the discriminative ability of the model. The area under the curve (AUC) value ranging from 0.7 to 0.8 showed the good discriminative ability of this model (Figure 6H). Figure 7 showed the decision curves for the training cohorts to predict the survival probability at 36-, 60-, and 120-month. The blue line represented the net benefit assuming all patients have died, while the green line represented that assuming no patients have died. If the model curve lies in the area between the blue and green lines, it indicates the clinical usefulness of the model. The further away the model curve is from the blue and green lines (that is, the greater the net benefit), the better the clinical value of the nomogram. Of note, great net benefit in the predictive model for almost all of the threshold probabilities at 36- (Figure 7A), 60- (Figure 7B), and 120-month (Figure 7C) was exhibited on the DCA curves. This observation highlighted the potential clinical usefulness of the nomogram.
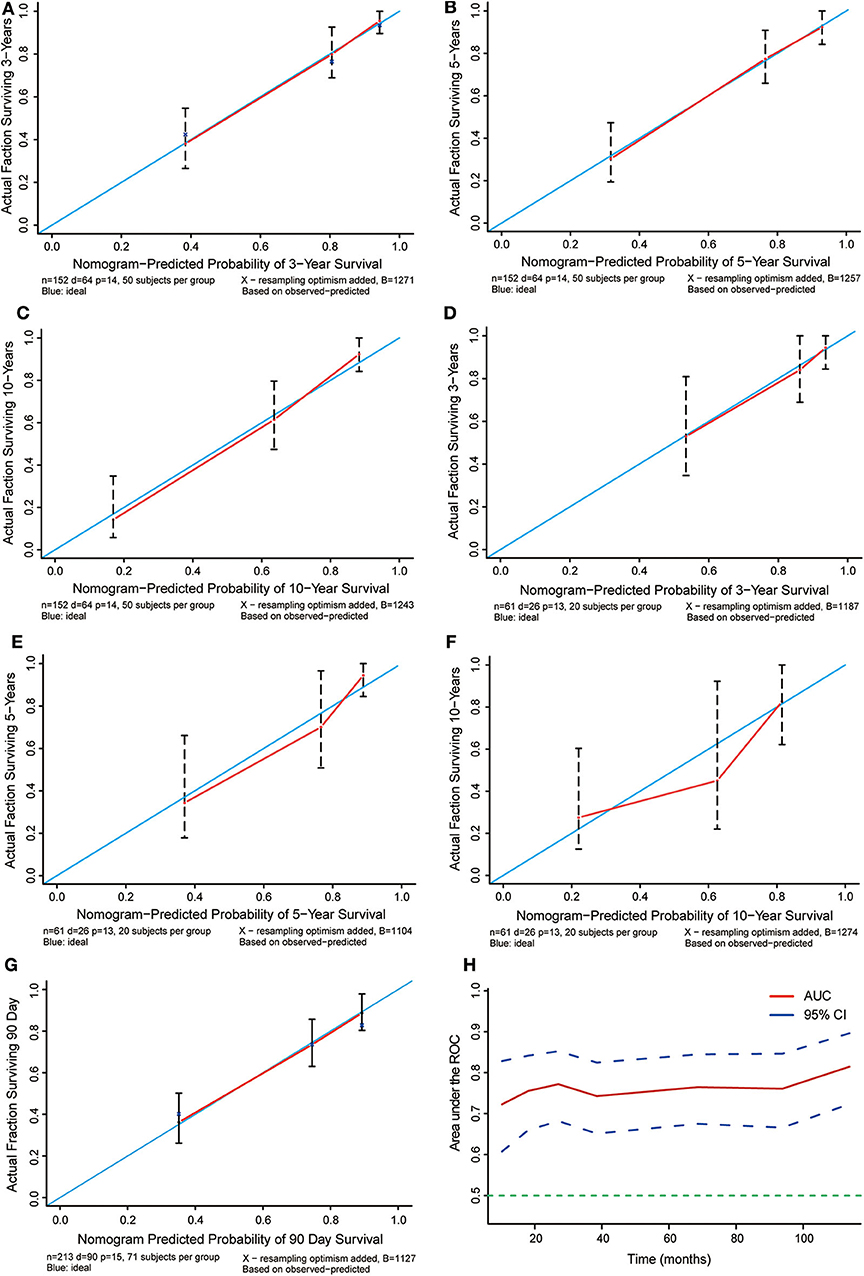
Figure 6. Calibration plots for the prediction of cancer-specific survival at 36-, 60-, and 120-months. (A–C) Calibration curves displaying the probability of 36-, 60-, and 120-month cancer-specific survival between the actual observation and the probability predicted by the nomogram in the primary cohort. (D–F) Calibration curves showing the probability of 36-,60-, and 120-month cancer-specific survival between the actual observation and the probability predicted by the nomogram in the validation cohort. The blue lines with a slope of 1 were ideal for prediction. (G) Cross-validation curves showing the probability of 90 day specific survival between the actual observation and the probability predicted by the nomogram in the validation cohort. The blue lines with a slope of 1 were ideal for prediction. (H) Time-dependent ROC curves showing the sensitivity and specificity of the cancer-specific survival prediction by the nomogram.
