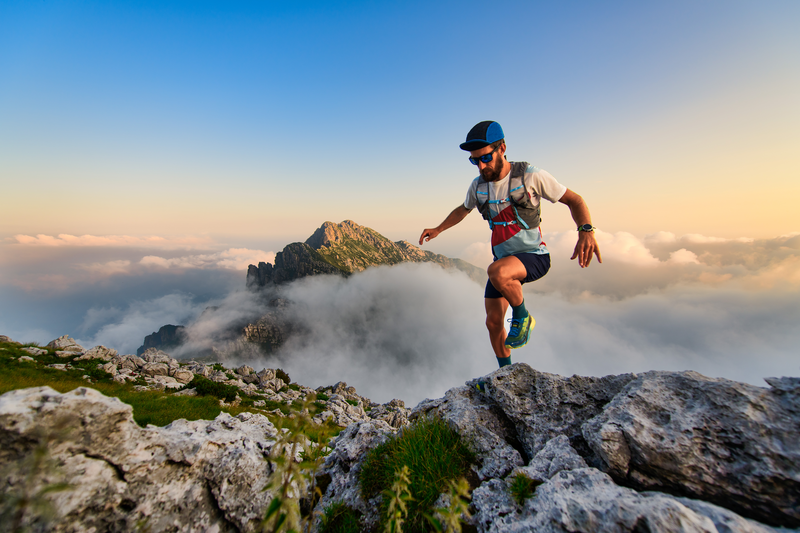
95% of researchers rate our articles as excellent or good
Learn more about the work of our research integrity team to safeguard the quality of each article we publish.
Find out more
ORIGINAL RESEARCH article
Front. Oncol. , 21 June 2019
Sec. Radiation Oncology
Volume 9 - 2019 | https://doi.org/10.3389/fonc.2019.00531
Background and purpose: Pediatric ependymoma carries a dismal prognosis, mainly owing to local relapse within RT fields. The current prospective European approach is to increase the radiation dose with a sequential hypofractionated stereotactic boost. In this study, we assessed the possibility of using a simultaneous integrated boost (SIB), comparing VMAT vs. IMPT dose delivery.
Material and methods: The cohort included 101 patients. The dose to planning target volume (PTV59.4) was 59.4/1.8 Gy, and the dose to SIB volume (PTV67.6) was 67.6/2.05 Gy. Gross tumor volume (GTV) was defined as the tumor bed plus residual tumor, clinical target volume (CTV59.4) was GTV + 5 mm, and PTV59.4 was CTV59.4 + 3 mm. PTV67.6 was GTV+ 3 mm. After treatment plan optimization, quality indices and doses to target volume and organs at risk (OARs) were extracted and compared with the standard radiation doses that were actually delivered (median = 59.4 Gy [50.4 59.4]).
Results: In most cases, the proton treatment resulted in higher quality indices (p < 0.001). Compared with the doses that were initially delivered, mean, and maximum doses to some OARs were no higher with SIB VMAT, and significantly lower with protons (p < 0.001). In the case of posterior fossa tumor, there was a lower dose to the brainstem with protons, in terms of V59 Gy, mean, and near-maximum (D2%) doses.
Conclusion: Dose escalation with intensity-modulated proton or photon SIB is feasible in some patients. This approach could be considered for children with unresectable residue or post-operative FLAIR abnormalities, particularly if they have supratentorial tumors. It should not be considered for infratentorial tumors encasing the brainstem or extending to the medulla.
Pediatric ependymoma is a frequent brain tumor in children, adolescents, and young adults. Treatment relies on maximum surgical resection, followed by localized radiotherapy at 59.4/1.8 Gy (1–3).
Intensity-modulated photon radiotherapy (IMRT) is currently used to reduce the dose to organs at risk (OARs). However, on account of its physical properties, proton-beam therapy (PBT) produces excellent dose distribution and often decreases the dose to OARs, potentially lowering the risk of radiation-induced secondary cancers for those pediatric patients who live well-beyond the end of their treatment (4–6).
Several studies have reported the advantages of PBT over different forms of photon radiotherapy, including IMRT (7–10). MacDonald et al. found that proton beams and IMRT had similar target coverage, but normal tissue sparing was better with IMPT for patients with ependymoma (11, 12). Pulsifer et al. (13) reported that early cognitive outcomes following PBT for pediatric central nervous system (CNS) tumors were encouraging, compared with published outcomes for photon radiotherapy.
However, despite the aggressiveness of the treatment and improvements in radiation techniques for this tumor, outcomes remain poor, with 5 year event-free survival and overall survival rates of 50 and 75% (14). Chemotherapy does not seem to be effective for this tumor. It is only used in very young patients to avoid irradiation and in children with residual disease after initial resection (15), although it is currently being assessed in the SIOP trial as a maintenance treatment. Based on a large national cohort study (16, 17), our team and others found that the majority of relapses occur within the high-dose regions (10, 16). Moreover, a survival benefit of stereotactic radiotherapy (SRT) to residue was described in a prospective clinical trial (18). This prompted us to examine a new dose escalation approach, with a view to sparing the surrounding OARs-particularly the brainstem. We therefore, undertook an in-silico dosimetric comparison between volumetric modulated arc therapy (VMAT) photon therapy and intensity-modulated proton therapy (IMPT), in order to identify the types of patients who would benefit from this escalation.
