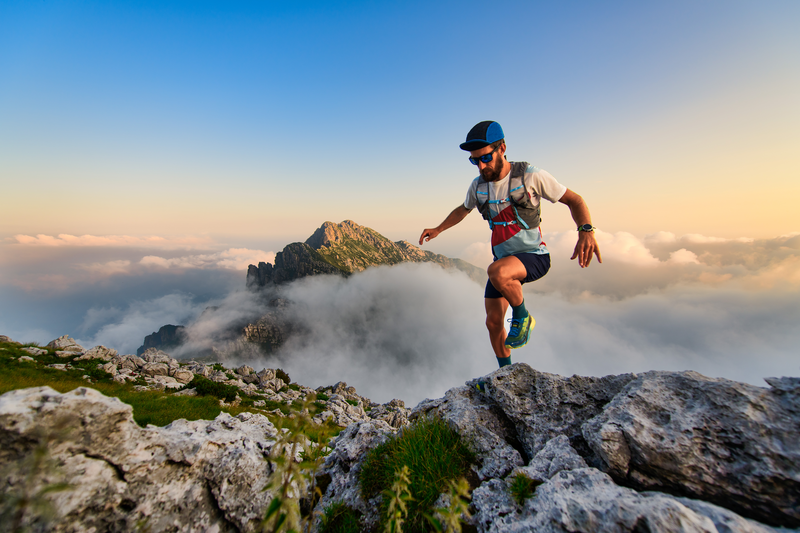
94% of researchers rate our articles as excellent or good
Learn more about the work of our research integrity team to safeguard the quality of each article we publish.
Find out more
PERSPECTIVE article
Front. Oncol. , 26 March 2019
Sec. Radiation Oncology
Volume 9 - 2019 | https://doi.org/10.3389/fonc.2019.00180
Targeted radiopharmaceuticals for therapeutic use deliver radionuclides directly to tumor anywhere in the body, and therefore, have renewed interest for clinical development in women with disseminated chemorefractory ovarian cancers. About two in every five women with advanced stage ovarian cancer outlive their disease after the first treatment phase, with the rest rendered incurable due to the chemorefractory nature of their disease. The National Cancer Institute (NCI) Cancer Therapy Evaluation Program conducted 67 phase I or phase Ib trials among women with relapsed or refractory ovarian cancer between 1989 and 2017 in an effort to uncover tolerable and effective drug combinations intended to increase survival rates. None of these early clinical development phase trials involved radiopharmaceuticals. Here, the NCI provides its perspective on targeted radiopharmaceutical conjugates alone or in combination with its experimental therapeutics portfolio for women with relapsed or refractory ovarian cancer. An infrastructure build for Federal radiopharmaceutical medical monitoring and adverse event reporting has begun.
In 2018, ovarian cancers collectively represent the tenth (2.5%) most common any-type cancer in American women (1). However, ovarian cancers are the fifth (5%) leading cause of cancer-related death in American women (1). This is mostly a consequence of the finding that four out of every five women with ovarian cancer present with advanced stage disease disseminating throughout the abdominal cavity at initial diagnosis (1). Half of all American women diagnosed with ovarian cancer are younger than age 63 years (1). First and subsequent phases of treatment for women with this disease undergo surgery followed by platinum-based chemotherapy, integrating second-look debulking surgeries for minimally chemotherapy responding disease under appropriate conditions. Five-year survival rates for such treated women might be as high as 73 percent when disease is confined to the abdominal cavity, but might be as low as 29 percent when disease metastasizes outside the abdominal cavity (1). For women with advanced stage ovarian cancer, the US National Cancer Institute (NCI) Cancer Therapy Evaluation Program has studied experimental therapeutic agents in 67 phase I or phase Ib trials between 1989 and 2017. All clinical development plans intended to raise overall disease remission and mortality rates. Through novel agent discovery, about one-half of women currently outlive their ovarian cancer long-term (2). Those women unable to achieve a disease-free status after initial phases of treatment, often due to occult chemorefractory disease in the abdomen, ultimately die of their disease (2).
