- 1Division of Physical Activity, Prevention and Cancer, German Cancer Research Center (DKFZ) and National Center for Tumor Diseases (NCT), Heidelberg, Germany
- 2Department for Molecular and Cellular Sports Medicine, Institute for Cardiovascular Research and Sports Medicine, German Sport University Cologne, Cologne, Germany
- 3DKTK Brain Cancer Metabolism Group, German Cancer Research Center (DKFZ), Heidelberg, Germany
- 4Department of Medical Oncology, National Center for Tumor Diseases (NCT), University Hospital Heidelberg, Heidelberg, Germany
- 5Neuroimmun GmbH, Karlsruhe, Germany
- 6Department of Radiation Oncology, National Center for Radiation Oncology (NCRO), Heidelberg Institute for Radiation Oncology (HIRO), University Hospital Heidelberg, Heidelberg, Germany
- 7Department of Population Health Sciences, Huntsman Cancer Institute, University of Utah, Salt Lake City, UT, United States
- 8Department of Neurology and National Center for Tumor Diseases (NCT), University Hospital Heidelberg, Heidelberg, Germany
Purpose: Evidence from preclinical studies and trials in healthy volunteers suggests that exercise may modulate the levels of tryptophan (TRP) metabolites along the kynurenine (KYN) pathway. As KYN and downstream KYN metabolites are known to promote cancer progression by inhibiting anti-tumor immune responses and by promoting the motility of cancer cells, we investigated if resistance exercise can also control the levels of KYN pathway metabolites in breast cancer patients undergoing radiotherapy (NCT01468766).
Patients and Methods: Chemotherapy-naïve breast cancer patients (n = 96) were either randomized to an exercise/intervention group (IG) or a control group (CG). The IG participated in a 12-week supervised progressive resistance exercise program twice a week, whereas the CG received a supervised relaxation program. Serum levels of TRP and KYN as well as urine levels of kynurenic acid (KYNA) and neurotoxic quinolinic acid (QUINA) were assessed before (t0), after radiotherapy, and mid-term of the exercise intervention (t1) and after the exercise intervention (t2). Additionally, 24 healthy women (HIG) participated in the exercise program to investigate potential differences in its effects on KYN metabolites in comparison to the breast cancer patients.
Results: At baseline (t0) the breast cancer patients showed a significantly elevated serum KYN/TRP ratio and urine QUINA/KYNA ratio, as well as increased urine QUINA levels in comparison to the healthy women. In response to exercise the healthy women and the breast cancer patients differed significantly in the levels of urine QUINA and the QUINA/KYNA ratio. Most importantly, serum KYN levels and the KYN/TRP ratio were significantly reduced in exercising patients (IG) compared to non-exercising patients (CG) both at t1 and t2.
Conclusion: Resistance exercise may represent a potent non-pharmacological avenue to counteract an activation of the KYN pathway in breast cancer patients undergoing radiotherapy.
Introduction
Increased levels of kynurenine (KYN), a catabolite of the amino acid tryptophan (TRP), are associated with progression and poor clinical outcome in numerous cancer types (1). The degradation of TRP to KYN is catalyzed by the isoenzymes tryptophan 2,3-dioxygenase (TDO) and indoleamine 2,3-dioxygenase 1 and 2 (IDO1, IDO2). Cancers often express high levels of IDO1 and/or TDO and TRP metabolism represents a critical metabolic pathway controlling tumor immune evasion as well as tumor cell malignant properties (2–7). Inhibitors of IDO1 and TDO as well as downstream modulators of the KYN pathway and its targets are therefore being developed for cancer immunotherapy and are advancing into clinical trials. KYN as well as its downstream metabolites kynurenic acid (KYNA) and quinolinic acid (QUINA) mediate various inhibitory effects on the immune system including suppression of T-cell proliferation, the differentiation of regulatory T-cells, suppression of Th17 cells, promotion of tolerogenic dendritic cells (DC), and an impaired function of effector cells such as natural killer (NK) cells and cytotoxic T-cells (8–14).
Regular physical exercise is associated with decreased cancer risk and progression (15). It has been speculated that these positive effects of exercise are partially mediated by influencing the body's (anti-) inflammatory homeostasis. In contrast to a single bout of exercise, which induces short-term systemic inflammation, long-term regular physical exercise is known to reduce systemic inflammation (16). As IDO1 and TDO are induced by pro-inflammatory stimuli (17, 18), distinct exercise modalities may hence differentially influence their activity and downstream effects.
Apart from cancer, a dysregulation of the KYN pathway has also been described for neuropsychiatric and neurodegenerative disorders, such as depression, Alzheimer's disease, and multiple sclerosis (19, 20). These effects of the KYN pathway in the central nervous system (CNS) may also be relevant for oncology, as many cancer patients suffer from depressive symptoms, fatigue, and cognitive impairments (21, 22). Elevated blood levels of KYN have been shown to induce depressive symptoms in mice (23) and have been measured in depressive patients (24). KYN generated by tumors or the tumor microenvironment is able to cross the blood brain barrier (25) and can further be metabolized to QUINA or KYNA in the CNS. QUINA stimulates excitatory N-methyl-D-aspartate (NMDA) receptors and can induce neuronal excitotoxicity, while KYNA inhibits NMDA-, kainate-, and α-amino-3-hydroxy-5-methyl-4-isoxazolepropionic acid (AMPA) receptors, in addition to negatively modulating α7 nicotinic receptors (26, 27). Due to their opposing neuroactive properties, alterations in the balance between QUINA and KYNA are considered to play an important role in neurodegenerative and neuropsychiatric diseases (26–28).
Regular physical exercise has been described as a promising (supportive) non-pharmacological treatment to counteract the development and progression of neurological disorders (29–32). In regard to cancer, accumulating evidence suggests that exercise reduces fatigue, depressive symptoms, and cancer related cognitive impairment (15, 33). Apart from the anti-inflammatory effects of physical exercise, Agudelo et al. have shown that physical exercise induces the expression of specific isoforms of the enzyme KYN amino transferase (KAT) in skeletal muscle of mice, leading to an enhanced peripheral conversion of KYN to KYNA (23). As KYNA is poorly able to cross the blood brain barrier (25), the conversion of KYN to KYNA in the periphery was thought to decrease CNS KYN levels and thus reduce neuroinflammation as well as depressive symptoms.