The cohort included 101 patients drawn from the PEPPI national multicenter database. These patients were treated in France's 13 national pediatric radiotherapy reference centers between 2000 and 2013. We were able to retrospectively replan 91 of them with VMAT or IMPT. Those patients who were not replanned (10 patients) were not eligible for dose escalation, as their brainstem would have been exposed to radiation on account of their tumor size or location (see Supplementary Figure 1 for three examples). This study was approved by the national French ethics committee and the French data protection authority (CNIL: Commission nationale de l'informatique et des libertés de France).
The gross tumor volume (GTV) was kept as originally delineated, defined as the tumor bed (border of the surgical cavity, tissues, and anatomical areas initially involved with disease) plus residual disease when present. Clinical target volume for 59.4 Gy (relative biological effectiveness, RBE) (CTV59.4) was GTV + 5 mm, and planning target volume (PTV59.4) was CTV59.4 + 3 mm. The PTV of 67.6 Gy (cobalt gray equivalent, CGE) (PTV67.6) was GTV+ 3 mm.
The dose to PTV59.4 was 59.4/1.8 Gy, and the dose to simultaneous integrated boost (SIB) volume (PTV67.6) was 67.6/2.05 Gy (equivalent to sequential boost in clinical trial NCT 02265770). We used a constant RBE value of 1.10 for prescribed doses. It should be noted that most of the other absorbed doses described here were relative doses, normalized to the prescribed dose.
As the data we collected came from patients attending 13 different pediatric radiation oncology reference centers, OAR delineation varied. To be able to compare dose distributions, an experienced radiation oncologist (AL) retrospectively added and corrected OARs so that all patients had the same set. The following 11 OARs were defined: brain, hippocampus, brainstem, inner ears, optic nerves, chiasm, eyes and lenses, pituitary gland, spinal cord, cerebellum, and temporal lobes.
Photon and proton dose plans were generated for all patients in a research version of the RayStation v5 treatment planning system (RaySearch Laboratories, Stockholm, Sweden). The CT calibration curves specific to each center were retrieved before dose calculation.
The photon plans were generated as dual-arc 6 MV VMAT plans, using beam data from a linear accelerator (Varian, Palo Alto, CA, USA). The photon dose was calculated with the clinically used collapsed cone algorithm. The proton plans were pencil-beam scanning (PBS) plans with two to three beams, using beam data obtained from a PBS-dedicated nozzle (Ion Beam Applications, Louvain-la-Neuve, Belgium). A range shifter (water equivalent thickness: 74.1 mm) was used for all the beams in all the treatment plans, as the skin-to-target distance was always <7 cm. All proton plans were optimized with IMPT (multifield optimization). The physical proton dose was optimized. For all photon and proton plans, the prescribed dose was set at the D50% of the PTV67.6. The aim for the treatment plans was to achieve a dose distribution comparable to photons or better. All treatment plans were optimized with the physical dose-volume objectives/constraints (for targets as well as OARs). The PTV coverage goals and clinical goals for the OARs are provided in Supplementary Tables 1, 2. All proton and photon plans were created by the same physicist and validated by the same radiation oncologist.
We analyzed three dose-volume descriptors for PTVs: D98, D50, and D2%.
Mean doses and relevant volume-dose values were derived for each OAR.
In addition, several International Commission on Radiation Units and Measurements (ICRU) indices (19), including homogeneity (HI), coverage (CO), conformity (CI), target coverage (TCO), and dice similarity coefficient (DSC), were derived from the dose-volume histograms.
Treated volume (V95% for the body), and integral dose (mean dose for the body) were calculated for each treatment plan (20, 21). All these data were extracted using a script developed with the RaySearch Laboratories team.
Additionally, we compared the mean and near-maximum doses to eight OARs (brainstem, pituitary gland, hippocampus, temporal lobes, chiasm, inner ears, eyes, optic nerves) calculated in silico for SIB dose escalation using VMAT and IMPT with those that were actually delivered to the patients in this cohort, including all the prescribed doses (Table 1) and all the radiation techniques (3D, IMRT, VMAT, IMRT, tomotherapy, protons, and mixed photon-proton).
Data were summarized by frequency and percentage for categorical variables, and by median and range for continuous variables. Comparisons between the two treatment plan modalities were performed using the Wilcoxon signed-rank test for paired data. The Benjamini-Hochberg correction was applied for multiple comparisons. For each comparison, we computed the difference between IMPT and VMAT for each patient (Δ). All reported p-values were two-sided. For all statistical tests, differences were considered significant at the 5% level. Statistical analyses were performed using STATA 13.1 and R 3.5.1 software.
We excluded 10 patients from the present dose escalation study because the location of their tumor meant that a substantial volume of brainstem was concerned. Four of them had a large tumor in close contact with much of the perimeter of the brainstem, and six had tumors that extended to the cerebellopontine angle with considerable cervical medullary involvement (below C2).
The results are summarized in Supplementary Tables 3–8, Figure 1, and Supplementary Figures 2, 3.
Figure 1. Doses to OARs and near-maximum D2% (top) and mean doses (bottom) as a % of the prescribed target dose. Infratentorial tumor (left panel) and supratentorial tumor (right panel).
