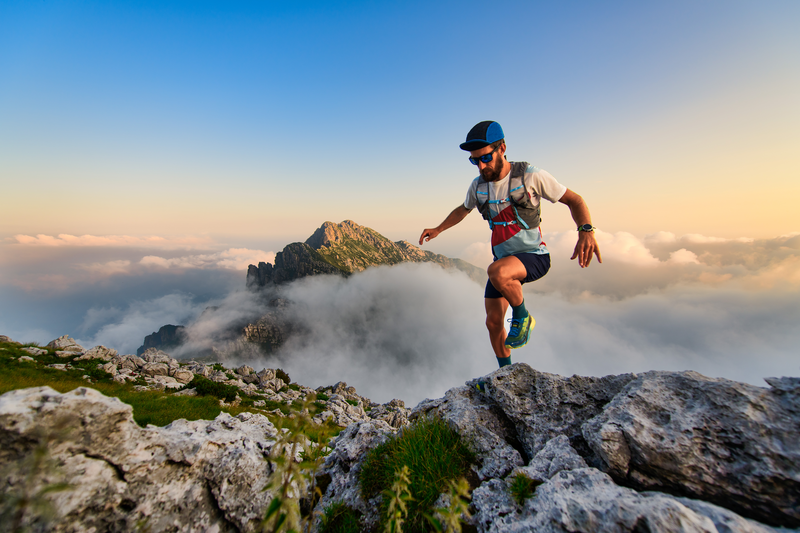
95% of researchers rate our articles as excellent or good
Learn more about the work of our research integrity team to safeguard the quality of each article we publish.
Find out more
PERSPECTIVE article
Front. Oncol. , 13 March 2018
Sec. Women's Cancer
Volume 8 - 2018 | https://doi.org/10.3389/fonc.2018.00068
This article is part of the Research Topic Targeting Phosphorylation and Glycosylation of Membrane-Bound Oncogenic Drivers, Receptors and Adhesion Molecules to Control Constitutive Proliferation of Human Carcinoma Cells View all 6 articles
Altered surface glycosylation is a key feature of cancers, including gynecologic malignancies. Hypersialylation, the overexpression of sialic acid, is known to promote tumor progression and to dampen antitumor responses by mechanisms that also involve sialic acid binding immunoglobulin-like lectins (Siglecs), inhibitory immune receptors. Here, we discuss the expression patterns of Siglecs and sialyltransferases (STs) in gynecologic cancers, including breast, ovarian, and uterine malignancies, based on evidence from The Cancer Genome Atlas. The balance between sialosides generated by specific STs within the tumor microenvironment and Siglecs on leukocytes may play a decisive role for antitumor immunity. An interdisciplinary effort is required to decipher the characteristics and biological impact of the altered tumor sialome in gynecologic cancers and to exploit this knowledge to the clinical benefit of patients.
Breast cancer and other gynecologic malignancies, involving the uterus and ovaries, are widely diagnosed tumor entities and, besides lung cancer, constitute the major cause of cancer-related death in women worldwide (1, 2). Although the development and implementation of novel strategies in the treatment of these diseases have positively influenced patient prognosis, novel and more personalized treatment approaches for the heterogeneous forms of gynecologic malignancies are urgently required (3–5). In the past decade, based on scientific evidence, immunotherapies rose to prominence as strong contenders in the fight against cancer in women (3, 6, 7). Different immunotherapeutic strategies are currently under evaluation, whereby off-target immunostimulatory effects of conventional chemotherapeutics may be synergistically embraced in combinatorial immune (chemo) therapeutic regimens (8). However, tumor-intrinsic and -extrinsic resistance factors account for heterogeneous treatment responses (9). The critical importance to decipher these immunosuppressive mechanisms is also illustrated by the unprecedented success of immune checkpoint blockade using antibodies to target immune regulatory checkpoints, such as the inhibitory receptors, CTLA-4 and PD-1 (10, 11). The elucidation of mechanisms that influence the host immune system, in particularly reference to specific gynecological cancers, may lead to novel diagnostic biomarkers and therapeutic strategies for these particular tumors.