A standard approach to the first phase of ovarian cancer treatment is abdominal surgery followed by carboplatin-paclitaxel chemotherapy (3). A novel approach for next-generation clinical trials seek to incorporate targeted radiopharmaceuticals that integrated into first-line treatment either to augment chemotherapy effects or to eradicate chemotherapy insensitive but radiation sensitive disease (4). There is historical precedence for such an approach. For example, intrabdominal instillation of the β-particle emitter 32P chromic phosphate was studied in four randomized clinical trials of women with advanced stage ovarian cancer (5–8). 32P chromic phosphate as a single infusion was well-tolerated and deemed clinically beneficial (5–8). The further clinical development of early radiopharmaceuticals was stopped due to clunky logistics for 32P chromic phosphate instillation and a clinical desire to test newer chemotherapy agents. Targeted α-particle radiopharmaceutical conjugates combine the affinity and specificity of molecular targeting with cytotoxic energy-rich radiation delivered anywhere in the body to cancer cells and their microenvironment for disease control (9). As expected, the radioactive payload of targeted α-particle radiopharmaceutical conjugates drives the efficacy and toxicity of these agents. But, there is also the possibility that the targeting ligand and cleaved/non-cleaved chemical linkers may also contribute to organ-specific toxicity. As NCI leads clinical development of radiopharmaceuticals in the US, it becomes increasingly important to collect and to report radiopharmaceutical-related adverse events in early phase clinical trials for treatment of patients with ovarian cancer or other targeted diseases.
Adverse event reporting, or colloquially toxicity reporting, is compulsory in human subject research to ensure research subject safety and to appreciate the safety profile of treatment agents alone or in combination (10). Existing methods like the NCI's Common Terminology Criteria for Adverse Events (CTCAE, version 5) are reliable and accurate for describing toxicities on a five-point scale based on clinical criteria. In trial reports, toxicity data are described often via a summary table of the number and the proportion of high-grade 3 (severe or medically significant), 4 (life-threatening), or 5 (lethal) toxicities during the observational timespan of a trial. But, these same tables include little to no data on the cumulative severity or onset of toxicities during the first or subsequent course of treatment. These factors might also factor into the determination of whether a treatment is deemed tolerable (11). Moreover, it is worthwhile to describe toxicities in relation to drug schedule or intensity (Table 1). In modern drug clinical development, acute toxicities are considered to arise over a brief timeframe after drug exposure and might be transient, reversible, or persistent (11). At the other extreme, chronic toxicities arise over a long time period and are considered persistent and unremitting, or intermittent and recurring. Chronic toxicities manifest late such as after the first phase or cycle of treatment (11). Cumulative toxicities intensify and arise after repeated phases of treatment exposure (11). Late toxicities result in subclinical manifestations that do not fit immediate, intermittent, or short-term adverse clinical events, but rather are evident over time such as after multiple phases of treatment (11). Radiopharmaceutical treatments introduce the need for a new term among these definitions—subacute toxicities. Subacute toxicities are adverse events that arise over an intermediate time period, such as 1–3 months post-therapy, and might be transient, reversible, or persistent in duration. An example could be radiation-induced pneumonitis manifesting as non-productive cough 3 months after initial radiation dose exposure.
For this article, the NCI provides its perspective on workflow for collecting and for reporting information about subacute toxicities and other categories of adverse events for the clinical development of radiopharmaceuticals. NCI's prior and possible future radiopharmaceutical experience in the treatment of patients with advanced stage relapsed or refractory ovarian cancer provides context for this workflow.
