- 1Department of Biochemistry and Molecular Biology, Institute of Glycobiology, Dalian Medical University, Dalian, China
- 2Department of Urology, First Affiliated Hospital of Dalian Medical University, Dalian, China
Bladder cancer is one of the most common malignant tumors of the urogenital system with high morbidity and mortality worldwide. Early diagnosis and personalized treatment are the keys to successful bladder cancer treatment. Due to high postoperative recurrence rates and poor prognosis, it is urgent to find suitable therapeutic targets and biomarkers. Glycans are one of the four biological macromolecules in the cells of an organism, along with proteins, nucleic acids, and lipids. Glycans play important roles in nascent peptide chain folding, protein processing, and translation, cell-to-cell adhesion, receptor-ligand recognition, and binding and cell signaling. Glycans are mainly divided into N-glycans, O-glycans, proteoglycans, and glycosphingolipids. The focus of this review is the discussion of glycans related to bladder cancer. Additionally, this review also addresses the clinical value of glycans in the diagnosis and treatment of bladder cancer. Abnormal glycans are likely to be potential biomarkers for bladder cancer.
Introduction
Bladder cancer is a malignant tumor of the urogenital system, which can be divided into bladder urothelial carcinoma, bladder squamous cell carcinoma, bladder adenocarcinoma, small cell carcinoma of bladder, and so on. The most common type of bladder cancer is bladder urothelial carcinoma. Smoking is considered to be the main risk factor of bladder cancer, and its incidence increases with age. There are significant gender differences in the incidence and prognosis of bladder cancer have obvious gender differences. The incidence of bladder cancer ranks fourth and eleventh among men and women with cancer, respectively (1). Early diagnosis and specific treatments are effective means of treating bladder cancer (2). Great progress has been made in the study of bladder cancer in various fields, but there are still has problems such as poor prognosis, serious complications, and high recurrence rate (3). Therefore, searching for specific molecular targets and treatment methods for bladder cancer is a hot spot of current research.
Polysaccharides are one of the basic components of animal and plant cells. They can be divided into N-glycans, O-glycans, glycosaminoglycans, and glycosphingolipids according to the different ways of combining sugar chains. Glycans play an important role in cell-to-cell interactions and signal transduction. Abnormal sugar chains often play important roles in cancer biology as tumor markers. Glycans bind to proteins, lipids, and other glycosyl groups through glycosylation, a common post-translational modification process (4). As a key regulatory mechanism, glycosylation regulates the physiological, and pathological processes of some cells. Abnormal glycosylation is a common phenomenon that occurs in cancer cells, and it can determines the stage, direction, and fate of tumor progression (5).
Compared with normal tissues, there are many changes in glycans in cancer cells, including fucosylation, sialylation, truncation of O-glycans, and increased branching of O-glycans (6). Abnormal glycoproteins are involved in regulating tumor cell proliferation and adhesion-related signaling pathways. The main cause for these phenomena is abnormal overexpression of glycosyltransferases or glycosidases (7). Glycans on the cell surface can be used as targets for the treatment of cancer, but due to heterogeneity and complexity of the sugar chain structure, it is still a problem for anaylzing the detailed sugar chain structure (8). This review summarizes the research progress of some abnormal glycans in bladder cancer, and details their biological functions in bladder cancer according to the types of glycans.
N-glycan
N-glycans are classified into high mannose type, complex type, and heterozygous type according to their structure. They all have a common pentasaccharide core, and their synthesis is catalyzed by specific glycosyltransferase or glycosidase. Abnormal glycosylation catalyzed by glycosyltransferases and glycosidases is often closely related to tumorigenesis, development, and metastasis (9). Glycosylation is one of the most common post-translational modifications of proteins. It is a process of transferring glycosyl group to the specific amino acid residues on proteins to form glycosidic bonds under the action of glycosyltransferase. In human tumors, cell surface growth factors and lectins play important roles in the transformation and development of tumors (10, 11). Studies have shown that when epithelial-mesenchymal transition (EMT) occurs in non-malignant bladder transitional epithelial HCV29 cells induced by transforming growth factor-β (TGFβ), the expression of hybrid-type glycans increases, while the expression of complex-type glycans decreases. Transcription of the fuca1 gene encoding Type 1 α-L-fucosidase was suppressed in bladder cells with EMT, which led to increased levels of fucosylated N-glycans (12). In addition, the change of specific N-glycans on the cell surface combined with EMT contributes to cell migration (13). This indicates that when EMT occurs in bladder cells, the levels of N-glycosylation changes, which in turn promotes tumor proliferation and metastasis. Therefore, further discovering and studying of the changes in the structure and function of N-glycans related to bladder tumors can better assess the development of bladder cancer, which will have important significance for the diagnosis, treatment, and prognosis of bladder cancer.
Fucosylation
Fucosylation is a process in which GDP-fucose is used as a donor to transfer glycosyl to proteins or lipids under the catalysis of fucosyltransferase, which is often involved in cell differentiation, development, and malignant transformation. According to the location of fucose, fucosylation can be divided into core fucosylation (α-1,6 fucosylation) and terminal fucosylation (α-1,2 and α-1,3/4 Fucosylation). There are currently 13 known fucosyltransferases involved in fucosylation, of which fut8 is the only transferase that catalyzes core fucosylation, fut1, and fut2 are involved in α1–2 linked fucose synthesis, fut3–9 participate in the synthesis of α1–3 and α1–4 linked fucose (14). Calreticulin can regulate the content of Fut1 in bladder cancer tissue. Modification of β1 integrin with α1,2 fucosylation can regulate cell adhesion and metastasis of bladder cancer cells when the expression levels of fut1 were upregulated (15). In tumor tissue, overexpression of fut4 transferring GDP-fucose to the Lewis Y antibody terminal N-GlcNac with the 1,3-linkage, which promoting neoplastic cell proliferation (16). MiR-125a-5p can inhibits cell proliferation and induce apoptosis, and reverse the EMT process of bladder cancer cells by targeting fut4, thereby, inhibiting tumor cell metastasis (17). Studies have found that expression levels of complex fucosylated N-glycan was abnormal in bladder cancer tissues (including core fucosylated N-glycans levels increased and terminal fucosylated N-glycan levels decreased), and the core fucose expression level was positively correlated with tumor tissue grade (18). Therefore, changes in intracellular fucose levels may be closely related to the progress of bladder cancer, but the specific molecular mechanisms need to be further explored.