In light of the anti-inflammatory properties of physical exercise and the important role of skeletal muscle as a modifier of the KYN pathway, we investigated the effect of a supervised 12-week resistance exercise program on TRP and downstream TRP metabolites in breast cancer patients undergoing radiotherapy.
Materials and Methods
The study was approved by the Ethics Committee of the University of Heidelberg (NCT01468766) and complied with the principles of the Declaration of Helsinki. Written informed consent was obtained from all participants.
General Procedure
Interested women underwent a baseline assessment, including a check for contraindications for resistance training, e.g., acute infectious disease, severe cardiac disease, severe respiratory insufficiency, as well as an ECG at rest followed by an endurance performance test on a cycle ergometer in combination with an exercise ECG. Women were included only if there were no concerns regarding progressive resistance training. Chemotherapy-naïve breast cancer patients (n = 96) were randomized into either an exercise/intervention group (IG) or a control group (CG). Allocation was done by a biometrician who was not involved in the recruitment procedure, based on predetermined lists with random block size, stratified by age and baseline physical fatigue level [fatigue was the primary outcome of this trial (34)]. Study personnel did not have access to the randomization lists. The IG participated in a 12-week supervised progressive resistance exercise program twice a week starting in parallel to adjuvant radiotherapy, whereas the CG received a supervised relaxation program (34). Resting blood and urine samples were taken prior to (t0), after completion of the 6-week radiation therapy i.e., in the middle of the exercise intervention (t1), and after completing the 12-week exercise program (t2). In addition, 24 healthy women (HIG) were recruited to participate in the same exercise program as the IG.
In- and Exclusion Criteria
The in- and exclusion criteria were defined as follows: patients were 18 years or older, diagnosed with breast cancer (stage 0–3), had not received adjuvant or neo-adjuvant chemotherapy, did not have physical impairment preventing participation in the exercise program (e.g., acute infectious disease, severe cardiac disease, severe respiratory insufficiency), and no other concomitant malignant diseases. Healthy subjects of comparable age were included that never had cancer, any other chronic internal disease or orthopedic impairment preventing participation in the exercise program.
Intervention
A detailed description of the exercise intervention was already published (35, 36). In brief, the 12-week whole body resistance exercise program was conducted twice weekly for 1 h and consisted of eight different, machine-based exercises (leg extension; leg curl; leg press; shoulder internal and external rotation; seated row; latissimus pull down; shoulder flexion and extension and butterfly and butterfly reverse). Patients performed three sets with a maximum of twelve repetitions (60–80% of the one repetition maximum) and 1 min resting periods in between sets. Training was progressive in terms of weight increase to the next machine weight level (at least by 5%) after successfully completing three sets of an exercise with 12 repetitions in three consecutive sessions. The control group participated in a progressive muscle relaxation training according to Jacobsen, which also took place twice weekly for 1 h.
Outcome Measures
Serum TRP and KYN levels as well as urine KYNA and QUINA levels were analyzed for all time points by Enzyme-linked Immunosorbent Assay (ELISA) according to the manufacturer's instructions using the following ELISA kits: #K7726, #K7735, and #K7736 (Neuroimmun GmbH, Germany).
The performance characteristics of the ELISAs are as follows:
#K7726
Precision and Reproducibility for L-kynurenine
Spiking Recovery
Three samples were spiked with different L-kynurenine concentrations and measured in this assay (n = 2). The mean recovery rate was 102.5%.
Dilution Recovery
Two spiked samples were diluted and analyzed. The mean recovery rate was 100.3% (n = 2).
Analytical Sensitivity
Limit of detection, LoD 0.12 μmol/l
Precision and Reproducibility for L-tryptophan
Spiking Recovery
Three serum samples were spiked with different L-tryptophan concentrations and measured in this assay (n = 2). The mean recovery rate for all concentrations was 97.2%.
Dilution Recovery
Two serum samples were diluted and measured in this assay. The mean recovery was 96.8% (n = 2).
Analytical Sensitivity
Limit of detection, LoD 8.0 μmol/l
#K7735
Precision and Reproducibility for Kynurenic Acid
Spiking Recovery
Three urine samples were spiked with different kynurenic acid concentrations and measured in this assay. The mean recovery rate was 95.8% (n = 2).
Dilution Recovery
Three urine samples were diluted with assay buffer and measured in this assay. The mean recovery rate was 106.8% (n = 2).
Analytical Sensitivity
The zero-standard was measured 48 times. The detection limit was set as B0−2 SD and estimated to be 0.3 μmol/l.
K7736
Precision and Reproducibility for Quinolinic Acid
Spiking Recovery
Three urine samples were spiked with different quinolinic acid concentrations and measured in this assay. The mean recovery rate was 101.0% (n = 2).
Dilution Recovery
Two urine samples were diluted with assay buffer and measured in this assay. The mean recovery rate was 101.0% (n = 2).
Analytical Sensitivity
The zero-standard was measured 50 times. The detection limit was set as B0−2 SD and estimated to be 2.8 μmol/l.
Analysis of ELISA values were performed with the Magellan software V 7.2 (Tecan Austria GmbH). Urine KYNA and QUINA were normalized to Creatinine. The KYN/TRP ratio was calculated to indirectly assess TRP degradation. The KYNA/KYN ratio was used as indicator for KAT activity. The QUINA/KYNA ratio was calculated as parameter to indirectly assess neurotoxicity.
Statistics
All statistical analysis were conducted using SPSS 25 (IBM). First, t-Tests were used to compare baseline values of all outcomes between healthy women and breast cancer patients. Second, a baseline-adjusted 2 (groups: IG, HIG) × 2 (t0, t2) ANCOVA was used to investigate potential differences of the exercise program on the KYN pathway between breast cancer patients (IG) and healthy subjects (HIG). Third, a baseline- and age-adjusted 2 (groups: IG, CG) × 3 (time points: t0, t1, t2) ANCOVA was conducted to determine differences for changes in each outcome variable between IG and CG. Significant time effects and group x time interactions were further analyzed by Bonferroni corrected simple effects analysis. Prior to ANCOVA a z-transformation was conducted to identify outliers (> +/– 3 SD). For all analysis alpha was set at 0.05.
Results
To investigate the effect of a supervised 12-week resistance exercise program on TRP metabolism, we measured TRP and KYN in the serum and KYNA and QUINA in the urine of healthy women as well as breast cancer patients undergoing radiotherapy in the BEST study (34). The baseline characteristics of the breast cancer patients (n = 96) who were randomized to the intervention group (IG) and control group (CG) as well those of the healthy intervention group (HIG, n = 24) are shown in Table 1. Overall adherence was similar in IG and CG with a median of 20 out of 24 scheduled visits.