Adapting NCI's CTCAE criteria either for the presence/absence of toxicity or for its grading of severity presents challenges to radiopharmaceutical clinical development. The NCI recognizes five discrete categories for any given CTCAE term that radiopharmaceutical-attributed toxicity must fit (Table 2) (10)—(A) laboratory/biomarker based toxicity that requires equipment to detect (like anemia, leukopenia, neutropenia, or thrombocytopenia); (B) observable/measurable toxicity that requires technical training to delineate (like eye examination for tearing caused by corneal or limbic irritation); (C) primarily subjective toxicity without observable components (like radiation-induced nausea); (D) primarily subjective toxicity with observable components (like radiation-induced diarrhea); and (E) primarily observable toxicity with subjective components (like radiation-induced alopecia). NCI considers categories A and B, as it applies to radiopharmaceuticals, to follow the established generic CTCAE terminology and grading of severity. This is because category A and B toxicities require either radiotherapy-independent clinical expertise for evaluation, or, technical equipment. Category E toxicities lend themselves to be identified by patients but require clinical expertise to assign severity and follow the established generic CTCAE terminology and grading of severity. Categories C and D toxicities have elements of frequency, severity, or interference with usual or daily activities noticed by patients (10). Thus, these categories of toxicity might be amenable to study by electronic patient-reported outcome measures, in a pilot or a formal trial project, rather than just description by generic CTCAE criteria (10). For instance, pretherapy severity of constipation prior to radium-223 administration might be important to capture using a patient-reported outcome-CTCAE (PRO-CTCAE) method. This is because radium-223 is actively eliminated from the body via the large intestine. Any delay in stool evacuation might intensify the frequency, severity, or interference of bowel toxicity as protracted bowel dwell time of radium-223 irradiates a longer length of radiation-sensitive bowel. For radiopharmaceuticals, category C or D toxicity can be anticipated due to prior clinical observations with conventional radiotherapy. Thus, NCI might plan to collect select category C or D toxicity (like constipation) as an adverse event of special interest. An adverse event of special interest identifies a toxicity for which an expedited adverse event report must be filed to the NCI in its sponsored trials (12). The NCI remains willing to lead clinical development of targeted radiopharmaceutical conjugates because elements of its clinical trial enterprise, such as medical monitoring and safety data reporting, are efficient and cost-effective for such endeavors now and in the foreseeable future. However, unique challenges arise, and these challenges can be appreciated in the context of radiopharmaceutical clinical use in women with advanced stage ovarian cancer.
Radiopharmaceuticals might be inhaled, ingested, instilled, or infused by vein. These radioactive drugs aim for accurate and precise molecular delivery of energy-rich radiation to cancer cells either circulating in the blood or in tumors (Figure 1). Radiopharmaceuticals are either neat (i.e., lack a targeting ligand) or conjugated (i.e., have a ligand-linker-payload construct). The radiopharmaceutical radium-223 dichloride falls into the neat class. This is because it is given by vein as a slow bolus solution that tracks to areas of bone turnover as a calcium mimetic without the aid of a ligand (9). In an opposite way, thorium-227-containing radiopharmaceuticals (the parent radionuclide of radium-223) are in the conjugated class. This conjugated molecular entity has three components—a radioactive payload, a linker, and an antibody. Each component possibly contributes to its safety profile. For this reason, NCI has adopted the approach for conjugated radiopharmaceuticals to consider the safety of radionuclide, its cleaved/non-cleaved linker, and “cold” radiopharmaceutical antibody prior to launching clinical trials of the radiopharmaceutical.
Figure 1. Strategy for radiopharmaceuticals targeting ovarian cancers. A mesothelin-targeted radiopharmaceutical and its anticipated normal organ toxicities are charted in relation to thorium-227 radionuclide delivery and adverse events (toxicities) of special interest. The peritoneum, pleura, cornea/limbus of the eye, and pericardium show molecular expression of mesothelin and are listed together with associated body fluids that might have detectable levels of mesothelin for off-target radiopharmaceutical localization. Marked in boxes are the mechanistic elements of antibody conjugate processing likely to be involved in intended irradiation of tumor cells or in unintended toxicity of normal cells. Challenges for radiopharmaceutical are illustrated and commented upon (blue boxes).
Thorium-227 has begun clinical trial testing among women with advanced stage ovarian cancer (NCT03507452). Thorium-227 can be encased by octadentate chelates of the 3,2 hydroxypyridinone (3,2-HOPO) class (13). As an alpha-particle emitting radionuclide, it is a highly potent cytotoxic payload. Given that general knowledge of organ-specific radiation-induced toxicities are sufficient to address safety from radionuclide radioactivity (14), the path for the thorium-227 anti-mesothelin monoclonal antibody conjugate radiopharmaceutical to enter the clinic was relatively straightforward. In a clinical study, a radiopharmaceutical like this might possibly associate with exhaustion, low blood cell or platelet count, gastrointestinal upset, or even specific normal organ toxicity due to the normal expression of the targeted antigen (Figure 1). NCI has reviewed its prior sponsored experience with radiopharmaceuticals in women with advanced stage ovarian cancer (5–8), and considers the toxicity profile of such experimental therapeutic radiopharmaceuticals of acceptable risk for early phase clinical trial study.