In the case of infratentorial tumor (Supplementary Table 3), IMPT yielded better results for HI (median: 0.06 vs. 0.07, padj < 0.0001, median Δ = −0.015), CI (median: 1.37 vs. 1.51, padj ≤ 0.0001, median Δ = −0.17), and DSC (median: 0.84 vs. 0.78, padj ≤ 0.0001, median Δ = 0.05) than VMAT did. In the case of supratentorial tumor, IMPT also yielded better results for HI (median: 0.05 vs. 0.06, padj = 0.40014, median Δ = −0.006), CI (median: 1.22 vs. 1.32, padj < 0.0001, median Δ = −0.12), and DSC (median: 0.90 vs. 0.85, padj < 0.0001, median Δ = 0.052) than VMAT did (Supplementary Table 6).
In the case of infratentorial tumor (Supplementary Table 4), IMPT yielded better results for HI (median: 0.18 vs. 0.20, padj < 0.0001, median Δ = −0.10), CI (median: 1.13 vs. 1.17, padj < 0.0001, median Δ = −0.040), and DSC (median: 0.93 vs. 0.91, padj < 0.0001, median Δ = 0.020) than VMAT did. In the case of supratentorial tumor (Supplementary Table 7), IMPT yielded better results for CO (median: 0.95 vs. 0.93, padj = 0.0088, median Δ = 0.016).
Results are provided for the whole cohort (Supplementary Tables 5, 8). Mean doses are reported for organs with a mainly parallel architecture, while near-maximum (D2%) doses are provided for serial-type organs. Dosimetric results are compared for each OAR. IMPT doses were generally lower than VMAT doses for six OARs (brain, hippocampus, inner ears, pituitary gland, cerebellum, and body), padj < 0.05, for both supra- and infratentorial tumors.
Near-maximum (D2%) doses to the optic nerves, chiasm, and brainstem were significantly lower for IMPT (padj < 0.0001), for both supra- and infratentorial tumors. The whole-body dose was lower for protons (padj < 0.0001).
Dmean and D2% doses were significantly lower for IMPT than for VMAT (padj < 0.0001), for both supra- and infratentorial tumors. D50% did not differ between IMPT and VMAT in the case of infratentorial tumors, but V59Gy for IMPT was significantly lower in the case of infratentorial tumors (padj < 0.0001, median Δ = −2.465).
The worst cases were infratentorial tumors arising from or centered on the lateral recess of the fourth ventricle (i.e., foramen of Luschka). It was more difficult to optimize treatment planning for this tumor location, which we observed in 48.33% of our infratentorial cohort, than for centrally located tumors.
The additional comparison revealed that, in all patients with infratentorial tumors (Figure 2), mean and near-maximum doses to some OARs in SIB-IMPT were significantly lower than the doses received during the initial treatment. For the brainstem, the mean dose was significantly lower, but the near-maximum dose was significantly higher. In the case of SIB-VMAT, this difference was not significant for any of the OARs except the brainstem where, despite the mean dose being significantly lower, the near-maximum dose was significantly higher. For supratentorial tumors (Figure 3), the mean and near-maximum doses to some OARs were significantly lower with SIB-IMPT, but the difference was not significant with SIB-VMAT.
Figure 2. Median of mean and near-maximum doses (cGy) to OARs received at treatment (tt) with SIB-VMAT or SIB-PBS in the case of infratentorial tumor. IER, right inner ear; IEL, left inner ear; BS MD, brainstem mean dose; BS NMD, brainstem near-maximum dose; PG, pituitary gland; HR, right hippocampus; HL, left hippocampus; ST, standard treatment.
Figure 3. Median of mean and near-maximum doses (cGy) to OARs received at treatment (tt) with SIB-VMAT or SIB-PBS in the case of supratentorial tumor. BS MD, brainstem mean dose; BS NMD, brainstem near-maximum dose; PG, pituitary gland; HR, right hippocampus; HL, left hippocampus; ST, standard treatment.
In the present study, we assessed the feasibility of escalating the radiation dose and quantified the differences between IMPT and VMAT in a large number of patients with pediatric supra- or infratentorial ependymoma. We did this to identify the types of patients who would gain the most from being treated with protons in the case of an escalating dose.
Results showed that in the majority of cases, the target coverage could be retained with a significant reduction in the dose to healthy surrounding tissues. Doses to the brain, hippocampus, inner ears, brainstem, pituitary gland, temporal lobes, cerebellum, chiasm, and optic nerves were significantly lower with IMPT than with VMAT, for both supra- and infratentorial tumors. Doses to the spinal cord of some patients with infratentorial tumor were not significantly lower with IMPT than with VMAT. Indeed, most of the difficult cases for target coverage were infratentorial tumors arising from or centered on the lateral recess of the fourth ventricle (i.e., foramen of Luschka)-a tumor location that is often associated with a poorer prognosis than centrally located tumors (20–23).
We compared VMAT, which is still the most common technique for pediatric radiation therapy, with IMPT. There is no doubt that protons can spare healthy tissue more than VMAT can, and for most of the patients in our cohort, we found considerable sparing of OARs and normal tissue. For the individual patient, this may lead to fewer acute side effects resulting from the radiation treatment, as described recently by Rombi et al. in their overview of clinical results (24).
