- 1Hematology, Department of Translational and Precision Medicine, Sapienza University of Rome, Rome, Italy
- 2GIMEMA Foundation, Rome, Italy
- 3Department of Molecular Medicine, Sapienza University of Rome, Rome, Italy
Introduction: Acute lymphoblastic leukemia (ALL) is the first neoplasm where the assessment of early response to therapy by minimal residual disease (MRD) monitoring has proven to be a fundamental tool to guide therapeutic choices. The most standardized methods to study MRD in ALL are multi-parametric flow cytometry (MFC) and polymerase chain reaction (PCR) amplification-based methods. Emerging technologies hold the promise to improve MRD detection in ALL patients. Moreover, novel therapies, such as monoclonal antibodies, bispecific T-cell engagers, and chimeric antigen receptor T cells (CART) represent exciting advancements in the management of B-cell precursor (BCP)-ALL.
Aims: Through a review of the literature and in house data, we analyze the current status of MRD assessment in ALL to better understand how some of its limitations could be overcome by emerging molecular technologies. Furthermore, we highlight the future role of MRD monitoring in the context of personalized protocols, taking into account the genetic complexity in ALL.
Results and Conclusions: Molecular rearrangements (gene fusions and immunoglobulin and T-cell receptor-IG/TR gene rearrangements) are widely used as targets to detect residual leukemic cells in ALL patients. The advent of novel techniques, namely next generation flow cytometry (NGF), digital-droplet-PCR (ddPCR), and next generation sequencing (NGS) appear important tools to evaluate MRD in ALL, since they have the potential to overcome the limitations of standard approaches. It is likely that in the forthcoming future these techniques will be incorporated in clinical trials, at least at decisional time points. Finally, the advent of new powerful compounds is further increasing MRD negativity rates, with benefits in long-term survival and a potential reduction of therapy-related toxicities. However, the prognostic relevance in the setting of novel immunotherapies still needs to be evaluated.
Introduction
Acute lymphoblastic leukemia (ALL) is a malignant disorder that originates from hemopoietic lymphoid precursors, that can be of B- (80–85%) or T-cell (20–25%) derivation: the acquisition of a series of genetic aberrations leads to an impaired maturation, with an arrest in the differentiation process and an abnormal proliferation (1). As a consequence, the accumulation of leukemic cells occurs in both the bone marrow, where it suppresses the physiologic hemopoiesis, as well as in extra-medullary sites.
The leukemic transformation generates a progeny of leukemic lymphoid blasts that have undergone a maturation block in an early phase of the differentiation process. The pathophysiological bases of the symptoms and signs of ALL consist in a suppression of normal hemopoiesis, in the infiltration and colonization of lymphoid organs and in the release of lymphokines and mediators of inflammation of both leukemic cells and normal cells. It is a heterogeneous malignancy also in terms of clinical manifestation and prognosis.
ALL is the most frequent cancer in childhood and is diagnosed also in adulthood, with peaks of incidence between the age of 2 and 5 years and after the age of 50 (2, 3) with 60% of cases occurring in individuals below 20 years of age (4).
Owing to the application of risk-adapted therapy and improved supportive care, the 5 years survival rate for children with ALL has significantly increased from 57 to 92% over time (5–10). However, relapses still occur in 20% of children with ALL (11) and are associated with a poor outcome (12). In adults, the frequency of high-risk leukemia and relapse risk is higher; implementing pediatric ALL treatment algorithms has led to substantial improvements in adult ALL. Nevertheless, 40–50% of adult patients still relapse (13, 14). This can be partly attributed to the higher incidence of high-risk molecular aberrations in older patients and also to the fact that older patients are less fit to tolerate intensive treatments.
ALL is the first neoplasm where the assessment of early response to therapy by minimal residual disease (MRD) monitoring has proven to be a fundamental tool for guiding therapeutic choices. At present, MRD detection is used for: the assessment of initial treatment response and subsequent definition of MRD-based risk groups with consequent risk-stratification; monitoring disease burden in the setting of stem cell transplantation (SCT); early marker of impending relapse.
Methodologies for MRD Detection
MRD is defined as any approach—including cytogenetics, flow cytometry, PCR-based tools, and high throughput sequencing methods—aimed at detecting and possibly quantifying residual tumor cells beyond the sensitivity level of cytomorphology. To be informative, MRD assays for ALL should allow to detect one leukemic cell among 10,000 normal cells or more in virtually all patients. Currently, the most standardized methods to study MRD in ALL are: multi-parametric flow cytometry (MFC) of leukemia-associated immunophenotypes (LAIP) and, more so, polymerase chain reaction (PCR) amplification-based methods that use leukemia-specific (fusion gene transcripts) or patient-specific (immunoglobulin/T-cell receptor (IG/TR) gene rearrangements) molecular markers (15–20).
Source of Material for MRD Evaluation
In the past, it has been debated if peripheral blood (PB), rather than bone marrow (BM), could be used for MRD monitoring, regardless of the technique used (MFC or PCR). It is nowadays clear that the scenario is different between B-lineage and T-lineage ALL: in fact, in BCP-ALL, MRD levels tend to be 1–3 logs lower in PB than in BM (21, 22) and that bone marrow assessments cannot be replaced by blood analyses; at variance, in T-ALL, it has been shown—both in children and adults—that PB is a reliable source for MRD monitoring since there are no significant differences with BM and therefore they could be used as an alternative source. Nevertheless, also in T-lineage ALL MRD assessments are normally carried out on BM samples.
Multi-Parametric Flow Cytometry Analysis
This approach takes advantage from the presence of proteic epitopes (antigens) in the nucleus, cytoplasm or surface of the cell, which are sequentially acquired during normal cell development. Antigens are differently expressed by B- and T-lymphoblasts, and their expression is assessed by quantification of the signal emitted by fluorochrome-conjugated-specific monoclonal antibodies (MoAb). The LAIP must be identified at diagnosis, before any therapy in each ALL case, by comparing the marker profile of leukemia cells to reference bone marrow samples, through various combinations of monoclonal antibodies against surface, cytoplasmic, or nuclear leukocyte antigens. A second approach is represented by the so-called “different from normal (DFN)” analysis, which defines leukemic blasts by recognizing immunophenotypic changes with respect to a normal counterpart population (either hematopoietic progenitors of similar lineage and maturational stage) thought the evaluation of antigenic patterns expression (23). This tool has the advantage of studying MRD without the need of a diagnostic immunophenotype, but it requires standardization and needs further implementation.
During the years, antibody-conjugated fluorochromes were developed to increase the number of “colors;” further advances in the field increased the possibility of studying a great number of functionally distinct lymphocyte populations in human PB (24–26). The introduction of violet lasers (405 nm) and of nanocrystals (called quantum dots) or organic polymers capable of conducting electrons (27) led to the current MFC capable of analyzing up to 18 colors in a single cell (28). At present, the most commonly used MFC panels comprise 6–8 MoAb combinations. The refinement of MFC has required a parallel advancement in hardware, software and reagents. Engineering advances in optics and signal processing (digital electronics) are areas of active development (24, 29). Different software packages are now available, including FACSDivaTM [Becton Dickinson], KaluzaTM [Beckman Coulter], FlowJoTM [www.treestar.com], CytobankTM [www.cytobank.org], SPICETM [http://exon.niaid.nih.gov/spice/], SamSPECTRALTM [R-package], FLAMETM, SPADETM [www.cytospade.org], FlowTypeTM [R-package], FlowCAPTM [flowcap.- flowsite.org], GemStoneTM [www.vsh.com], InfinicyteTM [www.infinicyt.com].
Flow cytometry can be successfully applied to the majority of cases (>90%) and can reach a sensitivity of 10−3-10−4 (one leukemic cell out of 1000–10,000 normal cells) (Table 1 and Figure 1) (30). Flow cytometry analysis is quick, can release MRD evaluations in few hours, and is, therefore, also useful to assess the therapeutic response following the first 2 induction weeks (31). However, some limitations exist, such as the fact that the samples must be analyzed shortly after collection to avoid cell death, a problem when shipment is required for the centralized evaluation of MRD referral laboratories. Furthermore, (a) post-induction regeneration of normal lymphoid cells co-expressing some ALL-type antigens can lead to false positive results in B-ALL cases, (b) the bone marrow sample hypocellularity and, in some patients, phenotypic shift can induce erroneous or difficult interpretations (32, 33). The EuroFlow Consortium has optimized and standardized immunostaining protocols for the diagnosis, classification and prognostic subclassification of hematologic malignancies, as well as for the detection of MRD during the clinical follow-up; however, experienced operators are still needed to correctly evaluate MRD results (34, 35).
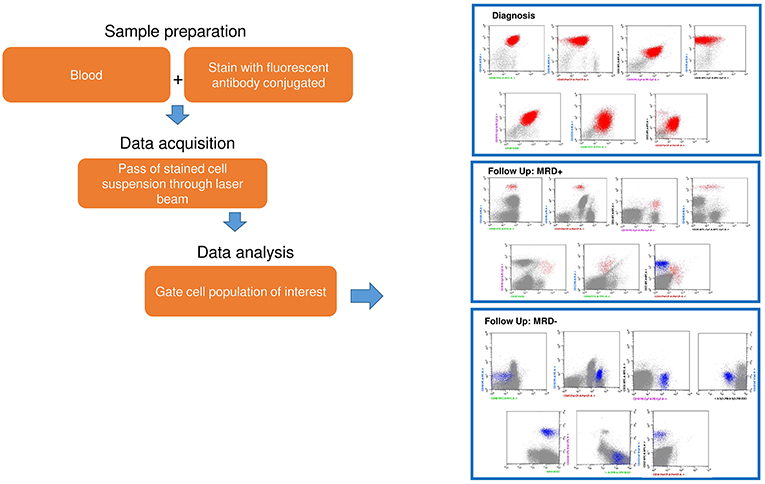
Figure 1. An example of standard flow cytometry MRD analysis. Sample preparation from peripheral and/or bone marrow blood requires staining with fluorescent conjugate antibodies. Data acquisition requires: (1) the stained cells through a laser beam, (2) registration of fluorescence emission from conjugate cells. This is followed by data analysis with a specific software.
Molecular Analysis
Antigen-Receptor Gene Rearrangements
The most commonly used technique is the molecular study based of antigen-receptor gene rearrangements. Immunoglobulin and T-cell receptor (IG/TR) gene rearrangements are physiological events not directly linked to the pathogenesis of the leukemia. During B- and T-lymphocytes ontogeny, the IG and TR genes are assembled by a somatic rearrangement process. The separated gene segments encoding the V, D, J regions are combined to form a single exon encoding the variable region. In this process, some nucleotides are randomly deleted or inserted at junctional sites of each segment, leading to final receptor sequences unique to each B or T lymphocytes (36). In the case of a neoplastic transformation of a single lymphoid cell, all leukemic cells will contain the same rearranged clonal IG and/or TR genes; this approach can also be exploited to detect a low number of ALL cells among a large number of normal lymphoid cells expressing gene rearrangements with different sequences. The study of these rearrangements has become the most sensitive method to assess the clonality of a lymphoid expansion. Although IG rearrangements are mostly found in B cells and TR rearrangements in T- lymphocytes, both B-lineage and T-lineage leukemic cells can display cross-lineage rearrangements, which can be used for MRD evaluation (37, 38): up to 90% of precursor B-ALL may express TR gene rearrangements (38) whereas a lower proportion of T-ALL (20%) shows IG rearrangements (39). To identify these molecular markers at diagnosis, genomic DNA derived from leukemic cells need to undergoes a PCR amplification process and the positive PCR products are then analyzed by heteroduplex or gene scan (40, 41) to establish clonality. Subsequently, clonal PCR fragments undergo Sanger sequencing to define the junctional regions and obtain complementary allele-specific oligonucleotide (ASO)-primers for MRD monitoring, mostly performed by real-time quantitative PCR (RQ-PCR). Amplification conditions and sensitivity testing for each ASO-primer are established on the diagnostic material serially diluted in normal mononuclear cells. This RQ-PCR protocol combined with fluorescently labeled probes allows the detection of up to 1 leukemic cell in 100,000 (10−5) normal lymphoid cells and leukemia cell dilutions are therefore used to quantify MRD in bone marrow samples collected during treatment (42) (Table 1 and Figure 2). This technology can generate at least one single sensitive molecular probe suitable for MRD analysis in over 90% of pediatric (18) and adult (43, 44) ALL patients.