Sialylation
Sialic acid is a nine-carbon monosaccharide with negatively charge, and exists on the surface of cells and the outermost ends of most vertebrate glycoproteins and glycolipid molecules. It participates in molecular recognition and adhesion processes, and it is an important information transfer molecule in the organisms. Free sialic acid is catalyzed by CMP-Sia synthase in the presence of CTP to generate donor CMP-Sia. Under the catalysis of sialyltransferase, donor CMP-Sia is attached to the sugar complex (N-glycans, O-glycans, and glycolipids) via a α2,3, α2,6, α2,8 linkage.
Abnormal glycosylation can often be found in tumor cells. One of the important changes is the alteration of sialylated glycans. The appearance of abnormal sialylated glycans is often accompanied by tumor occurrence, development, invasion, and metastasis. Abnormal sialylation is regulated by sialyltransferase and sialidase levels. Glycans related to human bladder cancer have been discovered as follows. The blood group antigen Lewis X (LeX) has been considered as a biomarker for urothelial cancer. It is usually not found in normal urothelial cells in adults, but is expressed in transitional cell tumors, and has nothing to do with the stage and grade of the tumor (19). α-2,3-linked sialyltransferases ST3Gal III, ST3Gal IV, and ST3Gal VI are key enzymes that mediate sialyl Lewis A and sialyl Lewis X synthesis. Sialyl Lewis A (sLeA, also known as CA19-9) and sialyl Lewis X (sLeX) play important roles in cancer progression. The clinical usefulness of monitoring CA19-9 in urothelial carcinoma is less commonly described. Monitoring the level of CA19-9 in urine may help diagnose bladder urothelial carcinoma (20). On the other hand, serum CA19-9 is almost always elevated in metastatic and spread tumors (21). However, it comes into view that the role of CA19-9 as a serum marker in urothelial cancers has not yet been defined. SLeX plays a key role in the selection of selectins and is involved in the spread of tumor cells (22). It is reported that the expression of sialylated Lewis X antigen is closely related to the invasiveness and metastatic potential of bladder cancer (23). Another sialylated glycan in bladder cancer is sTn, which is usually produced by overexpression of ST6GALNAC1 sialyltransferase. ST6GALNAC1 transfers sialic acid to the O atom at the sixth position of the N-acetylgalactosamine residue linked to a serine or threonine (GalNAc α-O-Ser/Thr) on specific proteins, thereby preventing extension of O-glycosidic chains in glycoproteins. Therefore, sTn is a truncated abnormal O-glycan, which often appears in tumor mucin. In high grade bladder cancer tissues, the significant overexpression of sTn antigen is associated with increased expression of ST6GALNAC1 sialyltransferase and down-regulates anti-cancer immune response through different mechanisms (24). Generally, polysialic acid (PSA) is formed when sialic acid is linked to another sialic acid residue through an α-2,8 glycosidic bond. PSA can regulate the interactions between cells and cells, cells and cell matrix, and cell surface molecules. PSA can promote cancer metastasis and participate in nervous system development. In summary, the change in the content of sialylated glycans is directly related to the development of bladder cancer, and the sialyltransferases that catalyze the production of glycans play very important roles in it, which provides clues for us to understand the mechanism of tumor development.
β1–6-Branching GlcNAc
N-acetylglucosaminotransferase V (GnT-V) alters the structure of specific N-glycans by modifying α1–6-linked mannose with the β1–6-linked N-acetylglucosamine (Figure 1). Increased β1–6 GlcNAc branch expression was observed in many cancers, and was closely related to malignant tumor metastasis and poor prognosis (25, 26). The formation of β1–6 branches on cell surface receptors accelerates cancer metastasis (27). And GnT-V expression is related to tumor stage or grade. The main reason affecting this biological behavior may be that GnT-V can affect downstream molecular signal transduction by changing the β1–6 branches of certain glycoproteins on the cell surface, such as cadherin, integrin, and cell surface growth factor receptors (GFR) (28, 29). It has been reported that in superficial bladder cancer, the expression of GnT-V is detected by immunohistochemistry, showing that its expression negatively correlated with tumor grade and stage (30). The incidence of GnT-V positive expression in bladder cancer is significantly higher in lower grade/less invasive cancer than in higher grade/higher invasive cancer, which indicates that immunohistochemical detection of GnT-V can be used as a reliable marker for predicting the recurrence of superficial bladder cancer. Therefore, we concluded that GnT-V and its catalytically formed β1–6GlcNAc branch N-glycan are closely related to tumor cell adhesion, invasion, and metastasis. Together, better understanding the relationship between GnT-V and tumor stage, grade, and prognosis will help clinical early diagnosis of the disease and evaluation of treatment effects.
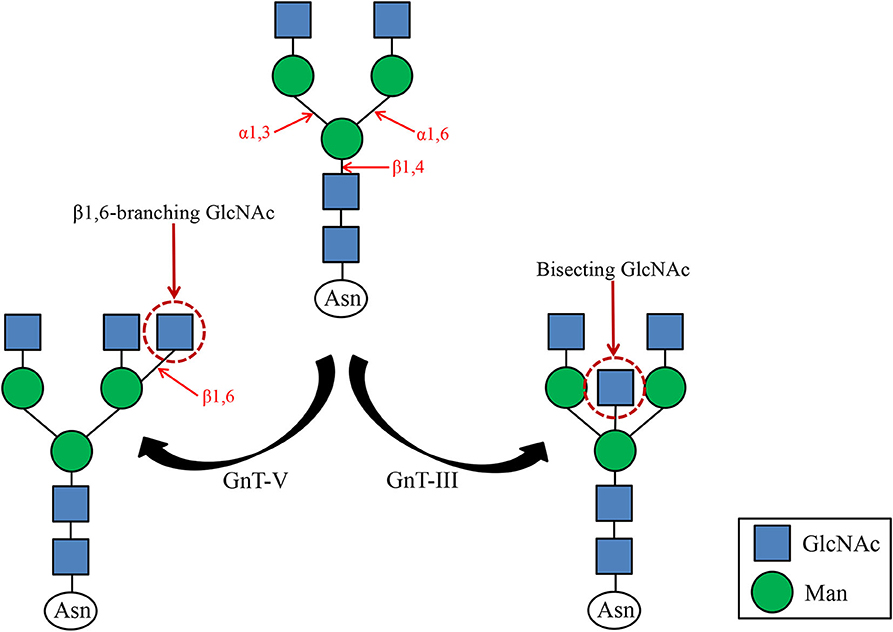
Figure 1. GnT-III and GnT-V catalyzed glycosylation reactions. GnT-III catalyzes the formation of bisecting GlcNAc and regulates tumor cell metastasis. GnT-V catalyzes the formation of β1,6 branched N-glycans, a structure commonly found in tumor cells.