Furthermore, despite escalating the dose to 67.6 Gy, mean and near-maximum doses to OARs were significantly lower with IMPT. With VMAT, they did not differ significantly from those initially delivered at a median dose of 59.4 Gy for most OARs. The exception was the brainstem, where the near-maximum dose was higher-possibly because of this organ's vicinity to the high-dose PTV. Radiation techniques and the doses that were actually delivered in the plans differed across patients. We nevertheless preferred to retain them all, in order to benefit from the statistical power afforded by having a larger sample, and also to demonstrate that substantially higher doses can be delivered to the tumor bed with SIB-VMAT without increasing doses to OARs, while doses to OARs may even decrease with SIB-IMPT. However, these results should be treated with caution, as the dose constraints applied to the delivery of standard-dose treatments varied slightly between treatment centers.
Based on previous results from our team (25), we assumed that high-dose SIB would not increase the dose to OARs. We ran a comparison on a more limited number of cases (i.e., those with contoured OARs available for actually delivered treatments), which yielded the expected results. We postulated that escalating the dose to the tumor bed can improve outcome in pediatric ependymoma and possibly lower the local recurrence rate in the tumor bed, while sparing the surrounding OARs, compared with standard IMRT dose. This postulate can be criticized, insofar as high doses may cause late side effects and toxicity in children, as they are more radiosensitive than adults on account of their ongoing growth and development (26). Nonetheless, we found that SIB dose escalation did not increase the dose to most OARs with SIB-VMAT, and actually decreased it with SIB-IMPT. Moreover, some studies of CNS reirradiation in pediatric patients (26–29) and irradiation of pediatric or adult brain tumors such as skull-base chordoma (30–32) have reported that OARs can tolerate high doses, without reaching high toxicity rates. In Rombi et al. (30), for instance, high radiation doses were used at levels similar to those used in adult patients [i.e., up to 74 Gy(RBE)]. Proton therapy combined with optimum surgery offers realistic chances of a cure, with acceptable risks of side effects.
Fung et al. (31) reported that no brainstem or spinal cord complications were observed in their series, even though doses of up to 64 and 55 Gy(RBE) were delivered to the surface of these two structures. Merchant et al. (33) reported that dose, volume, and recovery of brainstem function were unrelated in a cohort of patients treated with doses up to 59.4 Gy. Brainstem recovery after surgery for infratentorial ependymoma was time-dependent and mediated by a number of factors, including the number of tumor resections, age at time of irradiation, sex, CSF shunting, and tumor volume. There are no specific data on doses and toxicity to brainstem for the application of the prospective AIEOP protocol (18) to pediatric patients with ependymoma treated with high-dose SRT to tumor residue. Indelicato et al. (34) identified age <5 years, posterior fossa tumor location, and specific dosimetric parameters as factors associated with an increased risk of brainstem toxicity for pediatric brain tumors, including ependymomas, following passively scattered proton therapy (PSPT). They recommended lowering doses to the brainstem as much as possible, and established guidelines to allow for the safe delivery of proton radiation (35). However, as discussed by MacDonald (36), there is still much to be learned about the interplay between the long-term impact of proton therapy on normal tissues and an individual's radiation sensitivity, such that specific adjustments to treatment may ultimately be required to maximize the benefit of therapy and minimize the risks.
Until we have more data on brainstem toxicity with new proton techniques, we would advise against using dose escalation for infratentorial tumors encasing the brainstem or with medullary involvement.
We are not suggesting that SIB should substitute second-look surgery in the case of residue, but it should be seriously considered in the case of unresectable residue or FLAIR abnormalities in post-operative imaging (17). Therefore, we think that one promising avenue would be the careful stratification of the population eligible to undergo the dose increase, based on residue presence (18), advanced imaging factors (17, 37), and molecular biology (38).
The concept of integrated boost adopted in our study differs from the ongoing European protocol that recommends stereotactic boost solely in the case of residual disease. Given the high local relapse rate within fields even without residue, we think that this integrated boost technique is promising and seems safe for supratentorial tumors.
Until now, radiation oncologists have almost exclusively used PSPT or conformal proton therapy (11, 12, 34). IMPT is dosimetrically better than PSPT in terms of homogeneous target coverage and OAR sparing (11, 39, 40). However, current IMPT methods have many drawbacks. In particular, compared with IMRT, they are highly vulnerable to uncertainties.
In current proton therapy practice, the RBE of protons relative to photons is simplistically assumed to have a constant value of 1.1 in all situations. However, it is becoming increasingly obvious that RBE varies widely along the path of a proton beam. As a consequence, the biologically effective dose distributions that are currently delivered may lead to suboptimal treatments and unforeseen local failures or toxicities. Clinical evidence of the variable biological effectiveness of protons in pediatric patients treated for ependymoma was reported in a recent study (41).
Studies investigating the impact of a variable RBE have also reported substantial differences, compared with the use of a constant factor of 1.1 (42–46). At present, RBE-based treatment is not recommended, but LET-guided optimization (47) should improve outcomes.