Glycosylation changes are common in malignancies (12, 13), and several carbohydrate tumor markers are diagnostically exploited as biomarkers, such as the CA 125 antigen that is elevated in serum of patients with ovarian cancer (14). Distinct patterns of tumor surface glycosylation, in particular, hypersialylation, and the overexpression of sialic acids (15, 16), have been linked to immune escape and tumor progression (12). It has been suggested that sialic acid containing glycans (sialoglycans) may act as “self-associated molecular patterns (SAMPs)” (17) and that hypersialylation of tumors promotes escape from host immune responses by demonstrating “super-self” (18). The recognition of sialoglycan SAMPs by inhibitory receptors on the surface of immune cells, such as sialic acid binding immunoglobulin-like lectins (Siglecs) (19–21), may then lead to the downregulation of immune responses. Members of the Siglec family are heterogenously expressed in immune cells in a cell type- and differentiation-dependent manner, whereby different members recognize structurally distinct sialoglycans (19–21). As a further mechanism, hypersialylation might “mask” glycan ligands of other immunomodulatory receptors, if sialic acids are covalently linked by sialyltransferases (STs) to respective binding sites (12). For instance, the NKG2D-activating receptor on natural killer (NK) cells was shown to be involved in interactions with desialylated ligands on tumor cells (22). Interestingly, it appears that glycan epitopes with terminal sialic acids are less immunogenic and may escape humoral IgG responses (23). Cancer hypersialylation often involves the increased generation of sialoglycan ligands of selectins, such as sialyl-Lewis X and its structural isomer sialyl-Lewis A, which promotes metastatic spread by heterotypic interactions between cancer cells, leukocytes, and endothelial cells (12, 24, 25). In this context, high expression of sialyl-Lewis X in estrogen receptor (ER)-positive breast cancers was reported to correlate with metastasis to the bone, where sialyl-Lewis X receptor E-selectin is constitutively expressed (26). Interestingly, contrarily to this report, sialyl-Lewis X expression was shown to negatively correlate with progression in a breast cancer animal model (27). Many other mechanisms have been reported that contribute to immune escape and progression of hypersialylated tumors (12, 15, 28).
Hypersialylation in cancer has been linked to the enhanced expression and activity of STs (29, 30), which catalyze the covalent attachment of sialic acids via different glycosidic linkages (α2–3, α2–6, or α2–8) to subterminal carbohydrate moieties. Notably, high α2,3-sialyltransferase type I (ST3Gal I) expression is associated with advanced stage epithelial ovarian cancer and has been linked to ovarian cancer cell migration and peritoneal dissemination via an epidermal growth factor receptor-dependent mechanism (31). Figure 1 provides an overview on the expression of the 20 known human STs in gynecological cancers, including breast carcinoma (BRCA), ovarian serous cystadenocarcinoma (OV), uterine corpus endometrial carcinoma, and uterine carcinosarcoma (UCS), as well as non-gynecologic colonic adenocarcinoma (COAD) based on data from The Cancer Genome Atlas (TCGA) project. Broad, yet differential expression profiles of STs are found at tissue RNA levels across these tumor entities. In BRCA, ST3Gal I (mentioned above) exhibits highest RNA levels on average, followed by ST6Gal I, ST6GalNAc VI, ST6GalNAc II, and ST3Gal IV. Expression levels of these STs are similar in other tumors with the exception of ST6GalNAc II, which were lower in UCS and COAD. In BRCA, particularly low RNA levels are found for ST6GalNAc I, as opposed ST8Sia VI levels are higher. Compared to COAD, most gynecological tumors express at higher levels of ST6GalNAc II, ST8Sia II, and ST8Sia V, but lower levels of ST6GalNAc I.
Figure 1. Tissue RNA expression of sialyltransferases (STs) in gynecologic cancers. RNA tissue expression of known human STs in breast carcinoma (BRCA; n = 1,094), ovarian serous cystadenocarcinoma (n = 305), uterine corpus endometrial carcinoma (n = 545), uterine carcinosarcoma (n = 57), and colon adenocarcinoma (COAD; n = 455), ranked upon expression in BRCA. Data are expressed as box-and-whisker diagrams (median, lower, and upper quartiles; horizontal lines define minimum and maximum). The results shown here are in whole or part based upon data generated by the The Cancer Genome Atlas Research Network: http://cancergenome.nih.gov/. Figures were created in R version 3.4.2. **p < 0.01, ***p < 0.001, one-way ANOVA followed by Bonferroni’s post-test.
Although the exact expression of ST patterns and their consequences remain to be explored, the TCGA data suggest that common patterns of ST expression occur in gynecological tumors that may lead to universal tumor-associated carbohydrate antigens, e.g., sialyl-Tn antigen (14). On the other hand, ST expression differences may contribute to divergent tumor behavior, including immune escape or dissimilar responses to immunotherapeutic interventions. Depending on ST specificity and activity, responses of specific leukocyte subsets may be downregulated upon interaction with a hypersialylated tumor microenvironment, that harbors the cognate sialoside ligands, including specifically sialylated glycoproteins (e.g., mucins) or glycolipids (gangliosides) (12, 19, 24). A number of studies have shown that sialylated tumor cells exploit Siglec receptors to escape immune responses using the sialic acid–Siglec axis, including Siglec-7 or -9 on NK cells (32, 33), or Siglec-9 on myeloid cells (34, 35). The moderate (e.g., Siglec-7 or -9) or even high (e.g., Siglec-2 or -10) RNA expression in gynecological cancers as revealed by TCGA data (Figure 2), indicates the presence of leukocyte subsets that are potentially inhibited by tumor hypersialylation, as a consequence of increased ST expression and activity. Sialic acid–Siglec interactions may thus have important implications in terms of immune escape and for immunotherapeutic strategies in gynecological tumors.