Bisecting GlcNAc
N-acetylglucosamine transferase III (GnT-III) transfers N-acetylglucosamine (GlcNAc) from UDP-GlcNAc to the core mannose of N-glycan to form a β1,4 glycosidic bond, forming a bisecting GlcNAc (Figure 1). Since GnT-III and GnT-V act on the same substrate, and the bisecting GlcNAc prevents GnT-V from approaching the dual antenna substrate, it is speculated that GnT-III is an antagonist of GnT-V. For the same target protein integrin α3β1, opposite effects of GnT-III and GnT-V have been observed (31). GnT-V promotes α3β1 integrin-mediated cell migration, while GnT-III overexpression inhibits GnT-V-induced cell migration, proving that GnT-III and GnT-V can competitively modify the same glycoprotein, thereby further regulate their biological functions positively or negatively. Previous studies have shown that the expression of GnT-III and the emergence of GlcNAc can promote tumor migration and invasion, such as ovarian cancer (32). However, in some tumors, E-cadherin can up-regulate GnT-III level, forming a positive feedback loop between the two to promote cell adhesion (33). The reason is that GnT-III overexpression makes the retention time of E-cadherin on the cell surface prolonged, which leads to enhanced intercellular adhesion. Therefore, overexpression of GlcNAc can suppress tumor metastasis. It can be seen that GnT-III catalyzes the formation of bisecting GlcNAc and no longer forms branches, which affects the function of cell surface adhesion molecules and then the biological function of tumors. It has been reported that the structure and content of N-glycans on urinary fibronectin (Fn) in bladder cancer patients have changed significantly, especially the increase in bisecting GlcNAc content (34). However, there are few studies about the effect of bisecting GlcNAc on the biological behavior of bladder cancer, and the specific molecular mechanism needs to be further explored.
O-glycan
There are different core structures between O-glycans and N-glycans, but biologically active oligosaccharides are usually found on the outer chain attached to the core. Previous studies have found that O-glycan is involved in tumor metastasis. Interestingly, O-glycans show dual roles in tumor metastasis depending on their structure (35). The lack of the core 2 structure on human leukocyte antigen class I (HLA class I) O-glycans and the impairment of galectin-glycan lattice formation allow bladder cancer cells to escape cytotoxic T lymphocytes (CLTs) anti-tumor immunity (36). Mucin 1 (MUC1) on the surface of bladder cancer cells carrying core 2 O-glycans acts as a molecular barrier to resist the attack of natural killer (NK) cells, thereby promoting bladder tumor metastasis (37). Core 2 O-glycans is catalyzed by β-1,6-N-acetylglucosaminyltransferase (core 2 β-1,6 GlcNAc transferase or C2GnT), and found that C2GnT expression is positively correlated with urinary system tumors such as bladder cancer (38, 39). These results suggest that C2GnT expression promotes tumor metastasis. In contrast to C2GnT, core 3 O-glycan catalyzed by Core 3 synthase inhibits tumor metastasis by regulating integrin-mediated signaling (40). In summary, O-glycans with different structures play different roles in tumor metastasis. Therefore, we should thoroughly explore the specific molecular mechanism of the effect of O-glycan structure and corresponding glycosyltransferase on bladder tumor metastasis.
O-GalNAc Glycans
Many glycoproteins carry glycosyl groups induced by GalNAc, which are attached to the hydroxyl groups of serine or threonine residues. O-GalNAc glycans, also known as mucin-type O-glycans. The sugars found in O-GalNAc glycans include GalNAc, Gal, GlcNAc, Fuc, and Sia, while Man, Glc, or Xyl residues are absent. O-GalNAc glycans related to malignant tumors are generally incompletely synthesized O-glycans such as T antigen, Tn antigen, and their corresponding sialic acid variants (sT antigen and sTn antigen). The first step in the start of O-glycosylation is that N-acetylgalactosamine (GalNAc) is connected to the serine/threonine (Ser/Thr) residues of the polypeptide chain to form the Tn antigen. It is further added with galactose (Gal) to the three hydroxyl position to expand to core type 1 (T antigen), and then N-acetylglucosamine (GlcNAc) is added to the three or six hydroxyl position to form core type II and type III, and then sialylated as terminal modification, forming sTn antigen, sT antigen, and so on (Figure 2).
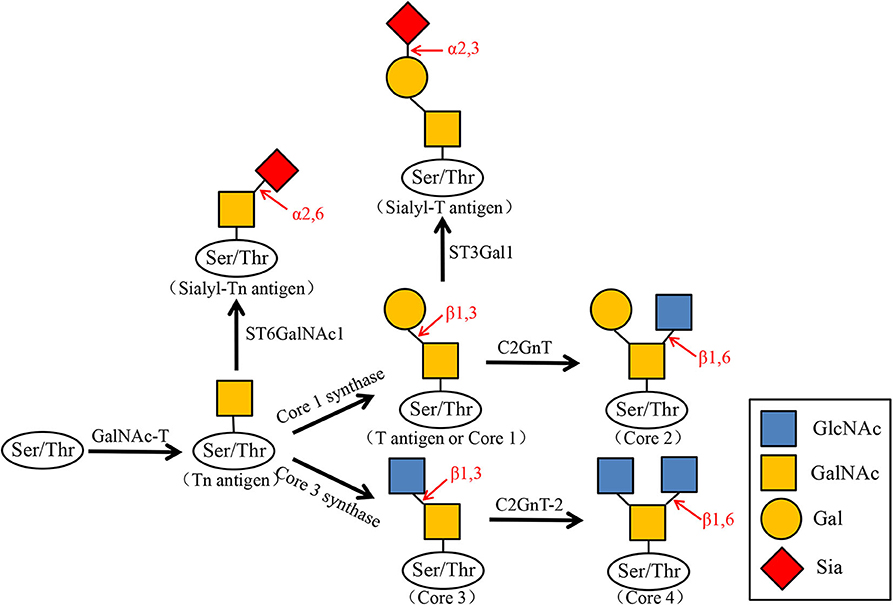
Figure 2. Biosynthesis of O-GalNAc glycans and their sialylated glycans. N-acetylgalactosamine (GalNAc) is transferred to the serine (Ser) or threonine (Thr) residues of the polypeptide by GalNAc transferase (GalNAc-T). GalNAcα1-Ser/Thr (Tn antigen) is converted to Galβ1–3GalNAcα1-Ser/Thr (T antigen or Core1) by Core1 synthase. Core1 is then converted by C2GnT to Core2, and the Tn antigen can also be converted to Core3 by Core3 synthase. Core3 is converted by C2GnT-2 to Core4. At the same time, Tn antigen is transformed into Sialyl-Tn antigen by ST6GalNAc1, and Core1 is transformed into Sialyl-T antigen by ST3Gal1.
