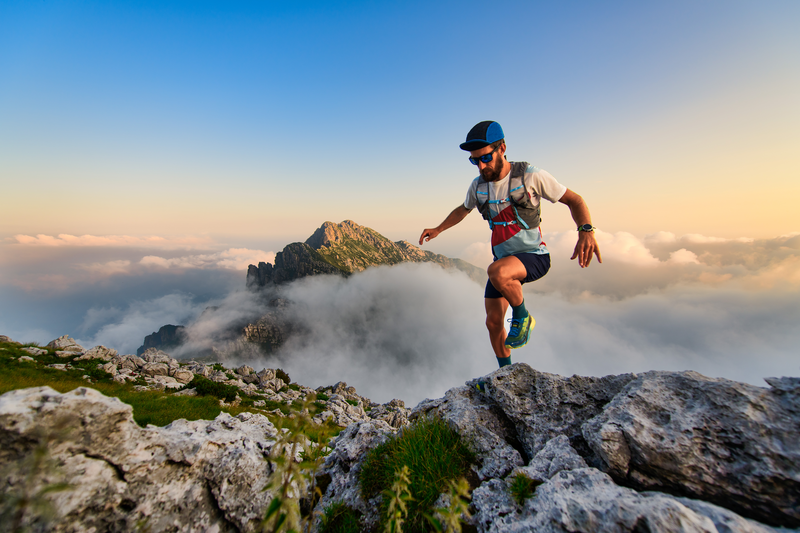
95% of researchers rate our articles as excellent or good
Learn more about the work of our research integrity team to safeguard the quality of each article we publish.
Find out more
REVIEW article
Front. Oncol. , 30 October 2017
Sec. Genitourinary Oncology
Volume 7 - 2017 | https://doi.org/10.3389/fonc.2017.00256
This article is part of the Research Topic Updates, Emerging Methodologies, and Tools for Prostate Cancer Precision Medicine View all 7 articles
Background: Active surveillance (AS) is a widely adopted strategy to monitor men with low-risk, localized prostate cancer (PCa). Current AS inclusion criteria may misclassify as many as one in four patients. The advent of multiparametric magnetic resonance imaging (mpMRI) and novel PCa biomarkers may offer improved risk stratification. We performed a review of recently published literature to characterize emerging evidence in support of these novel modalities.
Methods: An English literature search was conducted on PubMed for available original investigations on localized PCa, AS, imaging, and biomarkers published within the past 3 years. Our Boolean criteria included the following terms: PCa, AS, imaging, biomarker, genetic, genomic, prospective, retrospective, and comparative. The bibliographies and diagnostic modalities of the identified studies were used to expand our search.
Results: Our review identified 222 original studies. Our expanded search yielded 244 studies. Among these, 70 met our inclusion criteria. Evidence suggests mpMRI offers improved detection of clinically significant PCa, and MRI-fusion technology enhances the sensitivity of surveillance biopsies. Multiple studies demonstrate the promise of commercially available screening assays for prediction of AS failure, and several novel biomarkers show promise in this setting.
Conclusion: In the era of AS for men with low-risk PCa, improved strategies for proper stratification are needed. mpMRI has dramatically enhanced the detection of clinically significant PCa. The advent of novel biomarkers for prediction of aggressive disease and AS failure has shown some initial promise, but further validation is warranted.
In 2017, there will be an estimated 161,000 new cases of prostate cancer (PCa) in the United States, representing approximately 20% of new cancer diagnoses and the third leading cause of death in men (1). Since the adoption of prostate specific antigen (PSA) screening in the 1990s, the incidence of PCa has significantly increased, while age-adjusted cancer-specific mortality has declined nearly 45% (2). This is largely attributed to the increased detection of localized, low-risk disease, and immediate intervention in these patients may not be necessary (2, 3). Furthermore, early intervention exposes patients to the potential side effects of medications, radiation and surgery, and may cause undue damage to quality of life (4). Since cancer-specific survival in this subset of patients is very high, even with conservative management, a less-invasive management approach may be more appropriate (5).