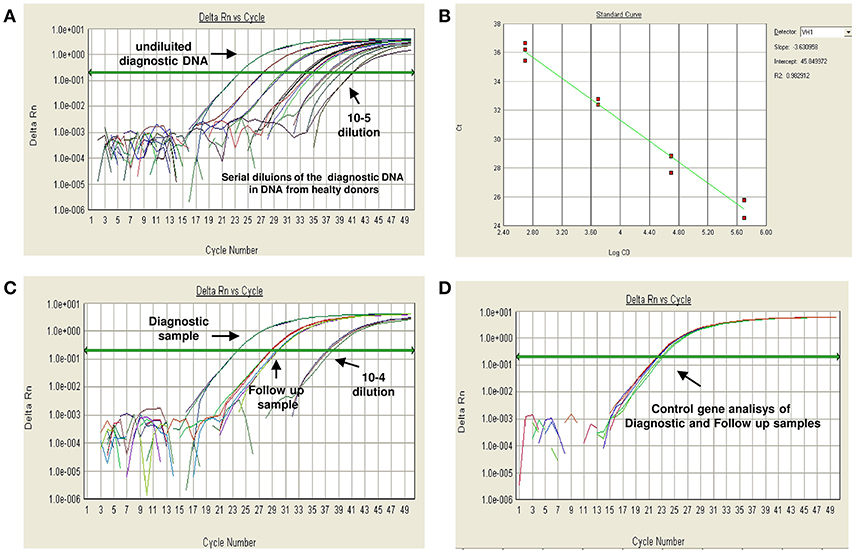
Figure 2. An example of RQ-PCR MRD analysis by a IGHV gene rearrangement according to EuroMRD Consortium guidelines. Patient-specific primers are used to detect malignant cells among normal lymphoid cells during follow-up. Serial dilutions of the diagnostic DNA in DNA from healthy donors are performed to verify the sensitivity and specificity of each designed primer and of each PCR assay (A), and to obtain a regression curve (B) for the precise quantification of fluorescent levels at the single time points. Afterwards, the primer suitable for analysis is used for MRD study (C). A control gene analysis (i.e., albumin) must be performed for the diagnostic and for each follow-up sample, in order to assess the amount and quality (amplificability) of the DNA in each reaction (D).
Antigen-receptor gene rearrangements analysis is the most broadly applied method for MRD detection and has been extensively standardized within the EuroMRD Consortium (previously known as European Study Group- ESG-MRD-ALL) that established guidelines for the analysis and interpretation of RQ-PCR data (16) to favor an homogeneous application of MRD studies within different treatment protocols for childhood and adult ALL. Of note, a small percentage of ALL, mostly those originating from more immature cells, does not carry IG/TR gene rearrangements and occasionally technical failures can impair MRD target identification. Overall, it is not possible to perform RQ-PCR-based MRD assessments in about 5–10% of ALL cases. Another limitation of this approach is represented by clonal evolution of IG/TR rearrangement patterns during the course of the disease and at relapse, which can sometimes occur in cases with oligoclonal rearrangements leading to false negative MRD results (45). These events depend on the type of marker, disease and time to relapse (i.e., early or late relapse). The amount of diagnostic DNA is another problematic factor for this type of MRD assessment, because diagnostic DNA is needed for each MRD experiment, as well as for the detection of IG/ TR clonal rearrangements, being the quantification related to the tumor load at diagnosis. Finally, RQ-PCR is not able to define precisely the amount of residual disease in those cases in which the disease burden is very low. These cases with low MRD levels are defined as “positive-not-quantifiable” (PNQ) and their identification represents today a primary unmet need in the clinical practice when treatment decisions are based on MRD monitoring.
Fusion Transcripts
Another method for molecular MRD detection and monitoring is based on fusion transcript analysis. Overall, more than 40% of ALL patients carry chromosomal translocations that generate chimeric transcripts: these are potentially ideal targets for MRD assessment (46, 47), since they are main driver events, are expressed in all leukemic cells and are extremely stable during the course of the disease. Within B-lineage ALL, the most common translocation detected in adult cases is the (9, 23), also called Philadelphia chromosome, leading to the BCR-ABL1 rearrangement (25–30% of cases); its frequency increases with age, being detected in about 50% of cases above the age of 50 years. At variance, the most common chimeric transcript in pediatric patients is represented by ETV6-RUNX1, that accounts for 25–30% of childhood ALL. Other fusion transcripts are KMT2A (alias MLL)-AFF1 and TCF3-PBX1 each accounting for 3–8% of cases, regardless of age. Infants (i.e., <1 year) carry a KMT2A gene rearrangement in 80% of cases. In T-ALL, TAL1 deletions (SIL/TAL1) occur in about 20% of T-ALL (48). Other rarer translocations in T-ALL involve the ABL1 gene (NUP/ABL1, EML1/ABL1, ETV6/ABL1). Because most of these chromosomal abnormalities have prognostic value, their detection must be performed in all cases at diagnosis (49) so that each patient can be monitored for MRD using a predefined marker throughout the course of the disease. Due to the large DNA portion in which the translocation breakpoints occurs, a patient-specific tool for MRD evaluation cannot be easily obtained; at variance, the RNA splicing process produces in all patients the same fusion transcript or few splicing variants. Thus, RNA is the optimal starting material to detect these lesions, allowing the use of a small number of quantitative-reverse transcriptase PCR (QRT-PCR) assays (50). This offers the opportunity to apply the same primer set to all patients bearing the same translocation, leading to an easy and rapid fusion transcript evaluation at diagnosis and during treatment (47).
Quantification of the fusion gene using RNA samples is achieved by comparing the amplified product to a standard curve derived from the amplification of serial dilutions of a cell line or plasmid DNA (i.e., BCR-ABL1+). This highly sensitive MRD assay is capable of detecting up to 1 leukemic cell within 100,000 (10−5) normal lymphoid cells, is not patient-specific, is relatively easy to perform and is not expensive (Table 1). However, the accuracy of this assay is hampered by the variability in the number of RNA transcripts per leukemic cell from patient to patient, and among different cells within the same leukemic clone. A full standardization is still not available and the EuroMRD Consortium is setting up guidelines for the correct interpretation of quantitative data (51).
At variance from the BCR/ABL1 rearrangement, in patients displaying other recurrent chromosomal translocations (i.e., KMT2A gene rearrangements and SIL/TAL1), quantification of the fusion gene is well standardized within the EuroMRD Consortium (52, 53).
New Methods for MRD Detection and Monitoring: Next-Generation Flow Cytometry, Digital-Droplet-PCR, Next Generation Sequencing
The advent of RQ-PCR (54) has represented a significant advancement with respect to conventional PCR based methods. However, the measurement of a dynamic process, such as the rate of target amplification, carries some intrinsic fluctuations that cannot be to fully eliminated: (a) non-specific amplification of spurious IG/TR rearrangements are hardly distinguishable from cases positive at a very low level (PNQ) in RQ-PCR, with an intrinsic risk of false positive/negative MRD detections; (b) the use of RQ-PCR can be limited by the lack of sufficient diagnostic material since the method is based on the comparison, for each experiment, with a standard curve based on neoplastic DNA collected at the onset of the disease, and this can limit the possibility of monitoring patients over time.
The novel next generation flow (NGF)-MRD approach takes advantage of innovative tools and procedures recently developed by the EuroFlow Consortium for sample preparation, antibody combinations (including choice of type of antibody and fluorochrome), and identification of B-cell precursor (BCP) pathway in the BM, which allows to define the degree of immunophenotypic deviation of BCP-ALL cells from normal BCP (also in regenerating BM). Also for T-ALL a comparable strategy is used to obtain reliable (evidence-based) antibody combinations, in order to discriminate from various types of normal T cells and other cells with cross-lineage marker expression (55, 56).
NGF-MRD is faster and reproducible, it has a greater applicability (>95%). Moreover, the costs of reagents and assays are estimated to be lower than those of NGS (57). However, it requires fresh material analyzed within 24 h after sampling. Finally, NGF-MRD strategies provide a full insight into the composition of normal cells and aberrant cells, and can help to better characterize ALL cell population changes such as treatment-induced immunophenotypic shifts (58, 59), heterogeneity in the blast cell population with a de-differentiation to immature stem like-cells and aberrations in other lineages.
A similar applicability associated with a significantly increased sensitivity for the novel EuroFlow-NGF approach vs. conventional flow-MRD has been described in multiple myeloma (59); NGF-MRD reaches a sensitivity close to 10−6, while conventional flow tools reach sensitivities in the range of 10−4-10−5 (Table 2 and Figure 3). The greater sensitivity of NGF-MRD is mostly due to the use of standardized approaches, including instrument setting, sample processing with bulk lysis procedure, immunostaining, data acquisition, and data analysis with standardized (even automated) gating strategies for definition of cell populations (59). However, the acquisition of a large number of cells is needed to reach the required sensitivity.
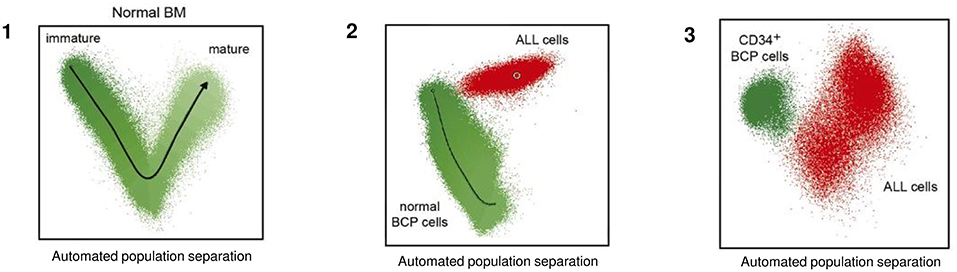
Figure 3. Schematic representation of normal and malignant B-cell precursor by multidimensional analysis based on EuroFiow-based NGF-MRD. This analysis is not based on a single marker but on multiple required antigens, allowing to define the degree of immunophenotypic deviation of BCP-ALL cells from normal BCP, visualized in multivariate analysis plots. (1) Representation of Automated population separation (APS) of physiological phases of B-cell maturation. (2) Plot of ALL cells (red dots) with respect to normal BCP cells (green dots). (3) Plot of ALL cells (red dots) with respect to immature CD34+ BCP cells only (green dots). Adapted from van Dongen et al. (60).
In the forthcoming decade, the new flow technologies will improve applicability and specificity of flow MRD measurements.
The digital PCR technology (ddPCR) (61) based on sample partitioning (mimicking limiting dilution) and Poisson statistics, has the potential to overcome the limitations of RQ-PCR. DdPCR (62, 63) is the third-generation implementation of conventional PCR that allows the quantitation of nucleic acid targets without the need of the calibration curves (64). As reported in several studies (65, 66), based on the dynamic nature of two methods, ddPCR appears more accurate than RQ-PCR since: (i) each sample is partitioned in droplets and each droplet is analyzed individually, (ii) small changes in fluorescence intensity are more readily detected, and (iii) the ratio between target DNA molecules to PCR reagents is substantially higher; this increases its amplification efficiency (67). Finally, the presence of inhibitors negatively affects the RQ-PCR efficiency but not that of ddPCR (68) (Table 2 and Figure 4).