It has been reported that the T antigen system and mean nuclear volume have been proposed as risk variables for bladder tumors. There is a correlation between the mean nuclear volume and the expression of the T antigen system in bladder cancer tumors of different stages and grades, and the primary tumor high average nuclear volume is associated with tumor invasion (41). We can speculate the clinical course and invasion of the tumor by measuring the amount of the T antigen system. The appearance of sTn antigen is generally inextricably linked to the early occurrence of tumors, and it is expressed in bladder cancer (42), ovarian cancer (43), colon cancer (44), lung cancer (45), gastric cancer (46), and prostate cancer (47). An α2,6 sialyl acyltransferase ST6GALNAC1 was found to be overexpressed in bladder cancer tissues with higher malignancy, and this change is related to the sTn antigen, which is also explained above. Recently, a new anti-STn monoclonal antibody named L2A5 was developed. L2A5 can specifically bind to the sialylated structure on the surface of bladder cancer cell lines expressing STn, which can effectively target and block cancer-related antigens (48). It shows that antibody-based bladder cancer treatment has a good application prospect. Since elevated Tn, sialyl-Tn, and T antigen levels are associated with bladder cancer, O-GalNAc glycans can be used as potential markers for cancer diagnosis and prognosis.
O-GlcNAcytion
O-GlcNac glycosylation is a progression in which GlcNac covalently bind to the ser/Thr hydroxyl group on a protein under the catalysis of OGT (O-linked GlcNAC transferase), and which can be eliminated by OGA (O-GlcNac glycosidase). O-GlcNac modification plays an important role in transcription regulation, signal transduction, and protein degradation (49). In addition, elevated O-GlcNac levels are closely related to tumor cell activity. For example, in tumor cells, overexpression of OGT adds O-GlcNac to Thr-352 of NF-κB p65, this process can decreases binding to IκBα, so that NF-κB can play its role of transcription factors through entering the nucleus, which leads to cell division defects and cloning (50). Bladder cancer cells prematurely terminate the O-glycosylation of proteins, allowing short-chain O-GlcNac glycans to accumulate on the cell surface, which can reduce the adhesion of tumor cells, thereby increasing their metastatic and invasive properties (51). Studies have found that OGT in urine is significantly different between early bladder cancer and advanced bladder cancer, so the content of OGT can be analyzed to classify bladder cancer (52). Chronic inflammation caused by some infection or anticancer drugs are important risk factors for bladder cancer (53), therefore, it can be concluded that abnormal O-GlcNac glycosylation may be related to the occurrence and development of bladder cancer. In summary, studies have shown that analysis of O-GlcNAcylation or OGT expression may be useful for the diagnosis of bladder cancer, and OGT may be used as a potential target for bladder cancer therapy in the future.
Proteoglycan
Proteoglycans are composed of core proteins and glycosaminoglycans [GAG, for example, hyaluronic acid (HA), chondroitin sulfate (CS), dermatan sulfate (DS), heparin sulfate (HS), heparin (HP), and sulfuric acid Keratin (KS)] with covalent bonds. The structure of proteoglycan is different from glycoprotein. Proteoglycan is a key biologically active molecule that can directly affect tumor development and controls its development to varying degrees. Studies have revealed that the expression of Biglycan (BGN) is related to high grade human bladder tumor, and a series of experiments have proved that BGN is an inhibitor of bladder tumor cell growth and proliferation (54). Decorin is also a versatile small leucine-rich extracellular matrix proteoglycan that has been shown to have potent antitumor activity (55, 56). Studies have found that human bladder cancer cells do not express decorin in vivo or in vitro, and that decorin expression is reduced in malignant human bladder tissue samples (57). And the destruction of the decorin will not lead to the spontaneous development of the tumor, but the absence of the decorin will allow the tumor to form (58). Therefore, both biglycan and decorin have inhibitory effects, but they are regulated differently in bladder cancer, the mechanisms of blocking decorin expression in human bladder cancer cells need further research and clarification by scientists, and this will provide the possibility to detect decorin as a bladder cancer treatment tool.
Glycosaminoglycan (GAG), also known as mucopolysaccharide, aminopolysaccharide, and acid polysaccharide, is a repeating disaccharide unit composed of hexuronic acid and hexosamine. As mentioned above, there are six types of important glycosaminoglycans in the human body. GAG is involved in many important physiological and pathological processes, and plays a vital role in cell-cell interactions. The glycosaminoglycan layer present on the bladder surface can non-specifically prevent bacteria, ions, and molecules from adhering to the epithelium. In addition, studies have observed that chondroitin sulfate (CS) has a growth inhibitory effect on human bladder cancer cell line HT-1376, and the combined use with chemotherapy drugs CS, gemcitabine (GEM), or mitomycin-C (MMC) can make the cell growth inhibition more obvious, but the exact molecular mechanisms need to be further explored (59).
Hyaluronic acid (HA) is a non-sulfated glycosaminoglycan, a repeating unit consisting of disaccharides of D-glucuronic acid and N-acetylglucosamine (60). HA synthase (HAS1, HAS2, HAS3), HA receptor, and HYAL1 in the HA family play a role in tumorigenesis and development. HA can help tumor cells overcome contact inhibition and have protective effects on immune surveillance to a certain extent (61). Hyaluronic acid synthesis is regulated by glycogen debranching enzyme. We found that the loss of amylo-α-1–6-glucosidase-4-α-glucosyltransferase (AGL) drives rapid proliferation of bladder cancer cells by upregulating HA synthase (HAS2) mediated HA synthesis (62, 63). Studies have shown that the overexpresseion of HAS1 in bladder cancer cells can cause an increase in the CD44 variant isoforms (HA receptor), thereby inhibite Fas mediated apoptosis (64), and promoting the growth and invasion of bladder cancer cells. At the same time, the expression of CD44 is directly proportional to the aggressiveness of bladder tumor cells (65). HYAL1 and tumor-associated HA/HAase system can promote tumor growth, invasion and metastasis (66–68). The accuracy of HA and HAase in the diagnosis of bladder cancer is more than 90%, which is higher than that of HA and HAase alone. Therefore, HA/HAase can be used as a biomarker for diagnosis of bladder cancer (69, 70). It can be seen that the determination of HA/HAase is of great significance in the diagnosis and prognosis of bladder cancer (71), and the hyaluronic acid family can be targeted for cancer treatment.
