- 1State Key Laboratory of Oral Diseases & National Clinical Research Center for Oral Diseases, Department of Oral and Maxillofacial Surgery, West China Hospital of Stomatology, Sichuan University, Chengdu, China
- 2State Key Laboratory of Oral Diseases & National Clinical Research Center for Oral Diseases, Department of Oral Pathology, West China Hospital of Stomatology, Sichuan University, Chengdu, China
- 3State Key Laboratory of Microbial Technology, Shandong University, Qingdao, China
- 4Hubei Key Laboratory of Industrial Microbiology, Key Laboratory of Fermentation Engineering (Ministry of Education), Hubei Provincial Cooperative Innovation Center of Industrial Fermentation, Hubei University of Technology, Wuhan, China
Non-coding RNAs (ncRNAs), which do not encode proteins, have pivotal roles in manipulating gene expression in development, physiology, and pathology. Emerging data have shown that ncRNAs can regulate lymphangiogenesis, which refers to lymphatics deriving from preexisting vessels, becomes established during embryogenesis, and has a close relationship with pathological conditions such as lymphatic developmental diseases, inflammation, and cancer. This review summarizes the molecular mechanisms of lymphangiogenesis in lymphatic development, inflammation and cancer metastasis, and discusses ncRNAs' regulatory effects on them. Therapeutic targets with regard to lymphangiogenesis are also discussed.
Introduction
Lymphangiogenesis are termed lymphatics deriving from preexisting vessels, and generally progress through a number of stages: establishment of lymphatic endothelial cell (LEC) identity, formation of the primary lymphatic structures, maturation, and remodeling of the lymphatic vessels (1, 2). The normal growth and development of lymphatics contribute to their non-negligible roles in the cardiovascular system, including maintaining tissue fluid homeostasis, directing the trafficking of immune cells during immunosurveillance, and absorbing dietary lipids from the digestive tract (3, 4). However, in developmental diseases such as lymphedema, lymphangiectasia, and vascular malformations, or in inflammatory conditions such as infectious diseases, and after surgical intervention, lymphatic function is impaired which might lead to swellings and edema (5). In other pathological conditions, such as cancer, it might be essential to inhibit lymphangiogenesis, thus preventing cancer metastasis (6). Our comprehension of lymphangiogenesis regulation is mainly based on understanding the functions of proteins and their interactions, while it is widely known that only 3–5% of our genome encodes proteins and protein-target therapy may cause drug resistance (7). Therefore, the clinical regulation of lymphangiogenesis further requires other types of targets for successful intervention.
Currently, emerging studies have implicated connections between lymphangiogenesis and non-coding RNAs (ncRNAs), especially the well-known microRNAs (miRNAs), long non-coding RNAs (lncRNAs), and the newly discovered circular RNAs (circRNAs), which are transcribed from the vast majority of human genome. ncRNAs, though most do not encode proteins, contain genetic information or have functions in the biological process of cells. ncRNAs include structural RNAs such as tRNAs and rRNAs, which are abundant and have well-defined roles in translation, and regulatory RNAs such as miRNAs, lncRNAs, and circRNAs. These regulatory RNAs contain physiological and pathological functions, by altering protein expressions, interacting as signaling partners with specific proteins or acting as scaffolds for protein complexes to change signaling pathways (8, 9). Preclinical studies and increased success rates of ncRNA-target therapy provide a possibility of targeting ncRNAs in lymphangiogenesis-related disorders (10, 11).
Just as the old Chinese saying goes that “one stone with three birds,” understanding the underlying mechanisms important for ncRNAs targeting lymphangiogenesis in lymphatic developmental diseases, inflammation and cancer metastasis will help build new therapeutics when more than one disorder exists. Here, we review the molecular mechanisms of lymphangiogenesis in lymphatic development, inflammation and cancer metastasis, emphasize the ncRNAs' regulation on them, and hope to harness this knowledge for translational medicine.
Lymphatic Development
Lymphatic Development
In vertebrates, the first definitive sign of lymphatic development is the presence of endothelial cells with the expression of PROX1, considered to be the master regulator of LEC fate specification in cardinal veins (12, 13). Prox1 deletion in mice led to a complete absence of the lymphatic vasculature. PROX1-positive LECs bud, sprout and migrate away from both the cardinal and intersomitic veins to form the primary lymphatic plexus and sacs (14–16). Once exiting the veins, primitive LECs exhibit LEC identity including podoplanin, and increased levels of VEGFR-3 and NRP2 (14–16). This exiting process is absolutely dependent on VEGF-C, which acts via its tyrosine kinase receptor VEGFR-3 and the non-signaling co-receptor NRP2 (16–18). PROX1-positive LECs do not egress from the veins in embryos when deficient in key regulators of VEGF-C/VEGFR-3 signaling, such as collagen and calcium binding EGF domains 1 (CCBE1) (16, 19, 20). As for the initiation of PROX1 expression in venous endothelial cells, transcription factor SOX18 (21) and NR2F2/COUP-TFII (22) are thought to be critical. Intriguingly, PROX1 expression is induced in the cells of dorsolateral aspect of veins, while its inducer/co-regulator, SOX18, and COUP-TFII, are expressed throughout the cardinal vein endothelial cells (12, 23). Current explanation for this polarization involves retinoic acid signaling (24), Notch signaling (25) and BMP2 signaling pathway (26). They are all researched to regulate the emergence of lymphatic endothelial progenitor cells from the veins.
After LECs migrating and forming primary lymphatic vascular structures, major events involved in lymphatic development includes formation of lympho-venous valves, induction of platelet aggregation in valve regions, and remodeling of the initial lymphatic plexus to form a hierarchical lymphatic vascular tree (27, 28). Upregulation of FOXC2, together with high levels of PROX1 and GATA2, exist in the clusters of cells destined to form valves (29, 30). FOXC2 and PROX1 coordinately control expression of the gap junction protein connexin37 and activation of calcineurin/NFAT signaling, which are required for the assembly and delimitation of lymphatic valve territory during development (31). And cell surface molecules including the planar cell polarity pathway members, CELSR1 and VANGL2 (32), signaling pathways including Notch (33), BMP (34), and Semaphorin/Neuropilin/Plexin axes (35), and mechanical stimuli including disturbed flow are also important for valve development (31). As for platelet aggregation, signaling induced by podoplanin on the surface of LECs can bind to platelet C-type lectin-like receptor2 (CLEC2) to prevent blood entering into the lymphatics (27, 36). Both valves and platelet thrombi are crucial for separating the blood and lymphatic vascular compartments. In addition, signaling pathways such as angiopoietin/Tie signaling (37, 38), EphrinB2 signaling (39), and Reelin signaling (40), are significant for primitive lymphatic plexus remodeling to form initial and collecting vessels.
miRNAs and Lymphatic Development
miRNAs (19–24 nucleotides) are endogenous, non-protein-coding small RNAs that serve as post-transcriptional gene regulators (41, 42). According to the miRBase (version 21.0), over 60% of the protein-coding genes in human are targeted by miRNAs (43). Recent studies have defined the critical roles of miRNAs in lymphangiogenesis. Kazenwadel et al. demonstrated that miR-181a, the first discovered miRNA that targets PROX1, could bind to the Prox1 3′-UTR, resulting in translational inhibition and transcript degradation (44). Increased miR-181a in primary embryonic LECs led to the substantial reduced levels of PROX1 and resulted in reversion of LEC identity toward a blood vascular phenotype. Inhibition of endogenous miR-181a in blood endothelial cells (BECs) leads to elevated PROX1 expression, therefore promoting the acquisition of LEC identity. miR-31, as a novel BEC-specific miRNA, inhibited lymphatic lineage-specific differentiation in BECs, at least in part by repressing PROX1 in vitro, and impaired lymphatic development and venous sprouting in vivo (45). miR-31 candidate targets also include FOXC2, which is required for specification of lymphatic capillaries vs. collecting lymphatic vessels (46, 47), and RAMP2, which triggers lymphangiogenesis in response to adrenomedullin signaling (48). Recent evidence has shown that BMP2 signaling, the negative modulator for lymphatic fate during development, could promote both miR-181a and miR-31 in a SMAD-dependent manner, thus negatively regulating PROX1 expression at the post-transcriptional level. BMP2 signaling is therefore essential for constructing therapeutic manipulation of lymphangiogenesis in development (26).
miR-182, which is induced by JunB and attenuates FoxO1 expression in zebrafish, is crucial for the formation of parachordal lymphangioblasts and the thoracic duct. This JunB/miR-182/FoxO1 axis is regarded as a novel key player in governing lymphangiogenesis (49). A recent study has identified miR-126a as a director of LEC migration and lymphatic assembly. In vivo studies by Chen et al. reported that VEGF-C/FLT4 signaling acted as a cooperator of miR-126a, allowing modulation of lymphangiogenic sprout formation. miR-126a upregulated CXCL12a by targeting its 5′-UTR, then inducing chemokine signaling, resulting in parachordal lymphangioblast extension along a horizontal myoseptum (50, 51). Subsequent research confirmed miR-126 as a conserved modulator of lymphatic development in vivo and in vitro, and put forward the potential of miR-126 in preventing lymphedema, the most recognized aspect of lymphatic system malfunction as a result of genetic cause (52) (Figure 1).