Close monitoring by active surveillance (AS), which includes serial PSAs, digital rectal exams and transrectal ultrasound (TRUS) biopsies, and more recently, MRI, has become a widely adopted strategy to monitor indolent disease (3–5). About 50% of men on AS will eventually require treatment (6). Unfortunately, the decision to place a patient on AS, as well as determining when surveillance may no longer be appropriate, remains controversial due to limitations in both risk stratification and disease monitoring (7, 8). In fact, current AS inclusion criteria may misclassify as many as one in four patients (9, 10). Moreover, studies of AS-eligible men who opted for early radical prostatectomy were found to have significant cancer in up to 20% of cases (7, 11). Therefore, optimization of diagnostic and surveillance strategies are necessary to minimize patient risk.
Over the past several years, the use of multiparametric MRI has increased dramatically for the initial diagnosis and monitoring of PCa (12, 13). In addition, the emergence of novel PCa biomarkers are increasingly available for clinical use (14). However, most well-established AS protocols have yet to formally incorporate these diagnostic modalities. We performed a review of recently published literature to characterize the emerging evidence in support of advanced imaging and genetic and epigenetic screening techniques for monitoring patients on AS for localized PCa.
An English literature search was conducted on PubMed for available original investigations on localized PCa, AS, imaging and biomarkers. All articles published within the past few years (January 1, 2014 to August 4, 2017) were considered. Our Boolean criteria included the following terms: PCa, AS, imaging, biomarker, genetic, genomic, prospective, retrospective, and comparative. Our search excluded publication types such as comments, editorials, guidelines, reviews, or interviews. The bibliographies and diagnostic modalities of the identified studies were used to expand our search.
Our review identified 222 original studies. Our expanded search yielded 244 studies. Among these, 70 publications met our inclusion criteria. A total of 44 studies assessed the role for updated imaging practices such as multiparametric magnetic resonance imaging (mpMRI), and 26 evaluated the reliability of novel biomarkers in AS cohorts (Figure 1) (15).
In recent years, mpMRI has emerged as a reliable tool for monitoring patients on AS (13, 16). The implementation of novel MRI analytic techniques such as diffusion-weighted imaging (DWI) and volumetric estimation algorithms have allowed for improved tumor characterization (17–19). More specifically, the apparent diffusion coefficient, which is calculated from DWI sequences, inversely correlates with PCa aggressiveness (18). Furthermore, automated calculations of lesion volume on mpMRI reliably correspond with PCa presence (18, 20). Marin et al. demonstrated that the use of semi-automated sizing algorithms to measure maximal tumor dimensions of suspicious lesions reliably correlated with actual tumor histologic diameter, and this practice should be considered during routine workup (20).
Several studies suggest using mpMRI in the initial diagnostic setting may classify patients as AS-eligible more reliably than traditional protocol criteria alone (21–23). In a study by Ouzzane et al., 10% of patients initially classified as appropriate for AS were reclassified as inappropriate following the detection of clinically silent lesions on mpMRI followed by targeted biopsy (22). When reviewed by an experienced radiologist, Porpiglia et al. suggests use of mpMRI alone may reliably predict pathologically significant PCa without confirmatory tissue biopsy (21). However, in a retrospective study of 118 patients, Dianat et al. found significant pathology could exist in the absence of a visible index lesion on MRI (24).
Over the past 10 years, the PIRADS has become an increasingly useful tool in both the initial diagnostic setting and for monitoring patients on AS (25–27). The PIRADS system is a five-point Likert scale applied to a suspicious lesion on MRI. An overall score is assigned to predict the likelihood that a suspicious lesion harbors clinically significant PCa (28, 29). In a retrospective analysis of more than 1,000 patients on AS, Venderink et al. demonstrated that a PIRADS cutoff of ≥3 could more reliably select patients for repeat biopsy than PSA density (PSAD) (26). In a study of 201 patients undergoing transperineal sector prostate biopsy, Grey et al. demonstrated that a strict cutoff of PI-RADS ≥3 would have allowed 44% of men to avoid undergoing repeat biopsy (25). However, 2.3% of those men still harbored significant PCa, raising the question as to what degree of specificity is necessary for proper management of patients on AS with presumably slow-growing disease (25). We identified three original investigations that compared a widely accepted AS protocol with a predictive nomogram incorporating PIRADS, and all three studies demonstrated a significant improvement in risk stratification (30–32).