Glycosphingolipid
Glycosphingolipids (GSLs) are glycoside compounds where ceramide is glycosylated. In general, GSL was found to interact with key molecules in a specific membrane microdomain called a “glycosynapse,” mediating cell adhesion and signal transduction (72). Regarding how the sugar synaptic microdomains regulate the phenotype of tumor cells, some studies have shown that this is achieved by altering cell growth, adhesion, and movement (73). Sialic acid-containing acidic glycosphingolipids are called gangliosides. It is reported that gangliosides, especially GM2 and GM3, can interact with GFR and inhibit growth factor-induced signal transduction (74). Studies have shown that GM3 is highly expressed in human benign, non-invasive tumor KK47 cells, but GM3 levels are very low in highly malignant, invasive bladder cancer YTS1 cells. High GM3 levels reduce tumor cell motility/invasiveness, while low GM3 levels enhance tumor cell motility/invasiveness (75). These indicate the expression levels of GM3 are negatively correlated with the migration, invasion ability, and malignancy of bladder cancer cells. Therefore, it is of great significance to study the effect of GM3 expression on the biological behavior of bladder tumor cells.
Conclusion and Perspectives
This article reviews the structure and function of glycans related to bladder cancer and the glycosyltransferases that catalyze glycan formation, and the roles of glycans on the biological functions of bladder cancer are summarized (Table 1). In recent years, people have paid more and more attention to the key role of glycans in cancer progression. The appearance of tumors is often accompanied by abnormal glycosylation, which determines the direction of tumor progression. Fucosylation and sialylation and other glycosylations affect the function of glycans, which is closely related to the abnormal expression of glycosyltransferase, or glycosidase. We know that the occurrence, development, proliferation, invasion, and metastasis of bladder cancer are inextricably linked to changes in glycosylation in cancer cells. However, due to the large differences in the molecular weight of the sugar chains formed by glycans, the complex linkages, and the immature technology of sequencing instrument analysis, the related molecular mechanisms need to be further explored. In addition, glycans are highly expressed in the body fluids of patients (such as serum and urine) and on the surface of cancer cells. We can use it as a marker for the daily diagnosis of cancer and provide assistance for tumor treatment and prognosis. Therefore, better understanding the changes of the structure and function of abnormal glycans in bladder cancer will be beneficial to the diagnosis and treatment of bladder cancer, and will provide the possibility for the development of new anti-tumor drugs.
Author Contributions
YJ and ZX wrote the manuscript. CX, LZ, XS provided language help and writing assistance. SW and DY conceived ideas and modified manuscript. All authors were involved in the conception, preparation of the manuscript, and the final version of the manuscript has been read and approved by all authors before its submission.
Funding
This work is supported by the National Natural Science Foundation of China (31470799) and Special Fund of Dalian city for Distinguished Young Scholars (2017RJ07).
Conflict of Interest
The authors declare that the research was conducted in the absence of any commercial or financial relationships that could be construed as a potential conflict of interest.
References
1. Siegel RL, Miller KD, Jemal A. Cancer statistics, 2015. CA Cancer J Clin. (2015) 65:5–29. doi: 10.3322/caac.21254
2. Kamat AM, Hahn NM, Efstathiou JA, Lerner SP, Malmstrom PU, Choi W, et al. Bladder cancer. Lancet. (2016) 388:2796–810. doi: 10.1016/S0140-6736(16)30512-8
3. Babjuk M. Trends in bladder cancer incidence and mortality: success or disappointment? Eur Urol. (2017) 71:109–10. doi: 10.1016/j.eururo.2016.06.040
5. Munkley J, Elliott DJ. Hallmarks of glycosylation in cancer. Oncotarget. (2016) 7:35478–89. doi: 10.18632/oncotarget.8155
6. Varki A, Kannagi R, Toole B, Stanley P. Glycosylation changes in cancer. In: rd, Varki A, Cummings RD, Esko JD, Stanley P, Hart GW, Aebi M, et al., editors, Essentials of Glycobiology. Cold Spring Harbor, NY: Cold Spring Harbor Laboratory Press (2015). p. 597–609.
7. Hakomori S, Kannagi R. Glycosphingolipids as tumor-associated and differentiation markers. J Natl Cancer Inst. (1983) 71:231–51.