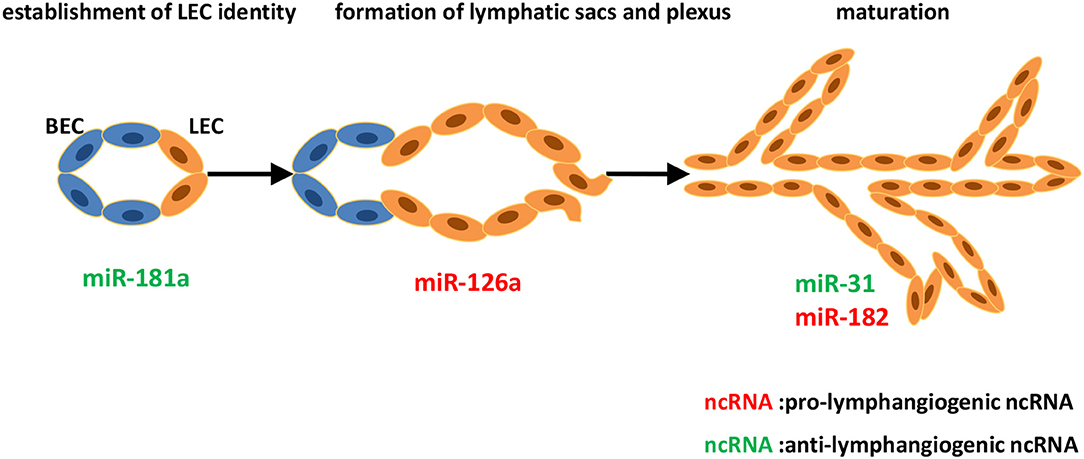
Figure 1. Identified ncRNAs regulating lymphangiogenesis in lymphatic development. The development of lymphatic vascular network starts with the cells of cardinal vein losing blood endothelial characteristics and acquiring lymphatic endothelial cell (LEC) identity. LECs then bud off the cardinal vein and form lymphatic sacs and plexus. Subsequently, remodeling of the primitive lymphatic vasculature begins, and becoming a hierarchical network. We described ncRNAs, mostly miRNAs identified to-date, which influence different steps of developmental lymphangiogenesis.
Inflammation
Lymphangiogenesis in Inflammation
Inflammation is a common feature of various conditions, characterized by pathological neovascularization, including hemangiogenesis and lymphangiogenesis. Hemangiogenesis refers to the new outgrowth from pre-existing blood vessels, and is an important pathological aspect of chronic inflammatory diseases (53). Lymphangiogenesis in inflammation is often induced by factors produced by macrophages and dendritic cells, and its existence involves tissue edema reduction, immune response initiation, and antigen clearance (54). Macrophages, especially CD11b+ macrophages, play a pivotal role in the inflammatory lymphangiogenesis mediated by VEGF ligands (55, 56). The VEGF family consists of five members that bind to and activate three distinct receptors. VEGF-A binds to VEGFR-1 and VEGFR-2; placental growth factor (PlGF) and VEGF-B bind only to VEGFR-1; and VEGF-C and VEGF-D bind to VEGFR-2 and VEGFR-3. Generally speaking, ligation of VEGF-A to VEGFR-2 induces only hemangiogenesis, while interactions of VEGF-C/D and VEGFR-3 mediate lymphangiogenesis (57–59). However, recent observations contradicted this notion and found that there was some crosstalk between them. VEGF-C produced by activated macrophages can induce local proliferating and sprouting of preexisting lymphatic cells (60, 61), while VEGF-A, expressed by activated leucocytes in inflammatory context, can recruit VEGFR-1-expressing macrophages, which are known to release VEGF-C/D, thus inducing inflammatory lymphangiogenesis (62). Maruyama et al. showed that VEGFR-3-expressing CD11b+ macrophages could directly transdifferentiate into lymphatic endothelial cells (LECs), forming cell aggregates that gradually developed into sprouting lymphatic vessels (63). In addition to the two ways of macrophages supporting lymphangiogenesis, dendritic cells activated by IL-1β and TNF-α in inflammation milieu can also migrate to lymphatic vessels, express VEGF-C, promoting lymphangiogenesis (64).
miRNAs and Inflammatory Lymphangiogenesis
The first example of the regulatory role of miRNA in inflammatory lymphangiogenesis is miR-1236, which is expressed in LECs and binds to VEGFR-3. Jones et al. demonstrated that miR-1236, induced by IL-1β, could negatively regulate VEGFR-3 expression and VEGFR-3-dependent signaling Akt and ERK1/2, and attenuate VEGF-C/VEGFR-3 mediated LECs migration and tube formation in primary human LECs in vitro. They also found that miR-1236 could reduce lymphangiogenesis in vivo (65, 66). Considering the fact that IL-1β contributes to initial lymphangiogenesis by inducing VEGFs and also induces miR-1236, which in turn suppresses VEGFR-3-dependent signaling, modulation of VEGFR-3 levels using miR-1236 may be a promising approach for the treatment of inflammatory diseases. Additionally, studies by Chakraborty et al. revealed that miR-9 expressed on inflamed LECs, which was induced by TNF-α, could inhibit NF-κB-mediated inflammation, increase VEGFR-3 and induce LEC proliferation and tube formation to activate VEGFR-3-mediated lymphangiogenesis (67). Studies concerning inflammatory lymphangiogenesis usually involved models of corneal lymphangiogenesis, as cornea exhibited alymphatic feature under normal condition and lymphangiogenesis under pathologic insults such as inflammation. miR-466, miR-184, and miR-199a/b-5p have all been reported to be significantly downregulated in corneal inflammatory lymphangiogenesis, and accordingly, inhibit corneal lymphatic growth in vivo and suppress LECs functions of adhesion, migration, and tube formation in vitro. This offers a clinical potential for lymphangiogenesis interference and lymphatic-related diseases treatment (68–70). Wang et al. revealed that miR-132 isolated from exosomes of VEGF-C-treated adipose-derived stem cells could be directly transferred to LECs and promote LECs proliferation, migration, and tube formation by targeting Smad-7 and activating TGF-β/Smad signaling, thus reversing acute to chronic inflammatory processes in inflammatory bowel disease (71).
Recently, circular RNA (circRNA) cZNF609 could serve as a sponge for miR-184 and subsequently elevated miR-184-target gene heparanase in inflamed corneas, which could significantly elevate VEGF-C expression and facilitate lymphangiogenesis in vitro and in vivo. However, whether cZNF609 intervention could act as a therapeutic strategy in preventing inflammation-induced lymphangiogenesis and treating ocular inflammatory diseases remains unknown, therefore requires further investigation (72) (Figure 2).
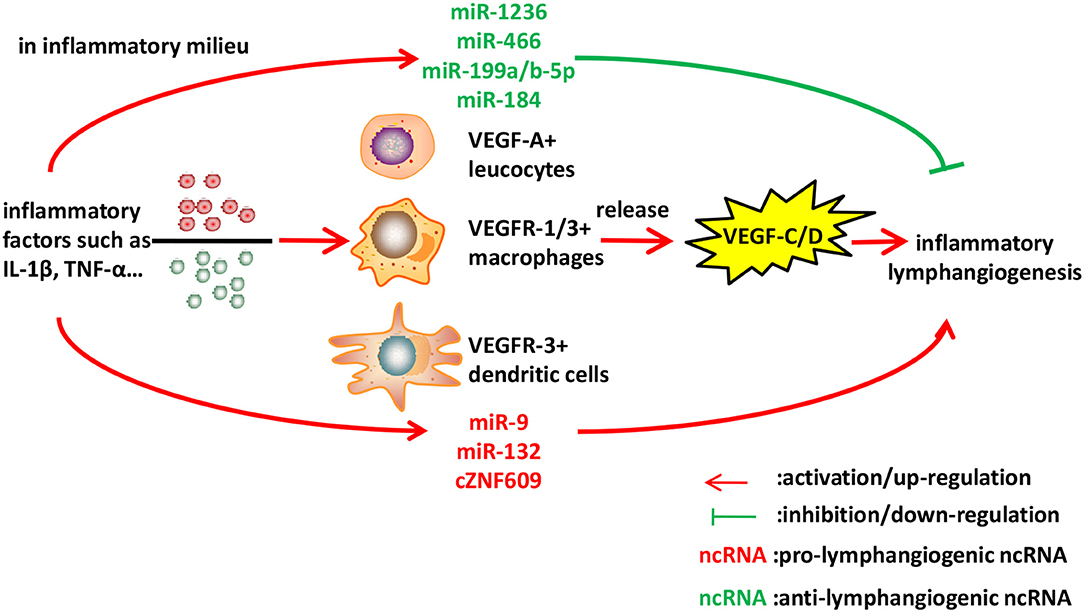
Figure 2. Identified ncRNAs regulating lymphangiogenesis in inflammation. Inflammatory cytokines such as IL-1β and TNF-α, stimulate macrophages, leucocytes, and dendritic cells to express VEGF-C/D, leading to inflammatory lymphangiogenesis. We described ncRNAs, mostly miRNAs identified to-date, which influence inflammatory lymphangiogenesis.
Cancer Metastasis
Lymphangiogenesis in Tumor
Cancer metastasis, the dissemination of cancer cells from primary tumors to distant organs, is considered to be the primary cause of cancer-related deaths. The majority of epithelial cancers firstly develop metastasis through spreading via lymphatic vessels (73). Tumor hypoxia microenvironment stimulates tumor cells, tumor stroma cells, and tumor-infiltrating inflammatory cells to express a series of lymphangiogenic factors, including the well-known VEGF family, especially VEGF-A/C/D (74), and other mediators such as PDGF-BB (75), IGF1/2 (76), FGF2 (77–79), HGF (80, 81), angiopoietin-2 (82), sphingosine-1-phosphate (83), adrenomedullin (84), and IL-7 (85, 86). In response to these factors, lymphangiogenesis can start from existing lymphatic vessels via sprouting, LEC proliferation, and formation of intra- and peri-tumoral lymphatics. Additionally, it can also derive from precursor LEC and bone marrow-derived cells (87, 88). After disseminating into sentinel lymph nodes (SLNs, the first tumor draining LN), lymphangiogenic factors induce LN lymphangiogenesis prior to the arrival of cancer cells. Besides inducing new lymphatic vessels, tumors can co-opt existing lymphatics at the primary site (73).