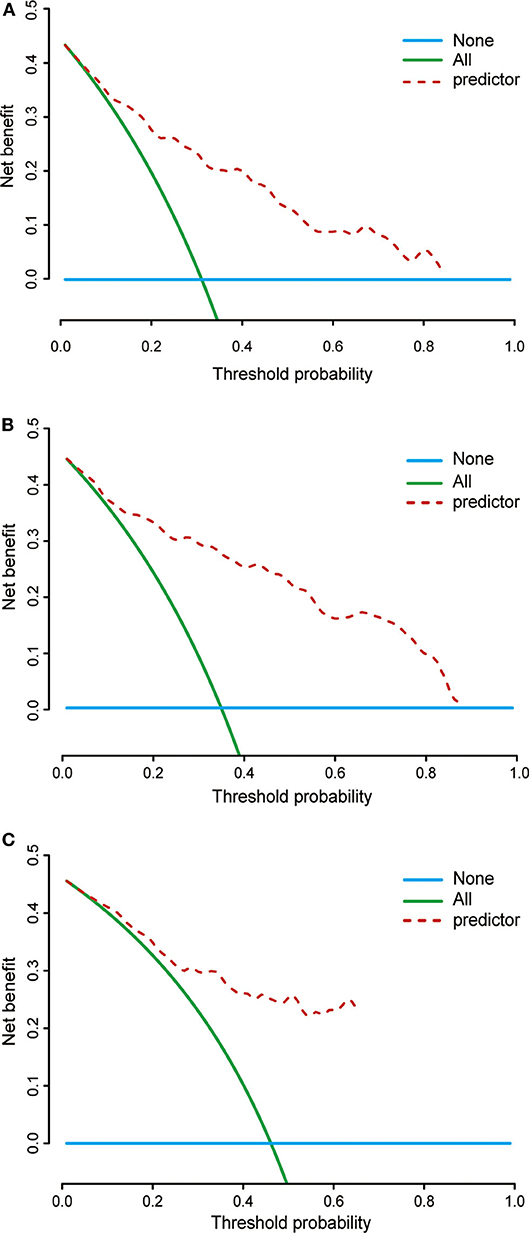
Figure 7. Decision curves of the nomogram predicting 36- (A), 60- (B), and 120-month (C) cancer-specific survival. The x-axis represents the threshold probability, while the y-axis indicates the net benefit calculated by adding the true positives and subtracting the false positives. The horizontal line in parallel with the x-axis assumes that cancer-specific death did not occur in any of the patients, while the solid green line assumes that all patients will have cancer-specific death at a specific threshold probability. The brown dashed line represents the net benefit of using the nomogram.
Comparison of Predictive Accuracy Between the Nomogram and a Single Independent Factor
The weights of extension and year of diagnosis for survival, shown in Figure 5, were higher than those of other factors. We compared the predictive power for the prognosis of patients with PB between the nomogram, scope of tumor extension, and year of diagnosis. C-indices for the prediction of prognosis by the scope of tumor extension and year of diagnosis were 0.72 and 0.63, respectively. These values were significantly lower than the C-index obtained through the nomogram (0.802; P < 0.01).