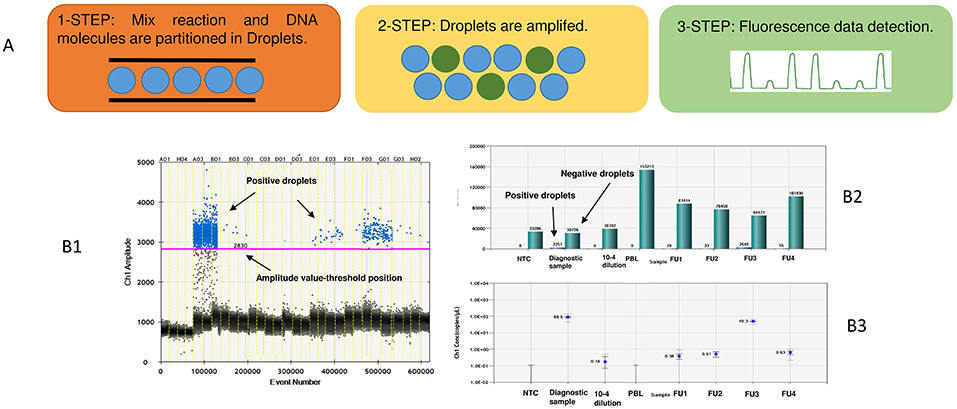
Figure 4. An example of ddPCR MRD analysis. In the (A) is reported a schematic diagram of a ddPCR experiment. 1-Step: the mix reaction is prepared with the same primer/probes of the TaqMan assay. Both the reaction and the DNA samples are partitioned into 20,000 droplets of identical volume through a microfluidic system. 2-Step: in a thermal cycler 20,000 PCR reactions are amplified and fluorescence is the output during the reaction of polymerization. 3-Step: a droplet reader analyzes each droplet individually and detects an increased fluorescence in positive droplets, which contains at least one copy of target DNA. (B) Each droplet is plotted on the graph of fluorescence intensity vs. droplet number (B1). The concentration is calculated on the fraction of empty droplets (green bar) that are the fraction that does not contain any target DNA by software (B2). Fraction of positive droplets is fitted to a Poisson algorithm to determine absolute copy number, results are presented in copies per 1-μL (B3).
Moreover, RQ-PCR, as an exponential process, is able to greatly amplify even small differences in reaction efficiency, leading to discrepancies in the final results with 68% confidence intervals (http://www.biorad.com/webroot/web/pdf/lsr/literature/Bulletin_6407.pdf).
ddPCR is an endpoint measurement with 95% confidence intervals, as reported by the manufacturer's application guide. The ddPCR technology has been applied to various fields of medical diagnostics, in particular in molecular oncology (62, 64, 65) and in prenatal diagnosis (69, 70). Several reports on the use of ddPCR in hematologic malignancies (71–74) are available in the literature. Three recent articles, including two from our Center, have compared ddPCR to RQ-PCR in adult patients affected by mature lymphoid malignancies and in Ph- ALL (75–77). These studies have established analytical parameters to investigate the applicability of ddPCR for MRD detection and concluded that ddPCR has a sensitivity, accuracy and reproducibility at least comparable to that of RQ-PCR. Regarding MRD evaluations, some discordances have been observed at very low disease levels; in this setting, ddPCR showed a good analytical performance to quantify those low positive samples defined as PNQ by RQ-PCR or to identify the false MRD+ cases. These results have been confirmed from our group in a wider comparative analysis including 175 patients with different lymphoid malignancies (78) (Figure 5). Recently, the clinical significance of ddPCR has been reported in a pediatric cohort of ALL patients (79). The authors showed that among “slow early responder” patients, most relapses occurred in cases with quantifiable ddPCR MRD at day +78, while patients with a negative or PNQ MRD by ddPCR at day +78 had a better outcome, emphasizing that high-risk treatment could be offered only to ddPCR quantifiable cases.
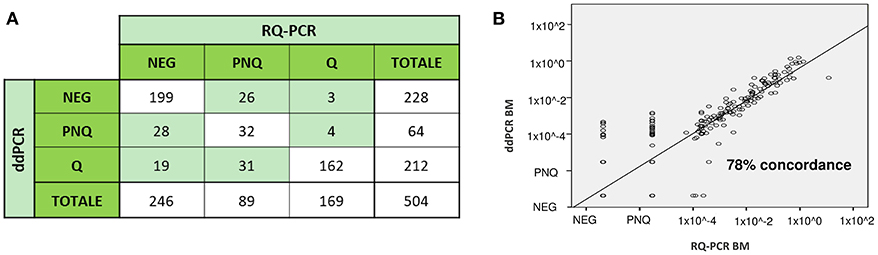
Figure 5. MRD analysis by both RQ-PCR and ddPCR in 504 follow up samples from 176 patients with several hematological malignancies (Acute Lymphoblastic Leukemia, n = 80, Follicular Lymphoma, n = 48, Chronic Lymphocytic Leukemia, n = 40, Mantle Cell Lymphoma, n = 8). The study was performed on bone marrow (BM) and peripheral blood (PB) samples, based on the material availability. MRD detection was concordantly positive or negative in 78% (393/504) of FU samples (r = 0.78, P < 0.0001), while 22% (111/504) were identified as discordant (A). Most of the discordances occurred in FU samples with a low level of disease - positive not quantifiable or negative—and did not appear to cluster in specific disease subsets. Overall, the use of ddPCR significantly reduced the proportion of PNQ samples compared to RQ-PCR (64/504 [13%] vs. 89/504 [18%], respectively) (p = 0.03), increasing the proportion of Q samples (212/504 [42%] vs. 169/504 [33.5%], p = 0.006). In (B) is reported the concordance rate (78%) between the two methods on all BM samples analyzed (unpublished data). Q, positive and quantifiable; PNQ, positive and not quantifiable; NEG, negative.
No established guidelines for ddPCR MRD analysis and interpretation have so far been defined. A major standardization effort is underway within the EuroMRD Consortium.
Several groups have shown the value of next-generation sequencing (NGS) technologies for MRD detection in precursor and mature B-cell tumors (80–82) NGS can be used to detect clone-specific IG/TR index sequences; clonal sequences detected at diagnosis can be re-detected and quantified in each follow-up sample. By using universal primers, this method allows to monitor all IG/TR gene rearrangements at the same time, providing a complete picture not only of the residual leukemia but also of the normal immune repertoire (82).
Sensitivity is a critical aspect in MRD detection. Methods allowing a sensitivity higher than 10−5 (routinely achieved by RQ-PCR) might be of interest to identify very low-level disease. Studies using the NGS platform in ALL and chronic lymphocytic leukemia have demonstrated that a sensitivity level of 10−6 (81, 83) is achievable when higher amounts of DNA are used (Table 2 and Figure 6). This is reflected in the possibility of detecting early clonal evolution, a relatively frequent occurrence in relapsed ALL (84).
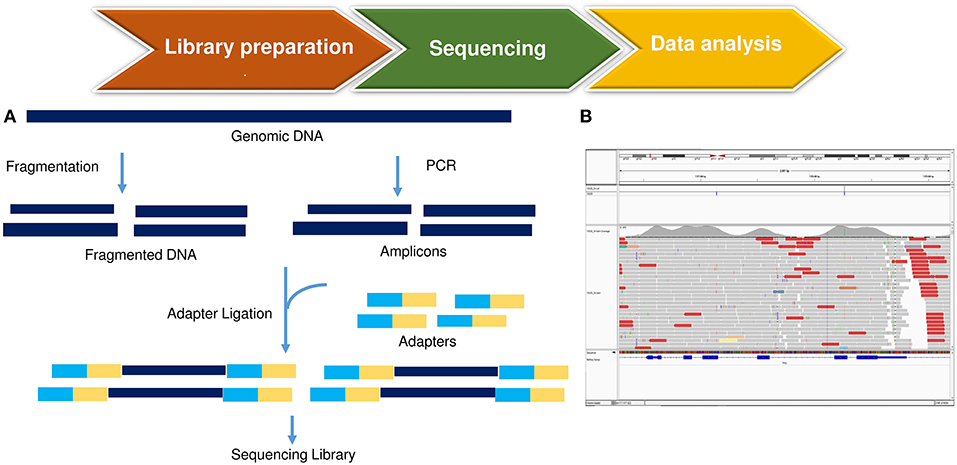
Figure 6. An example of NGS MRD analysis. (A) starting from genomic DNA, a library is prepared by fragmentation and conjugation with adaptive sequences, composed with few nucleotides. The library is subsequently amplified and sequenced, with the production of so-called ≪reads≫. (B) Data analysis is performed through the use of bioinformatic tools, that align experiment-derived reads to a reference genome.
Many authors have reported that NGS appears more specific than RQ-PCR in predicting relapse in ALL patients after induction (82) as well as after allogenic SCT (85). A comparative MRD analysis between RQ-PCR and NGS showed within the Berlin Frankfurt Münster (BFM) trials a change in the stratification risk, mainly due to different interpretations of the two techniques within low-positive samples (82). The NGS quantitative discrimination is always superimposable to the sensitivity, whilst the RQ-PCR quantitative range is usually inferior to the sensitivity threshold leading to cases which are defined as PNQ, as previously described (81). Another comparative analysis, performed at our Center, between RQ-PCR, ddPCR and NGS has documented a more precise prediction of relapse with the new methods compared to the standard technique and a change in the adult ALL risk stratification (86). However, NGS has a substantial intrinsic complexity and involves major costs. Furthermore, at the moment robust and broadly applicable NGS-based MRD standardized protocols are still not available in academic laboratories. The Euroclonality-NGS Consortium is working to standardize guidelines for analysis and data interpretation.
Through the integration of these technologic innovations, we are moving toward a system that can quickly integrate all the information necessary for a more precise evaluation of the response to treatment in ALL patients. ddPCR and NGS appear to be feasible and attractive alternative methods for MRD assessment that can help to classify more precisely cases that RQ-PCR is unable to detect or quantify.
Clinical Significance of MRD
MRD in All Without Major Molecular Transcripts
The clinical impact of MRD is now widely accepted and is regarded today as the most important prognostic factor in the state-of-the-art management of ALL. MRD can provide different information, according to the timing in which it is performed (very early during treatment, after induction/consolidation, before and after SCT) and, more recently, it can be refined by the evaluation of additional genomic markers.
It is now widely acknowledged that MRD detection should be carried out with molecular methods, as it occurs in European countries; at variance, in the United States MFC, with few exceptions, is more commonly used, also for the lack of standardized guidelines for molecular analysis and data interpretation.
In the modern era, treatments include combination chemotherapy for the achievement of a complete hematologic and clinical remission (CR), followed by post-remission consolidation therapy with or without SCT, plus an effective central nervous system prophylaxis. Several pediatric and adult ALL study groups have established informative checkpoints in their respective treatment protocols. Among these, the initial MRD response to therapy is a relevant prognostic factor in both childhood and adult ALL (87, 88); indeed, MRD negativity at very early time points during induction therapy correlates with a particularly good outcome both in childhood and adult ALL, and is indicative of a high sensitivity to chemotherapy. In children, it has been shown that MRD negativity achieved as early as at day 15 correlates with an excellent outcome; in adults, the early evaluation of MRD is usually carried out at a later timing (i.e., end of induction, week 4) and it again correlates with better survival rates. Some studies have reported that patients with a very rapid tumor clearance after 2 weeks of therapy have a very good prognosis (31, 89). Along the same line, in a MFC-based trial, high levels of disease at day 14 of treatment (>30%) identified a small subgroup of patients with a particularly poor prognosis and a median event-free survival (EFS) and overall survival (OS) of only 9 and 21 months, respectively; however, MRD evaluation was not significant if evaluated at CR achievement (90). It must be again underlined that in European protocols the use of MFC for MRD evaluation is normally substituted by molecular techniques.
The other time points that hold prognostic significance are the end of induction/early consolidation. Indeed, the first pivotal study on molecular MRD analysis was carried out by the German Multicenter Study Group for Adult ALL (GMALL) on a large cohort of Ph- patients with standard-risk and high-risk features (n = 580 in CR) that showed that the molecular response to standard induction and consolidation treatment was the only significant prognostic factor for remission duration and survival in both risk groups (91). These data have been confirmed by others groups, regardless of the cut-off values, MRD technique, timing of MRD analysis and the target patient population chosen (92–94). The PETHEMA group evaluated the role of MRD (by flow-cytometry, cut-off: 5 × 10−4) in 326 adult high-risk Ph- ALL and confirmed that the only prognostic factor was represented by MRD persistence after induction and early consolidation (92). The GRAAL conducted an analysis on 955 patients to assess the role of SCT, taking into account also MRD after induction (week 6, cut-off: 10−3), and again showed that the role of MRD persistence is not abrogated by transplant procedures and that MRD-negative patients could be spared this approach (93). The Northern Italian Study Group (NILG) performed MRD evaluations starting from the end of induction (week 4) and then at weeks 10, 16, and 22 in an adult patient population to assess the feasibility and efficacy of liposome-encapsulated cytarabine for central nervous system prophylaxis along with the use of lineage-targeted systemic methotrexate blocks (2.5 and 5 g/m2 in B- and T-lineage ALL, respectively) and other intensive pediatric-like elements (94). The early MRD response at weeks 4 (end of induction) and 10 had a profound prognostic effect. The relapse risk was very low (17% at 5 years) in the group of week 4 MRD responders and significantly lower (28%) than in non-responders (57%) when week 10 MRD results were examined. Similar results have been preliminary confirmed in the subsequent GIMEMA LAL 1913 (95).