8. Mechref Y, Hu Y, Garcia A, Hussein A. Identifying cancer biomarkers by mass spectrometry-based glycomics. Electrophoresis. (2012) 33:1755–67. doi: 10.1002/elps.201100715
9. Hauselmann I, Borsig L. Altered tumor-cell glycosylation promotes metastasis. Front Oncol. (2014) 4:28. doi: 10.3389/fonc.2014.00028
10. Ding D, Yao Y, Zhang S, Su C, Zhang Y. C-type lectins facilitate tumor metastasis. Oncol Lett. (2017) 13:13–21. doi: 10.3892/ol.2016.5431
11. Min X, Wen J, Zhao L, Wang K, Li Q, Huang G, et al. Role of hepatoma-derived growth factor in promoting de novo lipogenesis and tumorigenesis in hepatocellular carcinoma. Mol Oncol. (2018) 12:1480–97. doi: 10.1002/1878-0261.12357
12. Guo J, Li X, Tan Z, Lu W, Yang G, Guan F. Alteration of N-glycans and expression of their related glycogenes in the epithelial-mesenchymal transition of HCV29 bladder epithelial cells. Molecules (Basel, Switzerland). (2014) 19:20073–90. doi: 10.3390/molecules191220073
13. Xu Q, Niu X, Wang W, Yang W, Du Y, Gu J, et al. Specific N-glycan alterations are coupled in EMT induced by different density cultivation of MCF 10A epithelial cells. Glycoconj J. (2017) 34:219–27. doi: 10.1007/s10719-016-9754-3
14. T. de Vries, Knegtel RM, Holmes EH, Macher BA. Fucosyltransferases: structure/function studies. Glycobiology. (2001) 11:119r−28r. doi: 10.1093/glycob/11.10.119R
15. Lu YC, Chen CN, Chu CY, Lu J, Wang BJ, Chen CH, et al. Calreticulin activates β1 integrin via fucosylation by fucosyltransferase 1 in J82 human bladder cancer cells. Biochem J. (2014) 460:69–78. doi: 10.1042/BJ20131424
16. Yang X, Zhang Z, Jia S, Liu Y, Wang X, Yan Q. Overexpression of fucosyltransferase IV in A431 cell line increases cell proliferation. Int J Biochem Cell Biol. (2007) 39:1722–30. doi: 10.1016/j.biocel.2007.04.024
17. Zhang Y, Zhang D, Lv J, Wang S, Zhang Q. MiR-125a-5p suppresses bladder cancer progression through targeting FUT4. Biomed Pharmacother. (2018) 108:1039–47. doi: 10.1016/j.biopha.2018.09.100
18. Yang G, Tan Z, Lu W, Guo J, Yu H, Yu J, et al. Quantitative glycome analysis of N-glycan patterns in bladder cancer vs normal bladder cells using an integrated strategy. J Proteome Res. (2015) 14:639–53. doi: 10.1021/pr5006026
19. F. Dal Moro, Valotto C, Guttilla A, Zattoni F. Urinary markers in the everyday diagnosis of bladder cancer. Urologia. (2013) 80:265–75. doi: 10.5301/urologia.5000041
20. Pal K, Roy S, Mondal SA, Chatterjee U, Tiwari P, Bera M. Urinary level of CA19-9 as a tumor marker in urothelial carcinoma of the bladder. Urol J. (2011) 8:203–8.
21. Jha DK, Mittal A, Gupta SP, Sathian B. Significance of CA19-9 in predicting the prognosis of urothelial carcinoma: a hospital based study from Nepal. Asian Pac J Cancer Prev. (2013) 14:4067–9. doi: 10.7314/APJCP.2013.14.7.4067
22. Trinchera M, Aronica A, Dall'Olio F. Selectin Ligands Sialyl-Lewis a and Sialyl-Lewis x in gastrointestinal cancers. Biology (Basel). (2017) 6:16. doi: 10.3390/biology6010016
23. Numahata K, Satoh M, Handa K, Saito S, Ohyama C, Ito A, et al. Sialosyl-Le(x) expression defines invasive and metastatic properties of bladder carcinoma. Cancer. (2002) 94:673–85. doi: 10.1002/cncr.10268
24. Carrascal MA, Severino PF, Guadalupe Cabral M, Silva M, Ferreira JA, Calais F, et al. Sialyl Tn-expressing bladder cancer cells induce a tolerogenic phenotype in innate and adaptive immune cells. Mol Oncol. (2014) 8:753–65. doi: 10.1016/j.molonc.2014.02.008
25. Huang C, Huang M, Chen W, Zhu W, Meng H, Guo L, et al. N-acetylglucosaminyltransferase V modulates radiosensitivity and migration of small cell lung cancer through epithelial-mesenchymal transition. FEBS J. (2015) 282:4295–306. doi: 10.1111/febs.13419
26. Li B, Su S, Zhang MY, He L, Wang QD, He K. Effect of GnT-V knockdown on the proliferation, migration and invasion of the SMMC7721/R human hepatocellular carcinoma drug-resistant cell line. Mol Med Rep. (2016) 13:469–76. doi: 10.3892/mmr.2015.4492
27. Nagae M, Kizuka Y, Mihara E, Kitago Y, Hanashima S, Ito Y. Structure and mechanism of cancer-associated N-acetylglucosaminyltransferase-V. Nat Commun. (2018) 9:3380. doi: 10.1038/s41467-018-05931-w
28. Wang LY, Chen KL, Su JM, Jin JW, Chen HL, Zha XL. [GnT-V overexpression in human hepatocarcinoma cells affects its migration and expression of cell adhesion molecules]. Shi Yan Sheng Wu Xue Bao. (2001) 34:219–25.
29. Miyoshi E, Terao M, Kamada Y. Physiological roles of N-acetylglucosaminyltransferase V(GnT-V) in mice. BMB Rep. (2012) 45:554–9. doi: 10.5483/BMBRep.2012.45.10.190
30. Takahashi T, Hagisawa S, Yoshikawa K, Tezuka F, Kaku M, Ohyama C. Predictive value of N-acetylglucosaminyltransferase-V for superficial bladder cancer recurrence. J Urol. (2006) 175:90–3; discussion 93. doi: 10.1097/00005392-200601000-00021
31. Zhao Y, Nakagawa T, Itoh S, Inamori K, Isaji T, Kariya Y, et al. N-acetylglucosaminyltransferase III antagonizes the effect of N-acetylglucosaminyltransferase V on α3β1 integrin-mediated cell migration. J Biol Chem. (2006) 281:32122–30. doi: 10.1074/jbc.M607274200