Discussion
Identification of risk factors affecting patient survival and the effectiveness of currently applied treatments in rare diseases, such as PB, is of vital importance. Therefore, an abundance of high-quality clinical data is critical to achieving this goal. However, the rarity of PB renders the possibility of conducting prospective clinical trials a difficult task. In this study, the information on 213 adult patients with PB, collected by the SEER database from 1975 to 2016, was retrospectively analyzed. To our knowledge, thus far, this study analyzed the largest series of data regarding PB in adults. The results confirmed the predominant impact of the extent of tumor extension, tumor size, year of diagnosis, age, and different treatment regiments (including RT after surgery, and RT after surgery combined with CT) on the outcome of adult patients with PB. Young patients, especially those not receiving RT during their initial treatment, are often associated with extremely poor outcome (6). Interestingly, the results of this analysis revealed that application of beam RT failed to significantly improve the overall survival of adult patients. In addition, the younger, the more poor prognosis in adolescent and pediatric patients with PB. However, interestingly, our analysis found that there is a diametrically opposite situation in adult patients.
Owing to its rarity, there is limited information with regard to the clinical features and outcomes of adult PB. Moreover, the optimal treatment strategies for adult PB remain to be determined. Our primary target was to identify the clinical risk factors related to prognosis and describe their impact of different risk factors, especially treatment options, on the overall survival of patients. Previously developed nomograms have exhibited higher precision than the conventional staging systems for prognosis in some types of cancers (30, 31). Therefore, we attempted to establish a prognostic nomogram for adult PB based on the clinical data of 213 adult patients with PB evaluate its discriminative ability through a calibration plot and time-dependent ROC curve, and estimate its clinical utility by DCA.
Consistent with the literature (32, 33), the results of this study illustrated that tumor contiguous extension was the most dominant risk factor, and overall survival was greatly reduced in patients with PB for whom tumor extension exceeded the adjacent connective tissues, adjacent organs, or structures and continuously extended. The HR for patients where tumor extension had extended to adjacent connective tissues and adjacent organs or structures vs. those with tumors confined to the gland of origin were 3.70 and 4.74, respectively. This finding indicated that the risk of death in patients with tumor extended to adjacent connective tissues was ~3-fold of that calculated for patients with tumors confined to the gland of origin. Likewise, the HR for patients with further continuous extension vs. those with tumors confined to the gland of origin was 23.31. Interestingly, the similar results were exhibited in the results from the survival analysis of this study. This finding is in line with the results obtained from previous studies concerning metastasis of PB (12, 20). Given that only four patients with further continuous extension were included in this study, with an estimation of 95% CI (4.51–47.68) for the HR, we took into account the conclusions of other studies and consider that the results of this analysis are robust. However, the specific values of these results require further investigation.
As for the effect of tumor size on the prognosis of patients, to date, there is still some controversy in the published literature. Dittmar et al. (34) found that a large tumor size was one of the high-risk factors for mortality. Similarly, a study from Tirada et al. (35) illustrated that increased tumor size was an independent prognostic factor of poor outcome. In addition, Huang et al. (36) demonstrated that the risks of recurrence and death gradually increased when tumor size increased. By contrast, some studies suggested that smaller tumor size is associated with poor survival (37, 38). Consistent with those, the results of this study showed that a larger tumor size was an independent prognostic factor of good outcomes (HR = 0.96, P < 0.01), which revealed that for every 1 mm increase in tumor size, the risk of death is reduced by 4%.
Numerous studies demonstrated that the clinical outcome of patients with PB, irrespective of treatment regimens, is worse than that of patients with infratentorial primitive neuro-ectodermal tumors (39–41). It is important to note that surgery remains the first-line therapy in the management of PB, and aggressive surgical resection is recommended (9, 20). In this study, various treatment regimens (i.e., RT, surgical treatment, CT, RT combined with CT, RT after surgery, RT after surgery combined with CT, RT after non-primary surgical procedure combined with CT) were analyzed. The results indicated that these treatment approaches exerted different effects on the prognosis of patients with PB. Consistent with previous studies (42), the results of this study showed that RT after surgery combined with CT was the most dominant therapeutic risk factor, and RT after surgery appears to offer a significant advantage in reducing the risk of death. In detail, the HR for RT after surgery combined with CT vs. beam RT was 0.38, while that for RT after surgery vs. beam RT was 0.47. These findings indicated that the risk of death in patients undergoing RT after surgery combined with CT and those who received the RT after surgery was reduced by 62 and 53%, respectively. Furthermore, the findings were supported by the results of the survival analysis. To date, therapeutic management of adults with PBs is controversial since such few cases exist in the literatures. Multimodality therapy (surgery, RT, and CT) is often attempted and appears to be the optimal approach (43). In the United States, standard treatment of patients with PB currently includes maximal surgical resection followed by adjuvant cranial–spinal irradiation and systemic chemotherapy (43). It was reported that the median progression-free survival rate was 4 months in infants and children under 3 years of age who were treated according to infant brain tumor protocols with intensive chemotherapy alone (33). Surprisingly, Kang et al. (44) in their study found that long-term survival can be achieved for patients who received multimodality treatment, and the results of their study revealed that the median overall survival was 2.3 years, with 2- and 5-year survival rates of 63.6 and 36.4%, respectively, after multimodality treatment. Lee et al. (1) treated 34 adult patients with PB with cranial irradiation therapy and complete surgical resection, and the median survival time was 25.7 months.