Baseline Comparison Between Patients With Breast Cancer and Healthy Subjects
In comparison to the healthy subjects (HIG) the breast cancer patients (IG + CG) showed significantly elevated serum KYN/TRP ratios (p = 0.006) as well as urine QUINA levels (p = 0.003) and QUINA/KYNA ratios (p = 0.003) at baseline (t0) (Figure 1). Moreover, a tendency toward reduced serum TRP levels (p = 0.051) and increased serum KYN levels (p = 0.084) was observed in the patients (Figure 1). No differences were observed for KYNA levels (p = 0.308) and the KYNA/KYN ratio (p = 0.901) (Figure 1).
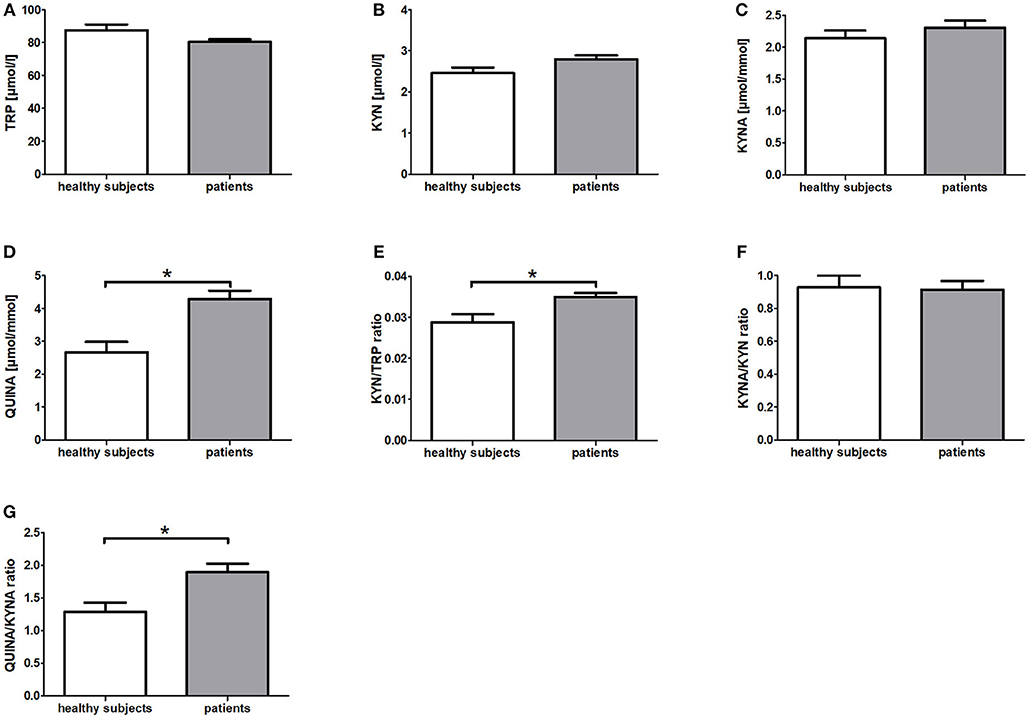
Figure 1. Baseline differences of KYN metabolites and their corresponding ratios between patients with breast cancer and healthy subjects. Data are presented as means +/− standard error of the mean. Significant baseline differences of TRP metabolites and their corresponding ratios (A–G) between patients with breast cancer and healthy subjects are marked by *.
Comparison of the Responses to the Resistance Training Between Breast Cancer Patients and Healthy Subjects
To investigate whether resistance exercise differentially affects healthy individuals and breast cancer patients, we compared serum TRP and KYN levels as well as urine QUINA and KYNA levels between the IG and the HIG at t0 and t2. Raw data and time courses of all outcome measures as well as post-hoc results of 2 (IG, CG) × 2 (t0, t2) ANCOVA are shown in Figure 2. ANCOVA results are listed in Table 2.
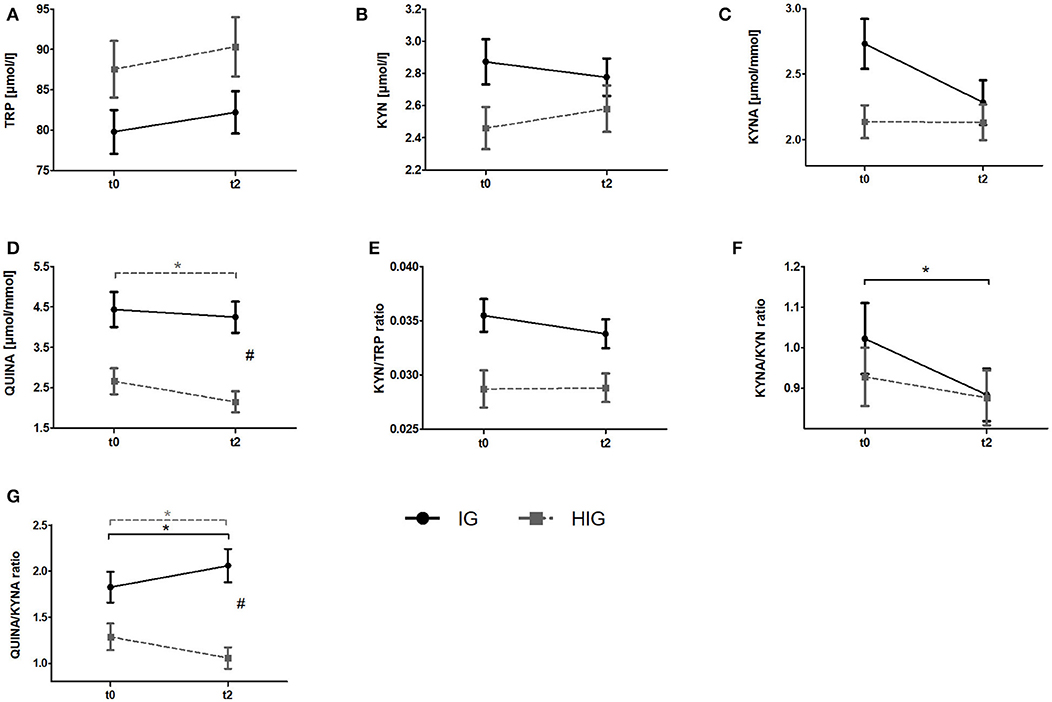
Figure 2. Comparison of responses to the resistance exercise program between patients with breast cancer and healthy subjects. Raw data are presented as means +/− standard error of the mean. IG, intervention group (black); HIG, healthy intervention group (gray); t0, before radiation and exercise program; t2, end of intervention/6 weeks after completing radiation. (A) Tryptophan (TRP) (B) Kynurenine (KYN), (C) Kynurenic acid, (D) QUINA, (E) KYN/TRP ratio, (F) KYNA/KYN ratio, (G) QUINA/KYNA ratio. Post-hoc results for 2 × 2 ANCOVA: *significant within-group differences. #significant between-group differences.