Crosstalk between tumors and lymphatic vessels are bidirectional. In addition to being influenced by tumors mentioned above, lymphatic vessels in return can contribute to cancer metastasis by secreting chemokines CCL21 (89) or CXCL12 (90), which bind to CCR7 or CXCR4 receptors, respectively, expressed in invading cancer cells, thus recruiting cancer cells toward lymphatic vessels. Lymphatic vessels can also provide a cancer stem cell niche (91) and modulate antitumor immune responses (92, 93), affecting metastatic tumor cells.
miRNAs and Lymphatic Metastasis
The most established lymphangiogenic factor, VEGF-C, can be targeted by miR-128 in human non-small cell lung cancer (NSCLC) cells and human umbilical vein endothelial cells (HUVECs). Hu et al. demonstrated that miR-128 could directly suppress VEGF-C and simultaneously decrease VEGF-A, VEGFR-2, and VEGFR-3 indirectly, thus reducing the phosphorylation of downstream VEGFR signaling pathways extracellular signal-regulated kinase (ERK1/2), phosphatidylinositol 3-kinase(AKT), and p38, resulting in tube formation inhibition in vitro. Furthermore, by analyzing immunohistochemical staining with anti-LYVE-1 antibodies of tumor tissues, they found out that miR-128 could suppress lymphangiogenesis of tumor xenografts in vivo, suggesting the therapeutic significance of miR-128 in NSCLC (94). VEGF-C can also be indirectly targeted by miR-206. Keklikoglou et al. revealed that, in pancreatic adenocarcinoma, miR-206 suppressed lymphangiogenesis through abrogating the expression of VEGF-C. Also, there was a striking reduction in the number of capillary-like tubes and intratumoral lymphatics coverage in the existence of miR-206, indicating that miR-206-based therapy might have important translational implications in pancreatic adenocarcinoma treatment (95). In chondrosarcoma, a series of studies have indicated that miR-381, miR-507, miR-27b, miR-624-3p, and miR-186 contributed to the inhibition of VEGF-C-dependent lymphangiogenesis with different mechanisms, all of which provided information on the potential miRNA-based molecular diagnosis and treatment for VEGF-C-mediated lymphangiogenesis in chondrosarcoma (96–100). In oral squamous cell carcinoma (OSCC) cells, Lin et al. found that decreased miR-300, which was suppressed by WNT1-inducible signaling pathway protein-1 (WISP-1), could contribute to VEGF-C-dependent lymphangiogenesis (101). And inhibited miR-195-3p, targeted by chemokine CCL4, could also induce VEGF-C and lymphangiogenesis in OSCC cells (102).
Besides VEGF-C, another member of VEGF family, VEGF-A, could induce lymphangiogenesis apart from angiogenesis, and accelerate nodal metastasis in OSCC (103). Research showed that miR-126 negatively regulated VEGF-A, and thus decreased lymphatic vessel density in OSCC specimens (104). Neuropilin-2 (NRP2), another important regulator of lymphangiogenesis, was directly suppressed by miR-486-5p in colorectal carcinoma cells, leading to the reduction of peritumoral lymphatic microvessels in vivo, and thus demonstrating the suppressor role of miR-486-5p in colorectal carcinoma (105). miR-93 was reported to inhibit angiogenesis and lymphangiogenesis by targeting angiopoietin2, and thus suppressed malignant pleural effusion, a sign of an advanced tumor stage (106).
Conversely, there were also pro-lymphangiogenetic miRNAs in cancer metastasis. miR-7 in gastric cancer cells promoted p65-mediated aberrant NF-κB activation and its downstream metastasis-related molecules including VEGF-C, and thus facilitated metastasis by alleviating hemangiogenesis, lymphangiogenesis, and inflammatory cells infiltration (107). miR-548k acted as a pro-lymphangiogenic miRNA in esophageal squamous cell carcinoma (ESCC) via promoting VEGF-C secretion and stimulating lymphangiogenesis, highlighting its crucial role as a new diagnostic and prognostic marker of ESCC (108). miR-27a could be induced in LECs by co-culturing with colon cancer cells, and promoted lymphangiogenesis via targeting SMAD4, a pivotal member of the TGF-β signaling and a tumor suppressor (109). Additionally, exosomes secreted from cancer cells could mediate lymphangiogenesis. A recent study showed that cervical squamous cell carcinoma (CSCC)-secreted exosomal miR-221-3p could transfer into LECs to promote lymphangiogenesis and lymphatic metastasis through downregulation of VASH1, representing a novel diagnostic biomarker and therapeutic target for metastatic CSCC patients in early stage (110). Furthermore, circulating miR-10b and miR-373 were shown to be potential biomarkers in detecting lymph node metastasis of breast cancer (111). There have been some studies focusing on miRNAs associated with lymphangiogenesis in various cancers, such as gastric cancer (112), lung cancer (113), and papillary thyroid cancer, of which the underlying mechanisms need to be further elucidated (114, 115).
LncRNAs and Lymphatic Metastasis
Although the functional roles of miRNAs in lymphangiogenesis are now established, relatively less is known about the regulatory roles of lncRNAs (>200 nts) (116, 117). Known as the “transcriptional noise,” lncRNAs rarely code for proteins, but are regulated like that of protein coding RNAs, being subjected to transcriptional regulation or even splicing (118, 119). Unlike miRNAs acting mainly as post-transcriptional repressors, functional lncRNAs can regulate gene expression at various levels, such as chromatin modification, transcriptional and post-transcriptional processing (120, 121). A number of findings have indicated the contribution of lncRNAs in cancer metastasis (122), while the question as to whether these lncRNAs are involved in lymphangiogenesis and lymph node metastasis is still being studied.
Recent evidence has shown that antisense non-coding RNA in the INK4 locus (ANRIL), a kind of lncRNA, induced lymphangiogenesis and lymphatic metastasis in colorectal cancer. ANRIL expression was correlated with the increased expressions of VEGF-C, VEGFR3, LYVE-1, and tube formation in both colorectal cancer cell lines and surgical specimens. ANRIL downregulation reduced lymphatic metastasis rate, lymphatic microvessel density (LMVD), and the expressions of VEGF-C, VEGFR3, LYVE-1, representing the potential role of ANRIL as a therapeutic target in colorectal cancer (123). In addition, a lncRNA termed Lymph Node Metastasis Associated Transcript 1 (LNMAT1), upregulated in lymph node-positive bladder cancer and associated with lymph node metastasis and prognosis, could epigenetically activate CCL2 expression and recruit macrophages into the tumor, which promoted lymphangiogenesis via VEGF-C secretion. LNMAT1 may represent a potential therapeutic target for clinical intervention in lymph node-metastatic bladder cancer (124). Other lymphangiogenesis-related lncRNAs still need further functional studies to verify their roles. For example, C21orF96 was overexpressed in positive lymph node and gastric cancer tissues, and promoted tubular formation in gastric cancer cell lines, while its pathogenesis was less well-characterized (125). MALAT-1 (126), UCA1 (127), HOTTIP (128), and HOTAIR (129) have all been proven to be associated with lymph node metastasis in various cancers and might serve as novel predictors, but well-designed studies are awaited to explain the mechanisms underlying it for uncovering better therapeutic strategies (Figure 3).
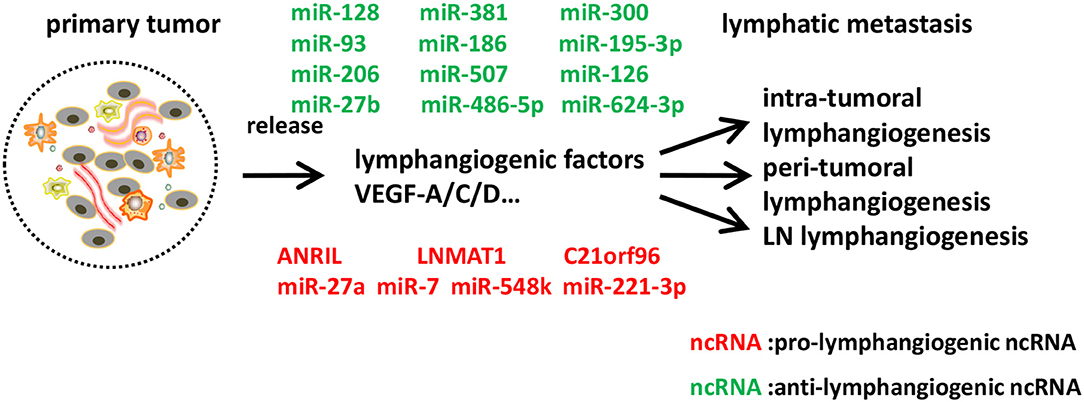
Figure 3. Identified ncRNAs regulating lymphangiogenesis in cancer metastasis. In tumor microenvironment, tumor cells, tumor stroma cells, and tumor-infiltrating inflammatory cells express a series of lymphangiogenic factors, and thus lead to tumor lymphangiogenesis and lymphatic metastasis. We described ncRNAs, mostly miRNAs identified to-date, which influence different steps of lymphangiogenesis in cancer metastasis.