Parikh et al. (21) suggested that maximal tumor resection should be the goal for patients aged >5 years with focal disease. Likewise, Mallick et al. (45) showed that patients with subtotal resection and adjuvant RT were often linked to a better survival outcome. In this study, the results of the multivariate analysis could not yet confirm whether surgical treatment alone was the factor affecting prognosis of patients with PB, while survival analysis revealed that the extent of resection is a factor affecting survival of patients with PB. Specifically, comparing to the gross total resection (GTR), RT after GTR, and RT after GTR combined with CT group, survival probability reduced significantly in subtotal resection, RT after subtotal resection, and RT after subtotal resection combined with CT group. In line with our results, another analysis from Tate et al. (20) revealed that a graded increase in survival was observed with increasing degrees of resection (5-year survival rate: 84% for patients who underwent gross total resection vs. 53% for patients who underwent subtotal resection vs. 29% for patients who underwent debulking).
The role of CT in PB remains controversial. According to studies (46, 47), PB appears to be responsive to CT. Nevertheless, the efficacy of CT was not supported by the results of this analysis, which may be related to the scarcity of patients who received CT in this study. Similarly, results from a study revealed that clinical outcomes did not improve after CT in older patients (6). Our analysis also showed that there was no disparity in the average survival time between patients who received CT and those who received RT.
Interestingly, there is also a lack of consensus concerning the effectiveness of RT against PB. Several studies reported that PB was sensitive to RT (40, 48, 49). However, another study supported that PB was radioresistant (50). Based on the results of our analysis, we could not determine whether RT alone was beneficial in the management of PB. In addition, the finiteness of the sample size in the investigation of PB may be the chief cause of this divergence. Consequently, high-caliber clinical trials are urgently warranted to clarify the effectiveness of RT in this setting.
Similar to other studies (51, 52), the results of this analysis revealed that age was a risk factor for the overall survival of patients with PB. Plenty of studies manifested that the younger, the worse the prognosis in pediatric and adolescent patients (20, 21, 53). Nevertheless, a totally opposite trend was presented by the results of this study. In other words, the older, the poorer prognosis in adult patients with PB (HR = 1.15). Addressed concretely, for every 10-year increase in the age of the patient, the risk of death increases by 15%. This may be due to the fact that PB presented the different biological behaviors in adults and juveniles. It is essential to confirm these results in high-quality clinical epidemiological studies involving larger populations.
Several limitations of this study must be acknowledged. First, heterogeneity in the workup, diagnosis, and treatment modalities was unavoidable due to the long period (i.e., >40 years) required to collect the data regarding diagnosis and treatment. Second, data on some critical items reflecting tumor characteristics, such as tumor size and extension, were not available. Although multiple imputations were conducted for missing data during the statistical analysis, the difference between the imputation data and real data was also unavoidable. Third, in the treatment regimens, it was still not known whether the CT regimens were performed before or after surgery. Despite its shortcomings, this analysis has perceptible strengths. Compared with some previous case reports and small-sample analyses, this analysis provided richer information regarding prognostic factors. In addition, inherent biases and heterogeneity were greatly limited, and statistical power was significantly increased. More importantly, the risk factors included in the model are those that are significantly related to PB and markedly easier to acquire than those determined by costly and time-consuming approaches.
In conclusion, five risk factors (i.e., age, year of diagnosis, therapy, extension, and tumor size) were identified in this analysis. Moreover, a nomogram which provided a precise and objective prediction of the prognosis for patients with PB was developed and validated using large-sample data from the SEER database. Importantly, this nomogram is a functional and practical tool that can assist clinicians in evaluating the prognosis of patients and determining appropriate therapeutic strategies. Additional studies are warranted to confirm the clinical value of this model.
Data Availability Statement
Publicly available datasets were analyzed in this study. This data can be found here: https://seer.cancer.gov/data/.