ANCOVA indicated significant time effects for all outcome measures. However, post-hoc analysis indicated only significant decreases for QUINA levels (p = 0.004) and the QUINA/KYNA (p = 0.040) ratio in healthy exercising individuals. In contrast QUINA/KYNA ratio increased in the patient group (p = 0.007). For QUINA levels and QUINA/KYNA ratio ANCOVA additionally showed significant group x time interactions. Post-hoc tests indicated significantly elevated QUINA levels (p = 0.008) and QUINA/KYNA ratio (p = 0.001) at t2 in the patient population.
Impact of Resistance Exercise on the KYN Pathway in Patients With Breast Cancer
To analyze the effects of a resistance exercise program on the KYN pathway in breast cancer patients, we compared serum TRP and KYN levels as well as urine QUINA and KYNA levels between the IG and the CG at t0, t1, and t2. Raw data and time courses of all outcome measures as well as post-hoc results of 2 (IG, CG) × 3 (t0, t1, t2) ANCOVA are shown in Figure 3. ANCOVA results are listed in Table 2.
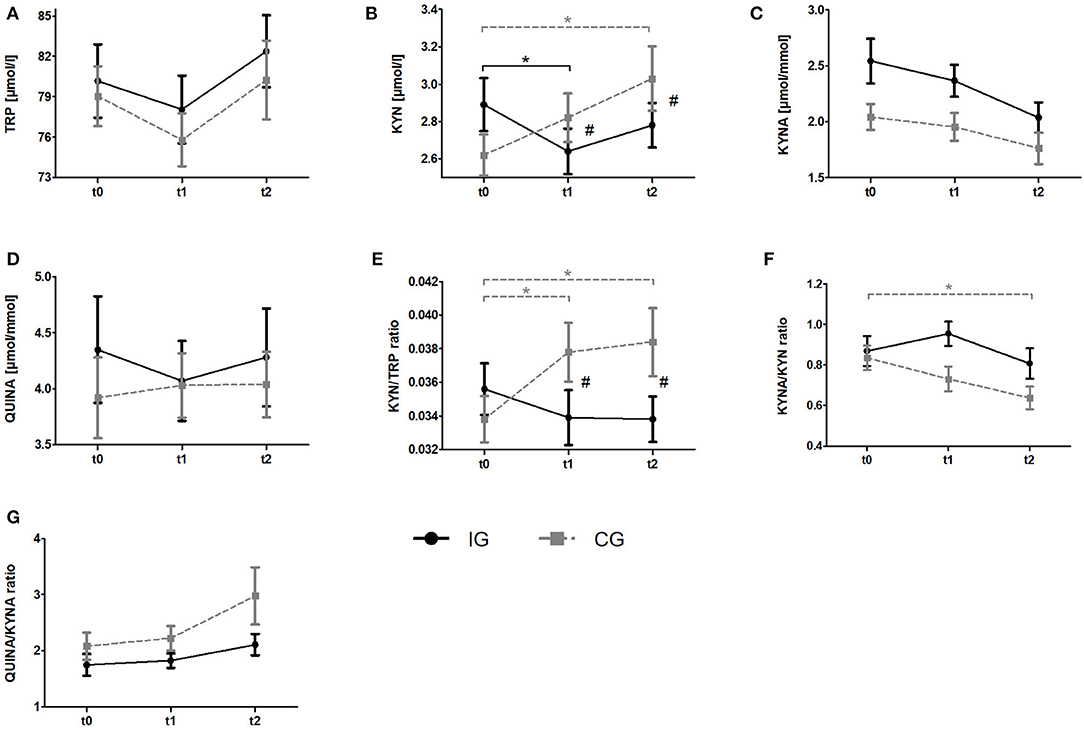
Figure 3. Impact of resistance exercise on the KYN pathway in breast cancer patients undergoing radiotherapy. Raw data are presented as means +/– standard error of the mean. IG, intervention group (black); CG, control group (gray); t0, before radiation and exercise program; t1, mid-intervention/after radiotherapy (t0 + 6 weeks); t2, end of intervention/6 weeks after completing radiation. (A) Tryptophan (TRP) (B) Kynurenine (KYN), (C) Kynurenic acid, (D) QUINA, (E) KYN/TRP ratio, (F) KYNA/KYN ratio, (G) QUINA/KYNA ratio. Post-hoc results for 2 × 3 ANCOCA: *significant within-group differences. #significant between-group differences.
Regarding Trp, ANCOVA revealed a significant time effect. However, post-hoc analysis revealed no significant within-group changes over time. KYN levels indicated a significant group x time interaction. Post-hoc analysis showed a decrease of KYN levels from baseline to t1 (p = 0.036) for the IG whereas the CG showed a constant increase over time which became significant comparing t0 and t2 (p = 0.002). Groups significantly differ at t1 (p = 0.003) and t2 (p = 0.003). For KYNA and QUINA neither time effects nor group × time interactions were found. In view of Kyn/Trp ratio, ANCOVA revealed a significant group × time interaction. Subsequent post-hoc tests indicated a significant increase of KYN/TRP ratio from baseline to t1 (p = 0.009) and to t2 (p = 0.006) in the CG whereas no alterations were detected in the IG. Again, groups significantly differed at t1 (p = 0.003) and t2 (p = 0.003). For KYNA/KYN ratio ANCOVA revealed a significant time effect. Subsequent post-hoc analysis revealed a significant decrease of this ratio from t0 to t2 (p = 0.002) in the CG whereas no changes were observed in the IG. Although a tendency for an interaction was observed in the ANCOVA it does not reach statistical significance (p = 0.053). In view of the QUINA/KYNA ratio neither time effects nor interactions were detected.