Conclusions and Future Perspectives
In summary, ncRNAs, the dark matter of the genome, account for >80% of total mature RNA and have many crucial, but as yet, undefined roles in regulating lymphangiogenesis concerning lymphatic developmental disorders, inflammatory diseases, and cancer metastasis (130). The two major types of regulatory ncRNAs, miRNAs, and lncRNAs, modulate inter-related steps and mediators of lymphangiogenesis, therefore exert their influence on lymphatic developmental disorders, inflammatory diseases or cancer metastasis, if not all of them. The identification of key pro-lymphangiogenic and anti-lymphangiogenic ncRNAs is currently the aim of investigation and will underpin the generation of novel therapeutic targets, as well as potential targets on diagnosis, prognosis and response prediction (Table 1).
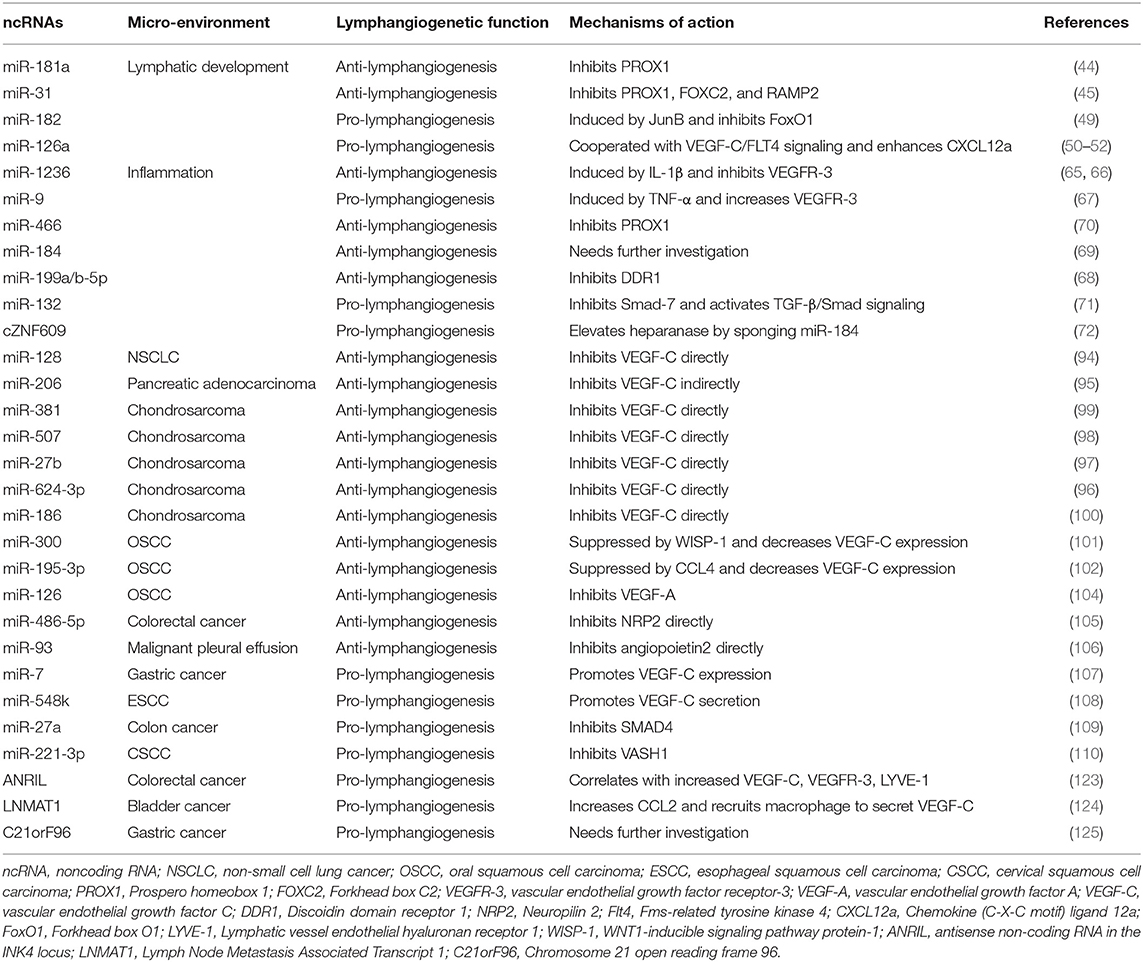
Table 1. ncRNAs that mediate lymphangiogenesis in lymphatic development, inflammation, and cancer metastasis.
The clinical potential function of ncRNAs as new targets has been carried out. For example, antisense oligonucleotide therapy can be applied to correct aberrant splicing (131, 132); via replacing or inhibiting ncRNAs especially miRNAs, affecting levels or functions of ncRNAs (133). Loss of MALAT1 with antisense oligonucleotide provided a potential therapeutic approach to prevent lung cancer metastasis via regulating gene expression, but not alternative splicing (134, 135). Though breakthroughs in targeted therapy have involved ncRNAs, major challenges exist with limited examples and acquired resistance.
Lymphangiogenesis has the potential to become the therapeutic target (44), since lymphatic vessels are mostly quiescent in adults and LEC identity is more plastic during adulthood than during embryo. However, some problems still need to be addressed. Interfering with tumor lymphangiogenesis can decrease or prevent lymphatic metastasis through blocking lymphatic drainage, while it may also lead to tissue fluid accumulation and cause lymphedema. Is there a target to solve both the metastasis and lymphedema? Furthermore, in a radical operation that resects primary or metastatic tumor, concurrent anti-lymphangiogenesis therapy could postpone wound healing, as lymphangiogenesis does good to inflammation in some conditions (136). That necessitates the identification of tumor lymphatics-specific markers. Recently, therapies aimed at blocking the VEGF-C/VEGF-D/VEGFR-3 signaling axis have entered clinical trials in some types of tumors, while these could not block metastasis completely (137, 138). Olmeda et al. have reported that tumors actually could induce lymphangiogenesis in distant organs rather than just SLNs before tumor cells colonization by secreting MIDKINE, and the molecular profiles and functions of LECs in distant and local lymphatic vessels might vary depending on which tissue they were in Olmeda et al. (139). As ncRNAs also have tissue-specificity expressions, their serving as a target to orchestrate lymphangiogenesis, even in more than one pathological conditions when required, is worth validation.
Author Contributions
MC, YT, Y-JT, and XL designed the subject of the review. MC and Y-JT were involved in material collection. MC, YT, and WZ were responsible for writing of the manuscript. Y-JT, WZ, and XL were responsible for figure drawing. All authors reviewed the manuscript.
Funding
This work was supported by National Natural Science Foundation of China grants (Nos. 81572650, 81672672, 81972542, and 81772891), and by State Key Laboratory of Oral Diseases Special Funded Projects.
Conflict of Interest Statement
The authors declare that the research was conducted in the absence of any commercial or financial relationships that could be construed as a potential conflict of interest.
Abbreviations
ncRNA, non-coding RNA; PROX1, Prospero homeobox 1; BMP2, bone morphogenetic protein 2; FOXC2, Forkhead box C2; IL-1β, Interleukine-1 beta; VEGFR-3, vascular endothelial growth factor receptor-3; NSCLC, non-small cell lung cancer; VEGF-A, vascular endothelial growth factor A; VEGF-C, vascular endothelial growth factor C; NF-κB, nuclear transcription factor-κB; OSCC, oral squamous cell carcinoma; NRP2, Neuropilin 2; Flt4, Fms-related tyrosine kinase 4; CXCL12a, Chemokine (C-X-C motif) ligand 12a; FoxO1, Forkhead box O1; TNF-α, Tumor Necrosis Factor alpha; LYVE-1, Lymphatic vessel endothelial hyaluronan receptor 1; WISP-1, WNT1-inducible signaling pathway protein-1; ANRIL, antisense non-coding RNA in the INK4 locus; C21orF96, Chromosome 21 open reading frame 96.