Ethics Statement
All authors signed the SEER Research Data Agreement to protect the privacy of the patients, which is consistent with ethical principles.
Author Contributions
All authors listed have made substantial, direct and intellectual contribution to the work, and approved it for publication.
Funding
This work was supported by the Key Research and Development Project of Shandong (Grant Number 2015GSF118177).
Conflict of Interest
The authors declare that the research was conducted in the absence of any commercial or financial relationships that could be construed as a potential conflict of interest.
Acknowledgments
We are grateful to the SEER database for providing these precious data free of charge to study these rare tumors.
Abbreviations
PB, pineoblastoma; C-index, concordance index; ROC, receiver operating characteristic; DCA, decision curve analysis; AUC, area under the curve; PPT, pineal parenchymal tumor; SEER database, Surveillance, Epidemiology, and End Results database; ICD-O-3, International Classification of Diseases for Oncology, third Edition; WHO, World Health Organization; BRT, beam radiotherapy; CT, chemotherapy; GTR, gross total resection
References
1. Lee JY, Wakabayashi, Yoshida TY. Management and survival of pineoblastoma: an analysis of 34 adults from the brain tumor registry of Japan. Neurol Med Chir. (2005) 45:132–41. doi: 10.2176/nmc.45.132
2. Wilson ER, Takei Y, Bikoff WT, O'Brien MS, Tindall GT, Boehm WM. Abdominal metastases of primary intracranial yolk sac tumors through ventriculoperitoneal shunts: report of three cases. Neurosurgery. (1979) 5:356–64. doi: 10.1227/00006123-197909000-00010
3. Hoffman HJ, Yoshida M, Becker LE, Hendrick EB, Humphreys RP. Pineal region tumors in childhood. Experience at the hospital for sick children. 1983. Pediatric Neurosurg. (1994) 21:91–103. doi: 10.1159/000120821
4. Louis DN, Perry A, Reifenberger G, von Deimling A, Figarella-Branger D, Cavenee WK, et al. The 2016 World Health Organization classification of tumors of the central nervous system: a summary. Acta neuropathologica. (2016) 131:803–20. doi: 10.1007/s00401-016-1545-1
5. Farnia B, Allen PK, Brown PD, Khatua S, Levine NB, Li J, et al. Clinical outcomes and patterns of failure in pineoblastoma: a 30-year, single-institution retrospective review. World Neurosurg. (2014) 82:1232–41. doi: 10.1016/j.wneu.2014.07.010
6. Mynarek M, Pizer B, Dufour C, van Vuurden D, Garami M, Massimino M, et al. Evaluation of age-dependent treatment strategies for children and young adults with pineoblastoma: analysis of pooled European Society for paediatric oncology (SIOP-E) and US head start data. Neuro-Oncol. (2017) 19:576–85. doi: 10.1093/neuonc/now234
7. Tate MC, Rutkowski MJ, Parsa AT. Contemporary management of pineoblastoma. Neurosurg Clin North Am. (2011) 22:409–12. doi: 10.1016/j.nec.2011.05.001
8. Mena H, Rushing EJ, Ribas JL, Delahunt B, McCarthy WF. Tumors of pineal parenchymal cells: a correlation of histological features, including nucleolar organizer regions, with survival in 35 cases. Hum Pathol. (1995) 26:20–30. doi: 10.1016/0046-8177(95)90110-8
9. Tian Y, Liu R, Qin J, Wang J, Ma Z, Gong J, et al. Retrospective analysis of the clinical characteristics, therapeutic aspects, and prognostic factors of 18 cases of childhood pineoblastoma. World Neurosurg. (2018) 116:e162–8. doi: 10.1016/j.wneu.2018.04.135