The thorium-227 anti-mesothelin monoclonal antibody conjugate radiopharmaceutical uses a 3,2-HOPO chelate to house thorium-227 and link it to a tumor-targeting antibody, and in this case, against mesothelin (13). This antibody has undergone prior clinical development as anetumab ravtansine (15–17). Usual radiochemical purity is 99 percent or greater (13), meaning the 3,2-HOPO chelate linker-antibody is in excess of the thorium-227.
Linker chemistry is important to the distribution of a radioactive payload. While linker as a molecular entity may not contribute to the frequency, severity, or interference of radiopharmaceutical-related toxicity, the stability of the linker does have an impact upon which organs or tissue are affected. Stable non-cleaved linkers restrict payloads to targets for narrow toxicity profiles, whilst less stable cleaved linkers might allow payloads to drift away from targets creating much broader toxicity. Cleavable linkers typically release payloads after processing in endosomes or lysosomes via a variety of mechanisms including acidic degradation, protease cleavage, and thiol-disulfide exchange reactions (Figure 1). The thorium-227 anti-mesothelin monoclonal antibody conjugate radiopharmaceutical is viewed as not having a cleavable linker (13). And so, complete lysosomal degradation of the antibody, alpha-particle mediated damage to the 3,2-HOPO chelate, or loss of decayed daughter radionuclides must manifest for unintended organ or tissue toxicity (Figure 1).
Radiopharmaceutical antibody chemistry demands high affinity target antigen binding for tumor cells and low affinity for normal cells for a realistic therapeutic window. It is therefore desirable for cancer cell-directed antibodies to seek antigens expressed on the surface of those cancer cells prior to internalization and endosomal processing (Figure 1). But, normal cells might also express the target antigen. The antigen mesothelin provides an illustrative example as it is highly expressed in ovarian cancers (16) but also found on the surface of some normal cells like the abdominal peritoneum, lung pleura, eye cornea/limbus, and heart pericardium (17, 18). “Cold” pharmaceutical ligands might have off-target biological effects alone that reflect non-specific or inappropriate target antigen recognition on normal cells. Off-target effects might manifest as toxicity. In the example of thorium-227 anti-mesothelin monoclonal antibody conjugate, the “cold” 3,2-HOPO chelate-antibody pharmaceutical toxicity has not been well-characterized. The ongoing phase I trial in women with advanced stage ovarian cancer should provide this clinical context (NCT03507452).
It bears to reiterate that low level target antigen expression on normal cells may result in specific toxicity, and, cleavage or damage to the linker may induce unintended organ toxicities from free radionuclide. In its effort to study novel radiopharmaceuticals, the NCI has placed an emphasis on a few key pharmacokinetic properties and toxicities (Table 3, Figure 1). One Category C CTCAE example is fatigue. Radiation exposure has been shown to upregulate expression of nucleoside transporters and kinases in cancer cells, perhaps rescuing cells from replication stress (22). It has been speculated that radiation-related exhaustion might result from skeletal muscle expending energy to furnish deoxynucleosides via the bloodstream to irradiated cells demanding their supply for DNA repair. There may be an opportunity to study circulating deoxynucleoside levels further as biomarkers of response in women with chemorefractory advanced stage ovarian cancer treated by radiopharmaceuticals. Anemia, leukopenia or neutropenia, and thrombocytopenia from enhanced elimination or impaired production of marrow constituents, and not circulating mature cells or platelets, are Category A CTCAE acute or cumulative toxicity effects of interest to the NCI. Pneumonitis or eye corneal/limbic keratitis represent two transient, reversible, or persistent Category B CTCAE subacute toxicity effects attributable in a 3-month window following radiopharmaceutical exposure. These are of particular interest to the NCI in its clinical development plans.