References
1. Kazenwadel J, Harvey NL. Morphogenesis of the lymphatic vasculature: a focus on new progenitors and cellular mechanisms important for constructing lymphatic vessels. Dev Dyn. (2016) 245:209–19. doi: 10.1002/dvdy.24313
2. Albrecht I, Christofori G. Molecular mechanisms of lymphangiogenesis in development and cancer. Int J Dev Biol. (2011) 55:483–94. doi: 10.1387/ijdb.103226ia
3. Koltowska K, Betterman KL, Harvey NL, Hogan BM. Getting out and about: the emergence and morphogenesis of the vertebrate lymphatic vasculature. Development. (2013) 140:1857–70. doi: 10.1242/dev.089565
4. Schulte-Merker S, Sabine A, Petrova TV. Lymphatic vascular morphogenesis in development, physiology, and disease. J Cell Biol. (2011) 193:607–18. doi: 10.1083/jcb.201012094
5. Witte MH, Bernas MJ, Martin CP, Witte CL. Lymphangiogenesis and lymphangiodysplasia: from molecular to clinical lymphology. Microsc Res Techniq.(2001) 55:122–45. doi: 10.1002/jemt.1163.abs
6. Tammela T, Alitalo K. Lymphangiogenesis: molecular mechanisms and future promise. Cell. (2010) 140:460–76. doi: 10.1016/j.cell.2010.01.045
7. Edwards SL, Beesley J, French JD, Dunning AM. Beyond GWASs: illuminating the dark road from association to function. Am J Hum Genet. (2013) 93:779–97. doi: 10.1016/j.ajhg.2013.10.012
8. Rupaimoole R, Slack FJ. MicroRNA therapeutics: towards a new era for the management of cancer and other diseases. Nat Rev Drug Discov. (2017) 16:203–22. doi: 10.1038/nrd.2016.246
9. Guttman M, Rinn JL. Modular regulatory principles of large non-coding RNAs. Nature. (2012) 482:339–46. doi: 10.1038/nature10887
10. Matsui M, Corey DR. Non-coding RNAs as drug targets. Nat Rev Drug Discov. (2017) 16:167–79. doi: 10.1038/nrd.2016.117
11. Bader AG. miR-34 - a microRNA replacement therapy is headed to the clinic. Front Genet. (2012) 3:120. doi: 10.3389/fgene.2012.00120
12. Wigle JT, Oliver G. Prox1 function is required for the development of the murine lymphatic system. Cell. (1999) 98:769–78. doi: 10.1016/S0092-8674(00)81511-1
13. Wigle JT, Harvey N, Detmar M, Lagutina I, Grosveld G, Gunn MD, et al. An essential role for Prox1 in the induction of the lymphatic endothelial cell phenotype. EMBO J. (2002) 21:1505–13. doi: 10.1093/emboj/21.7.1505
14. Francois M, Short K, Secker GA, Combes A, Schwarz Q, Davidson TL, et al. Segmental territories along the cardinal veins generate lymph sacs via a ballooning mechanism during embryonic lymphangiogenesis in mice. Dev Biol. (2012) 364:89–98. doi: 10.1016/j.ydbio.2011.12.032
15. Yang Y, Garcia-Verdugo JM, Soriano-Navarro M, Srinivasan RS, Scallan JP, Singh MK, et al. Lymphatic endothelial progenitors bud from the cardinal vein and intersomitic vessels in mammalian embryos. Blood. (2012) 120:2340–8. doi: 10.1182/blood-2012-05-428607
16. Hagerling R, Pollmann C, Andreas M, Schmidt C, Nurmi H, Adams RH, et al. A novel multistep mechanism for initial lymphangiogenesis in mouse embryos based on ultramicroscopy. EMBO J. (2013) 32:629–44. doi: 10.1038/emboj.2012.340
17. Karkkainen MJ, Haiko P, Sainio K, Partanen J, Taipale J, Petrova TV, et al. Vascular endothelial growth factor C is required for sprouting of the first lymphatic vessels from embryonic veins. Nat Immunol. (2004) 5:74–80. doi: 10.1038/ni1013
18. Secker GA, Harvey NL. VEGFR signaling during lymphatic vascular development: from progenitor cells to functional vessels. Dev Dyn. (2015) 244:323–31. doi: 10.1002/dvdy.24227
19. Hogan BM, Bos FL, Bussmann J, Witte M, Chi NC, Duckers HJ, et al. Ccbe1 is required for embryonic lymphangiogenesis and venous sprouting. Nat Genet. (2009) 41:396–8. doi: 10.1038/ng.321
20. Bos FL, Caunt M, Peterson-Maduro J, Planas-Paz L, Kowalski J, Karpanen T, et al. CCBE1 is essential for mammalian lymphatic vascular development and enhances the lymphangiogenic effect of vascular endothelial growth factor-C in vivo. Circulat Res. (2011) 109:486–91. doi: 10.1161/CIRCRESAHA.111.250738
21. Francois M, Caprini A, Hosking B, Orsenigo F, Wilhelm D, Browne C, et al. Sox18 induces development of the lymphatic vasculature in mice. Nature. (2008) 456:643–7. doi: 10.1038/nature07391
22. Srinivasan RS, Geng X, Yang Y, Wang Y, Mukatira S, Studer M, et al. The nuclear hormone receptor Coup-TFII is required for the initiation and early maintenance of Prox1 expression in lymphatic endothelial cells. Genes Dev. (2010) 24:696–707. doi: 10.1101/gad.1859310
23. van Impel A, Zhao Z, Hermkens DM, Roukens MG, Fischer JC, Peterson-Maduro J, et al. Divergence of zebrafish and mouse lymphatic cell fate specification pathways. Development. (2014) 141:1228–38. doi: 10.1242/dev.105031
24. Bowles J, Secker G, Nguyen C, Kazenwadel J, Truong V, Frampton E, et al. Control of retinoid levels by CYP26B1 is important for lymphatic vascular development in the mouse embryo. Dev Biol. (2014) 386:25–33. doi: 10.1016/j.ydbio.2013.12.008
25. Murtomaki A, Uh MK, Choi YK, Kitajewski C, Borisenko V, Kitajewski J, et al. Notch1 functions as a negative regulator of lymphatic endothelial cell differentiation in the venous endothelium. Development. (2013) 140:2365–76. doi: 10.1242/dev.083865
26. Dunworth WP, Cardona-Costa J, Bozkulak EC, Kim JD, Meadows S, Fischer JC, et al. Bone morphogenetic protein 2 signaling negatively modulates lymphatic development in vertebrate embryos. Circulat Res. (2014) 114:56–66. doi: 10.1161/CIRCRESAHA.114.302452
27. Hess PR, Rawnsley DR, Jakus Z, Yang Y, Sweet DT, Fu J, et al. Platelets mediate lymphovenous hemostasis to maintain blood-lymphatic separation throughout life. J Clin Invest. (2014) 124:273–84. doi: 10.1172/JCI70422
28. Srinivasan RS, Oliver G. Prox1 dosage controls the number of lymphatic endothelial cell progenitors and the formation of the lymphovenous valves. Genes Dev. (2011) 25:2187–97. doi: 10.1101/gad.16974811
29. Kazenwadel J, Secker GA, Liu YJ, Rosenfeld JA, Wildin RS, Cuellar-Rodriguez J, et al. Loss-of-function germline GATA2 mutations in patients with MDS/AML or MonoMAC syndrome and primary lymphedema reveal a key role for GATA2 in the lymphatic vasculature. Blood. (2012) 119:1283–91. doi: 10.1182/blood-2011-08-374363
30. Norrmen C, Ivanov KI, Cheng J, Zangger N, Delorenzi M, Jaquet M, et al. FOXC2 controls formation and maturation of lymphatic collecting vessels through cooperation with NFATc1. J Cell Biol. (2009) 185:439–57. doi: 10.1083/jcb.200901104
31. Sabine A, Agalarov Y, Maby-El Hajjami H, Jaquet M, Hagerling R, Pollmann C, et al. Mechanotransduction, PROX1, and FOXC2 cooperate to control connexin37 and calcineurin during lymphatic-valve formation. Dev Cell. (2012) 22:430–45. doi: 10.1016/j.devcel.2011.12.020
32. Tatin F, Taddei A, Weston A, Fuchs E, Devenport D, Tissir F, et al. Planar cell polarity protein Celsr1 regulates endothelial adherens junctions and directed cell rearrangements during valve morphogenesis. Dev Cell. (2013) 26:31–44. doi: 10.1016/j.devcel.2013.05.015
33. Murtomaki A, Uh MK, Kitajewski C, Zhao J, Nagasaki T, Shawber CJ, et al. Notch signaling functions in lymphatic valve formation. Development. (2014) 141:2446–51. doi: 10.1242/dev.101188
34. Levet S, Ciais D, Merdzhanova G, Mallet C, Zimmers TA, Lee SJ, et al. Bone morphogenetic protein 9 (BMP9) controls lymphatic vessel maturation and valve formation. Blood. (2013) 122:598–607. doi: 10.1182/blood-2012-12-472142
35. Bouvree K, Brunet I, Del Toro R, Gordon E, Prahst C, Cristofaro B, et al. Semaphorin3A, Neuropilin-1, and PlexinA1 are required for lymphatic valve formation. Circulat Res. (2012) 111:437–45. doi: 10.1161/CIRCRESAHA.112.269316
36. Debrincat MA, Josefsson EC, James C, Henley KJ, Ellis S, Lebois M, et al. Mcl-1 and Bcl-x(L) coordinately regulate megakaryocyte survival. Blood. (2012) 119:5850–8. doi: 10.1182/blood-2011-12-398834