10. Gaillard F, Jones J. Masses of the pineal region: clinical presentation and radiographic features. Postgraduate Med J. (2010) 86:597–607. doi: 10.1136/pgmj.2009.087460
11. Villa S, Miller RC, Krengli M, Abusaris H, Baumert BG, Servagi-Vernat S, et al. Primary pineal tumors: outcome and prognostic factors–a study from the rare cancer network (RCN). Clin Transl Oncol. (2012) 14:827–34. doi: 10.1007/s12094-012-0869-0
12. Golbin D, Nikitin KV, Konovalov AN, Pitskhelauri DI, Shishkina LV, Golanov AV, et al. Intraosseous metastasizing of pineoblastoma into the anterior skull base, calvarial bones, and vertebrae. Cureus. (2015) 7:e437. doi: 10.7759/cureus.437
13. Charafe-Jauffret E, Lehmann G, Fauchon F, Michiels JF, Paquis P, Maraninchi D, et al. Vertebral metastases from pineoblastoma. Arch Pathol Lab Med. (2001) 125:939–43. doi: 10.1043/0003-9985(2001)125<0939:VMFP>2.0.CO;2
14. Banerjee AK, Kak VK. Pineoblastoma with spontaneous intra and extracranial metastasis. J Pathol. (1974) 114:9–12. doi: 10.1002/path.1711140103
15. Cranston PE, Hatten MT, Smith EE. Metastatic pineoblastoma via a ventriculoperitoneal shunt: CT demonstration. Comput Med Imaging Graphics. (1992) 16:349–51. doi: 10.1016/0895-6111(92)90148-3
16. Zhao P, Strohl A, Gonzalez C, Fishbein T, Rosen-Bronson S, Kallakury B, et al. Donor transmission of pineoblastoma in a two-yr-old male recipient of a multivisceral transplant: a case report. Pediatric Transplant. (2012) 16:E110–4. doi: 10.1111/j.1399-3046.2010.01463.x
17. Fraser G, Rampling R, Smith C, Nicoll J, Stephen M. Long-term survival following extra-neural metastasis from a pineoblastoma. J Neuro oncol. (2000) 48:141–4. doi: 10.1023/A:1006430022068
18. Ghosal N, Furtado SV, Hegde AS. Pinealoblastoma with prominent retinoblastic differentiation: an unusual case in an adult. Neuropathology. (2010) 30:439–42. doi: 10.1111/j.1440-1789.2009.01083.x
19. Gadish T, Tulchinsky H, Deutsch AA, Rabau M. Pinealoblastoma in a patient with familial adenomatous polyposis: variant of Turcot syndrome type 2? Report of a case and review of the literature. Dis Colon Rectum. (2005) 48:2343–6. doi: 10.1007/s10350-005-0201-y
20. Tate M, Sughrue ME, Rutkowski MJ, Kane AJ, Aranda D, McClinton L, et al. The long-term postsurgical prognosis of patients with pineoblastoma. Cancer. (2012) 118:173–9. doi: 10.1002/cncr.26300
21. Parikh KA, Venable GT, Orr BA, Choudhri AF, Boop FA, Gajjar AJ, et al. Pineoblastoma-the experience at St. Jude children's research hospital. Neurosurgery. (2017) 81:120–8. doi: 10.1093/neuros/nyx005
22. Resche-Rigon M, White IR. Multiple imputation by chained equations for systematically and sporadically missing multilevel data. Stat Methods Med Res. (2018) 27:1634–49. doi: 10.1177/0962280216666564
23. Collins GS, Reitsma JB, Altman DG, Moons KG. Transparent Reporting of a multivariable prediction model for Individual Prognosis Or Diagnosis (TRIPOD): the TRIPOD Statement. Br J Surg. (2015) 102:148–58. doi: 10.1002/bjs.9736
24. Harrell FE Jr, Lee KL, Mark DB. Multivariable prognostic models: issues in developing models, evaluating assumptions and adequacy, and measuring and reducing errors. Stat Med. (1996) 15:361–87.