The first potential limitation of the present study is our use of the geometry-based PTV for IMPT. However, as we wanted to compare the two different modalities, it made more sense to use it for IMPT and VMAT planning. Furthermore, the dose-volume constraints in the pediatric treatment protocol were specifically set for photons, and not for protons. Nonetheless, as there is not yet any consensus in the medical community, PTV-based planning remains the standard of care for proton therapy in France. The second potential limitation is that we did not include a comparison of normal tissue complication probabilities for selected organs and expected tumor control probability, as has been done in some studies (48, 49). However, image-guidance technology may vary for proton and photon radiotherapy, affecting the PTV margins, and therefore, normal tissue complication probabilities, with an attendant impact on the selection of patients eligible for proton therapy (50).
In our study, doses for IMPT were calculated with the pencil beam algorithm. Using Monte Carlo (MC) techniques, or their accelerated variants (accelerated MC), would help to overcome the limitations of such approximations and assumptions. MC methods are also essential for calculating other physical quantities (e.g., LET) required for computing RBE. In our study, however, we used a version of RayStation in which proton MC was not yet available.
Owing to the use of a range shifter, the penumbra was relatively large. This factor needs to be taken into account when looking for ways of improving results. We intuitively assume that the smaller the spot size, the sharper the penumbra and the more conformal the PT dose distribution. Aside from the use of smaller spot sizes, there is now a method for significantly improving the penumbra, by introducing an aperture in the IMPT plan. This technique is now supported in RayStation and is in clinical use in several proton sites. All current systems use the smallest possible spot size for all spots and energies. As reported in Mohan et al. (39), it will be possible to improve IMPT if treatment planning is first improved, by reducing uncertainties in proton range, enhancing adaptive treatment planning and delivery, and considering how to exploit the variability of proton RBE for clinical purposes.
Establishing a radiotherapy treatment plan is a complex procedure, and its quality and results depend on both the planner's expertise and fixed constraints. To allow for unbiased comparisons between IMPT and IMRT for each patient, automation of the treatment planning process should be considered (51) and explored. Then again, these treatment plans are highly personalized, compared with other tumor sites that are more easily automatized (50). Finally, since IMPT will be used largely for pediatric patients, it is important to consider models and measures of the neutron dose contribution induced by the secondary neutrons.
Dose escalation in pediatric ependymoma with either modulated-intensity photon or proton techniques is feasible in some patients, and in most cases, the use of protons results in a significant reduction in the dose to OARs. Caution needs to be exercised with infratentorial tumors, given the recent data on brainstem toxicity, and dose escalation should be contraindicated in the case of large tumors encasing more than half the brainstem perimeter or with cervical medullary involvement. We think that this approach could be considered in patients with supratentorial tumors with a high risk of relapse (i.e., unresectable residue or post-operative FLAIR abnormalities).
This study was approved by the national French ethics committee and the French data protection authority (CNIL: Commission nationale de l'informatique et des libertés de France).
FT contributed to the study design, data collection, data analysis, treatment plan generation, and manuscript writing. AD contributed to data collection, study design, and manuscript revision. LéC performed statistical analysis and manuscript revision. SB, JH, CA, BC-D, VB, LiC, CC, LP, XM, SS, AH, JL, and CK contributed to the clinical aspect of the study design, data collection, and manuscript revision. GH, AlL, FG, and RF contributed to the medical physics aspect of the study design, treatment planning, data collection, and manuscript revision. AnL was the PI of this project, contributed to the study design, validation of treatment planning and manuscript revision, and provided overall guidance.
We are grateful to the French Society for Childhood Cancer (SFCE) and to the association Hubert Gouin Enfance et Santé for supporting this work.
The authors declare that the research was conducted in the absence of any commercial or financial relationships that could be construed as a potential conflict of interest.
We would like to thank: Anne Beaudre, Pauline Comte, Eliane Graulières, Emilie Meknaci, Frédérique Recordon, Laetitia Solignac, Amandine Saint-Martin, Marie Vidal, Laure Vieillevigne, les clubs Rotary de Toulouse, and the French league against cancer.
The Supplementary Material for this article can be found online at: https://www.frontiersin.org/articles/10.3389/fonc.2019.00531/full#supplementary-material
1. Rousseau P, Habrand JL, Sarrazin D, Kalifa C, Terrier-Lacombe MJ, Rekacewicz C, et al. Treatment of intracranial ependymomas of children: review of a 15-year experience. Int J Radiat Oncol Biol Phys. (1994) 28:381–6. doi: 10.1016/0360-3016(94)90061-2
2. Perilongo G, Massimino M, Sotti G, Belfontali T, Masiero L, Rigobello L, et al. Analyses of prognostic factors in a retrospective review of 92 children with ependymoma: Italian pediatric neuro-oncology group. Med Pediatr Onco. (1997) 29:79–85.