37. Dellinger M, Hunter R, Bernas M, Gale N, Yancopoulos G, Erickson R, et al. Defective remodeling and maturation of the lymphatic vasculature in Angiopoietin-2 deficient mice. Dev Biol. (2008) 319:309–20. doi: 10.1016/j.ydbio.2008.04.024
38. Qu X, Tompkins K, Batts LE, Puri M, Baldwin HS. Abnormal embryonic lymphatic vessel development in Tie1 hypomorphic mice. Development. (2010) 137:1285–95. doi: 10.1242/dev.043380
39. Zhang G, Brady J, Liang WC, Wu Y, Henkemeyer M, Yan M. EphB4 forward signalling regulates lymphatic valve development. Nat Commun. (2015) 6:6625. doi: 10.1038/ncomms7625
40. Lutter S, Xie S, Tatin F, Makinen T. Smooth muscle-endothelial cell communication activates Reelin signaling and regulates lymphatic vessel formation. J Cell Biol. (2012) 197:837–49. doi: 10.1083/jcb.201110132
41. Ling H, Fabbri M, Calin GA. MicroRNAs and other non-coding RNAs as targets for anticancer drug development. Nat Rev Drug Discov. (2013) 12:847–65. doi: 10.1038/nrd4140
42. Cao MX, Jiang YP, Tang YL, Liang XH. The crosstalk between lncRNA and microRNA in cancer metastasis: orchestrating the epithelial-mesenchymal plasticity. Oncotarget. (2016) 8: 12472–83. doi: 10.18632/oncotarget.13957
43. Kozomara A, Griffiths-Jones S. miRBase: annotating high confidence microRNAs using deep sequencing data. Nucleic Acids Res. (2014) 42:D68–73. doi: 10.1093/nar/gkt1181
44. Kazenwadel J, Michael MZ, Harvey NL. Prox1 expression is negatively regulated by miR-181 in endothelial cells. Blood. (2010) 116:2395–401. doi: 10.1182/blood-2009-12-256297
45. Pedrioli DM, Karpanen T, Dabouras V, Jurisic G, van de Hoek G, Shin JW, et al. miR-31 functions as a negative regulator of lymphatic vascular lineage-specific differentiation in vitro and vascular development in vivo. Mol Cell Biol. (2010) 30:3620–34. doi: 10.1128/MCB.00185-10
46. Adams RH, Alitalo K. Molecular regulation of angiogenesis and lymphangiogenesis. Nat Rev Mol Cell Biol. (2007) 8:464–78. doi: 10.1038/nrm2183
47. Cueni LN, Detmar M. New insights into the molecular control of the lymphatic vascular system and its role in disease. J Invest Dermatol. (2006) 126:2167–77. doi: 10.1038/sj.jid.5700464
48. Fritz-Six KL, Dunworth WP, Li M, Caron KM. Adrenomedullin signaling is necessary for murine lymphatic vascular development. J Clin Invest. (2008) 118:40–50. doi: 10.1172/JCI33302
49. Kiesow K, Bennewitz K, Miranda LG, Stoll SJ, Hartenstein B, Angel P, et al. Junb controls lymphatic vascular development in zebrafish via miR-182. Sci Rep. (2015) 5:15007. doi: 10.1038/srep15007
50. Chen J, Zhu RF, Li FF, Liang YL, Wang C, Qin YW, et al. MicroRNA-126a directs lymphangiogenesis through interacting with chemokine and Flt4 Signaling in Zebrafish. Arterioscler Thromb Vasc Biol. (2016) 36:2381–93. doi: 10.1161/ATVBAHA.116.308120
51. Cha YR, Fujita M, Butler M, Isogai S, Kochhan E, Siekmann AF, et al. Chemokine signaling directs trunk lymphatic network formation along the preexisting blood vasculature. Dev Cell. (2012) 22:824–36. doi: 10.1016/j.devcel.2012.01.011
52. Kontarakis Z, Rossi A, Ramas S, Dellinger MT, Stainier DYR. Mir-126 is a conserved modulator of lymphatic development. Dev Biol. (2018) 437:120–30. doi: 10.1016/j.ydbio.2018.03.006
53. Folkman J. Angiogenesis in cancer, vascular, rheumatoid and other disease. Nat Med. (1995) 1:27–31. doi: 10.1038/nm0195-27
54. Martinez-Corral I, Olmeda D, Dieguez-Hurtado R, Tammela T, Alitalo K, Ortega S. In vivo imaging of lymphatic vessels in development, wound healing, inflammation, and tumor metastasis. Proc Natl Acad Sci USA. (2012) 109:6223–8. doi: 10.1073/pnas.1115542109
55. Kerjaschki D, Regele HM, Moosberger I, Nagy-Bojarski K, Watschinger B, Soleiman A, et al. Lymphatic neoangiogenesis in human kidney transplants is associated with immunologically active lymphocytic infiltrates. J Am Soc Nephrol. (2004) 15:603–12. doi: 10.1097/01.ASN.0000113316.52371.2E
56. Skobe M, Hamberg LM, Hawighorst T, Schirner M, Wolf GL, Alitalo K, et al. Concurrent induction of lymphangiogenesis, angiogenesis, and macrophage recruitment by vascular endothelial growth factor-C in melanoma. Am J Pathol. (2001) 159:893–903. doi: 10.1016/S0002-9440(10)61765-8
57. Kerbel R, Folkman J. Clinical translation of angiogenesis inhibitors. Nat Rev Cancer. (2002) 2:727–39. doi: 10.1038/nrc905
58. Stacker SA, Achen MG, Jussila L, Baldwin ME, Alitalo K. Lymphangiogenesis and cancer metastasis. Nat Rev Cancer. (2002) 2:573–83. doi: 10.1038/nrc863
59. Carmeliet P. Angiogenesis in health and disease. Nat Med. (2003) 9:653–60. doi: 10.1038/nm0603-653
60. Kerjaschki D. The crucial role of macrophages in lymphangiogenesis. J Clin Invest. (2005) 115:2316–9. doi: 10.1172/JCI26354
61. Kataru RP, Jung K, Jang C, Yang H, Schwendener RA, Baik JE, et al. Critical role of CD11b+ macrophages and VEGF in inflammatory lymphangiogenesis, antigen clearance, and inflammation resolution. Blood. (2009) 113:5650–9. doi: 10.1182/blood-2008-09-176776
62. Cursiefen C, Chen L, Borges LP, Jackson D, Cao J, Radziejewski C, et al. VEGF-A stimulates lymphangiogenesis and hemangiogenesis in inflammatory neovascularization via macrophage recruitment. J Clin Invest. (2004) 113:1040–50. doi: 10.1172/JCI20465
63. Maruyama K, Ii M, Cursiefen C, Jackson DG, Keino H, Tomita M, et al. Inflammation-induced lymphangiogenesis in the cornea arises from CD11b-positive macrophages. J Clin Invest. (2005) 115:2363–72. doi: 10.1172/JCI23874
64. Baluk P, Tammela T, Ator E, Lyubynska N, Achen MG, Hicklin DJ, et al. Pathogenesis of persistent lymphatic vessel hyperplasia in chronic airway inflammation. J Clin Invest. (2005) 115:247–57. doi: 10.1172/JCI22037
65. Jones D, Li Y, He Y, Xu Z, Chen H, Min W. Mirtron microRNA-1236 inhibits VEGFR-3 signaling during inflammatory lymphangiogenesis. Arterioscler Thromb Vasc Biol. (2012) 32:633–42. doi: 10.1161/ATVBAHA.111.243576
66. Huggenberger R, Siddiqui SS, Brander D, Ullmann S, Zimmermann K, Antsiferova M, et al. An important role of lymphatic vessel activation in limiting acute inflammation. Blood. (2011) 117:4667–78. doi: 10.1182/blood-2010-10-316356
67. Chakraborty S, Zawieja DC, Davis MJ, Muthuchamy M. MicroRNA signature of inflamed lymphatic endothelium and role of miR-9 in lymphangiogenesis and inflammation. Am J Physiol Cell Physiol. (2015) 309:C680–92. doi: 10.1152/ajpcell.00122.2015
68. Oh S, Seo M, Choi JS, Joo CK, Lee SK. MiR-199a/b-5p inhibits lymphangiogenesis by targeting discoidin domain receptor 1 in corneal injury. Molecules Cells. (2018) 41:93–102. doi: 10.14348/molcells.2018.2163
69. Grimaldo S, Yuen D, Theis J, Ng M, Ecoiffier T, Chen L. MicroRNA-184 regulates corneal lymphangiogenesis. Invest Ophthalmol Vis Sci. (2015) 56:7209–13. doi: 10.1167/iovs.15-17733
70. Seo M, Choi JS, Rho CR, Joo CK, Lee SK. MicroRNA miR-466 inhibits lymphangiogenesis by targeting prospero-related homeobox 1 in the alkali burn corneal injury model. J Biomed Sci. (2015) 22:3. doi: 10.1186/s12929-014-0104-0
71. Wang X, Wang H, Cao J, Ye C. Exosomes from Adipose-Derived Stem Cells Promotes VEGF-C-Dependent Lymphangiogenesis by Regulating miRNA-132/TGF-beta Pathway. Cell Physiol Biochem. (2018) 49:160–71. doi: 10.1159/000492851
72. Lin T, Zhang X, Lu Y, Gong L. Identification of circular RNA related to inflammation-induced lymphangiogenesis by microarray analysis. DNA Cell Biol. (2019) 38:887–94. doi: 10.1089/dna.2018.4590
73. Karaman S, Detmar M. Mechanisms of lymphatic metastasis. J Clin Invest. (2014) 124:922–8. doi: 10.1172/JCI71606
74. Wang J, Huang Y, Zhang J, Wei Y, Mahoud S, Bakheet AM, et al. Pathway-related molecules of VEGFC/D-VEGFR3/NRP2 axis in tumor lymphangiogenesis and lymphatic metastasis. Clin Chim Acta. (2016) 461:165–71. doi: 10.1016/j.cca.2016.08.008
75. Cao R, Bjorndahl MA, Religa P, Clasper S, Garvin S, Galter D, et al. PDGF-BB induces intratumoral lymphangiogenesis and promotes lymphatic metastasis. Cancer Cell. (2004) 6:333–45. doi: 10.1016/j.ccr.2004.08.034