The NCI has considered its position on the conduct and the medical monitoring of radiopharmaceutical clinical investigations. The NCI aligns its thoughts on this topic with those guidances provided by the US Food and Drug Administration (23). The NCI considers principal investigator as the responsible leader of a clinical team, meaning they are the individual who both initiates and conducts a clinical investigation and under whose immediate direction an investigational radiopharmaceutical drug is administered or dispensed. No investigator may participate in an NCI-sponsored clinical investigation of a radiopharmaceutical drug until that individual provides the NCI with a completed, signed Statement of Investigator, Form FDA 1572 [21 CFR 312.53(c)]. An investigator personally conducts or supervises a clinical investigation, follows protocol-only changes, ensures that all study staff are informed of protocol-only obligations, informs subjects that radiopharmaceuticals are being used for investigational purposes, ensures informed consent, provides ethics board review, approval and reporting, reports adverse events to NCI as sponsor, reads, and understands the radiopharmaceutical investigator brochure, and maintains adequate and accurate records as well as make those records available for audit by NCI as the sponsor. NCI expects that an investigator administering radiopharmaceuticals complies with all state radiation license regulations and rosters onto a site's radiation authorized user list for the named radiopharmaceutical. NCI also presumes of the radiopharmaceutical investigator study oversight, responsibility for delegation of study tasks or training of study staff, comprehensive supervision inclusive of any third parties. NCI requires a clinical investigator using experimental therapeutics under its sponsored trials to report immediately any adverse event that is alarming (e.g., an unexpected event that is serious or life-threatening) or timely any non-serious adverse events according to its establish NCI timetable recorded in the trial protocol. As trial sponsor, the NCI registers its radiopharmaceutical trials (www.clinicaltrials.gov).
The NCI's current thinking for radiopharmaceutical regulatory safety and pharmacovigilance appears in Figure 2. The components of a site's radiopharmaceutical monitoring plan might include—(A) description of each monitoring method employed during the study, how the plan addresses important risks and ensures validity of timing, frequency, logs, and extent of planned monitoring activities as well as definitions of events triggering changes or deviations in planned monitoring activities; (B) communication of monitoring results inclusive of format, content, timing, and archiving requirements for reports and other documentation of monitoring activities from study management and other stakeholders (like site staff, IRB, NCI, FDA), as necessary; (C) processes for addressing unresolved or significant non-compliance with the investigational plan, inclusive of root cause analyses and appropriate corrective and preventive actions for quality management practices applicable to a clinical investigation; (D) description of specific training required for personnel carrying out monitoring activities, including personnel conducting internal data monitoring, statistical monitoring, or other centralized review activities or planned audits of monitoring; and (E) accounting of monitoring plan amendments.
Figure 2. Proposed workflow for monitoring and audit in radiopharmaceutical trials. Proposed workflow steps are charted in relation to the trial site, the regulatory safety and pharmacovigilance group (PV), or the NCI's Cancer Therapy Evaluation Program (CTEP).
In summary, this article provides NCI's perspective on radiopharmaceutical clinical development from its vantage of medical monitoring of radionuclide, linker, and ligand toxicity. The current NCI position used prior experience from radiopharmaceutical clinical use in ovarian cancer to sharpen its thinking on conjugated radiopharmaceuticals that might be considered for chemorefractory ovarian cancer patient treatment in the near-term. This article does not outlay NCI's position on inhaled, ingested, or otherwise injected radiopharmaceuticals. Important overarching topics related to regulatory safety and pharmacovigilance such as radiochemical impurity, stability, handling, or distribution are not discussed here. Guidances for some of these topics are found elsewhere (14). Patient and clinical provider education on radiopharmaceuticals remains integral to agent clinical development.
The research presented in this article involved the collection or study of existing data, documents, and records that were publicly available, or the information was recorded by NCI in such a manner that trial subjects cannot be identified directly or through identifiers linked to the subjects. The research is regarded exempt from Institutional Review Board oversight.
CK, JC, SF, GS, and SI contributed to the collection and review of any perspective or trial data, analysis, and authentication, and the writing and approval of this manuscript. The views expressed are those of the authors and not those of the U.S. federal government. Links or discussion of specific radiopharmaceutical drug products do not constitute endorsement.
The authors declare that the research was conducted in the absence of any commercial or financial relationships that could be construed as a potential conflict of interest.