Figure 2. Tissue RNA expression of sialic acid binding immunoglobulin-like lectins (Siglecs) in gynecologic cancers. RNA tissue expression of Siglecs in breast carcinoma, ovarian serous cystadenocarcinoma, uterine corpus endometrial carcinoma, uterine carcinosarcoma, and colonic adenocarcinoma, computed by a dendrogram clustering algorithm in R version 3.4.2. The results shown here are in whole or part based upon data generated by the The Cancer Genome Atlas Research Network: http://cancergenome.nih.gov/.
Although the knowledge concerning the dysregulation of the sialome and altered biosynthesis pathways is growing (16), the role of sialic acids in tumor development and immunity remains poorly understood. Yet, a better understanding of the impact of glycosylation changes in gynecological cancer has a high potential for the identification of diagnostic biomarkers and therapeutic targets. Glycan-based therapeutics may include specific sialyltransferase inhibitors (36), sialic acid mimetics (37), glycan-coated nanoparticles (19), glycan-modifying enzymes, as well as antibodies to glycans or their receptors (lectins) (12). The potential gain for patients with gynecologic cancers is high, but so are the challenges. The latter not only demand increased interdisciplinary efforts between clinicians and scientists, but also the improved training of glycoscientists (38) and the enhanced awareness of the biological implications of altered glycosylation on tumor biology and immunity.
SG and QH designed the study. QH, CS, and SG analyzed the data. Computational analysis of the data set was performed by QH. All authors had full access to the data, helped to draft the report or critically revised the draft, contributed to data interpretation, and reviewed and approved the final version of the report.
The authors declare that the research was conducted in the absence of any commercial or financial relationships that could be construed as a potential conflict of interest.
The authors thank The Cancer Genome Atlas Research Network. This work was supported in part by the Swiss National Science Foundation (grants 310030_162552/1) and Swiss Cancer League/Swiss Cancer Research grants KFS-3941-08-2016 and KFS-3248-08-2013 to SG.
1. Faubion SS, Maclaughlin KL, Long ME, Pruthi S, Casey PM. Surveillance and care of the gynecologic cancer survivor. J Womens Health (2015) 24(11):899–906. doi:10.1089/jwh.2014.5127
2. Allemani C, Weir HK, Carreira H, Harewood R, Spika D, Wang X-S, et al. Global surveillance of cancer survival 1995–2009: analysis of individual data for 25 676 887 patients from 279 population-based registries in 67 countries (CONCORD-2). Lancet (2015) 385(9972):977–1010. doi:10.1016/S0140-6736(14)62038-9
3. Bourla AB, Zamarin D. Immunotherapy: new strategies for the treatment of gynecologic malignancies. Oncology (Williston Park) (2016) 30(1):59–66, 69.