Discussion
To our knowledge, this is the first clinical trial investigating the influence of resistance exercise on the KYN pathway. Specifically, we investigated the potential exercise-induced modulation of KYN pathway metabolites in the serum and urine of healthy women and breast cancer patients undergoing radiotherapy. At baseline (t0) the KYN/TRP ratio was elevated in breast cancer patients (IG + CG) compared to healthy women (Figure 1). This finding is in line with a previous study reporting increased TRP metabolism in comparison to healthy controls in breast cancer patients as well as in patients with other cancer entities such as non-small cell lung cancer and adult T cell leukemia/lymphoma (37, 38). Moreover, QUINA levels were significantly elevated in the urine of breast cancer patients in comparison to healthy women (Figure 1), which likely is a direct consequence of the enhanced TRP degradation in these patients. Elevated QUINA levels and QUINA/KYNA ratios in patients may also be the consequence of an inflammation driven induction of kynurenine 3-monooxygenase (KMO) (39). Comparison of the effects of resistance exercise between breast cancer patients (IG) and healthy women (HIG) revealed that while urine QUINA levels and the QUINA/KYNA ratio were reduced in the healthy subjects (HIG), breast cancer patients (IG) showed reduced KYNA/KYN ratios and elevated QUINA/KYNA ratios after completion of the 12-week exercise training (Figure 2). It is not clear why these two populations showed opposite effects of exercise on these downstream KYN pathway metabolites. However, it is important to acknowledge that the two populations not only differed in their disease state but that the breast cancer patients received 6-weeks of radiotherapy during the exercise intervention, which may have contributed to the observed effects on the KYN pathway metabolites.
Interestingly, breast cancer patients who did not exercise (CG) showed a significant and sustainable increase in serum KYN levels and the KYN/TRP ratio after completing radiotherapy (Figure 3). This increase is likely mediated by the radiotherapy, causing tissue damage and subsequent inflammation, which is known to potently induce IDO1 (40). Of note, resistance exercise (IG) counteracted this increase in circulating KYN levels (Figure 3). Previous results from this study and many others suggest that exercise reduces resting levels of inflammatory markers, such as IL-6 (41) and CRP (42). The lower levels of inflammatory cytokines may result in reduced IDO1 expression thus explaining the effect of exercise on the KYN/TRP ratio. However, it remains speculative whether the detected effect of resistance exercise on the KYN/TRP ratio is exclusively related to less expression of IDO1. Exercise is also known to suppress (43) the levels of cortisol (e g., in people with depressive disorders), a well-described inducer of TDO, suggesting that exercise could also downregulate this TRP degrading enzyme (44, 45).
The exercise-induced decrease in resting KYN levels seems to be in line with some of its immune-modulatory properties. Thus far, it was demonstrated that NK-cell cytotoxicity is increased in patients with breast cancer after participating in an endurance exercise program (46). As mentioned in the introduction, NK-cell cytotoxicity is known to be suppressed by increased KYN levels. Additionally, enhanced physical capacity (reached by regular training) is associated with elevated numbers and proportions of regulatory T-cells in the peripheral blood of healthy subjects (47). Regulatory T-cells are known to be induced by elevated KYN levels (48). These findings can be reconciled with our results, as each single bout of physical exercise induces short-term inflammatory processes, including subsequently elevated KYN levels (49, 50). Therefore, a long-term increase in regulatory T-cells may represent the body's response to repeated (short-term exercise-induced) inflammatory stress leading to transiently elevated KYN levels. In contrast, exercise is not expected to increase regulatory T-cells in the tumor microenvironment (51).
Despite reducing serum KYN levels, resistance exercise did not alter the concentrations or ratios of KYNA and QUINA in the urine of the breast cancer patients. Currently it is unclear how the urine levels of these TRP metabolites are associated with the corresponding blood and CNS levels. Agudelo et al. reported an increased conversion of KYN to KYNA after physical exercise in rodents (23). The authors described that muscle KAT4 expression was stimulated by PGC-1α activation in response to endurance exercise. In line with our findings in urine, Millischer et al. reported no changes of the plasma KYNA/KYN ratio in depressive patients in response to varying 12-week home-based exercise programs (yoga like, moderate endurance exercise, intensive mixed endurance, and strength training) (52). The authors hypothesized that relevant alterations of KAT expression are rather expected acutely after exercise, since PGC-1α returns to baseline levels within 24 h after cessation of physical activity. Indeed, this hypothesis is partially confirmed by the results of Schlittler et al. who found increased KAT levels in skeletal muscle in response to a single bout of endurance exercise (53).
Cancer patients frequently suffer from disease- and treatment-related adverse effects, such as cognitive impairment and fatigue which have a certain behavioral and biological (e.g., increased inflammation) overlap with major depression (15). We and others have previously shown that physical exercise during and after medical treatment is able to reduce fatigue (34, 54, 55). Further research is necessary to establish if exercise-induced changes in TRP metabolites can modulate behavioral and patient-reported outcomes. We had few missing data, especially for urine KYNA and QUINA levels because of missing creatinine measurements. This could be avoided by assessing these outcomes in blood samples as well. Moreover, it will be important to analyze the influence of different exercise modalities on cytokines (such as IL-6, IFN-γ, and IL-10), and enzyme expression/activity (e.g., IDO1, KATs, and KMO) in different cell types and tissues (e.g., immune cells, muscle tissue, and tumor tissue) within the KYN pathway. A combined approach assessing acute and chronic effects of exercise-induced alterations of TRP metabolites on the frequencies and properties of specific immune cells (e.g., regulatory T-cells and NK-cells) could provide important mechanistic information on how exercise modulates tumor-relevant immune functions. In regards of the exercise program, endurance exercise or a combination of resistance and endurance exercise could be even more effective than resistance exercise alone. In fact, endurance exercise provokes different and more pronounced physiological adaptions, especially on the metabolic and immunological level. Investigating separate and additional effects of these exercise modalities should be essential next steps in this field of research. Since this study presents a secondary analysis, and therefore an explorative analysis of the BEST trial (35), we only had the option to investigate resistance exercise. Although BMI did not change over time in IG and CG, following studies should screen patients' nutrition status (e.g., intake of supplements) and provide more detailed information on body composition due to potential interactions with TRP metabolism.
In conclusion, the results of this investigation suggest that resistance exercise is able to sustainably reduce KYN levels in breast cancer patients undergoing radiotherapy. In light of the quest for inhibitors of the KYN pathway, resistance exercise may provide a non-pharmacological avenue through which cancer patients can actively reduce the flux through this tumor-promoting metabolic pathway.