32. Allam H, Johnson BP, Zhang M, Lu Z, Cannon MJ, Abbott KL. The glycosyltransferase GnT-III activates Notch signaling and drives stem cell expansion to promote the growth and invasion of ovarian cancer. J Biol Chem. (2017) 292:16351–9. doi: 10.1074/jbc.M117.783936
33. Akama R, Sato Y, Kariya Y, Isaji T, Fukuda T, Lu L, et al. N-acetylglucosaminyltransferase III expression is regulated by cell-cell adhesion via the E-cadherin-catenin-actin complex. Proteomics. (2008) 8:3221–8. doi: 10.1002/pmic.200800038
34. Guo JM, Zhang XY, Chen HL, Wang GM, Zhang YK. Structural alterations of sugar chains in urine fibronectin from bladder cancer patients and its enzymatic mechanism. J Cancer Res Clin Oncol. (2001) 127:512–9. doi: 10.1007/s004320100245
35. Tsuboi S, Hatakeyama S, Ohyama C, Fukuda M. Two opposing roles of O-glycans in tumor metastasis. Trends Mol Med. (2012) 18:224–32. doi: 10.1016/j.molmed.2012.02.001
36. M. Sutoh Yoneyama, Tobisawa Y, Hatakeyama S, Sato M, Tone K, Tatara Y, et al. A mechanism for evasion of CTL immunity by altered O-glycosylation of HLA class I. J Biochem. (2017) 161:479–92. doi: 10.1093/jb/mvw096
37. Suzuki Y, Sutoh M, Hatakeyama S, Mori K, Yamamoto H, Koie T, et al. MUC1 carrying core 2 O-glycans functions as a molecular shield against NK cell attack, promoting bladder tumor metastasis. Int J Oncol. (2012) 40:1831–8. doi: 10.3892/ijo.2012.1411
38. Hagisawa S, Ohyama C, Takahashi T, Endoh M, Moriya T, Nakayama J, et al. Expression of core 2 β1,6-N-acetylglucosaminyltransferase facilitates prostate cancer progression. Glycobiology. (2005) 15:1016–24. doi: 10.1093/glycob/cwi086
39. Tsuboi S, Sutoh M, Hatakeyama S, Hiraoka N, Habuchi T, Horikawa Y, et al. A novel strategy for evasion of NK cell immunity by tumours expressing core2 O-glycans. EMBO J. (2011) 30:3173–85. doi: 10.1038/emboj.2011.215
40. Lee SH, Hatakeyama S, Yu SY, Bao X, Ohyama C, Khoo KH, et al. Core3 O-glycan synthase suppresses tumor formation and metastasis of prostate carcinoma PC3 and LNCaP cells through down-regulation of α2β1 integrin complex. J Biol Chem. (2009) 284:17157–69. doi: 10.1074/jbc.M109.010934
41. Langkilde NC, Wolf H, Clausen H, Kjeldsen T, Orntoft TF. Nuclear volume and expression of T-antigen, sialosyl-Tn-antigen, and Tn-antigen in carcinoma of the human bladder. Relation to tumor recurrence and progression. Cancer. (1992) 69:219–27. doi: 10.1002/1097-0142(19920101)69:1<219::AID-CNCR2820690136>3.0.CO;2-A
42. Lima L, Neves M, Oliveira MI, Dieguez L, Freitas R, Azevedo R, et al. Sialyl-Tn identifies muscle-invasive bladder cancer basal and luminal subtypes facing decreased survival, being expressed by circulating tumor cells and metastases. Urol Oncol. (2017) 35:675.e1–e8. doi: 10.1016/j.urolonc.2017.08.012
43. Eavarone DA, Al-Alem L, Lugovskoy A, Prendergast JM, Nazer RI, Stein JN, et al. Humanized anti-Sialyl-Tn antibodies for the treatment of ovarian carcinoma. PLoS One. (2018) 13:e0201314. doi: 10.1371/journal.pone.0201314
44. Berriel E, Hill M, Barcia JJ, Ubillos L, Gonzalez M, Detjen G, et al. Simple mucin-type cancer associated antigens are pre-cancerous biomarkers during 1,2-dimethylhydrazine-induced rat colon carcinogenesis. Oncol Rep. (2005) 14:219–27.
45. D'Amico TA, Aloia TA, Moore MB, Herndon JE 2nd, Brooks KR, Lau CL, et al. Molecular biologic substaging of stage I lung cancer according to gender and histology. Ann Thorac Surg. (2000) 69:882–6. doi: 10.1016/S0003-4975(99)01522-2
46. Freitas D, Campos D, Gomes J, Pinto F, Macedo JA, Matos R, et al. O-glycans truncation modulates gastric cancer cell signaling and transcription leading to a more aggressive phenotype. EBioMedicine. (2019) 40:349–62. doi: 10.1016/j.ebiom.2019.01.017
47. Munkley J, Oltean S, Vodak D, Wilson BT, Livermore KE, Zhou Y, et al. The androgen receptor controls expression of the cancer-associated sTn antigen and cell adhesion through induction of ST6GalNAc1 in prostate cancer. Oncotarget. (2015) 6:34358–74. doi: 10.18632/oncotarget.6024
48. Loureiro LR, Sousa DP. Novel monoclonal antibody L2A5 specifically targeting sialyl-Tn and short glycans terminated by α-2-6 sialic acids. (2018) 8:12196. doi: 10.1038/s41598-018-30421-w
49. Zeidan Q, Hart GW. The intersections between O-GlcNAcylation and phosphorylation: implications for multiple signaling pathways. J Cell Sci. (2010) 123:13–22. doi: 10.1242/jcs.053678
50. Yang WH, Park SY, Nam HW, Kim DH, Kang JG, Kang ES, et al. NFκB activation is associated with its O-GlcNAcylation state under hyperglycemic conditions. Proc Natl Acad Sci U S A. (2008) 105:17345–50. doi: 10.1073/pnas.0806198105
51. Bapu D, Runions J, Kadhim M, Brooks SA. N-acetylgalactosamine glycans function in cancer cell adhesion to endothelial cells: a role for truncated O-glycans in metastatic mechanisms. Cancer Lett. (2016) 375:367–74. doi: 10.1016/j.canlet.2016.03.019
52. Rozanski W, Krzeslak A, Forma E, Brys M, Blewniewski M, Wozniak P, et al. Prediction of bladder cancer based on urinary content of MGEA5 and OGT mRNA level. Clin Lab. (2012) 58:579–83.