4. de la Mare J-A, Contu L, Hunter MC, Moyo B, Sterrenberg JN, Dhanani KCH, et al. Breast cancer: current developments in molecular approaches to diagnosis and treatment. Recent Pat Anticancer Drug Discov (2014) 9(2):153–75. doi:10.2174/15748928113086660046
5. Liu J, Matulonis UA. New strategies in ovarian cancer: translating the molecular complexity of ovarian cancer into treatment advances. Clin Cancer Res (2014) 20:5150–6. doi:10.1158/1078-0432.CCR-14-1312
6. Vanderstraeten A, Luyten C, Verbist G, Tuyaerts S, Amant F. Mapping the immunosuppressive environment in uterine tumors: implications for immunotherapy. Cancer Immunol Immunother (2014) 63:545–57. doi:10.1007/s00262-014-1537-8
7. Law AMK, Lim E, Ormandy CJ, Gallego-Ortega D. The innate and adaptive infiltrating immune systems as targets for breast cancer immunotherapy. Endocr Relat Cancer (2017) 24(4):R123–44. doi:10.1530/ERC-16-0404
8. Galluzzi L, Vacchelli E, Bravo-San Pedro J-M, Buqué A, Senovilla L, Baracco EE, et al. Classification of current anticancer immunotherapies. Oncotarget (2014) 5:12472–508. doi:10.18632/oncotarget.2998
9. Pitt JM, Vétizou M, Daillère R, Roberti MP, Yamazaki T, Routy B, et al. Resistance mechanisms to immune-checkpoint blockade in cancer: tumor-intrinsic and -extrinsic factors. Immunity (2016) 44:1255–69. doi:10.1016/J.IMMUNI.2016.06.001
10. Sharma P, Allison JP. Immune checkpoint targeting in cancer therapy: toward combination strategies with curative potential. Cell (2015) 161:205–14. doi:10.1016/j.cell.2015.03.030
11. Xu-Monette ZY, Zhang M, Li J, Young KH. PD-1/PD-L1 blockade: have we found the key to unleash the antitumor immune response? Front Immunol (2017) 8:1597. doi:10.3389/fimmu.2017.01597
12. Boligan KF, Mesa C, Fernandez LE, Von Gunten S. Cancer intelligence acquired (CIA): tumor glycosylation and sialylation codes dismantling antitumor defense. Cell Mol Life Sci (2015) 72:1231–48. doi:10.1007/s00018-014-1799-5
13. Pinho SS, Reis CA. Glycosylation in cancer: mechanisms and clinical implications. Nat Rev Cancer (2015) 15:540–55. doi:10.1038/nrc3982
14. Stowell SR, Ju T, Cummings RD. Protein glycosylation in cancer. Annu Rev Pathol (2015) 10:473–510. doi:10.1146/annurev-pathol-012414-040438
15. Büll C, Stoel MA, Den Brok MH, Adema GJ. Sialic acids sweeten a tumor’s life. Cancer Res (2014) 74(12):3199–204. doi:10.1158/0008-5472.CAN-14-0728
16. Cohen M, Varki A. The sialome—far more than the sum of its parts. OMICS (2010) 14(4):455–64. doi:10.1089/omi.2009.0148
17. Varki A. Letter to the glyco-forum: since there are PAMPs and DAMPs, there must be SAMPs? Glycan “self-associated molecular patterns” dampen innate immunity, but pathogens can mimic them. Glycobiology (2011) 21:1121–4. doi:10.1093/glycob/cwr087
18. Fomby P, Cherlin AJ. Glyco-engineering “Super-self.”. Nat Chem Biol (2011) 72:181–204. doi:10.1038/nature13314.A
19. Macauley MS, Crocker PR, Paulson JC. Siglec-mediated regulation of immune cell function in disease. Nat Rev Immunol (2014) 14:653–66. doi:10.1038/nri3737
20. Adams OJ, Stanczak MA, von Gunten S, Läubli H. Targeting sialic acid–Siglec interactions to reverse immune suppression in cancer. Glycobiology (2017). doi:10.1093/glycob/cwx108
21. Jandus C, Simon H-U, von Gunten S. Targeting Siglecs—a novel pharmacological strategy for immuno- and glycotherapy. Biochem Pharmacol (2011) 82:323–32. doi:10.1016/J.BCP.2011.05.018
22. Cohen M, Elkabets M, Perlmutter M, Porgador A, Voronov E, Apte RN, et al. Sialylation of 3-methylcholanthrene-induced fibrosarcoma determines antitumor immune responses during immunoediting. J Immunol (2010) 185:5869–78. doi:10.4049/jimmunol.1001635
23. Schneider C, Smith DF, Cummings RD, Boligan KF, Hamilton RG, Bochner BS, et al. The human IgG anti-carbohydrate repertoire exhibits a universal architecture and contains specificity for microbial attachment sites. Sci Transl Med (2015) 7:269ra1. doi:10.1126/scitranslmed.3010524
24. Natoni A, Macauley MS, O’Dwyer ME. Targeting selectins and their ligands in cancer. Front Oncol (2016) 6:93. doi:10.3389/fonc.2016.00093
25. Läubli H, Borsig L. Selectins promote tumor metastasis. Semin Cancer Biol (2010) 20:169–77. doi:10.1016/j.semcancer.2010.04.005