4. Brodin NP, Munck Af Rosenschöld P, Aznar MC, Kiil-Berthelsen A, Vogelius IR, Nilsson P, et al. Radiobiological risk estimates of adverse events and secondary cancer for proton and photon radiation therapy of pediatric medulloblastoma. Acta Oncol. (2011) 50:806–16. doi: 10.3109/0284186X.2011.582514
5. Miralbell R, Lomax A, Cella L, Schneider U. Potential reduction of the incidence of radiation-induced second cancers by using proton beams in the treatment of pediatric tumors. Int J Radiat Oncol Biol Phys. (2002) 54:824–9. doi: 10.1016/S0360-3016(02)02982-6
6. Athar BS, Paganetti H. Comparison of second cancer risk due to out-of-field doses from 6-MV IMRT and proton therapy based on 6 pediatric patient treatment plans. Radiother Oncol. (2011) 98:87–92. doi: 10.1016/j.radonc.2010.11.003
7. Kristensen I, Nilsson K, Nilsson P. Comparative proton and photon treatment planning in pediatric patients with various diagnoses. Int J Part Ther. (2015) 2:367–75. doi: 10.14338/IJPT-14-00026.1
8. Armoogum KS, Thorp N. Dosimetric Comparison and potential for improved clinical outcomes of paediatric CNS patients treated with protons or IMRT. Cancers (Basel). (2015) 7:706–22. doi: 10.3390/cancers7020706
9. Takizawa D, Mizumoto M, Yamamoto T, Oshiro Y, Fukushima H, Fukushima T, et al. A comparative study of dose distribution of PBT, 3D-CRT and IMRT for pediatric brain tumors. Radiat Oncol. (2017) 12:40. doi: 10.1186/s13014-017-0775-2
10. Sato M, Gunther JR, Mahajan A, Jo E, Paulino AC, Adesina AM, et al. Progression-free survival of children with localized ependymoma treated with intensity-modulated radiation therapy or proton-beam radiation therapy. Cancer. (2017) 123:2570–8. doi: 10.1002/cncr.30623
11. MacDonald SM, Safai S, Trofimov A, Wolfgang J, Fullerton B, Yeap BY, et al. Proton radiotherapy for childhood ependymoma: initial clinical outcomes and dose comparisons. Int J Radiat Oncol Biol Phys. (2008) 71:979–86. doi: 10.1016/j.ijrobp.2007.11.065
12. Mac Donald SM, Sethi R, Lavally B, Yeap BY, Marcus KJ, Caruso P, et al. Proton radiotherapy for pediatric central nervous system ependymoma: Clinical outcomes for 70 patients. Neuro Oncol. (2013) 15:1552–9. doi: 10.1093/neuonc/not121
13. Pulsifer MB, Sethi R V, Kuhlthau KA, MacDonald SM, Tarbell NJ, Yock TI. Early cognitive outcomes following proton radiation in pediatric patients with brain and central nervous system tumors. Int J Radiat Oncol Biol Phys. (2015) 93:400–7. doi: 10.1016/j.ijrobp.2015.06.012
14. Ducassou A, Padovani L, Chaltiel L, Bolle S, Habrand J-L, Claude L, et al. Pediatric localized intra cranial ependymomas: a multicenter analysis of the children's cancer french society (SFCE). Int J Radiat Oncol. (2018) 102:166–73. doi: 10.1016/j.ijrobp.2018.05.036
15. Merchant TE. Current clinical challenges in childhood ependymoma: a focused review. J Clin Oncol. (2017) 35:2364–9. doi: 10.1200/JCO.2017.73.1265
16. Tensaouti F, Ducassou A, Chaltiel L, Bolle S, Muracciole X, Coche-Dequeant B, et al. Patterns of failure after radiotherapy for pediatric patients with intracranial ependymoma. Radiother Oncol. (2017) 122:362–7. doi: 10.1016/j.radonc.2016.12.025
17. Tensaouti F, Ducassou A, Chaltiel L, Sevely A, Bolle S, Padovani L, et al. Imaging biomarkers of outcome after radiotherapy for pediatric ependymoma. Radiother Oncol. (2018) 127:103–7. doi: 10.1016/j.radonc.2018.02.008
18. Massimino M, Miceli R, Giangaspero F, Boschetti L, Modena P, Antonelli M, et al. Final results of the second prospective AIEOP protocol for pediatric intracranial ependymoma. Neuro Oncol. (2016) 18:1451–60. doi: 10.1093/neuonc/now108
19. Prescribing recording and reporting photon-beam intensity-modulated radiation therapy (IMRT): contents. J ICRU. (2010) 10. doi: 10.1093/jicru/ndq002
20. van Veelen-Vincent M-LC, Pierre-Kahn A, Kalifa C, Sainte-Rose C, Zerah M, Thorne J, et al. Ependymoma in childhood: prognostic factors, extent of surgery, and adjuvant therapy. J Neurosurg. (2002) 97:827–35. doi: 10.3171/jns.2002.97.4.0827
21. Figarella-Branger D, Civatte M, Bouvier-Labit C, Gouvernet J, Gambarelli D, Gentet J-C, et al. Prognostic factors in intracranial ependymomas in children. J Neurosurg. (2000) 93:605–13. doi: 10.3171/jns.2000.93.4.0605
22. Witt H, Mack SC, Ryzhova M, Bender S, Sill M, Isserlin R, et al. Delineation of two clinically and molecularly distinct subgroups of posterior fossa ependymoma. Cancer Cell. NIH (2011) 20:143–57. doi: 10.1016/j.ccr.2011.07.007