Data Availability Statement
The datasets generated for this study are available on request to the corresponding author.
Ethics Statement
The studies involving human participants were reviewed and approved by the Ethics Committee of the University of Heidelberg (NCT01468766) and complied with the principles of the Declaration of Helsinki. The patients/participants provided their written informed consent to participate in this study.
Author Contributions
KS, CU, JW, JD, and MS designed the umbrella study. CO, PZ, KS, KK, and MS designed this specific substudy. JW conducted the exercise intervention. CO, BB, and KK conducted and supported the lab work. JD recruited participants. PZ, CO, KS, MP, and MS performed statistical analysis and wrote the paper.
Funding
This work was supported by the Interdisciplinary Research Funding (intramural) of the National Center for Tumor Diseases (NCT), Heidelberg, Germany (grant number IFP project VI.1); the foundations Stiftung Leben mit Krebs and the Manfred-Lautenschlaeger-Stiftung that partially supported our intervention programs and it received funding from the European Union's Horizon 2020 research and innovation programme under grant agreement No. 754688.
Conflict of Interest
KK is the CEO of Neuroimmun GmbH, which produces and sells ELISA kits for the measurement of tryptophan, kynurenine, kynurenic acid, and quinolinic acid.
The remaining authors declare that the research was conducted in the absence of any commercial or financial relationships that could be construed as a potential conflict of interest.
Acknowledgments
The authors thank the study participants who willingly spent their time to complete the study procedures, the breast cancer centers supporting the recruitment, Dr. Oliver Klassen, Petra Armbrust, Dr. Sabine Wessels, Dr. Karen Potthoff, Dr. Jan Oelmann, and Dr. Andrea Koffka for recruitment, study coordination, and data collection in the BEST study, and Lin Zielske, Annett Brendel, Marita Wenzel for technical support in the lab, and Werner Diehl for data management. Biospecimen storage was supported by the infrastructure of the NCT Liquid Biobank. We thank Isabell Adam for help with data analysis, Zeynep Nakipoglu for performing ELISA measurements of the urine samples and Alexander Schenk for editorial and graphical support.
References
1. Platten M, Knebel Doeberitz N, von Oezen I, Wick W, Ochs K. Cancer immunotherapy by targeting IDO1/TDO and their downstream effectors. Front Immunol. (2014) 5:673. doi: 10.3389/fimmu.2014.00673
2. Muller AJ, DuHadaway JB, Donover PS, Sutanto-Ward E, Prendergast GC. Inhibition of indoleamine 2,3-dioxygenase, an immunoregulatory target of the cancer suppression gene Bin1, potentiates cancer chemotherapy. Nat Med. (2005) 11:312–9. doi: 10.1038/nm1196
3. Opitz CA, Litzenburger UM, Sahm F, Ott M, Tritschler I, Trump S, et al. An endogenous tumour-promoting ligand of the human aryl hydrocarbon receptor. Nature. (2011) 478:197–203. doi: 10.1038/nature10491
4. Pilotte L, Larrieu P, Stroobant V, Colau D, Dolusic E, Frédérick R, et al. Reversal of tumoral immune resistance by inhibition of tryptophan 2,3-dioxygenase. Proc Natl Acad Sci USA. (2012) 109:2497–502. doi: 10.1073/pnas.1113873109
5. Smith C, Chang MY, Parker KH, Beury DW, DuHadaway JB, Flick HE, et al. IDO is a nodal pathogenic driver of lung cancer and metastasis development. Cancer Discov. (2012) 2:722–35. doi: 10.1158/2159-8290.CD-12-0014
6. Uyttenhove C, Pilotte L, Théate I, Stroobant V, Colau D, Parmentier N, et al. Evidence for a tumoral immune resistance mechanism based on tryptophan degradation by indoleamine 2,3-dioxygenase. Nat Med. (2003) 9:1269–74. doi: 10.1038/nm934
7. Huang A, Fuchs D, Widner B, Glover C, Henderson DC, Allen-Mersh TG. Serum tryptophan decrease correlates with immune activation and impaired quality of life in colorectal cancer. Br J Cancer. (2002) 86:1691–6. doi: 10.1038/sj.bjc.6600336
8. Chung DJ, Rossi M, Romano E, Ghith J, Yuan J, Munn DH, et al. Indoleamine 2,3-dioxygenase-expressing mature human monocyte-derived dendritic cells expand potent autologous regulatory T cells. Blood. (2009) 114:555–63. doi: 10.1182/blood-2008-11-191197
9. Frumento G, Rotondo R, Tonetti M, Damonte G, Benatti U, Ferrara GB. Tryptophan-derived catabolites are responsible for inhibition of T and natural killer cell proliferation induced by indoleamine 2,3-dioxygenase. J Exp Med. (2002) 196:459–68. doi: 10.1084/jem.20020121
10. Hwu P, Du MX, Lapointe R, Do M, Taylor MW, Young HA. Indoleamine 2,3-dioxygenase production by human dendritic cells results in the inhibition of T cell proliferation. J Immunol. (2000) 164:3596–9. doi: 10.4049/jimmunol.164.7.3596
11. Mondal A, Smith C, DuHadaway JB, Sutanto-Ward E, Prendergast GC, Bravo-Nuevo A, et al. IDO1 is an integral mediator of inflammatory neovascularization. EBioMedicine. (2016) 14:74–82. doi: 10.1016/j.ebiom.2016.11.013
12. Munn DH, Mellor AL. Indoleamine 2,3 dioxygenase and metabolic control of immune responses. Trends Immunol. (2013) 34:137–43. doi: 10.1016/j.it.2012.10.001
13. Munn DH, Shafizadeh E, Attwood JT, Bondarev I, Pashine A, Mellor AL. Inhibition of T cell proliferation by macrophage tryptophan catabolism. J Exp Med. (1999) 189:1363–72. doi: 10.1084/jem.189.9.1363
14. Wirthgen E, Hoeflich A, Rebl A, Günther J. Kynurenic acid: the Janus-faced role of an immunomodulatory tryptophan metabolite and its link to pathological conditions. Front Immunol. (2017) 8:1957. doi: 10.3389/fimmu.2017.01957