26. Julien S, Ivetic A, Grigoriadis A, QiZe D, Burford B, Sproviero D, et al. Selectin ligand sialyl-Lewis x antigen drives metastasis of hormone-dependent breast cancers. Cancer Res (2011) 71:7683–93. doi:10.1158/0008-5472.CAN-11-1139
27. Monzavi-Karbassi B, Whitehead TL, Jousheghany F, Artaud C, Hennings L, Shaaf S, et al. Deficiency in surface expression of E-selectin ligand promotes lung colonization in a mouse model of breast cancer. Int J Cancer (2005) 117(3):398–408. doi:10.1002/ijc.21192
28. Pearce OMT, Läubli H. Sialic acids in cancer biology and immunity. Glycobiology (2016) 26(2):111–28. doi:10.1093/glycob/cwv097
29. Ito H, Hiraiwa N, Sawada-Kasugai M, Akamatsu S, Tachikawa T, Kasai Y, et al. Altered mRNA expression of specific molecular species of fucosyl- and sialyl-transferases in human colorectal cancer tissues. Int J Cancer (1997) 71:556–64. doi:10.1002/(SICI)1097-0215(19970516)71:4<556::AID-IJC9>3.0.CO;2-T
30. Swindall AF, Londoño-Joshi AI, Schultz MJ, Fineberg N, Buchsbaum DJ, Bellis SL. ST6Gal-I protein expression is upregulated in human epithelial tumors and correlates with stem cell markers in normal tissues and colon cancer cell lines. Cancer Res (2013) 73:2368–78. doi:10.1158/0008-5472.CAN-12-3424
31. Wen K-C, Sung P-L, Hsieh S-L, Chou Y-T, Lee OK-S, Wu C-W, et al. α2,3-sialyltransferase type I regulates migration and peritoneal dissemination of ovarian cancer cells. Oncotarget (2017) 8:29013–27. doi:10.18632/oncotarget.15994
32. Jandus C, Boligan KF, Chijioke O, Liu H, Dahlhaus M, Démoulins T, et al. Interactions between Siglec-7/9 receptors and ligands influence NK cell-dependent tumor immunosurveillance. J Clin Invest (2014) 124:1810–20. doi:10.1172/JCI65899DS1
33. Hudak JE, Canham SM, Bertozzi CR. Glycocalyx engineering reveals a Siglec-based mechanism for NK cell immunoevasion. Nat Chem Biol (2014) 10:69–75. doi:10.1038/nchembio.1388
34. Läubli H, Pearce OMT, Schwarz F, Siddiqui SS, Deng L, Stanczak MA, et al. Engagement of myelomonocytic Siglecs by tumor-associated ligands modulates the innate immune response to cancer. Proc Natl Acad Sci USA (2014) 111:14211–6. doi:10.1073/pnas.1409580111
35. Beatson R, Tajadura-Ortega V, Achkova D, Picco G, Tsourouktsoglou T-D, Klausing S, et al. The mucin MUC1 modulates the tumor immunological microenvironment through engagement of the lectin Siglec-9. Nat Immunol (2016) 17:1273–81. doi:10.1038/ni.3552
36. Szabo R, Skropeta D. Advancement of sialyltransferase inhibitors: therapeutic challenges and opportunities. Med Res Rev (2017) 37:219–70. doi:10.1002/med.21407
37. Büll C, Heise T, Adema GJ, Boltje TJ. Sialic acid mimetics to target the sialic acid–Siglec axis. Trends Biochem Sci (2016) 41:519–31. doi:10.1016/j.tibs.2016.03.007
Keywords: gynecologic malignancies, sialyltransferases, sialic acid binding immunoglobulin-like lectins, The Cancer Genome Atlas, cancer immunotherapy
Citation: Haas Q, Simillion C and von Gunten S (2018) A Cartography of Siglecs and Sialyltransferases in Gynecologic Malignancies: Is There a Road Towards a Sweet Future? Front. Oncol. 8:68. doi: 10.3389/fonc.2018.00068
Received: 02 February 2018; Accepted: 01 March 2018;
Published: 13 March 2018
Edited by:
Viive Maarika Howell, University of Sydney, AustraliaReviewed by:
Behjatolah Monzavi-Karbassi, University of Arkansas for Medical Sciences, United StatesCopyright: © 2018 Haas, Simillion and von Gunten. This is an open-access article distributed under the terms of the Creative Commons Attribution License (CC BY). The use, distribution or reproduction in other forums is permitted, provided the original author(s) and the copyright owner are credited and that the original publication in this journal is cited, in accordance with accepted academic practice. No use, distribution or reproduction is permitted which does not comply with these terms.
*Correspondence: Stephan von Gunten, c3RlcGhhbi52b25ndW50ZW5AcGtpLnVuaWJlLmNo
Disclaimer: All claims expressed in this article are solely those of the authors and do not necessarily represent those of their affiliated organizations, or those of the publisher, the editors and the reviewers. Any product that may be evaluated in this article or claim that may be made by its manufacturer is not guaranteed or endorsed by the publisher.
Research integrity at Frontiers
Learn more about the work of our research integrity team to safeguard the quality of each article we publish.