Pivotal studies on the clinical role of MRD in Ph-ALL are summarized in Table 3.
MRD in Ph+ All
The Ph chromosome, leading to the BCR/ABL1 rearrangement, defines the more frequent high risk ALL subset in adults. It is found in 25–30% of patients and its incidence increases with age progression (101). Ph+ ALL was previously regarded as the subgroup with the worse outcome: the introduction of TK inhibitors (TKI) such as imatinib, dasatinib, and ponatinib (102–106), has led to the achievement of a CR in virtually all patients, to improve disease-free survival (DFS) and OS, and to increase the percentage of patients who can undergo a SCT. A common therapeutic approach for adult Ph+ ALL patients is based on the use of a TKI, with or without systemic chemotherapy for CR induction, followed by consolidation and when possible SCT. As in Ph- ALL, MRD has an important role in the management of this disease. The GIMEMA has clearly shown that the degree of MRD reduction correlates with improved DFS, regardless of the inhibitor used (107). Lee and colleagues showed that also the timing of MRD clearance is important for patients' stratification: in fact, patients who display a very early clearance have a significantly better outcome (108).
Pivotal studies on the clinical role of MRD in Ph+ ALL are summarized in Table 4.
In Ph+ ALL, MRD can also drive therapeutic decisions: indeed, a persistent MRD positivity and/or its reappearance can underlie the presence of resistant mutations, particularly the T315I for which alternative approaches, including novel TKIs (namely ponatinib) and/or therapies based on the combination of TKI with immunotherapeutic strategies, particularly blinatumomab have been evaluated (116).
An open issue in this setting is represented by the cases who are persistently MRD negative; in fact, while in the past SCT was considered mandatory in all Ph+ ALL patients, if clinically fit, it is currently debated if these patients might be spared this procedure, in line with the clinical policy routinely applied to Ph- ALL (102–107, 113, 115).
Finally, there is growing interest in evaluating MRD using more than one marker: in pediatric Ph+ ALL, Hovorkova and colleagues (53) showed that roughly 20% of children have significantly higher BCR/ABL1 levels (assessed by evaluating both genomic DNA and RNA fusion levels) than IG/TR or IKZF1 deletions, indicating that the BCR/ABL1 signal arises from other hemopoietic cells. Along the same line, Cazzaniga et al. (117) showed that MRD positivity after induction and consolidation (evaluated by IG/TR) is strongly associated with relapse. A formal comparison between IG/TR and BCR/ABL1 evaluation showed comparable results in terms of relapse risk: however, concordance between the two techniques was only 69% and, again, BCR/ABL1 levels were significantly higher than IG/TR. In the adult setting, Clappier et al. (118) evaluated IG/TR and BCR/ABL1 levels (using genomic DNA and RNA) and again proved that there is an overall poor concordance between genomic DNA BCR/ABL1 and IG/TR results (correlation coefficient = 0.51), while a good concordance is detected between genomic and RNA BCR/ABL1 levels (correlation coefficient = 0.8). Furthermore, the authors showed that there are two subsets of patients: the first group with concordant MRD results and the second displaying discordant results among IG/TR and BCR/ABL1, with BCR/ABL1 levels being higher. Interestingly, discordant cases harbor more often the p210 protein isoform, and have less frequently IKZF1 deletions, again suggesting that the BCR/ABL1 signal derives from other cells rather than lymphoblasts and that these cases might resemble a “CML-like” subset. At present, however, it is not defined which marker is most suitable for therapeutic decisions.
MRD and Stem Cell Transplantation
As mentioned, MRD after induction/early consolidation is the most important decision-making parameter for on allogeneic transplant, a procedure still aggravated by transplant-related mortality and toxicity, observed in about 20% of patients (119, 120). In addition, several studies have analyzed the prognostic impact on outcome of a MRD (+) status at the time of SCT (121, 122) and others have shown the prognostic relevance of pre-transplant MRD assessment in adults (98, 101, 123). In particular, Bassan et al. (98) showed that patients with MRD levels ≥10−3 at week 16 and/or week 22 (i.e., after consolidation) had a worse post-transplant outcome with a 6 years relapse incidence of 64% compared to 23% in patients with MRD levels <10−3. A recent metanalysis on 21 published reports, including over 2,000 patients, confirms that a pre-transplant positive MRD is a significant negative predictor of relapse-free survival (RFS), event-free survival (EFS), and OS; as expected, a positive MRD prior to transplant was not associated with a higher rate of non-relapse mortality (124). Taken together, these results show that MRD evaluation before transplant is extremely useful for treatment intensification, since we can offer the opportunity to adequately use immunotherapeutic compounds (e.g., blinatumomab, inotuzumab, and in the future possibly CAR-T cells) aimed at obtaining a MRD negative status.
With regard to the post-transplant setting, MRD monitoring has been much less frequently used after SCT because donor chimerism monitoring provides an alternative for early relapse detection; nevertheless, it has been shown that a IG/TR-based MRD assessment allowed an earlier and more specific detection of an impending relapse compared to chimerism analysis, showing that MRD positivity was an independent significant predictor of risk of relapse (125). Another MFC-based trial showed that patients with evidence of MRD after SCT had significantly worse outcomes compared to patients without evidence of MRD (126). In the pediatric context, pre-SCT MRD resulted the only independent prognostic factor in a multivariate analysis and after-SCT MRD is considered a reliable marker for early detection of impending relapses (127, 128).
Relapse and Clonal Evolution
At relapse, molecular evaluation of IG/TR markers previously used for MRD monitoring might be useful to confirm the persistence of the same clone. However, it has some potential pitfalls, mostly represented by the phenomenon of clonal evolution. The analysis of all molecular markers at first diagnosis and at the time of relapse may reveal a different origin of the predominant clone (84, 129). Indeed, the loss of a molecular marker at relapse, or the expansion of a clonal marker expressed at the subclonal level at diagnosis, is a relatively frequent event: therefore, MRD assessment should be carried out with several targets (84). Finally, it must be reminded the clonal evolution can vary according to the site of relapse (medullary or extramedullary).
MRD and Novel Markers
The extensive characterization of the genetic bases of ALL is leading to an attempt of combining MRD with other markers: a pivotal study was carried out by the GRAAL group (130) which evaluated 423 young adults with Ph- ALL in first remission (both B- and T-lineage ALL), demonstrating that a higher risk of relapse was associated with MRD persistence and can be further refined by the presence of IKZF1 deletions in B-lineage ALL, and by the absence of NOTCH1/FBXW7 mutation, and/or by the presence of N/K-RAS mutation and/or PTEN gene alteration in T-cell ALL.
Likewise, in childhood ALL, the generation of a risk score based on the combination of MRD at day 33, the presence of IKZF1 intragenic deletion and P2RY8-CRLF2, which proved more discriminative of outcome that MRD evaluation alone, has been recently reported (131).
MRD and Novel Agents
Novel therapies, such as monoclonal antibodies, bispecific T-cell engagers, or chimeric antigen receptor T cells (CART), are an exciting advancement in the immunotherapeutic treatment of relapse/refractory BCP-ALL. These new therapeutic approaches make MRD an almost perfect therapeutic target, considering that MRD+ patients harbor significantly less leukemic cells and therefore a more manageable clinical profile than cases in hematologic relapse.
Blinatumomab
Blinatumomab is a bispecific anti-CD19 and anti-CD3 construct recruiting cytotoxic T cells against CD19+ blast T cells. Blinatumomab can bridge malignant B cells directly to CD3-positive T cells, bypassing TCR specificity and major histocompatibility complex (MHC) class I molecules (132, 133) and induces T-cell activation, release of inflammatory cytokine production, specifically IL-2, IFN-γ, TNF-α, IL-4, IL-6, and IL-10 (134). Blinatumomab is the first antibody approved for treatment of refractory ALL and, more recently, for the treatment of MRD+ patients. In relapsed/refractory ALL, blinatumomab has led to morphologic responses ranging from 43 to 69% of patients (135, 136) with 76 to 88% of responding patients being MRD negative. Patients who achieved a negative molecular MRD status had a longer survival than patients who remained MRD positive (137, 138). In MRD+ patients, blinatumomab induced a complete MRD response in 78% of cases and, as expected, MRD responders has a longer RFS than non-responders. A small fraction of complete MRD responders did not undergo transplant and is still in continuous CR (139, 140).
Inotuzumab Ozogamicin
Inotuzumab ozogamicin is a conjugated antibody-drug (ADC) consisting of a monoclonal antibody (mAb) directed to CD22, an antigen present on the cancer cells of almost all patients with B-cell precursor ALL, linked to a cytotoxic agent. When inotuzumab ozogamicin binds to the CD22 antigen on B cells, it is internalized in the cell, where the cytotoxic agent, calicheamicin, is released to destroy the malignant cell. The drug is approved for the treatment, in monotherapy, of relapsed/refractory adult ALL patients CD22-positive. Patients treated with inotuzumab ozogamicin reached response rates ranging from 58 to 81%, with 72–78% of these having MRD results below 0.01% (141, 142) by flow cytometry assessment. While this compound appears to be extremely effective in reinducing responses, it must be underlined that CR duration is usually short, and therefore SCT must be performed as soon as possible.
CAR-T
CAR-T cells are patient or, less frequently, donor-derived normal T cells molecularly engineered to express a T-cell receptor mediating cytotoxicity toward anti-CD19 (in most cases). After CAR-T cells are infused into a patient, they act as a “living drug” against cancer cells: they bind to the target, become activated, proliferate and exert their cytotoxic activity. Several groups have shown that most of the responding patients (both children and adults) become MRD negative (at least by FCM) (143–145) and maintain this status for several months or years (146, 147). Data on the prognostic value of MRD in this setting are still preliminary. However, differently from first-line chemotherapeutic approaches, relapse is observed also in patients reaching a MRD negativity, mostly because of the loss of CD19. Therefore, MRD response in this setting seems to be an essential but not sufficient criterion for the definition of long-term remissions. Higher sensitivities or earlier MRD assessments might be necessary to identify a subgroup of patients with a particularly rapid and deep MRD response and a better prognosis.
Conclusions
MRD is a powerful and independent predictor of outcome in both children and adult ALL, during treatment, in the pre- and post-SCT settings, with different prognostic meanings on the base of the clinical context.
Molecular rearrangements (gene fusion and IG/TR gene rearrangements) are widely used as targets to detect residual leukemic cells in ALL patients, although new molecular markers could be used for prognostic and therapeutic purposes, also to improve the number of evaluable patients. The alterations of IKZF1 and FLT3-ITD, might potentially represent new MRD molecular targets
Technically, MFC and RQ-PCR are the most broadly applied consolidated methods for MRD monitoring, although they present intrinsic limitations that must necessarily be overcome. ddPCR, NGS, and NGF, the standardization of which is underway, have shown to be important tools to evaluate MRD in the research setting, and will probably be soon incorporated in clinical trials due to their ability to overcome the limitation of standard approaches.
At present, the advent of powerful new agents such as ponatinib for Ph+ ALL and novel immunotherapeutic agents for B-lineage ALL (blinatumomab, inotuzumab ozogamicin) and CAR-T cells are further improving CR and MRD negativity rates, with benefits in long-term survival and a potential reduction of therapy-related toxicities. However, the prognostic relevance of MRD in the setting of novel immunotherapies still needs to be evaluated.
In the next future, MRD will certainly be the main tool to design innovative treatment algorithms including immunotherapeutic strategies and possibly sparing chemotherapy/transplant with the final aim of curing always more patients with ALL.
Author Contributions
ID wrote the manuscript. SC wrote the manuscript and critically revised the manuscript. MD performs flow cytometry analysis. LE performs molecular analysis of fusion genes by RQ-PCR and ddPCR. MC, LD, and RS perform molecular analysis of antigen-receptor gene rearrangements by RQ-PCR and ddPCR. MM performs next generation sequencing analysis. AV provides clinical data. AG and RF critically revised the manuscript.
Funding
The authors wish to thank the Associazione Italiana per la Ricerca sul Cancro (AIRC), Metastases Special Program (N° 21198), 5 × 1000, Milan, Italy (RF).