15. Cormie P, Zopf EM, Zhang X, Schmitz KH. The impact of exercise on cancer mortality, recurrence, and treatment-related adverse effects. Epidemiol Rev. (2017) 39:71–92. doi: 10.1093/epirev/mxx007
16. Hojman P. Exercise protects from cancer through regulation of immune function and inflammation. Biochem Soc Trans. (2017) 45:905–11. doi: 10.1042/BST20160466
17. Bessede A, Gargaro M, Pallotta MT, Matino D, Servillo G, Brunacci C, et al. Aryl hydrocarbon receptor control of a disease tolerance defence pathway. Nature. (2014) 511:184–90. doi: 10.1038/nature13323
18. Opitz CA, Wick W, Steinman L, Platten M. Tryptophan degradation in autoimmune diseases. Cell Mol Life Sci. (2007) 64:2542–63. doi: 10.1007/s00018-007-7140-9
19. Lovelace MD, Varney B, Sundaram G, Franco NF, Ng ML, Pai S, et al. Current evidence for a role of the kynurenine pathway of tryptophan metabolism in multiple sclerosis. Front Immunol. (2016) 7:246. doi: 10.3389/fimmu.2016.00246
20. Lovelace MD, Varney B, Sundaram G, Lennon MJ, Lim CK, Jacobs K, et al. Recent evidence for an expanded role of the kynurenine pathway of tryptophan metabolism in neurological diseases. Neuropharmacology. (2017) 112(Pt B):373–88. doi: 10.1016/j.neuropharm.2016.03.024
21. Ebede CC, Jang Y, Escalante CP. Cancer-related fatigue in cancer survivorship. Med Clin North Am. (2017) 101:1085–97. doi: 10.1016/j.mcna.2017.06.007
22. Wefel JS, Kesler SR, Noll KR, Schagen SB. Clinical characteristics, pathophysiology, and management of noncentral nervous system cancer-related cognitive impairment in adults. CA Cancer J Clin. (2015) 65:123–38. doi: 10.3322/caac.21258
23. Agudelo LZ, Femenía T, Orhan F, Porsmyr-Palmertz M, Goiny M, Martinez-Redondo V, et al. Skeletal muscle PGC-1α1 modulates kynurenine metabolism and mediates resilience to stress-induced depression. Cell. (2014) 159:33–45. doi: 10.1016/j.cell.2014.07.051
24. Serafini G, Adavastro G, Canepa G, Capobianco L, Conigliaro C, Pittaluga F, et al. Abnormalities in kynurenine pathway metabolism in treatment-resistant depression and suicidality: a systematic review. CNS Neurol Disord Drug Targets. (2017) 16:440–53. doi: 10.2174/1871527316666170413110605
25. Fukui S, Schwarcz R, Rapoport SI, Takada Y, Smith QR. Blood-brain barrier transport of kynurenines: implications for brain synthesis and metabolism. J Neurochem. (1991) 56:2007–17. doi: 10.1111/j.1471-4159.1991.tb03460.x
26. Dantzer R. Role of the kynurenine metabolism pathway in inflammation-induced depression: preclinical approaches. Curr Top Behav Neurosci. (2017) 31:117–38. doi: 10.1007/7854_2016_6
27. Schwarcz R, Stone TW. The kynurenine pathway and the brain: challenges, controversies and promises. Neuropharmacology. (2017) 112(Pt B):237–47. doi: 10.1016/j.neuropharm.2016.08.003
28. Schwarcz R, Bruno JP, Muchowski PJ, Wu H-Q. Kynurenines in the mammalian brain: when physiology meets pathology. Nat Rev Neurosci. (2012) 13:465–77. doi: 10.1038/nrn3257
29. Ahlskog JE, Geda YE, Graff-Radford NR, Petersen RC. Physical exercise as a preventive or disease-modifying treatment of dementia and brain aging. Mayo Clin Proc. (2011) 86:876–84. doi: 10.4065/mcp.2011.0252
30. Amara AW, Chahine L, Seedorff N, Caspell-Garcia CJ, Coffey C, Simuni T. Self-reported physical activity levels and clinical progression in early Parkinson's disease. Parkinsonism Relat Disord. (2018) 61:118–25. doi: 10.1016/j.parkreldis.2018.11.006
31. Andel R, Crowe M, Pedersen NL, Fratiglioni L, Johansson B, Gatz M. Physical exercise at midlife and risk of dementia three decades later: a population-based study of Swedish twins. J Gerontol Ser A. (2008) 63:62–6. doi: 10.1093/gerona/63.1.62
32. Riemenschneider M, Hvid LG, Stenager E, Dalgas U. Is there an overlooked “window of opportunity” in MS exercise therapy? Perspectives for early MS rehabilitation. Mult Scler. (2018) 24:886–94. doi: 10.1177/1352458518777377
33. Zimmer P, Baumann FT, Oberste M, Wright P, Garthe A, Schenk A, et al. Effects of exercise interventions and physical activity behavior on cancer related cognitive impairments: a systematic review. Biomed Res Int. (2016) 2016:1820954. doi: 10.1155/2016/1820954
34. Steindorf K, Schmidt ME, Klassen O, Ulrich CM, Oelmann J, Habermann N, et al. Randomized, controlled trial of resistance training in breast cancer patients receiving adjuvant radiotherapy: results on cancer-related fatigue and quality of life. Ann Oncol. (2014) 25:2237–43. doi: 10.1093/annonc/mdu374
35. Potthoff K, Schmidt ME, Wiskemann J, Hof H, Klassen O, Habermann N, et al. Randomized controlled trial to evaluate the effects of progressive resistance training compared to progressive muscle relaxation in breast cancer patients undergoing adjuvant radiotherapy: the BEST study. BMC Cancer. (2013) 13:162. doi: 10.1186/1471-2407-13-162
36. Wiskemann J, Schmidt ME, Klassen O, Debus J, Ulrich CM, Potthoff K, et al. Effects of 12-week resistance training during radiotherapy in breast cancer patients. Scand. J. Med. Sci. Sports. (2017) 27:1500–10. doi: 10.1111/sms.12777
37. Lyon DE, Walter JM, Starkweather AR, Schubert CM, McCain NL. Tryptophan degradation in women with breast cancer: a pilot study. BMC Res Notes. (2011) 4:156. doi: 10.1186/1756-0500-4-156
38. Masaki A, Ishida T, Maeda Y, Suzuki S, Ito A, Takino H, et al. Prognostic significance of tryptophan catabolism in adult T-cell leukemia/lymphoma. Clin Cancer Res. (2015) 21:2830–9. doi: 10.1158/1078-0432.CCR-14-2275