25. Hanley JA, McNeil BJ. The meaning and use of the area under a receiver operating characteristic (ROC) curve. Radiology. (1982) 143:29–36. doi: 10.1148/radiology.143.1.7063747
26. Wolbers M, Koller MT, Witteman JC, Steyerberg EW. Prognostic models with competing risks: methods and application to coronary risk prediction. Epidemiology. (2009) 20:555–61. doi: 10.1097/EDE.0b013e3181a39056
27. Coutant C, Olivier C, Lambaudie E, Fondrinier E, Marchal F, Guillemin F, et al. Comparison of models to predict nonsentinel lymph node status in breast cancer patients with metastatic sentinel lymph nodes: a prospective multicenter study. J Clin Oncol. (2009) 27:2800–8. doi: 10.1200/JCO.2008.19.7418
28. Vickers AJ, Elkin EB. Decision curve analysis: a novel method for evaluating prediction models. Med Decis Making. (2006) 26:565–74. doi: 10.1177/0272989X06295361
29. Camp RL, Dolled-Filhart M, Rimm DL. X-tile: a new bio-informatics tool for biomarker assessment and outcome-based cut-point optimization. Clin Cancer Res. (2004) 10:7252–9. doi: 10.1158/1078-0432.CCR-04-0713
30. Chen S, Liu Y, Yang J, Liu Q, You H, Dong Y, et al. Development and validation of a nomogram for predicting survival in male patients with breast cancer. Front Oncol. (2019) 9:361. doi: 10.3389/fonc.2019.00361
31. Martini A, Cumarasamy S, Beksac AT, Abaza R, Eun DD, Bhandari A, et al. A nomogram to predict significant estimated glomerular filtration rate reduction after robotic partial nephrectomy. Eur Urol. (2018) 74:833–9. doi: 10.1016/j.eururo.2018.08.037
32. Lutterbach J, Fauchon F, Schild SE, Chang SM, Pagenstecher A, Volk B, et al. Malignant pineal parenchymal tumors in adult patients: patterns of care and prognostic factors. Neurosurgery. (2002) 51:44–55. doi: 10.1097/00006123-200207000-00006
33. Jakacki RI, Zeltzer PM, Boyett JM, Albright AL, Allen JC, Geyer JR, et al. Survival and prognostic factors following radiation and/or chemotherapy for primitive neuroectodermal tumors of the pineal region in infants and children: a report of the Childrens Cancer Group. J Clin Oncol. (1995) 13:1377–83. doi: 10.1200/JCO.1995.13.6.1377
34. Dittmar Y, Ardelt M, Scheuerlein H, Rauchfuss F, Dondorf F, Settmacher U. The impact of tumor size on the prognosis of gastric cancer: experiences from a European study group. J Surg. (2016) 3:1082. Available online at: https://austinpublishinggroup.com/surgery/fulltext/ajs-v3-id1082.php
35. Park JY, Kim DY, Kim JH, Kim YM, Kim YT, Nam JH. Outcomes after radical hysterectomy according to tumor size divided by 2-cm interval in patients with early cervical cancer. Ann Oncol. (2011) 22:59–67. doi: 10.1093/annonc/mdq321
36. Huang B, Feng Y, Mo SB, Cai SJ, Huang LY. Smaller tumor size is associated with poor survival in T4b colon cancer. World J Gastroenterol. (2016) 22:6726–35. doi: 10.3748/wjg.v22.i29.6726
37. Han HS, Paik HJ, Ryu JM, Park S, Yi HW, Bae S, et al. Comparison of prognosis and specific features according to tumor size in small-sized breast cancer with extensive lymph node involvement. J Clin Oncol. (2015) 33:81. doi: 10.1200/jco.2015.33.28_suppl.81
38. Tirada N, Aujero M, Khorjekar G, Richards S, Chopra J, Dromi S, et al. Breast cancer tissue markers genomic profiling, and other prognostic factors: a primer for radiologists. Radiographics. (2018) 38:1902–20. doi: 10.1148/rg.2018180047
39. Reddy AT, Janss AJ, Phillips PC, Weiss HL, Packer RJ. Outcome for children with supratentorial primitive neuroectodermal tumors treated with surgery, radiation, and chemotherapy. Cancer. (2000) 88:2189–93. doi: 10.1002/(sici)1097-0142(20000501)88:9<2189::aid-cncr27>3.0.co;2-g
40. Gururangan S, McLaughlin C, Quinn J, Rich J, Reardon D, Halperin EC, et al. High-dose chemotherapy with autologous stem-cell rescue in children and adults with newly diagnosed pineoblastomas. J Clin Oncol. (2003) 21:2187–91. doi: 10.1200/JCO.2003.10.096
41. Gilheeney SW, Saad A, Chi S, Turner C, Ullrich NJ, Goumnerova L, et al. Outcome of pediatric pineoblastoma after surgery, radiation and chemotherapy. J Neuro Oncol. (2008) 89:89–95. doi: 10.1007/s11060-008-9589-2