Conflict of Interest Statement
The authors declare that the research was conducted in the absence of any commercial or financial relationships that could be construed as a potential conflict of interest.
References
1. Inaba H, Greaves M, Mullighan CG. Acute lymphoblastic leukaemia. Lancet. (2013) 381:1943–55. doi: 10.1016/S0140-6736(12)62187-4
2. Faderl S, O'Brien S, Pui C-H, Stock W, Wetzler M, Hoelzer D, et al. Adult acute lymphoblastic leukemia: concepts and strategies. Cancer. (2010) 116:1165–76. doi: 10.1002/cncr.24862
3. Redaelli A, Laskin BL, Stephens JM, Botteman MF, Pashos CL. A systematic literature review of the clinical and epidemiological burden of acute lymphoblastic leukaemia (ALL). Eur J Cancer Care. (2005) 14:53–62. doi: 10.1111/j.1365-2354.2005.00513.x
4. Pui C-H, Evans WE. Treatment of acute lymphoblastic leukemia. N Engl J Med. (2006) 354:166–78. doi: 10.1056/NEJMra052603
5. Siegel R, Miller K, Jemal A. Cancer statistics, 2015. CA Cancer J Clin. (2015) 65:5–29. doi: 10.3322/caac.21254
6. Gökbuget N, Raff R, Brüggemann M, Flohr T, Scheuring U, Pfeifer H, et al. Risk/MRD adapted GMALL trials in adult ALL. Ann Hematol. (2004) 83:S129–31. doi: 10.1007/s00277-004-0850-2
7. Conter V, Bartram CR, Valsecchi MG, Schrauder A, Panzer-Grumayer R, Moricke A, et al. Molecular response to treatment redefines all prognostic factors in children and adolescents with B-cell precursor acute lymphoblastic leukemia: results in 3184 patients of the AIEOP-BFM ALL 2000 study. Blood. (2010) 115:3206–14. doi: 10.1182/blood-2009-10-248146
8. Stary J, Zimmermann M, Campbell M, Castillo L, Dibar E, Donska S, et al. Intensive chemotherapy for childhood acute lymphoblastic leukemia: Results of the randomized intercontinental trial ALL IC-BFM 2002. J Clin Oncol. (2014) 32:174–84. doi: 10.1200/JCO.2013.48.6522
9. Vora A, Goulden N, Wade R, Mitchell C, Hancock J, Hough R, et al. Treatment reduction for children and young adults with low-risk acute lymphoblastic leukaemia defined by minimal residual disease (UKALL 2003): a randomised controlled trial. Lancet Oncol. (2013) 14:199–209. doi: 10.1016/S1470-2045(12)70600-9
10. Pieters R, de Groot-Kruseman H, Van der Velden V, Fiocco M, van den Berg H, de Bont E, et al. Successful therapy reduction and intensification for childhood acute lymphoblastic leukemia based on minimal residual disease monitoring: Study ALL10 From the Dutch Childhood Oncology Group. J Clin Oncol. (2016) 34:2591–601. doi: 10.1200/JCO.2015.64.6364
11. Tzoneva G, Perez-Garcia A, Carpenter Z, Khiabanian H, Tosello V, Allegretta M, et al. Activating mutations in the NT5C2 nucleotidase gene drive chemotherapy resistance in relapsed ALL. Nat Med. (2013) 19:368–71. doi: 10.1038/nm.3078
12. Einsiedel HG, Von Stackelberg A, Hartmann R, Fengler R, Schrappe M, Janka-Schaub G, et al. Long-term outcome in NGS for MRD Assessment in ALL 489 children with relapsed ALL by risk-stratified salvage therapy: Results of trial Acute Lymphoblastic Leukemia-Relapse Study of the Berlin-Frankfurt-Münster Group 87. J Clin Oncol. (2005) 23:7942–50. doi: 10.1200/JCO.2005.01.1031
13. Fielding AK, Richards SM, Chopra R, Lazarus HM, Litzow MR, Buck G, et al. Medical research council of the United Kingdom adult ALL working party; eastern cooperative oncology group. outcome of 609 adults after relapse of acute lymphoblastic leukemia (ALL); an MRC UKALL12/ECOG 2993 study. Blood. (2007) 109:944–50. doi: 10.1182/blood-2006-05-018192
14. Gökbuget N, Stanze D, Beck J, Diedrich H, Horst HA, Hüttmann A, et al. German multicenter study group for adult acute lymphoblastic leukemia. Outcome of relapsed adult lymphoblastic leukemia depends on response to salvage chemotherapy, prognostic factors, and performance of stem cell transplantation. Blood. (2012) 120:2032–41. doi: 10.1182/blood-2011-12-399287
15. van der Velden VH, Hochhaus A, Cazzaniga G, Szczepański T, Gabert J, van Dongen JJ. Detection of minimal residual disease in hematologic malignancies by real-time quantitative PCR: Principles, approaches, and laboratory aspects. Leukemia. (2003) 17:1013–34. doi: 10.1038/sj.leu.2402922
16. van der Velden VH, Cazzaniga G, Schrauder A, Hancock J, Bader P, Panzer-Grumayer ER, et al. European Study Group on MRD detection in ALL (ESG-MRD-ALL). Analysis of minimal residual disease by Ig/TCR gene rearrangements: Guidelines for interpretation of real-time quantitative PCR data. Leukemia. (2007) 21:604–11. doi: 10.1038/sj.leu.2404586
17. Campana D. Status of minimal residual disease testing in childhood haematological malignancies. Br J Haematol. (2008) 143:481–9. doi: 10.1111/j.1365-2141.2008.07350.x
18. Flohr T, Schrauder A, Cazzaniga G, Panzer-Grümayer R, van der Velden V, Fischer S, et al. International BFM Study Group (I-BFM-SG). Minimal residual disease directed risk stratification using real-time quantitative PCR analysis of immunoglobulin and T-cell receptor gene rearrangements in the international multicenter trial AIEOP-BFM ALL 2000 for childhood acute lymphoblastic leukemia. Leukemia. (2008) 22:771–82. doi: 10.1038/leu.2008.5
19. Dworzak MN, Gaipa G, Ratei R, Veltroni M, Schumich A, Maglia O, et al. Standardization of flow cytometric minimal residual disease evaluation in acute lymphoblastic leukemia: multicentric assessment is feasible. Cytometry B Clin Cytom. (2008) 74B:331–40. doi: 10.1002/cyto.b.20430
20. Cazzaniga G, Valsecchi MG, Gaipa G, Conter V, Biondi A. Defining the correct role of minimal residual disease tests in the management of acute lymphoblastic leukaemia. Br J Haematol. (2011) 155:45–52. doi: 10.1111/j.1365-2141.2011.08795.x
21. Coustan-Smith E, Sancho J, Hancock ML, Razzouk BI, Ribeiro RC, Rivera GK, et al. Use of peripheral blood instead of bone marrow to monitor residual disease in children with acute lymphoblastic leukemia. Blood. (2002) 100:2399–402. doi: 10.1182/blood-2002-04-1130
22. Brüggemann M, Kotrova M. Minimal residual disease in adult ALL: technical aspects and implications for correct clinical interpretation. Blood Adv. (2017) 1:2456–66. doi: 10.1182/asheducation-2017.1.13
23. Chen X, Wood BL. Monitoring minimal residual disease in acute leukemia: technical challenges and interpretive complexities. Blood Rev. (2017) 31:63–75. doi: 10.1016/j.blre.2016.09.006
24. Baumgarth N, Roederer M. A practical approach to multicolor flow cytometry for immunophenotyping. J Immunol Methods. (2000) 243:77–97. doi: 10.1016/S0022-1759(00)00229-5
25. De Rosa SC, Brenchley J, Roederer M. Beyond six colors: a new era in flow cytometry. Nature Med. (2003) 9:112–7. doi: 10.1038/nm0103-112
26. Bendall SC, Nolan GP, Roederer M, Chattopadhyay PK. A deep profiler's guide to cytometry. Trends Immunol. (2012) 33:323–32. doi: 10.1016/j.it.2012.02.010
27. Service RF. Getting a charge out of plastics. Science. (2000) 290:425–7. doi: 10.1126/science.290.5491.425
28. Chattopadhyay PK, Price DA, Harper TF, Betts MR, Yu J, Gostick E, et al. Quantum dot semiconductor nanocrystals for immunophenotyping by polychromatic flow cytometry. Nature Med. (2006) 12:972–7. doi: 10.1038/nm1371
29. Preffer F, Dombkowski D. Advances in complex multiparameter flow cytometry technology: applications in stem cell research. Cytometry B Clin Cytom. (2009) 76B:295–314. doi: 10.1002/cyto.b.20480
30. Denys B, van der Sluijs-Gelling AJ, Homburg C, van der Schoot CE, deHaas V, Philippe J, et al. Improved flow cytometric detection of minimal residual disease in childhood acute lymphoblastic leukemia. Leukemia. (2012) 27:635–41. doi: 10.1038/leu.2012.231
31. Basso G, Veltroni M, Valsecchi MG, Dworzak MN, Ratei R, Silvestri D, et al. Risk of relapse of childhood acute lymphoblastic leukemia is predicted by flow cytometric measurement of residual disease on day 15 bone marrow. J Clin Oncol. (2009) 27:5168–74. doi: 10.1200/JCO.2008.20.8934
32. Fronkova E, Muzikova K, Mejstrikova E, Kovac M, Formankova R, Sedlacek P, et al. B-cell reconstitution after allogeneic SCT impairs minimal residual disease monitoring in children with ALL. Bone Marrow Transplant. (2008) 42:187–96. doi: 10.1038/bmt.2008.122
33. Dworzak MN, Gaipa G, Schumich A, Maglia O, Ratei R, Veltroni M, et al. Modulation of antigen expression in B-cell precursor acute lymphoblastic leukemia during induction therapy is partly transient: evidence for a drug-induced regulatory phenomenon. Results of the AIEOP-BFM-ALL-FLOW-MRD-Study group. Cytometry B Clin Cytom. (2010) 78:147–53. doi: 10.1002/cyto.b.20516
34. Kalina T, Flores-Montero J, van der Velden VH, Martin-Ayuso M, Bottcher S, Ritgen M, et al. EuroFlow standardization of flow cytometer instrument settings and immunophenotyping protocols. Leukemia. (2012) 26:1986–2010. doi: 10.1038/leu.2012.122
35. Kalina T, Brdickova N, Glier H, Fernandez P, Bitter M, Flores-Montero J, et al. Frequent issues and lessons learned from EuroFlow QA. J Immunol Methods. (2018). doi: 10.1016/j.jim.2018.09.008. [Epub ahead of print].
36. Cazzaniga G, Biondi A. Molecular monitoring of childhood acute lymphoblastic leukemia using antigen receptor gene rearrangements and quantitative polymerase chain reaction technology. Haematologica. (2005) 90:382–90.
37. van Dongen JJ, Langerak AW, Brüggemann M, Evans PA, Hummel M, Lavender FL, et al. Design and standardization of PCR primers and protocols for detection of clonal immunoglobulin and T-cell receptor gene recombinations in suspect lymphoproliferations: report of the BIOMED-2 Concerted Action BMH4-CT98-3936. Leukemia. (2003) 17:2257–317. doi: 10.1038/sj.leu.2403202
38. Szczepański T, Beishuizen A, Pongers-Willemse MJ, Hählen K, Van Wering ER, Wijkhuijs AJ, et al. Cross lineage T cell receptor gene rearrangements occur in more than ninety percent of childhood precursor-B acute lymphoblastic leukemias: alternative PCR targets for detection of minimal residual disease. Leukemia. (1999) 13:196–205. doi: 10.1038/sj.leu.2401277
39. Szczepański T, Pongers-Willemse MJ, Langerak AW, Harts WA, Wijkhuijs AJ, van Wering ER, et al. Ig heavy chain gene rearrangements in T-cell acute lymphoblastic leukemia exhibit predominant DH6-19 and DH7-27 gene usage, can result in complete V-D-J rearrangements, and are rare in T-cell receptor alpha beta lineage. Blood. (1999) 93:4079–85.