76. Bjorndahl M, Cao R, Nissen LJ, Clasper S, Johnson LA, Xue Y, et al. Insulin-like growth factors 1 and 2 induce lymphangiogenesis in vivo. Proc Natl Acad Sci USA. (2005) 102:15593–8. doi: 10.1073/pnas.0507865102
77. Shin JW, Min M, Larrieu-Lahargue F, Canron X, Kunstfeld R, Nguyen L, et al. Prox1 promotes lineage-specific expression of fibroblast growth factor (FGF) receptor-3 in lymphatic endothelium: a role for FGF signaling in lymphangiogenesis. Mol Biol Cell. (2006) 17:576–84. doi: 10.1091/mbc.e05-04-0368
78. Schulz MM, Reisen F, Zgraggen S, Fischer S, Yuen D, Kang GJ, et al. Phenotype-based high-content chemical library screening identifies statins as inhibitors of In vivo lymphangiogenesis. Proc Natl Acad Sci USA. (2012) 109:E2665–74. doi: 10.1073/pnas.1206036109
79. Platonova N, Miquel G, Regenfuss B, Taouji S, Cursiefen C, Chevet E, et al. Evidence for the interaction of fibroblast growth factor-2 with the lymphatic endothelial cell marker LYVE-1. Blood. (2013) 121:1229–37. doi: 10.1182/blood-2012-08-450502
80. Kajiya K, Hirakawa S, Ma B, Drinnenberg I, Detmar M. Hepatocyte growth factor promotes lymphatic vessel formation and function. EMBO J. (2005) 24:2885–95. doi: 10.1038/sj.emboj.7600763
81. Cao R, Bjorndahl MA, Gallego MI, Chen S, Religa P, Hansen AJ, et al. Hepatocyte growth factor is a lymphangiogenic factor with an indirect mechanism of action. Blood. (2006) 107:3531–6. doi: 10.1182/blood-2005-06-2538
82. Holopainen T, Saharinen P, D'Amico G, Lampinen A, Eklund L, Sormunen R, et al. Effects of angiopoietin-2-blocking antibody on endothelial cell-cell junctions and lung metastasis. J Natl Cancer Instit. (2012) 104:461–75. doi: 10.1093/jnci/djs009
83. Nagahashi M, Ramachandran S, Kim EY, Allegood JC, Rashid OM, Yamada A, et al. Sphingosine-1-phosphate produced by sphingosine kinase 1 promotes breast cancer progression by stimulating angiogenesis and lymphangiogenesis. Cancer Res. (2012) 72:726–35. doi: 10.1158/0008-5472.CAN-11-2167
84. Karpinich NO, Kechele DO, Espenschied ST, Willcockson HH, Fedoriw Y, Caron KM. Adrenomedullin gene dosage correlates with tumor and lymph node lymphangiogenesis. FASEB J. (2013) 27:590–600. doi: 10.1096/fj.12-214080
85. Iolyeva M, Aebischer D, Proulx ST, Willrodt AH, Ecoiffier T, Haner S, et al. Interleukin-7 is produced by afferent lymphatic vessels and supports lymphatic drainage. Blood. (2013) 122:2271–81. doi: 10.1182/blood-2013-01-478073
86. Jian M, Qingfu Z, Yanduo J, Guocheng J, Xueshan Q. Anti-lymphangiogenesis effects of a specific anti-interleukin 7 receptor antibody in lung cancer model in vivo. Mol Carcinogen. (2015) 54:148–55. doi: 10.1002/mc.22082
87. Jiang S, Bailey AS, Goldman DC, Swain JR, Wong MH, Streeter PR, et al. Hematopoietic stem cells contribute to lymphatic endothelium. PLoS ONE. (2008) 3:e3812. doi: 10.1371/journal.pone.0003812
88. Zumsteg A, Baeriswyl V, Imaizumi N, Schwendener R, Ruegg C, Christofori G. Myeloid cells contribute to tumor lymphangiogenesis. PLoS ONE. (2009) 4:e7067. doi: 10.1371/journal.pone.0007067
89. Miteva DO, Rutkowski JM, Dixon JB, Kilarski W, Shields JD, Swartz MA. Transmural flow modulates cell and fluid transport functions of lymphatic endothelium. Circulat Res. (2010) 106:920–31. doi: 10.1161/CIRCRESAHA.109.207274
90. Hirakawa S, Detmar M, Kerjaschki D, Nagamatsu S, Matsuo K, Tanemura A, et al. Nodal lymphangiogenesis and metastasis: role of tumor-induced lymphatic vessel activation in extramammary Paget's disease. Am J Pathol. (2009) 175:2235–48. doi: 10.2353/ajpath.2009.090420
91. Kim M, Koh YJ, Kim KE, Koh BI, Nam DH, Alitalo K, et al. CXCR4 signaling regulates metastasis of chemoresistant melanoma cells by a lymphatic metastatic niche. Cancer Res. (2010) 70:10411–21. doi: 10.1158/0008-5472.CAN-10-2591
92. Shields JD, Kourtis IC, Tomei AA, Roberts JM, Swartz MA. Induction of lymphoidlike stroma and immune escape by tumors that express the chemokine CCL21. Science. (2010) 328:749–52. doi: 10.1126/science.1185837
93. Lund AW, Duraes FV, Hirosue S, Raghavan VR, Nembrini C, Thomas SN, et al. VEGF-C promotes immune tolerance in B16 melanomas and cross-presentation of tumor antigen by lymph node lymphatics. Cell Rep. (2012) 1:191–9. doi: 10.1016/j.celrep.2012.01.005
94. Hu J, Cheng Y, Li Y, Jin Z, Pan Y, Liu G, et al. microRNA-128 plays a critical role in human non-small cell lung cancer tumourigenesis, angiogenesis and lymphangiogenesis by directly targeting vascular endothelial growth factor-C. Eur J Cancer. (2014) 50:2336–50. doi: 10.1016/j.ejca.2014.06.005
95. Keklikoglou I, Hosaka K, Bender C, Bott A, Koerner C, Mitra D, et al. MicroRNA-206 functions as a pleiotropic modulator of cell proliferation, invasion and lymphangiogenesis in pancreatic adenocarcinoma by targeting ANXA2 and KRAS genes. Oncogene. (2015) 34:4867–78. doi: 10.1038/onc.2014.408
96. Lin CY, Wang SW, Chen YL, Chou WY, Lin TY, Chen WC, et al. Brain-derived neurotrophic factor promotes VEGF-C-dependent lymphangiogenesis by suppressing miR-624-3p in human chondrosarcoma cells. Cell Death Dis. (2017) 8:e2964. doi: 10.1038/cddis.2017.354
97. Yang WH, Chang AC, Wang SW, Wang SJ, Chang YS, Chang TM, et al. Leptin promotes VEGF-C production and induces lymphangiogenesis by suppressing miR-27b in human chondrosarcoma cells. Sci Rep. (2016) 6:28647. doi: 10.1038/srep28647
98. Wang LH, Lin CY, Liu SC, Liu GT, Chen YL, Chen JJ, et al. CCL5 promotes VEGF-C production and induces lymphangiogenesis by suppressing miR-507 in human chondrosarcoma cells. Oncotarget. (2016) 7:36896–908. doi: 10.18632/oncotarget.9213
99. Tzeng HE, Chang AC, Tsai CH, Wang SW, Tang CH. Basic fibroblast growth factor promotes VEGF-C-dependent lymphangiogenesis via inhibition of miR-381 in human chondrosarcoma cells. Oncotarget. (2016) 7:38566–78. doi: 10.18632/oncotarget.9570
100. Su CM, Tang CH, Chi MJ, Lin CY, Fong YC, Liu YC, et al. Resistin facilitates VEGF-C-associated lymphangiogenesis by inhibiting miR-186 in human chondrosarcoma cells. Biochem Pharmacol. (2018) 154:234–42. doi: 10.1016/j.bcp.2018.05.001
101. Lin CC, Chen PC, Lein MY, Tsao CW, Huang CC, Wang SW, et al. WISP-1 promotes VEGF-C-dependent lymphangiogenesis by inhibiting miR-300 in human oral squamous cell carcinoma cells. Oncotarget. (2016) 7:9993–10005. doi: 10.18632/oncotarget.7014
102. Lien MY, Tsai HC, Chang AC, Tsai MH, Hua CH, Wang SW, et al. Chemokine CCL4 induces vascular endothelial growth factor C expression and lymphangiogenesis by miR-195–3p in oral squamous cell carcinoma. Front Immunol. (2018) 9:412. doi: 10.3389/fimmu.2018.00412
103. Sasahira T, Kirita T, Kurihara M, Yamamoto K, Bhawal UK, Bosserhoff AK, et al. MIA-dependent angiogenesis and lymphangiogenesis are closely associated with progression, nodal metastasis and poor prognosis in tongue squamous cell carcinoma. Eur J Cancer. (2010) 46:2285–94. doi: 10.1016/j.ejca.2010.04.027
104. Sasahira T, Kurihara M, Bhawal UK, Ueda N, Shimomoto T, Yamamoto K, et al. Downregulation of miR-126 induces angiogenesis and lymphangiogenesis by activation of VEGF-A in oral cancer. Br J Cancer. (2012) 107:700–6. doi: 10.1038/bjc.2012.330
105. Liu C, Li M, Hu Y, Shi N, Yu H, Liu H, et al. miR-486-5p attenuates tumor growth and lymphangiogenesis by targeting neuropilin-2 in colorectal carcinoma. Oncotargets Ther. (2016) 9:2865–71. doi: 10.2147/OTT.S103460
106. Qian Q, Sun W, Zhu W, Liu Y, Ge A, Ma Y, et al. The role of microRNA-93 regulating angiopoietin2 in the formation of malignant pleural effusion. Cancer Med. (2017) 6:1036–48. doi: 10.1002/cam4.1000
107. Ye T, Yang M, Huang D, Wang X, Xue B, Tian N, et al. MicroRNA-7 as a potential therapeutic target for aberrant NF-kappaB-driven distant metastasis of gastric cancer. J Exp Clin Cancer Res. (2019) 38:55. doi: 10.1186/s13046-019-1074-6