42. Gerber NU, von Hoff K, Resch A, Ottensmeier H, Kwiecien R, Faldum A, et al. Treatment of children with central nervous system primitive neuroectodermal tumors/pinealoblastomas in the prospective multicentric trial HIT 2000 using hyperfractionated radiation therapy followed by maintenance chemotherapy. Int J Radiat Oncol Biol Phys. (2014) 89:863–71. doi: 10.1016/j.ijrobp.2014.04.017
43. Palled S, Kalavagunta S, Beerappa Gowda J, Umesh K, Aal M, Abdul Razack TP, et al. Tackling a recurrent pinealoblastoma. Case Rep Oncol Med. (2014) 2014:135435. doi: 10.1155/2014/135435
44. Kang YM, Lin SC, Lee YY, Chang FC, Liang ML, Chen HH, et al. Treatment outcomes for pediatric pineoblastoma: a single institute experience in Taiwan. Ther Radiol Oncol. (2018) 2:19–27. doi: 10.21037/tro.2018.07.03
45. Mallick S, Benson R, Rath GK. Patterns of care and survival outcomes in patients with pineal parenchymal tumor of intermediate differentiation: an individual patient data analysis. Radiother Oncol. (2016) 121:204–8. doi: 10.1016/j.radonc.2016.10.025
46. Schild SE, Scheithauer BW, Schomberg PJ, Hook CC, Kelly PJ, Frick L, et al. Pineal parenchymal tumors. Clinical, pathologic, therapeutic aspects. Cancer. (1993) 72:870–80. doi: 10.1002/1097-0142(19930801)72:3<870::aid-cncr2820720336>3.0.co;2-x
47. Ghim TT, Davis P, Seo JJ, Crocker I, O'Brien M, Krawiecki N. Response to neoadjuvant chemotherapy in children with pineoblastoma. Cancer. (1993) 72:1795–800. doi: 10.1002/1097-0142(19930901)72:5<1795::aid-cncr2820720546>3.0.co;2-n
48. Massimino M, Gandola L, Spreafico F, Luksch R, Collini P, Giangaspero F, et al. Supratentorial primitive neuroectodermal tumors (S-PNET) in children: a prospective experience with adjuvant intensive chemotherapy and hyperfractionated accelerated radiotherapy. Int J Radiat Oncol biol Phys. (2006) 64:1031–7. doi: 10.1016/j.ijrobp.2005.09.026
49. Timmermann B, Kortmann RD, Kuhl J, Meisner C, Dieckmann K, Pietsch T, et al. Role of radiotherapy in the treatment of supratentorial primitive neuroectodermal tumors in childhood: results of the prospective German brain tumor trials HIT 88/89 and 91. J Clin Oncol. (2002) 20:842–9. doi: 10.1200/JCO.2002.20.3.842
50. Clark AJ, Sughrue ME, Ivan ME, Aranda D, Rutkowski MJ, Kane AJ, et al. Factors influencing overall survival rates for patients with pineocytoma. J Neuro Oncol. (2010) 100:255–60. doi: 10.1007/s11060-010-0189-6
51. Tsumanuma I, Tanaka R, Washiyama K. Clinicopathological study of pineal parenchymal tumors: correlation between histopathological features, proliferative potential, and prognosis. Brain Tumor Pathol. (1999) 16:61–8. doi: 10.1007/BF02478904
52. Fauchon F, Jouvet A, Paquis P, Saint-Pierre G, Mottolese C, Ben Hassel M, et al. Parenchymal pineal tumors: a clinicopathological study of 76 cases. Int J Radiat Oncol Biol Phys. (2000) 46:959–68. doi: 10.1016/S0360-3016(99)00389-2
Keywords: pineoblastoma, nomogram, prognosis, risk factor, C-index
Citation: Jing Y, Deng W, Zhang H, Jiang Y, Dong Z, Fan F and Sun P (2020) Development and Validation of a Prognostic Nomogram to Predict Cancer-Specific Survival in Adult Patients With Pineoblastoma. Front. Oncol. 10:1021. doi: 10.3389/fonc.2020.01021
Received: 04 March 2020; Accepted: 22 May 2020;
Published: 24 July 2020.
Edited by:
Theodore Nicolaides, New York University, United StatesReviewed by:
Jian Zhou, Fudan University, ChinaRobin Arthur Buerki, University Hospitals Cleveland Medical Center, United States
Copyright © 2020 Jing, Deng, Zhang, Jiang, Dong, Fan and Sun. This is an open-access article distributed under the terms of the Creative Commons Attribution License (CC BY). The use, distribution or reproduction in other forums is permitted, provided the original author(s) and the copyright owner(s) are credited and that the original publication in this journal is cited, in accordance with accepted academic practice. No use, distribution or reproduction is permitted which does not comply with these terms.
*Correspondence: Fan Fan, ffan@umc.edu; Peng Sun, sunpeng@qdu.edu.cn