40. Langerak AW, Szczepański T, van der Burg M, Wolvers-Tettero IL, van Dongen JJ. Heteroduplex PCR analysis of rearranged T cell receptor genes for clonality assessment in suspect T cell proliferations. Leukemia. (1997) 11:2192–9. doi: 10.1038/sj.leu.2400887
41. Germano G, del Giudice L, Palatron S, Giarin E, Cazzaniga G, Biondi A, et al. Clonality profile in relapsed precursor-B-ALL children by GeneScan and sequencing analyses. Consequences on minimal residual disease monitoring. Leukemia. (2003) 17:1573–82. doi: 10.1038/sj.leu.2403008
42. Verhagen OJ, Willemse MJ, Breunis WB, Wijkhuijs AJ, Jacobs DC, Joosten SA, et al. Application of germline IGH probes in real-time quantitative PCR for the detection of minimal residual disease in acute lymphoblastic leukemia. Leukemia. (2000) 14:1426–35. doi: 10.1038/sj.leu.2401801
43. Bruggemann M, Raff T, Flohr T, Gokbuget N, Nakao M, Droese J, et al. Clinical significance of minimal residual disease quantification in adult patients with standard-risk acute lymphoblastic leukemia. Blood. (2006) 107:1116–23 doi: 10.1182/blood-2005-07-2708
44. Bruggemann M, Schrauder A, Raff T, Pfeifer H, Dworzak M, Ottmann OG, et al. Standardized MRD quantification in European ALL trials: proceedings of the Second International Symposium on MRD assessment in Kiel, Germany, 18-20 September 2008. Leukemia. (2010) 24:521–35. doi: 10.1038/leu.2009.268
45. Szczepanski T, van der Velden VH, Raff T, Jacobs DC, van Wering ER, Brüggemann M, et al. Comparative analysis of T-cell receptor gene rearrangements at diagnosis and relapse of T-cell acute lymphoblastic leukemia (T-ALL) shows high stability of clonal markers for monitoring of minimal residual disease and reveals the occurrence of second T-ALL. Leukemia. (2003) 17:2149–56. doi: 10.1038/sj.leu.2403081
46. van Dongen JJ, Macintyre EA, Gabert JA, Delabesse E, Rossi V, Saglio G, et al. Standardized RT-PCR analysis of fusion gene transcripts from chromosome aberrations in acute leukemia for detection of minimal residual disease. Report of the BIOMED-1 Concerted Action: investigation of minimal residual disease in acute leukemia. Leukemia. (1999) 13:1901–28. doi: 10.1038/sj.leu.2401592
47. Gabert J, Beillard E, van der Velden VH, Bi W, Grimwade D, Pallisgaard N, et al. Standardization and quality control studies of ‘real-time’ quantitative reverse transcriptase polymerase chain reaction of fusion gene transcripts for residual disease detection in leukemia - a Europe against cancer program. Leukemia. (2003) 17:2318–57. doi: 10.1038/sj.leu.2403135
48. D'Angiò M, Valsecchi MG, Testi AM, Conter V, Nunes V, Parasole R, et al. Clinical features and outcome of SIL/TAL1-positive T-cell acute lymphoblastic leukemia in children and adolescents: a 10-year experience of the AIEOP group. Haematologica. (2015) 100:e10–13. doi: 10.3324/haematol.2014.112151
49. Elia L, Mancini M, Moleti L, Meloni G, Buffolino S, Krampera M, et al. A multiplex reverse transcriptase polymerase chain reaction strategy for the diagnostic molecular screening of chimeric genes: a clinical evaluation on 170 patients with acute lymphoblastic leukemia. Haematologica. (2003) 88:275–9.
50. Campana D. Determination of minimal residual disease in leukaemia patients. Br J Haematol. (2003) 121:823–38. doi: 10.1046/j.1365-2141.2003.04393.x
51. Pfeifer H, Cazzaniga G, van der Velden VHJ, Cayuela JM, Schäfer B, Spinelli O, et al. Standardisation and consensus guidelines for minimal residual disease assessment in Philadelphia-positive acute lymphoblastic leukemia (Ph + ALL) by real-time quantitative reverse transcriptase PCR ofe1a2 BCR-ABL1. Leukemia. (2019). doi: 10.1038/s41375-019-0413-0. [Epub ahead of print].
52. Burmeister T, Marschalek R, Schneider B, Meyer C, Gökbuget N, Schwartz S, et al. Monitoring minimal residual disease by quantification of genomic chromosomal breakpoint sequences in acute leukemias with MLL aberrations. Leukemia. (2006) 20:451–7. doi: 10.1038/sj.leu.2404082
53. Hovorkova L, Zaliova M, Venn NC, Bleckmann K, Trkova M, Potuckova E, et al. Monitoring of childhood ALL using BCR-ABL1 genomic breakpoints identifies a subgroup with CML-like biology. Blood. (2017) 129:2771–81. doi: 10.1182/blood-2016-11-749978
54. Leutenegger CM, Higgins J, Matthews TB, Tarantal AF, Luciw PA, Pedersen NC, et al. Real-time TaqMan PCR as a specific and more sensitive alternative to the branched-chain DNA assay for quantitation of simian immunodeficiency virus RNA. AIDS Res Hum Retroviruses. (2001) 17:243–51. doi: 10.1089/088922201750063160
55. van Dongen JJ, Lhermitte L, Böttcher S, Almeida J, van der Velden VH, Flores-Montero J, et al. EuroFlow Consortium (EU-FP6, LSHB-CT-2006-018708). EuroFlow antibody panels for standardized n-dimensional flow cytometric immunophenotyping of normal, reactive and malignant leukocytes. Leukemia. (2012) 26:1908–75. doi: 10.1038/leu.2012.120
56. Pedreira CE, Costa ES, Lecrevisse Q, van Dongen JJ, Orfao A, EuroFlow Consortium. Overview of clinical flow cytometry data analysis: recent advances and future challenges. Trends Biotechnol. (2013) 31:415–25. doi: 10.1016/j.tibtech.2013.04.008
57. Paiva B, van Dongen JJ, Orfao A. New criteria for response assessment: role of minimal residual disease in multiple myeloma. Blood. (2015) 125:3059–68. doi: 10.1182/blood-2014-11-568907
58. Gaipa G, Basso G, Maglia O, Leoni V, Faini A, Cazzaniga G, et al. I-BFM-ALL-FCM-MRD study group. Drug induced immunophenotypic modulation in childhood ALL: implications for minimal residual disease detection. Leukemia. (2005) 19:49–56. doi: 10.1038/sj.leu.2403559
59. lores-Montero J, Sanoja-Flores L, Paiva B, Puig N, García-Sánchez O, Böttcher S, et al. Next generation flow for highly sensitive and standardized detection of minimal residual disease in multiple myeloma. Leukemia. (2017) 31:2094–103. doi: 10.1038/leu.2017.29
60. van Dongen JJ, van der Velden VH, Brüggemann M, Orfao A. Minimal residual disease diagnostics in acute lymphoblastic leukemia: need for sensitive, fast, and standardized technologies. Blood. (2015) 125:3996–4009. doi: 10.1182/blood-2015-03-580027
61. Huggett JF, Whale A. Digital PCR as a novel technology and its potential implications for molecular diagnostics. Clin Chem. (2013) 59:1691–3. doi: 10.1373/clinchem.2013.214742
62. Hindson BJ, Ness KD, Masquelier DA, Belgrader P, Heredia NJ, Makarewicz AJ, et al. High-throughput droplet digital PCR system for absolute quantitation of DNA copy number. Anal Chem. (2011) 83:8604–10. doi: 10.1021/ac202028g
63. Pinheiro LB, Coleman VA, Hindson CM, Herrmann J, Hindson BJ, Bhat S, et al. Evaluation of a droplet digital polymerase chain reaction format for DNA copy number quantification. Anal Chem. (2012) 84:1003–11. doi: 10.1021/ac202578x
64. Sanders R, Huggett JF, Bushell CA, Cowen S, Scott DJ, Foy CA. Evaluation of digital PCR for absolute DNA quantification. Anal Chem. (2011) 83:6474–84. doi: 10.1021/ac103230c
65. Whale AS, Huggett JF, Cowen S, Speirs V, Shaw J, Ellison S, et al. Comparison of microfluidic digital PCR and conventional quantitative PCR for measuring copy number variation. Nucleic Acids Res. (2012) 40:e82. doi: 10.1093/nar/gks203
66. Hindson CM, Chevillet JR, Briggs HA, Gallichotte EN, Ruf IK, Hindson BJ, et al. Absolute quantification by droplet digital PCR versus analog real time PCR. Nat Methods. (2013) 10:1003–5. doi: 10.1038/nmeth.2633
67. Vincent ME, Liu W, Haney EB, Ismagilov RF. Microfluidic stochastic confinement enhances analysis of rare cells by isolating cells and creating high density environments for control of diffusible signals. Chem Soc Rev. (2010) 39:974–84. doi: 10.1039/b917851a
68. Yang R, Paparini A, Monis P, Ryan U. Comparison of next-generation droplet digital PCR (ddPCR) with quantitative PCR (qPCR) for enumeration of Cryptosporidium oocysts in faecal samples. Int J Parasitol. (2014) 44:1105–13. doi: 10.1016/j.ijpara.2014.08.004
69. Tsui NB, Kadir RA, Chan KC, Chi C, Mellars G, Tuddenham EG, et al. Non invasive prenatal diagnosis of hemophilia by microfluidics digital PCR analysis of maternal plasma DNA. Blood. (2011) 117:3684–91. doi: 10.1182/blood-2010-10-310789
70. Pornprasert S, Prasing W. Detection of alpha (0)-thalassemia South-East Asian-type deletion by droplet digital PCR. Eur J Haematol. (2014) 92:244–8. doi: 10.1111/ejh.12246
71. Stahl T, Böhme MU, Kröger N, Fehse B. Digital PCR to assess hematopoietic chimerism after allogeneic stem cell transplantation. Exp Hematol. (2015) 43:462–8. doi: 10.1016/j.exphem.2015.02.006
72. Zagaria A, Anelli L, Coccaro N, Tota G, Casieri P, Cellamare A, et al. BCR-ABL1 e6a2 transcript in chronic myeloid leukemia: biological features and molecular monitoring by droplet digital PCR. Virchows Archiv. (2015) 467:357–63. doi: 10.1007/s00428-015-1802-z
73. Albano F, Zagaria A, Anelli L, Coccaro N, Tota G, Brunetti C, et al. Absolute quantification of the pretreatment PML-RARA transcript defines the relapse risk in acute promyelocytic leukemia. Oncotarget. (2015) 6:13269–77. doi: 10.18632/oncotarget.3773
74. Coccaro N, Anelli L, Zagaria A, Casieri P, Tota G, Orsini P, et al. Droplet digital PCR is a robust tool for monitoring minimal residual disease in adult Philadelphia-positive acute lymphoblastic leukemia. J Mol Diagn. (2018) 20:474–82. doi: 10.1016/j.jmoldx.2018.03.002
75. Drandi D, Kubiczkova-Besse L, Ferrero S, Dani N, Passera R, Mantoan B, et al. Minimal residual disease detection by droplet digital PCR in multiple myeloma, mantle cell lymphoma, and follicular lymphoma: a comparison with real-time PCR. J Mol Diagn. (2015) 17:652–60. doi: 10.1016/j.jmoldx.2015.05.007
76. Della Starza I, Nunes V, Cavalli M, De Novi LA, Ilari C, Apicella V, et al. Comparative analysis between RQ-PCR and digital-droplet-PCR of immunoglobulin/Tcell receptor gene rearrangements to monitor minimal residual disease in acute lymphoblastic leukaemia. Br J Haematol. (2016) 174:541–9. doi: 10.1111/bjh.14082
77. Cavalli M, De Novi LA, Della Starza I, Cappelli LV, Nunes V, Pulsoni A, et al. Comparative analysis between RQ-PCR and digital droplet PCR of BCL2/IGH gene rearrangement in the peripheral blood and bone marrow of early stage follicular lymphoma. Br J Haematol. (2017) 177:588–96. doi: 10.1111/bjh.14616
78. Della Starza I, Del Giudice I, Menale L, Cappelli LV, Cavalli M, De Novi LA, et al. Minimal residual disease (MRD) detection by digital-droplet-PCR (ddPCR) in lymphoid malignancies. Haematologica. (2018) 103:S1–129.