23. Sabin ND, Merchant TE, Li X, Li Y, Klimo P, Boop FA, et al. Quantitative imaging analysis of posterior fossa ependymoma location in children. Childs Nerv Syst. (2016) 32:1441–7. doi: 10.1007/s00381-016-3092-4
24. Rombi B, Vennarini S, Vinante L, Ravanelli D, Amichetti M. Proton radiotherapy for pediatric tumors: review of first clinical results. Ital J Pediatr. (2014) 40:74. doi: 10.1186/s13052-014-0074-6
25. Ken S, Vieillevigne L, Franceries X, Simon L, Supper C, Lotterie J-A, et al. Integration method of 3D MR spectroscopy into treatment planning system for glioblastoma IMRT dose painting with integrated simultaneous boost. Radiat Oncol. (2013) 8:1. doi: 10.1186/1748-717X-8-1
26. Rao AD, Rashid AS, Chen Q, Villar RC, Kobyzeva D, Nilsson K, et al. Reirradiation for recurrent pediatric central nervous system malignancies: a multi-institutional review. Int J Radiat Oncol. (2017) 99:634–41. doi: 10.1016/j.ijrobp.2017.07.026
27. Eaton BR, Chowdhry V, Weaver K, Liu L, Ebb D, MacDonald SM, et al. Use of proton therapy for re-irradiation in pediatric intracranial ependymoma. Radiother Oncol. (2015) 116:301–8. doi: 10.1016/j.radonc.2015.07.023
28. Tsang DS, Burghen E, Klimo P, Boop FA, Ellison DW, Merchant TE. Outcomes after reirradiation for recurrent pediatric intracranial ependymoma. Int J Radiat Oncol. (2018) 100:507–15. doi: 10.1016/j.ijrobp.2017.10.002
29. Régnier E, Laprie A, Ducassou A, Bolle S, Supiot S, Muracciole X, et al. Re-irradiation of locally recurrent pediatric intracranial ependymoma: Experience of the French society of children's cancer. Radiother Oncol. (2019) 132:1–7. doi: 10.1016/j.radonc.2018.11.009
30. Rombi B, Ares C, Hug EB, Schneider R, Goitein G, Staab A, et al. Spot-scanning proton radiation therapy for pediatric chordoma and chondrosarcoma: Clinical outcome of 26 patients treated at paul scherrer institute. Int J Radiat Oncol Biol Phys. (2013) 86:578–84. doi: 10.1016/j.ijrobp.2013.02.026
31. Fung V, Calugaru V, Bolle S, Mammar H, Alapetite C, Maingon P, et al. Proton beam therapy for skull base chordomas in 106 patients: a dose adaptive radiation protocol. Radiother Oncol. (2018) 128:198–202. doi: 10.1016/j.radonc.2017.12.017
32. Verma V, Rwigema J-CM, Malyapa RS, Regine WF, Simone CB. Systematic assessment of clinical outcomes and toxicities of proton radiotherapy for reirradiation. Radiother Oncol. (2017) 125:21–30. doi: 10.1016/j.radonc.2017.08.005
33. Merchant TE, Chitti RM, Li C, Xiong X, Sanford RA, Khan RB. Factors associated with neurological recovery of brainstem function following post-operative conformal radiation therapy for infratentorial ependymoma. Int J Radiat Oncol Biol Phys. (2010) 76:496–503. doi: 10.1016/j.ijrobp.2009.01.079
34. Indelicato DJ, Flampouri S, Rotondo RL, Bradley JA, Morris CG, Aldana PR, et al. Incidence and dosimetric parameters of pediatric brainstem toxicity following proton therapy. Acta Oncol (Madr). (2014) 53:1298–304. doi: 10.3109/0284186X.2014.957414
35. Haas-Kogan D, Indelicato D, Paganetti H, Esiashvili N, Mahajan A, Yock T, et al. National cancer institute workshop on proton therapy for children: considerations regarding brainstem injury. Int J Radiat Oncol. (2018) 101:152–68. doi: 10.1016/j.ijrobp.2018.01.013
36. MacDonald SM, Laack NN, Terezakis S. Humbling advances in technology: protons, brainstem necrosis, and the self-driving car. Int J Radiat Oncol Biol Phys. (2017) 97:216–9. doi: 10.1016/j.ijrobp.2016.08.001
37. Tensaouti F, Ducassou A, Chaltiel L, Sevely A, Bolle S, Muracciole X, et al. Prognostic and predictive values of diffusion and perfusion MRI in paediatric intracranial ependymomas in a large national study. Br J Radiol. (2016) 89:20160537. doi: 10.1259/bjr.20160537
38. Pajtler KW, Witt H, Sill M, Jones DTW, Hovestadt V, Kratochwil F, et al. Molecular classification of ependymal tumors across all CNS compartments, histopathological grades, and age groups. Cancer Cell. (2015) 27:728–43. doi: 10.1016/j.ccell.2015.04.002
39. Mohan R, Das IJ, Ling CC. Empowering intensity modulated proton therapy through physics and technology: an overview. Int J Radiat Oncol. (2017) 99:304–16. doi: 10.1016/j.ijrobp.2017.05.005
40. Ladra MM, MacDonald SM, Terezakis SA. Proton therapy for central nervous system tumors in children. Pediatr Blood Cancer. (2018) 65:e27046. doi: 10.1002/pbc.27046
41. Peeler CR, Mirkovic D, Titt U, Blanchard P, Gunther JR, Mahajan A, et al. Clinical evidence of variable proton biological effectiveness in pediatric patients treated for ependymoma. Radiother Oncol. (2016) 121:395–401. doi: 10.1016/j.radonc.2016.11.001