53. Hirao Y, Kim WJ, Fujimoto K. Environmental factors promoting bladder cancer. Curr Opin Urol. (2009) 19:494–9. doi: 10.1097/MOU.0b013e32832eb4ef
54. Niedworok C, Rock K, Kretschmer I, Freudenberger T, Nagy N, Szarvas T, et al. Inhibitory role of the small leucine-rich proteoglycan biglycan in bladder cancer. PLoS One. (2013) 8:e80084. doi: 10.1371/journal.pone.0080084
55. Horvath Z, Kovalszky I, Fullar A, Kiss K, Schaff Z, Iozzo RV, et al. Decorin deficiency promotes hepatic carcinogenesis. Matrix Biol. (2014) 35:194–205. doi: 10.1016/j.matbio.2013.11.004
56. Appunni S, Anand V, Khandelwal M, Gupta N, Rubens M, Sharma A. Small leucine rich proteoglycans (decorin, biglycan and lumican) in cancer. Clin Chim Acta. (2019) 491:1–7. doi: 10.1016/j.cca.2019.01.003
57. Sainio A, Nyman M, Lund R, Vuorikoski S, Bostrom P, Laato M, et al. Lack of decorin expression by human bladder cancer cells offers new tools in the therapy of urothelial malignancies. PLoS One. (2013) 8:e76190. doi: 10.1371/journal.pone.0076190
58. Iozzo RV, Chakrani F, Perrotti D, McQuillan DJ, Skorski T, Calabretta B, et al. Cooperative action of germ-line mutations in decorin and p53 accelerates lymphoma tumorigenesis. Proc Natl Acad Sci U S A. (1999) 96:3092–7. doi: 10.1073/pnas.96.6.3092
59. Ferro M, Giuberti G, Zappavigna S, Perdona S, Facchini G, Sperlongano P, et al. Chondroitin sulphate enhances the antitumor activity of gemcitabine and mitomycin-C in bladder cancer cells with different mechanisms. Oncol Rep. (2012) 27:409–15. doi: 10.3892/or.2011.1526
60. Toole BP, Zoltan-Jones A, Misra S, Ghatak S. Hyaluronan: a critical component of epithelial-mesenchymal and epithelial-carcinoma transitions. Cells Tissues Organs. (2005) 179:66–72. doi: 10.1159/000084510
61. Itano N, Atsumi F, Sawai T, Yamada Y, Miyaishi O, Senga T, et al. Abnormal accumulation of hyaluronan matrix diminishes contact inhibition of cell growth and promotes cell migration. Proc Natl Acad Sci U S A. (2002) 99:3609–14. doi: 10.1073/pnas.052026799
62. Oldenburg D, Ru Y, Weinhaus B, Cash S, Theodorescu D, Guin S. CD44 and RHAMM are essential for rapid growth of bladder cancer driven by loss of Glycogen Debranching Enzyme (AGL). BMC Cancer. (2016) 16:713. doi: 10.1186/s12885-016-2756-5
63. Guin S, Ru Y, Agarwal N, Lew CR, Owens C, Comi GP, et al. Loss of glycogen debranching enzyme AGL drives bladder tumor growth via induction of hyaluronic acid synthesis. Clin Cancer Res. (2016) 22:1274–83. doi: 10.1158/1078-0432.CCR-15-1706
64. Golshani R, Lopez L, Estrella V, Kramer M, Iida N, Lokeshwar VB. Hyaluronic acid synthase-1 expression regulates bladder cancer growth, invasion, and angiogenesis through CD44. Cancer Res. (2008) 68:483–91. doi: 10.1158/0008-5472.CAN-07-2140
65. Wu CT, Lin WY, Chen WC, Chen MF. Predictive value of CD44 in muscle-invasive bladder cancer and its relationship with IL-6 signaling. Ann Surg Oncol. (2018) 25:3518–26. doi: 10.1245/s10434-018-6706-0
66. Jordan AR, Lokeshwar SD, Lopez LE, Hennig M, Chipollini J, Yates T, et al. Antitumor activity of sulfated hyaluronic acid fragments in pre-clinical models of bladder cancer. Oncotarget. (2017) 8:24262–74. doi: 10.18632/oncotarget.10529
67. McAtee CO, Berkebile AR, Elowsky CG, Fangman T, Barycki JJ, Wahl JK, et al. Hyaluronidase Hyal1 increases tumor cell proliferation and motility through accelerated vesicle trafficking. J Biol Chem. (2015) 290:13144–56. doi: 10.1074/jbc.M115.647446
68. Benitez A, Yates TJ, Lopez LE, Cerwinka WH, Bakkar A, Lokeshwar VB. Targeting hyaluronidase for cancer therapy: antitumor activity of sulfated hyaluronic acid in prostate cancer cells. Cancer Res. (2011) 71:4085–95. doi: 10.1158/0008-5472.CAN-10-4610
69. Liang Z, Zhang Q, Wang C, Shi F, Cao H, Yu Y, et al. Hyaluronic acid/ Hyaluronidase as biomarkers for bladder cancer: a diagnostic meta-analysis. Neoplasma. (2017) 64:901–8. doi: 10.4149/neo_2017_612
70. Kramer MW, Escudero DO, Lokeshwar SD, Golshani R, Ekwenna OO, Acosta K, et al. Association of hyaluronic acid family members (HAS1, HAS2, and HYAL-1) with bladder cancer diagnosis and prognosis. Cancer. (2011) 117:1197–209. doi: 10.1002/cncr.25565
71. Schroeder GL, Lorenzo-Gomez MF, Hautmann SH, Friedrich MG, Ekici S, Huland H, et al. A side by side comparison of cytology and biomarkers for bladder cancer detection. J Urol. (2004) 172:1123–6. doi: 10.1097/01.ju.0000134347.14643.ab
72. S.I. Hakomori Si. The glycosynapse. Proc Natl Acad Sci U S A. (2002) 99:225–32. doi: 10.1073/pnas.012540899
73. Hakomori SI. Glycosynaptic microdomains controlling tumor cell phenotype through alteration of cell growth, adhesion, and motility. FEBS Lett. (2010) 584:1901–6. doi: 10.1016/j.febslet.2009.10.065
74. Sachinidis A, Kraus R, Seul C, Meyer zu Brickwedde MK, Schulte K, Ko Y, et al. Gangliosides GM1, GM2 and GM3 inhibit the platelet-derived growth factor-induced signalling transduction pathway in vascular smooth muscle cells by different mechanisms. Eur J Cell Biol. (1996) 71:79–88.
Keywords: glycans, glycosyltransferases, bladder cancer, abnormal glycosylation, biomarkers
Citation: Jian Y, Xu Z, Xu C, Zhang L, Sun X, Yang D and Wang S (2020) The Roles of Glycans in Bladder Cancer. Front. Oncol. 10:957. doi: 10.3389/fonc.2020.00957
Received: 23 March 2020; Accepted: 15 May 2020;
Published: 12 June 2020.
Edited by:
Fabio Grizzi, Humanitas Research Hospital, ItalyReviewed by:
Scot Niglio, National Institutes of Health (NIH), United StatesJaleh Fallah, Cleveland Clinic, United States
Copyright © 2020 Jian, Xu, Xu, Zhang, Sun, Yang and Wang. This is an open-access article distributed under the terms of the Creative Commons Attribution License (CC BY). The use, distribution or reproduction in other forums is permitted, provided the original author(s) and the copyright owner(s) are credited and that the original publication in this journal is cited, in accordance with accepted academic practice. No use, distribution or reproduction is permitted which does not comply with these terms.
*Correspondence: Deyong Yang, eWFuZ2RleW9uZyYjeDAwMDQwO2RtdS5lZHUuY24=; Shujing Wang, d2FuZ3NodWppbmcmI3gwMDA0MDtkbXUuZWR1LmNu
†These authors have contributed equally to this work