79. Della Starza I, Nunes V, Lovisa F, Silvestri D, Cavalli M, Garofalo A, et al. Digital-droplet PCR, an accurate method for IG/TR PCR-MRD stratification in childhood acute lymphoblastic leukemia. Blood. (2018) 132:1544. doi: 10.1182/blood-2018-99-117954
80. Faham M, Zheng J, Moorhead M, Carlton VE, Stow P, Coustan-Smith E, et al. Deep-sequencing approach for minimal residual disease detection in acute lymphoblastic leukemia. Blood. (2012) 120:5173–80. doi: 10.1182/blood-2012-07-444042
81. Ladetto M, Brïggemann M, Monitillo L, Ferrero S, Pepin F, Drandi D, et al. Next-generation sequencing and real-time quantitative PCR for minimal residual disease detection in B-cell disorders. Leukemia. (2014) 28:1299–307. doi: 10.1038/leu.2013.375
82. Kotrova M, Muzikova K, Mejstrikova E, Novakova M, Bakardjieva-Mihaylova V, Fiser K, et al. The predictive strength of next-generation sequencing MRD detection for relapse compared with current methods in childhood ALL. Blood. (2015) 126:1045–7. doi: 10.1182/blood-2015-07-655159
83. Logan AC, Zhang B, Narasimhan B, Carlton V, Zheng J, Moorhead M, et al. Minimal residual disease quantification using consensus primers and high-throughput IGH sequencing predicts post-transplant relapse in chronic lymphocytic leukemia. Leukemia. (2013) 27:1659–65. doi: 10.1038/leu.2013.52
84. Eckert C, Flohr T, Koehler R, Hagedorn N, Moericke A, Stanulla M, et al. Very early/early relapses of acute lymphoblastic leukemia show unexpected changes of clonal markers and high heterogeneity in response to initial and relapse treatment. Leukemia. (2011) 25:1305–13. doi: 10.1038/leu.2011.89
85. Kotrova M, van der Velden VH, van Dongen JJ, Formankova R, Sedlacek P, Brüggemann M, et al. Next-generation sequencing indicates false-positive MRD results and better predicts prognosis after SCT in patients with childhood ALL. Bone Marrow Transplant. (2017) 52:962–8. doi: 10.1038/bmt.2017.16
86. Della Starza I, De Novi LA, Santoro A, Salemi D, Cavalli M, Soscia R, et al. Comparative analysis between RQ-PCR, digital-droplet-PCR and Next-Generation-Sequencing (NGS) of immunoglobulin/T-cell receptor gene rearrangements to monitor minimal residual disease in adult acute lymphoblastic leukemia patients. Blood. (2018) 132:2828. doi: 10.1182/blood-2018-99-118219
87. Brüggemann M, Gökbuget N, Kneba M. Acute lymphoblastic leukemia: monitoring minimal residual disease as a therapeutic principle. Semin Oncol. (2012) 39:47–57. doi: 10.1053/j.seminoncol.2011.11.009
88. Gökbuget N, Dombret H, Giebel S, Bruggemann M, Doubek M, Foà R, et al. Minimal residual disease level predicts outcome in adults with Ph-negative B-precursor acute lymphoblastic leukemia. Hematology. (2019) 24:337–48. doi: 10.1080/16078454.2019.1567654
89. Salek C, Folber F, Fronkova E, Prochazka B, Marinov I, Cetkovsky P, et al. Czech Leukemia Study Group - for Life. Early MRD response as a prognostic factor in adult patients with acute lymphoblastic leukemia. Eur J Haematol. (2016) 96:276–84. doi: 10.1111/ejh.12587
90. Short NJ, Kantarjian HM, Sasaki K, Cortes JE, Ravandi F, Thomas DA, et al. Prognostic significance of day 14 bone marrow evaluation in adults with Philadelphia chromosome-negative acute lymphoblastic leukemia. Cancer. (2016) 122:3812–20. doi: 10.1002/cncr.30262
91. Gökbuget N, Kneba M, Raff T, Trautmann H, Bartram CR, Arnold R, et al. German Multicenter Study Group for Adult Acute Lymphoblastic Leukemia. Adult patients with acute lymphoblastic leukemia and molecular failure display a poor prognosis and are candidates for stem cell transplantation and targeted therapies. Blood. (2012) 120:1868–76. doi: 10.1182/blood-2011-09-377713
92. Ribera JM, Oriol A, Morgades M, Montesinos P, Sarrà J, González-Campos J, et al. Treatment of high-risk Philadelphia chromosome-negative acute lymphoblastic leukemia in adolescents and adults according to early cytologic response and minimal residual disease after consolidation assessed by flow cytometry: final results of the PETHEMA ALL-AR-03 trial. J Clin Oncol. (2014) 32:1595–604. doi: 10.1200/JCO.2013.52.2425
93. Dhédin N, Huynh A, Maury S, Tabrizi R, Beldjord K, Asnafi V, et al. GRAALL group. Role of allogeneic stem cell transplantation in adult patients with Ph-negative acute lymphoblastic leukemia. Blood. (2015) 125:2486–96. doi: 10.1182/blood-2014-09-599894
94. Bassan R, Masciulli A, Intermesoli T, Spinelli O, Tosi M, Pavoni C, et al. Final results of Northern Italy Leukemia Group (NILG) trial 10/07 combining pediatric-type therapy with minimal residual disease study and risk-oriented hematopoietic cell transplantation in adult acute lymphoblastic leukemia (ALL). Blood. (2016) 128:176.
95. Bassan R, Chiaretti S, Paoloni F, Audisio E, Marbello L, Borlenghi E, et al. First results of new GIMEMA trial LAL 1913 for adult patients with Philadelphia-negative acute lymphoblastic leukemia (Ph-ALL). In: 23th EHA Annual Congress Stockolm, 14-17 June. Stockolm. (2018) p. PS919.
96. Patel B, Rai L, Buck G, Richards SM, Mortuza Y, Mitchell W, et al. Minimal residual disease is a significant predictor of treatment failure in non T-lineage adult acute lymphoblastic leukaemia: final results of the international trial UKALL XII/ECOG2993. Br J Haematol. (2010) 148:80–9. doi: 10.1111/j.1365-2141.2009.07941.x
97. Giebel S, Stella-Holowiecka B, Krawczyk-Kulis M, Gökbuget N, Hoelzer D, Doubek M, et al. Status of minimal residual disease determines outcome of autologous hematopoietic SCT in adult ALL. Bone Marrow Transplant. (2010) 45:1095–101. doi: 10.1038/bmt.2009.308
98. Bassan R, Spinelli O, Oldani E, Intermesoli T, Tosi M, Peruta B, et al. Different molecular levels of post-induction minimal residual disease may predict hematopoietic stem cell transplantation outcome in adult Philadelphia-negative acute lymphoblastic leukemia. Blood Cancer J. (2014) 4:e225. doi: 10.1038/bcj.2014.48
99. Salah-Eldin M, Abousamra NK, Azzam H. Clinical significance of minimal residual disease in young adults with standard-risk/Ph-negative precursor B-acute lymphoblastic leukemia: results of prospective study. Med Oncol. (2014) 31:938. doi: 10.1007/s12032-014-0938-z
100. Berry DA, Zhou S, Higley H, Mukundan L, Fu S, Reaman GH, et al. Association of minimal residual disease with clinical outcome in pediatric and adult acute lymphoblastic leukemia: a meta-analysis. JAMA Oncol. (2017) 3:e170580. doi: 10.1001/jamaoncol.2017.0580
101. Hoelzer D. Personalized medicine in adult acute lymphoblastic leukemia. Haematologica. (2015) 100:855–8. doi: 10.3324/haematol.2015.127837
102. Chalandon Y, Thomas X, Hayette S, Cayuela JM, Abbal C, Huguet F, et al. Randomized study of reduced-intensity chemotherapy combined with imatinib in adults with Ph-positive acute lymphoblastic leukemia. Blood. (2015) 125:3711–9. doi: 10.1182/blood-2015-02-627935
103. Foà R, Vitale A, Vignetti M, Meloni G, Guarini A, De Propris MS, et al. Dasatinib as first-line treatment for adult patients with Philadelphia chromosome-positive acute lymphoblastic leukemia. Blood. (2011) 118:6521–8. doi: 10.1182/blood-2011-05-351403
104. Yoon JH, Yhim HY, Kwak JY, Ahn JS, Yang DH, Lee JJ, et al. Minimal residual disease-based effect and long-term outcome of first-line dasatinib combined with chemotherapy for adult Philadelphia chromosome-positive acute lymphoblastic leukemia. Ann Oncol. (2016) 27:1081–8. doi: 10.1093/annonc/mdw123
105. Maino E, Sancetta R, Viero P, Imbergamo S, Scattolin AM, Vespignani M, et al. Current and future management of Ph/BCRABL positive ALL. Expert Rev Anticancer Ther. (2014) 14:723–40. doi: 10.1586/14737140.2014.895669
106. Pfeifer H, Wassmann B, Bethge W, Dengler J, Bornhauser M, Stadler M, et al. Randomized comparison of prophylactic and minimal residual disease-triggered imatinib after allogeneic stem cell transplantation for BCR-ABL1-positive acute lymphoblastic leukemia. Leukemia. (2013) 27:1254–62. doi: 10.1038/leu.2012.352
107. Chiaretti S, Vitale A, Vignetti M, Piciocchi A, Fazi P, Elia L, et al. A sequential approach with imatinib, chemotherapy and transplant for adult Ph+ acute lymphoblastic leukemia: final results of the GIMEMA LAL 0904 study. Haematologica. (2016) 101:1544–52. doi: 10.3324/haematol.2016.144535
108. Lee S, Kim DW, Cho BS, Yoon JH, Shin SH, Yahng SA, et al. Impact of minimal residual disease kinetics during imatinib-based treatment on transplantation outcome in Philadelphia chromosome-positive acute lymphoblastic leukemia. Leukemia. (2012) 26:2367–74. doi: 10.1038/leu.2012.164
109. Mizuta S, Matsuo K, Maeda T, Yujiri T, Hatta Y, Kimura Y, et al. Prognostic factors influencing clinical outcome of allogeneic hematopoietic stem cell transplantation following imatinib-based therapy in BCR-ABL positive ALL. Blood Cancer J. (2012) 2:e72. doi: 10.1038/bcj.2012.18
110. Ravandi F, Jorgensen JL, Thomas DA, O'Brien S, Garris R, Faderl S, et al. Detection of MRD may predict the outcome of patients with Philadelphia chromosome-positive ALL treated with tyrosine kinase inhibitors plus chemotherapy. Blood. (2013) 122:1214–21. doi: 10.1182/blood-2012-11-466482
111. Chiaretti S, Vitale A, Elia L, Albino S, Piciocchi A, Fazi P, et al. Multicenter total therapy Gimema LAL 1509 protocol for de novo adult Ph+ acute lymphoblastic leukemia (ALL) patients. Updated results and refined genetic based prognostic stratification. Blood. (2015) 126:81.
112. Kim DY, Joo YD, Lim SN, Kim SD, Lee JH, Lee JH, et al. Nilotinib combined with multiagent chemotherapy for newly diagnosed Philadelphia-positive acute lymphoblastic leukemia. Blood. (2015) 126:746–56. doi: 10.1182/blood-2015-03-636548
113. Lussana F, Intermesoli T, Gianni F, Boschini C, Masciulli A, Spinelli O, et al. Achieving molecular remission before allogeneic stem cell transplantation in adult patients with Philadelphia chromosome-positive acute lymphoblastic leukemia: impact on relapse and long-term outcome. Biol Blood Marrow Transplant. (2016) 22:1983–7. doi: 10.1016/j.bbmt.2016.07.021
114. Ravandi F, Jorgensen JL, O'Brien SM, Jabbour E, Thomas DA, Borthakur G, et al. Minimal residual disease assessed by multiparameter flow cytometry is highly prognostic in adult patients with acute lymphoblastic leukaemia. Br J Haematol. (2016) 172:392–400. doi: 10.1111/bjh.13834
115. Nishiwaki S, Imai K, Mizuta S, Kanamori H, Ohashi K, Fukuda T, et al. Impact of MRD and TKI on allogeneic hematopoietic cell transplantation for Ph+ ALL: a study from the adult ALL WG of the JSHCT. Bone Marrow Transplant. (2016) 51:43–50. doi: 10.1038/bmt.2015.217
116. Martinelli G, Boissel N, Chevallier P, Ottmann O, Gökbuget N, Topp MS, et al. Complete hematologic and molecular response in adult patients with relapsed/refractory philadelphia chromosome-positive B-precursor acute lymphoblastic leukemia following treatment with blinatumomab: results from a phase, I. I, Single-arm, multicenter study. J Clin Oncol. (2017) 35:1795–802. doi: 10.1200/JCO.2016.69.3531
117. Cazzaniga G, De Lorenzo P, Alten J, Röttgers S, Hancock J, Saha V, et al. Predictive value of minimal residual disease in Philadelphia-chromosome-positive acute lymphoblastic leukemia treated with imatinib in the European intergroup study of post-induction treatment of Philadelphia-chromosome-positive acute lymphoblastic leukemia, based on immunoglobulin/T-cell receptor and BCR/ABL1 methodologies. Haematologica. (2018) 103:107–15. doi: 10.3324/haematol.2017.176917
118. Clappier E, Kim R, Cayuela JM, Rousselot P, Chalandon Y, Passet M, et al. Persistent BCR-ABL1 clonal hematopoiesis after blast clearance identifies a CML-like subgroup of patients with Philadelphia chromosome-positive (Ph+) ALL: Interim results from GRAAPH-2014 trial. In: 23th EHA Annual Congress Stockolm, 14-17 June. Stockolm. (2018) p. S1568.