108. Zhang W, Hong R, Li L, Wang Y, Du P, Ou Y, et al. The chromosome 11q13.3 amplification associated lymph node metastasis is driven by miR-548k through modulating tumor microenvironment. Mol Cancer. (2018) 17:125. doi: 10.1186/s12943-018-0871-4
109. Xu Q, Tong JL, Zhang CP, Xiao Q, Lin XL, Xiao XY. miR-27a induced by colon cancer cells in HLECs promotes lymphangiogenesis by targeting SMAD4. PLoS ONE. (2017) 12:e0186718. doi: 10.1371/journal.pone.0186718
110. Zhou CF, Ma J, Huang L, Yi HY, Zhang YM, Wu XG, et al. Cervical squamous cell carcinoma-secreted exosomal miR-221-3p promotes lymphangiogenesis and lymphatic metastasis by targeting VASH1. Oncogene. (2019) 38:1256–68. doi: 10.1038/s41388-018-0511-x
111. Chen W, Cai F, Zhang B, Barekati Z, Zhong XY. The level of circulating miRNA-10b and miRNA-373 in detecting lymph node metastasis of breast cancer: potential biomarkers. Tumour Biol. (2013) 34:455–62. doi: 10.1007/s13277-012-0570-5
112. Yang B, Jing C, Wang J, Guo X, Chen Y, Xu R, et al. Identification of microRNAs associated with lymphangiogenesis in human gastric cancer. Clin Transl Oncol. (2014) 16:374–9. doi: 10.1007/s12094-013-1081-6
113. Fazi F, Fontemaggi G. MicroRNAs and lymph node metastatic disease in lung cancer. Thorac Surg Clin. (2012) 22:167–75. doi: 10.1016/j.thorsurg.2011.11.001
114. Saiselet M, Gacquer D, Spinette A, Craciun L, Decaussin-Petrucci M, Andry G, et al. New global analysis of the microRNA transcriptome of primary tumors and lymph node metastases of papillary thyroid cancer. BMC Genomics. (2015) 16:828. doi: 10.1186/s12864-015-2082-3
115. Mutalib NS, Yusof AM, Mokhtar NM, Harun R, Muhammad R, Jamal R. MicroRNAs and lymph node metastasis in papillary thyroid cancers. Asian Pac J Cancer Prev. (2016) 17:25–35. doi: 10.7314/APJCP.2016.17.1.25
116. Tsai MC, Spitale RC, Chang HY. Long intergenic noncoding RNAs: new links in cancer progression. Cancer Res. (2011) 71:3–7. doi: 10.1158/0008-5472.CAN-10-2483
117. Gutschner T, Diederichs S. The hallmarks of cancer: a long non-coding RNA point of view. RNA Biol. (2012) 9:703–19. doi: 10.4161/rna.20481
118. Li CH, Chen Y. Targeting long non-coding RNAs in cancers: progress and prospects. Int J Biochem Cell Biol. (2013) 45:1895–910. doi: 10.1016/j.biocel.2013.05.030
119. Li W, Kang Y. A new Lnc in metastasis: long noncoding RNA mediates the prometastatic functions of TGF-beta. Cancer Cell. (2014) 25:557–9. doi: 10.1016/j.ccr.2014.04.014
120. Tuck AC, Tollervey D. A transcriptome-wide atlas of RNP composition reveals diverse classes of mRNAs and lncRNAs. Cell. (2013) 154:996–1009. doi: 10.1016/j.cell.2013.07.047
121. Li Y, Chen H, Pan T, Jiang C, Zhao Z, Wang Z, et al. LncRNA ontology: inferring lncRNA functions based on chromatin states and expression patterns. Oncotarget. (2015) 6:39793–805. doi: 10.18632/oncotarget.5794
122. Lin Z, Hu Y, Lai S, Xue M, Lin J, Qian Y, et al. Long Noncoding RNA: its partners and their roles in cancer. Neoplasma. (2015) 62:846–54. doi: 10.4149/neo_2015_103
123. Sun Z, Ou C, Ren W, Xie X, Li X, Li G. Downregulation of long non-coding RNA ANRIL suppresses lymphangiogenesis and lymphatic metastasis in colorectal cancer. Oncotarget. (2016) 7:47536–55. doi: 10.18632/oncotarget.9868
124. Chen C, He W, Huang J, Wang B, Li H, Cai Q, et al. LNMAT1 promotes lymphatic metastasis of bladder cancer via CCL2 dependent macrophage recruitment. Nat Commun. (2018) 9:3826. doi: 10.1038/s41467-018-06152-x
125. Yang Z, Zhi Q, Wang D, Zhang L, Preston B, Brandon C, et al. Long noncoding RNA C21orF96 promotes the migration, invasion and lymph node metastasis in gastric cancer. Anti Cancer Agents Med Chem. (2016) 16:1101–8. doi: 10.2174/1871520616666151116122921
126. Wang J, Pan Y, Wu J, Zhang C, Huang Y, Zhao R, et al. The association between abnormal long noncoding RNA MALAT-1 expression and cancer lymph node metastasis: a meta-analysis. Biomed Res Int. (2016) 2016:1823482. doi: 10.1155/2016/1823482
127. He A, Hu R, Chen Z, Liao X, Li J, Wang D, et al. Role of long noncoding RNA UCA1 as a common molecular marker for lymph node metastasis and prognosis in various cancers: a meta-analysis. Oncotarget. (2016) 8:1937–43. doi: 10.18632/oncotarget.12463
128. Chen Z, He A, Wang D, Liu Y, Huang W. -Long noncoding RNA HOTTIP as a novel predictor of lymph node metastasis and survival in human cancer: a systematic review and meta-analysis. Oncotarget. (2016) 8:14126–32. doi: 10.18632/oncotarget.12981
129. Cai B, Wu Z, Liao K, Zhang S. Long noncoding RNA HOTAIR can serve as a common molecular marker for lymph node metastasis: a meta-analysis. Tumour Biol. (2014) 35:8445–50. doi: 10.1007/s13277-014-2311-4
130. Diederichs S, Bartsch L, Berkmann JC, Frose K, Heitmann J, Hoppe C, et al. The dark matter of the cancer genome: aberrations in regulatory elements, untranslated regions, splice sites, non-coding RNA and synonymous mutations. EMBO Mol Med. (2016) 8:442–57. doi: 10.15252/emmm.201506055
131. Anczukow O, Buisson M, Leone M, Coutanson C, Lasset C, Calender A, et al. BRCA2 deep intronic mutation causing activation of a cryptic exon: opening toward a new preventive therapeutic strategy. Clin Cancer Res. (2012) 18:4903–9. doi: 10.1158/1078-0432.CCR-12-1100
132. Staropoli JF, Li H, Chun SJ, Allaire N, Cullen P, Thai A, et al. Rescue of gene-expression changes in an induced mouse model of spinal muscular atrophy by an antisense oligonucleotide that promotes inclusion of SMN2 exon 7. Genomics. (2015) 105:220–8. doi: 10.1016/j.ygeno.2015.01.007
133. Rothschild SI. microRNA therapies in cancer. Mol Cell Ther. (2014) 2:7. doi: 10.1186/2052-8426-2-7
134. Sanchez Y, Huarte M. Long non-coding RNAs: challenges for diagnosis and therapies. Nucleic Acid Ther. (2013) 23:15–20. doi: 10.1089/nat.2012.0414
135. Gutschner T, Hammerle M, Eissmann M, Hsu J, Kim Y, Hung G, et al. The noncoding RNA MALAT1 is a critical regulator of the metastasis phenotype of lung cancer cells. Cancer Res. (2013) 73:1180–9. doi: 10.1158/0008-5472.CAN-12-2850
136. Saaristo A, Tammela T, Farkkila A, Karkkainen M, Suominen E, Yla-Herttuala S, et al. Vascular endothelial growth factor-C accelerates diabetic wound healing. Am J Pathol. (2006) 169:1080–7. doi: 10.2353/ajpath.2006.051251
137. Alitalo AK, Proulx ST, Karaman S, Aebischer D, Martino S, Jost M, et al. VEGF-C and VEGF-D blockade inhibits inflammatory skin carcinogenesis. Cancer Res. (2013) 73:4212–21. doi: 10.1158/0008-5472.CAN-12-4539
138. Tammela T, Zarkada G, Wallgard E, Murtomaki A, Suchting S, Wirzenius M, et al. Blocking VEGFR-3 suppresses angiogenic sprouting and vascular network formation. Nature. (2008) 454:656–60. doi: 10.1038/nature07083
Keywords: non-coding RNA (ncRNA), lymphangiogenesis, lymphatic development, inflammation, cancer metastasis
Citation: Cao M, Tang Y, Zhang W, Tang Y-J and Liang X (2019) Non-coding RNAs as Regulators of Lymphangiogenesis in Lymphatic Development, Inflammation, and Cancer Metastasis. Front. Oncol. 9:916. doi: 10.3389/fonc.2019.00916
Received: 15 March 2019; Accepted: 03 September 2019;
Published: 20 September 2019.
Edited by:
César López-Camarillo, Universidad Autónoma de la Ciudad de México, MexicoReviewed by:
Alessandra Ghigo, University of Turin, ItalyPrasanna Ekambaram, University of Pittsburgh, United States
Copyright © 2019 Cao, Tang, Zhang, Tang and Liang. This is an open-access article distributed under the terms of the Creative Commons Attribution License (CC BY). The use, distribution or reproduction in other forums is permitted, provided the original author(s) and the copyright owner(s) are credited and that the original publication in this journal is cited, in accordance with accepted academic practice. No use, distribution or reproduction is permitted which does not comply with these terms.
*Correspondence: Ya-Jie Tang, eWFqaWV0YW5nJiN4MDAwNDA7c2R1LmVkdS5jbg==; Xin-hua Liang, bHhoODg4NjYmI3gwMDA0MDtzY3UuZWR1LmNu
†These authors have contributed equally to this work