39. Herrstedt A, Marie LB, Simonsen C, Sundberg A, Egeland C, Thorsen-Streit S, et al. Exercise-mediated improvement of depression in patients with gstro-esophageal junction cancer is linked to kynurenine metabolism. Acta Oncol. (2019) 58:579–87. doi: 10.1080/0284186X.2018.1558371
40. Schröcksnadel K, Wirleitner B, Winkler C, Fuchs D. Monitoring tryptophan metabolism in chronic immune activation. Clin Chim Acta. (2006) 364:82–90. doi: 10.1016/j.cca.2005.06.013
41. Schmidt ME, Meynköhn A, Habermann N, Wiskemann J, Oelmann J, Hof H, et al. Resistance exercise and inflammation in breast cancer patients undergoing adjuvant radiation therapy: mediation analysis from a randomized, controlled intervention trial. Int J Radiat Oncol Biol Phys. (2016) 94:329–37. doi: 10.1016/j.ijrobp.2015.10.058
42. Fedewa MV, Hathaway ED, Ward-Ritacco CL. Effect of exercise training on C reactive protein: a systematic review and meta-analysis of randomised and non-randomised controlled trials. Br J Sports Med. (2017) 51:670–6. doi: 10.1136/bjsports-2016-095999
43. Beserra AHN, Kameda P, Deslandes AC, Schuch FB, Laks J, Moraes HS, et al. Can physical exercise modulate cortisol level in subjects with depression? A systematic review and meta-analysis. Trends Psychiatry Psychother. (2018) 40:360–8. doi: 10.1590/2237-6089-2017-0155
44. Greengard O, Feigelson P. A difference between the modes of action of substrate and hormonal inducers of rat liver tryptophan pyrrolase. Nature. (1961) 190:446–7. doi: 10.1038/190446a0
45. O'Leary CB, Hackney AC. Acute and chronic effects of resistance exercise on the testosterone and cortisol responses in obese males: a systematic review. Physiol Res. (2014) 63:693–704.
46. Fairey AS, Courneya KS, Field CJ, Bell GJ, Jones LW, Mackey JR. Randomized controlled trial of exercise and blood immune function in postmenopausal breast cancer survivors. J Appl Physiol. (2005) 98:1534–40. doi: 10.1152/japplphysiol.00566.2004
47. Weinhold M, Shimabukuro-Vornhagen A, Franke A, Theurich S, Wahl P, Hallek M, et al. Physical exercise modulates the homeostasis of human regulatory T cells. J Allergy Clin Immunol. (2016) 137:1607–10.e8. doi: 10.1016/j.jaci.2015.10.035
48. Mezrich JD, Fechner JH, Zhang X, Johnson BP, Burlingham WJ, Bradfield CA. An interaction between kynurenine and the aryl hydrocarbon receptor can generate regulatory T cells. J Immunol. (2010) 185:3190–8. doi: 10.4049/jimmunol.0903670
49. Bansi J, Koliamitra C, Bloch W, Joisten N, Schenk A, Watson M, et al. Persons with secondary progressive and relapsing remitting multiple sclerosis reveal different responses of tryptophan metabolism to acute endurance exercise and training. J Neuroimmunol. (2018) 314:101–5. doi: 10.1016/j.jneuroim.2017.12.001
50. Strasser B, Geiger D, Schauer M, Gatterer H, Burtscher M, Fuchs D. Effects of exhaustive aerobic exercise on tryptophan-kynurenine metabolism in trained athletes. PLoS ONE. (2016) 11:e0153617. doi: 10.1371/journal.pone.0153617
51. McClellan JL, Steiner JL, Day SD, Enos RT, Davis MJ, Singh UP, et al. Exercise effects on polyp burden and immune markers in the ApcMin/+ mouse model of intestinal tumorigenesis. Int J Oncol. (2014) 45:861–8. doi: 10.3892/ijo.2014.2457
52. Millischer V, Erhardt S, Ekblom Ö, Forsell Y, Lavebratt C. Twelve-week physical exercise does not have a long-lasting effect on kynurenines in plasma of depressed patients. Neuropsychiatr Dis Treat. (2017) 13:967–72. doi: 10.2147/NDT.S131746
53. Schlittler M, Goiny M, Agudelo LZ, Venckunas T, Brazaitis M, Skurvydas A, et al. Endurance exercise increases skeletal muscle kynurenine aminotransferases and plasma kynurenic acid in humans. Am J Physiol Cell Physiol. (2016) 310:C836–40. doi: 10.1152/ajpcell.00053.2016
54. Hilfiker R, Meichtry A, Eicher M, Nilsson Balfe L, Knols RH, Verra ML, et al. Exercise and other non-pharmaceutical interventions for cancer-related fatigue in patients during or after cancer treatment: a systematic review incorporating an indirect-comparisons meta-analysis. Br J Sports Med. (2018) 52:651–8. doi: 10.1136/bjsports-2016-096422
Keywords: exercise, physical activity, cancer, tryptophan, kynurenine, kynurenic acid, quinolinic acid
Citation: Zimmer P, Schmidt ME, Prentzell MT, Berdel B, Wiskemann J, Kellner KH, Debus J, Ulrich C, Opitz CA and Steindorf K (2019) Resistance Exercise Reduces Kynurenine Pathway Metabolites in Breast Cancer Patients Undergoing Radiotherapy. Front. Oncol. 9:962. doi: 10.3389/fonc.2019.00962
Received: 27 May 2019; Accepted: 11 September 2019;
Published: 25 September 2019.
Edited by:
Benjamin Frey, University of Erlangen Nuremberg, GermanyReviewed by:
Dietmar Fuchs, Innsbruck Medical University, AustriaAbel Santamaria, National Institute of Neurology and Neurosurgery (INNN), Mexico
Copyright © 2019 Zimmer, Schmidt, Prentzell, Berdel, Wiskemann, Kellner, Debus, Ulrich, Opitz and Steindorf. This is an open-access article distributed under the terms of the Creative Commons Attribution License (CC BY). The use, distribution or reproduction in other forums is permitted, provided the original author(s) and the copyright owner(s) are credited and that the original publication in this journal is cited, in accordance with accepted academic practice. No use, distribution or reproduction is permitted which does not comply with these terms.
*Correspondence: Philipp Zimmer, p.zimmer@dshs-koeln.de
†These authors share first authorship
‡These authors share last authorship