119. Huguet F, Leguay T, Raffoux E, Thomas X, Beldjord K, Delabesse E, et al. Pediatric-inspired therapy in adults with Philadelphia chromosome-negative acute lymphoblastic leukemia: the GRAALL-2003 study. J Clin Oncol. (2009) 27:911–8. doi: 10.1200/JCO.2008.18.6916
120. Stock W, Luger SM, Advani AS, Geyer S, Harvey RC, Mullighan CG, et al. Favorable outcomes for older adolescents and young adults (AYA) with acute lymphoblastic leukemia (ALL): early results of U.S. Intergroup Trial C10403. Blood. (2014) 124:796.
121. Buckley SA, Appelbaum FR, Walter RB. Prognostic and therapeutic implications of minimal residual disease at the time of transplantation in acute leukemia. Bone Marrow Transplant. (2013) 48:630–41. doi: 10.1038/bmt.2012.139
122. Campana D, Leung W. Clinical significance of minimal residual disease in patients with acute leukaemia undergoing haematopoietic stem cell transplantation. Br J Haematol. (2013) 162:147–61. doi: 10.1111/bjh.12358
123. Zhou Y, Slack R, Jorgensen JL, Wang SA, Rondon G, de Lima M, et al. The effect of peritransplant minimal residual disease in adults with acute lymphoblastic leukemia undergoing allogeneic hematopoietic stem cell transplantation. Clin Lymphoma Myeloma Leuk. (2014) 14:319–26. doi: 10.1016/j.clml.2014.01.002
124. Shen Z, Gu X, Mao W, Yin L, Yang L, Zhang Z, et al. Influence of pre-transplant minimal residual disease on prognosis after Allo-SCT for patients with acute lymphoblastic leukemia: systematic review and meta-analysis. BMC Cancer. (2018) 18:755. doi: 10.1186/s12885-018-4670-5
125. Terwey TH, Hemmati PG, Nagy M, Pfeifer H, Gökbuget N, Brüggemann M, et al. Comparison of chimerism and minimal residual disease monitoring for relapse prediction after allogeneic stem cell transplantation for adult acute lymphoblastic leukemia. Biol Blood Marrow Transplant. (2014) 20:1522–9. doi: 10.1016/j.bbmt.2014.05.026
126. Bar M, Wood BL, Radich JP, Doney KC, Woolfrey AE, Delaney C, et al. Impact of minimal residual disease, detected by flow cytometry, on outcome of myeloablative hematopoietic cell transplantation for acute lymphoblastic leukemia. Leuk Res Treatment. (2014) 2014:421723. doi: 10.1155/2014/421723
127. Bader P, Kreyenberg H, Henze GH, Eckert C, Reising M, Willasch A, et al. Prognostic value of minimal residual disease quantification before allogeneic stem-cell transplantation in relapsed childhood acute lymphoblastic leukemia: the ALL-REZ BFM study group. J Clin Oncol. (2009) 27:377–84. doi: 10.1200/JCO.2008.17.6065
128. Bader P, Kreyenberg H, von Stackelberg A, Eckert C, Salzmann-Manrique E, Meisel R, et al. Monitoring of minimal residual disease after allogeneic stem-cell transplantation in relapsed childhood acute lymphoblastic leukemia allows for the identification of impending relapse: results of the ALL-BFM-SCT 2003 trial. J Clin Oncol. (2015) 33:1275–84. doi: 10.1200/JCO.2014.58.4631
129. Szczepanski T, van der Velden VH, Waanders E, Kuiper RP, Van Vlierberghe P, Gruhn B, et al. Late recurrence of childhood T-cell acute lymphoblastic leukemia frequently represents a second leukemia rather than a relapse: first evidence for genetic predisposition. J Clin Oncol. (2011) 29:1643–9. doi: 10.1200/JCO.2010.30.2877
130. Beldjord K, Chevret S, Asnafi V, Huguet F, Boulland ML, Leguay T, et al. Group for Research on Adult Acute Lymphoblastic Leukemia (GRAALL). Oncogenetics and minimal residual disease are independent outcome predictors in adult patients with acute lymphoblastic leukemia. Blood. (2014) 123:3739–49. doi: 10.1182/blood-2014-01-547695
131. Sutton R, Venn NC, Law T, Boer JM, Trahair TN, Ng A, et al. A risk score including microdeletions improves relapse prediction for standard and medium risk precursor B-cell acute lymphoblastic leukaemia in children. Br J Haematol. (2018) 180:550–62. doi: 10.1111/bjh.15056
132. Loffler A, Gruen M, Wuchter C, Schriever F, Kufer P, Dreier T, et al. Efficient elimination of chronic lymphocytic leukaemia B cells by autologous T cells with a bispecific anti-CD19/anti-CD3 single-chain antibody construct. Leukemia. (2003) 17:900–9. doi: 10.1038/sj.leu.2402890
133. Leone P, Shin EC, Perosa F, Vacca A, Dammacco F, Racanelli V. MHC class I antigen processing and presenting machinery: organization, function, and defects in tumor cells. J Natl Cancer Inst. (2013) 105:1172–87. doi: 10.1093/jnci/djt184
134. Brandl C, Haas C, d'Argouges S, Fisch T, Kufer P, Brischwein K, et al. The effect of dexamethasone on polyclonal T cell activation and redirected target cell lysis as induced by a CD19/CD3-bispecific single-chain antibody construct. Cancer Immunol Immunother. (2007) 56:1551–63. doi: 10.1007/s00262-007-0298-z
135. Topp MS, Gökbuget N, Zugmaier G, Klappers P, Stelljes M, Neumann S, et al. Phase II trial of the anti-CD19 bispecific T cell-engager blinatumomab shows hematologic and molecular remissions in patients with relapsed or refractory B-precursor acute lymphoblastic leukemia. J Clin Oncol. (2014) 32:4134–40. doi: 10.1200/JCO.2014.56.3247
136. Topp MS, Gökbuget N, Stein AS, Zugmaier G, O'Brien S, Bargou RC, et al. Safety and activity of blinatumomab for adult patients with relapsed or refractory B-precursor acute lymphoblastic leukaemia: a multicentre, single-arm, phase 2 study. Lancet Oncol. (2015) 16:57–66. doi: 10.1016/S1470-2045(14)71170-2
137. Kantarjian H, Stein A, Gökbuget N, Fielding AK, Schuh AC, Ribera JM, et al. Blinatumomab versus chemotherapy for advanced acute lymphoblastic leukemia. N Engl J Med. (2017) 376:836–47. doi: 10.1056/NEJMoa1609783
138. Zugmaier G, Gökbuget N, Klinger M, Viardot A, Stelljes M, Neumann S, et al. Long-term survival and T-cell kinetics in relapsed/refractory ALL patients who achieved MRD response after blinatumomab treatment. Blood. (2015) 126:2578–84. doi: 10.1182/blood-2015-06-649111
139. Gökbuget N, Zugmaier G, Klinger M, Kufer P, Stelljes M, Viardot A, et al. Long-term relapse-free survival in a phase 2 study of blinatumomab for the treatment of patients with minimal residual disease in B-lineage acute lymphoblastic leukemia. Haematologica. (2017) 102:e132–5. doi: 10.3324/haematol.2016.153957
140. Gökbuget N, Dombret H, Bonifacio M, Reichle A, Graux C, Faul C, et al. Blinatumomab for minimal residual disease in adults with B-cell precursor acute lymphoblastic leukemia. Blood. (2018) 131:1522–31. doi: 10.1182/blood-2017-08-798322
141. Kantarjian H, Thomas D, Jorgensen J, Kebriaei P, Jabbour E, Rytting M, et al. Results of inotuzumab ozogamicin, a CD22 monoclonal antibody, in refractory and relapsed acute lymphocytic leukemia. Cancer. (2013) 119:2728–36. doi: 10.1002/cncr.28136
142. Kantarjian HM, De Angelo DJ, Stelljes M, Martinelli G, Liedtke M, Stock W, et al. Inotuzumab ozogamicin versus standard therapy for acute lymphoblastic leukemia. N Engl J Med. (2016) 375:740–53. doi: 10.1056/NEJMoa1509277
143. Davila ML, Riviere I, Wang X, Bartido S, Park J, Curran K, et al. Efficacy and toxicity management of 19-28z CAR T cell therapy in B cell acute lymphoblastic leukemia. Sci Transl Med. (2014) 6:224ra25. doi: 10.1126/scitranslmed.3008226
144. Lee DW, Kochenderfer JN, Stetler-Stevenson M, Cui YK, Delbrook C, Feldman SA, et al. T cells expressing CD19 chimeric antigen receptors for acute lymphoblastic leukaemia in children and young adults: a phase 1 dose-escalation trial. Lancet. (2015) 385:517–28. doi: 10.1016/S0140-6736(14)61403-3
145. Gardner RA, Finney O, Annesley C, Brakke H, Summers C, Leger K, et al. Intent-to-treat leukemia remission by CD19 CAR T cells of defined formulation and dose in children and young adults. Blood. (2017) 129:3322–31. doi: 10.1182/blood-2017-02-769208
146. Brentjens RJ, Davila ML, Riviere I, Park J, Wang X, Cowell LG, et al. CD19-targeted T cells rapidly induce molecular remissions in adults with chemotherapy-refractory acute lymphoblastic leukemia. Sci Transl Med. (2013) 5:177ra138. doi: 10.1126/scitranslmed.3005930
Keywords: acute lymphoblastic leukemia (ALL), minimal residual disease (MRD), flow cytometry, real time quantitative PCR (RQ-PCR), next generation flow cytometry (NGF), digital droplet PCR (ddPCR), next generation sequencing (NGS), novel agents
Citation: Della Starza I, Chiaretti S, De Propris MS, Elia L, Cavalli M, De Novi LA, Soscia R, Messina M, Vitale A, Guarini A and Foà R (2019) Minimal Residual Disease in Acute Lymphoblastic Leukemia: Technical and Clinical Advances. Front. Oncol. 9:726. doi: 10.3389/fonc.2019.00726
Received: 12 April 2019; Accepted: 22 July 2019;
Published: 07 August 2019.
Edited by:
Giuseppe Alberto Palumbo, University of Catania, ItalyReviewed by:
Paul Armistead, University of North Carolina at Chapel Hill, United StatesVikram Mathews, Christian Medical College & Hospital, India
Copyright © 2019 Della Starza, Chiaretti, De Propris, Elia, Cavalli, De Novi, Soscia, Messina, Vitale, Guarini and Foà. This is an open-access article distributed under the terms of the Creative Commons Attribution License (CC BY). The use, distribution or reproduction in other forums is permitted, provided the original author(s) and the copyright owner(s) are credited and that the original publication in this journal is cited, in accordance with accepted academic practice. No use, distribution or reproduction is permitted which does not comply with these terms.
*Correspondence: Sabina Chiaretti, Y2hpYXJldHRpQGJjZS51bmlyb21hMS5pdA==; Robin Foà, cmZvYUBiY2UudW5pcm9tYTEuaXQ=
†These authors have contributed equally to this work