42. Carabe A, España S, Grassberger C, Paganetti H. Clinical consequences of relative biological effectiveness variations in proton radiotherapy of the prostate, brain and liver. Phys Med Biol. (2013) 58:2103–17. doi: 10.1088/0031-9155/58/7/2103
43. Frese MC, Wilkens JJ, Huber PE, Jensen AD, Oelfke U, Taheri-Kadkhoda Z. Application of constant vs. variable relative biological effectiveness in treatment planning of intensity-modulated proton therapy. Int J Radiat Oncol Biol Phys. (2011) 79:80–8. doi: 10.1016/j.ijrobp.2009.10.022
44. Giovannini G, Böhlen T, Cabal G, Bauer J, Tessonnier T, Frey K, et al. Variable RBE in proton therapy: comparison of different model predictions and their influence on clinical-like scenarios. Radiat Oncol. (2016) 11:68. doi: 10.1186/s13014-016-0642-6
45. Tilly N, Johansson J, Isacsson U, Medin J, Blomquist E, Grusell E, et al. The influence of RBE variations in a clinical proton treatment plan for a hypopharynx cancer. Phys Med Biol. (2005) 50:2765–77. doi: 10.1088/0031-9155/50/12/003
46. Underwood T, Giantsoudi D, Moteabbed M, Zietman A, Efstathiou J, Paganetti H, et al. Can we advance proton therapy for prostate? considering alternative beam angles and relative biological effectiveness variations when comparing against intensity modulated radiation therapy. Int J Radiat Oncol Biol Phys. (2016) 95:454–64. doi: 10.1016/j.ijrobp.2016.01.018
47. Giantsoudi D, Grassberger C, Craft D, Niemierko A, Trofimov A, Paganetti H. Linear energy transfer-guided optimization in intensity modulated proton therapy: feasibility study and clinical potential. Int J Radiat Oncol Biol Phys. (2013) 87:216–22. doi: 10.1016/j.ijrobp.2013.05.013
48. Cheng Q, Roelofs E, Ramaekers BLT, Eekers D, Van Soest J, Lustberg T, et al. Development and evaluation of an online three-level proton vs photon decision support prototype for head and neck cancer–Comparison of dose, toxicity, and cost-effectiveness. Radiother Oncol. (2016) 118:281–5. doi: 10.1016/j.radonc.2015.12.029
49. Langendijk JA, Lambin P, De Ruysscher D, Widder J, Bos M, Verheij M. Selection of patients for radiotherapy with protons aiming at reduction of side effects: the model-based approach. Radiother Oncol. (2013) 107:267–73. doi: 10.1016/j.radonc.2013.05.007
50. Hoogeman M, Arts T, van de Water S, der Voort S, Perkó Z, Lathouwers D, et al. SP-0010: Selection of patients for proton therapy: a physicists view. Radiother Oncol. (2016) 119:S4. doi: 10.1016/S0167-8140(16)31259-2
Keywords: photon therapy, proton therapy, boost, treatment planning, ependymoma, intracranial
Citation: Tensaouti F, Ducassou A, Chaltiel L, Bolle S, Habrand JL, Alapetite C, Coche-Dequeant B, Bernier V, Claude L, Carrie C, Padovani L, Muracciole X, Supiot S, Huchet A, Leseur J, Kerr C, Hangard G, Lisbona A, Goudjil F, Ferrand R and Laprie A (2019) Feasibility of Dose Escalation in Patients With Intracranial Pediatric Ependymoma. Front. Oncol. 9:531. doi: 10.3389/fonc.2019.00531
Received: 22 December 2018; Accepted: 31 May 2019;
Published: 21 June 2019.
Edited by:
John Varlotto, University of Massachusetts Medical School, United StatesReviewed by:
Joshua Silverman, New York University, United StatesCopyright © 2019 Tensaouti, Ducassou, Chaltiel, Bolle, Habrand, Alapetite, Coche-Dequeant, Bernier, Claude, Carrie, Padovani, Muracciole, Supiot, Huchet, Leseur, Kerr, Hangard, Lisbona, Goudjil, Ferrand and Laprie. This is an open-access article distributed under the terms of the Creative Commons Attribution License (CC BY). The use, distribution or reproduction in other forums is permitted, provided the original author(s) and the copyright owner(s) are credited and that the original publication in this journal is cited, in accordance with accepted academic practice. No use, distribution or reproduction is permitted which does not comply with these terms.
*Correspondence: Fatima Tensaouti, dGVuc2FvdXRpLmZhdGltYUBpdWN0LW9uY29wb2xlLmZy; ZmF0aW1hLnRlbnNhb3V0aUBpbnNlcm0uZnI=
Disclaimer: All claims expressed in this article are solely those of the authors and do not necessarily represent those of their affiliated organizations, or those of the publisher, the editors and the reviewers. Any product that may be evaluated in this article or claim that may be made by its manufacturer is not guaranteed or endorsed by the publisher.
Research integrity at Frontiers
Learn more about the work of our research integrity team to safeguard the quality of each article we publish.