CK, JC, SF, GS, and SI would like to acknowledge the Cancer Therapy Evaluation Program and Radiation Research Program of the Division of Cancer Treatment and Diagnosis, National Cancer Institute for supporting this work.
1. American Cancer Society. Cancer Facts and Figures 2018. Atlanta, GA: American Cancer Society (2018) p. 1–73.
2. American Cancer Society. Cancer Treatment and Survivorship Facts and Figures 2016-2017. Atlanta, GA: American Cancer Society. (2016) p. 1–42.
3. Ozols RF, Bundy BN, Greer BE, Fowler JM, Clarke-Pearson D, Burger RA, et al. Phase III trial of carboplatin and paclitaxel compared with cisplatin and paclitaxel in patients with optimally resected stage III ovarian cancer: a Gynecologic Oncology Group study. J Clin Oncol. (2003) 21:3194–200. doi: 10.1200/JCO.2003.02.153
4. Kunos CA, Capala J. National cancer institute programmatic collaboration for investigational radiopharmaceuticals. Am Soc Clin Oncol Educ Book. (2018) 23:488–94. doi: 10.1200/EDBK_200199
5. Young RC, Walton LA, Ellenberg SS, Homesley HD, Wilbanks GD, Decker DG, et al. Adjuvant therapy in stage I and stage II epithelial ovarian cancer. Results of two prospective randomized trials. N Engl J Med. (1990) 322:1021–7. doi: 10.1056/NEJM199004123221501
6. Young RC, Brady MF, Nieberg RK, Long HJ, Mayer AR, Lentz SS, et al. Adjuvant treatment for early ovarian cancer: a randomized phase III trial of intraperitoneal 32P or intravenous cyclophosphamide and cisplatin–a gynecologic oncology group study. J Clin Oncol. (2003) 21:4350–5. doi: 10.1200/JCO.2003.02.154
7. Vergote IB, Vergote-De Vos LN, Abeler VM, Aas M, Lindegaard MW, Kjørstad KE, et al. Randomized trial comparing cisplatin with radioactive phosphorus or whole-abdomen irradiation as adjuvant treatment of ovarian cancer. Cancer. (1992) 69:741–9.
8. Varia MA, Stehman FB, Bundy BN, Benda JA, Clarke-Pearson DL, Alvarez RD, et al. Intraperitoneal radioactive phosphorus (32P) versus observation after negative second-look laparotomy for stage III ovarian carcinoma: a randomized trial of the Gynecologic Oncology Group. J Clin Oncol. (2003) 21:2849–55. doi: 10.1200/JCO.2003.11.018
9. Parker C, Lewington V, Shore N, Kratochwil C, Levy M, Lindén O, et al. Targeted alpha therapy, an emerging class of cancer agents: a review. JAMA Oncol. (2018) 4:1765–72. doi: 10.1001/jamaoncol.2018.4044
10. Basch E, Reeve BB, Mitchell SA, Clauser SB, Minasian LM, Dueck AC, et al. Development of the National Cancer Institute's patient-reported outcomes version of the common terminology criteria for adverse events (PRO-CTCAE). J Natl Cancer Inst. (2014). 106:dju244. doi: 10.1093/jnci/dju244
11. Thanarajasingam G, Minasian LM, Baron F, Cavalli F, De Claro RA, Dueck AC, et al. Beyond maximum grade: modernising the assessment and reporting of adverse events in haematological malignancies. Lancet Haematol. (2018) 5:e563–98. doi: 10.1016/S2352-3026(18)30051-6
12. National Cancer Institute and Division of Cancer Treatment and Diagnosis. NCI Guidelines for Investigators: Adverse Event Reporting Requirements for DCTD (CTEP and CIP) and DCP INDs and IDEs Available online at: (https://ctep.cancer.gov/protocolDevelopment/electronic_applications/docs/aeguidelines.pdf). 2013 (accessed October 24, 2018).
13. Hagemann UB, Kristian A, Ellingsen C, Cruciani V, Wickstroem K, Mobergslien A, et al. Mesothelin-targeted thorium-227 conjugate (MSLN-TTC; BAY 2287411): preclinical evaluation of a new targeted alpha therapeutic in mesothelin-positive cancers. Proc Am Assoc Cancer Res. (2018). 59:216.
14. Food and Drug Administration Center for Drug Evaluation and Research (CDER) Oncology Therapeutic Radiopharmaceuticals: Nonclinical Studies and Labeling Recommendations Guidance for Industry. (2018). (accessed October 24, 2018).
15. Quanz M, Hagemann UB, Zitzmann-Kolbe S, Stelte-Ludwig B, Golfier S, Elbi C, et al. Anetumab ravtansine inhibits tumor growth and shows additive effect in combination with targeted agents and chemotherapy in mesothelin-expressing human ovarian cancer models. Oncotarget. (2018) 9:34103–21. doi: 10.18632/oncotarget.26135
16. Golfier S, Kopitz C, Kahnert A, Heisler I, Schatz CA, Stelte-Ludwig B, et al. Anetumab ravtansine: a novel mesothelin-targeting antibody-drug conjugate cures tumors with heterogeneous target expression favored by bystander effect. Mol Cancer Ther. (2014) 13:1537–48. doi: 10.1158/1535-7163.MCT-13-0926
17. Hassan R, Thomas A, Alewine C, Le DT, Jaffee EM, Pastan I, et al. Mesothelin immunotherapy for cancer: ready for prime time? J Clin Oncol. (2016) 34:4171–79. doi: 10.1200/JCO.2016.68.3672
18. Jirsova K, Neuwirth A, Kalasova S, Vesela V, Merjava S. Mesothelial proteins are expressed in the human cornea. Exp Eye Res. (2010). 91:623–9. doi: 10.1016/j.exer.2010.08.002
19. Chittenden SJ, Hindorf C, Parker CC, Lewington VJ, Pratt BE, Johnson B, et al. A Phase 1, open-label study of the biodistribution, pharmacokinetics, and dosimetry of 223Ra-dichloride in patients with hormone-refractory prostate cancer and skeletal metastases. J Nucl Med. (2015) 56:1304–9. doi: 10.2967/jnumed.115.157123
20. Nicolas GP, Mansi R, McDougall L, Kaufmann J, Bouterfa H, Wild D, et al. Biodistribution, pharmacokinetics, and dosimetry of (177)Lu-, (90)Y-, and (111)In-labeled somatostatin receptor antagonist OPS201 in comparison to the agonist (177)Lu-DOTATATE: the mass effect. J Nucl Med. (2017) 58:1435–41. doi: 10.2967/jnumed.117.191684
21. Bayer HealthCare. First-in-Human Study of BAY2287411 Injection, a Thorium-227 Labeled Antibody-chelator Conjugate, in Patients With Tumors Known to Express Mesothelin. (2018). Available online at: https://clinicaltrials.gov/ct2/show/NCT03507452
Keywords: radiopharmaceutical, radiation, radionuclides, nuclear medicine, ovarian cancer
Citation: Kunos CA, Capala J, Finnigan S, Smith GL and Ivy SP (2019) Radiopharmaceuticals for Relapsed or Refractory Ovarian Cancers. Front. Oncol. 9:180. doi: 10.3389/fonc.2019.00180
Received: 08 January 2019; Accepted: 04 March 2019;
Published: 26 March 2019.
Edited by:
Brian Timothy Collins, Georgetown University, United StatesReviewed by:
Michael Wayne Epperly, University of Pittsburgh, United StatesCopyright © 2019 Kunos, Capala, Finnigan, Smith and Ivy. This is an open-access article distributed under the terms of the Creative Commons Attribution License (CC BY). The use, distribution or reproduction in other forums is permitted, provided the original author(s) and the copyright owner(s) are credited and that the original publication in this journal is cited, in accordance with accepted academic practice. No use, distribution or reproduction is permitted which does not comply with these terms.
*Correspondence: Charles A. Kunos, Y2hhcmxlcy5rdW5vc0BuaWguZ292
Disclaimer: All claims expressed in this article are solely those of the authors and do not necessarily represent those of their affiliated organizations, or those of the publisher, the editors and the reviewers. Any product that may be evaluated in this article or claim that may be made by its manufacturer is not guaranteed or endorsed by the publisher.
Research integrity at Frontiers
Learn more about the work of our research integrity team to safeguard the quality of each article we publish.