More recently, a proposed PIRADS version 2 (PIRADSv2) was developed to reflect increasingly complex MRI interpretation with the application of multiple sequences such as DWI and dynamic contrast enhancement for interpretation of a single lesion (27, 29, 33). Validation studies comparing its utility to the original PIRADS algorithm are currently underway. However, early results indicate significant PCa may be overlooked as often as 5% of the time when using PIRADSv2 ≥3 as a cutoff (27). Furthermore, there is concern that the PIRADS algorithm may inaccurately characterize benign central zone (CZ) lesions. In a retrospective review of 73 patients who underwent MRI-fusion biopsy by Tan et al., 26 CZ lesions were graded as PIRADS 3 or greater. Only two (7.7%) of these suspicious lesions harbored PCa (34). Since evidence suggests PCa arising from the CZ can be more aggressive than peripheral and transitional zone cancers, modification of the PIRADS algorithm may be needed to better characterize CZ lesions (35, 36).
With mpMRI becoming an increasingly reliable predictor of histologic tumor burden, MRI–US fusion technology has been developed to perform targeted biopsy of suspicious prostatic lesions (37, 38). Several recently published investigations demonstrate performing targeted biopsies on men undergoing AS is superior to standard TRUS biopsy for the detection of newly significant PCa (39–42). Furthermore, Siddiqui et al. suggest performing MRI–US fusion targeted biopsy may reduce the number of insignificant PCa diagnoses, thus sparing patients from unnecessary biopsies (43).
However, there is conflicting data whether it is appropriate to only use MRI–US fusion biopsy, thus abandoning initial random TRUS guided sampling, for the detection of Gleason score (GS) upgrades in AS patients (39–41, 44). For example, Da Rosa et al. reported a 100% negative predictive value for detecting GS 6 to 7 upgrade when using MRI–US fusion in their cohort of 72 men on AS (40). Furthermore, a retrospective study by Nassiri et al. of more than 250 patients showed that 32 of 33 upgraded cases were from MRI-guided cores (41). In contrast, a study by Marliere et al., albeit with a smaller cohort, demonstrated both standard TRUS and targeted MRI–US fusion biopsies in the initial AS setting may still be necessary for adequate detection of all new GS upgrades (44).
Compared to standard TRUS sampling and MRI–US fusion targeted biopsy, evidence demonstrates saturation biopsy (24 or 30 core templated sampling) provides the greatest sensitivity for detection of significant PCa in the initial AS period (45–47). While this approach may provide enhanced detection, it also subjects patients to the burden of increased tissue acquisition. Pepe et al. proposed using a hybrid approach they referred to as “cognitive zonal fusion biopsy” (46). In this approach, all patients undergo mpMRI prior to a MRI–US fusion biopsy. If during the biopsy there is a discrepancy between what was found on MRI and what is visualized on US, two to six cores are obtained from the MRI region of interest. In their prospective study of 58 individuals who were either biopsy naïve or on AS, this approach reliably detected significant PCa (46).
There is mounting evidence that men on AS who underwent proper initial evaluation may be monitored with serial mpMRIs, and the interval for repeat biopsies may be lengthened (8, 48–52). In two separate studies of men on AS who underwent serial mpMRIs and MRI–US fusion biopsy, both Walton Diaz et al. and Felker et al. observed that stable findings on mpMRI significantly correlated with GS stability (48, 50). In a 2-year retrospective review of 162 men on AS, Frye et al. demonstrated that progression on repeat mpMRI significantly predicted pathological progression (8).
For men with small index lesions (≤7 mm), a retrospective review of more than 150 patients on AS demonstrated no change in either lesion size or pathologic characteristics during a 2-year surveillance period (51). This suggests that men on AS, whose lesions meet this size criteria, could potentially forgo any surveillance whatsoever for intervals up to 2 years. Siddiqui et al. developed a predictive nomogram based on serial mpMRI results for men on AS who underwent repeat MRI–US fusion biopsies over the course of 5 years. Based on whether targeted biopsy prompted disqualification from AS, their nomogram incorporating mpMRI could have potentially avoided repeat tissue biopsy sessions in up to 68% of men (49).
Recent advances in genomic sequencing and molecular classification led to development of a plethora of assays for PCa diagnosis. Unquestionably, serum PSA is the most frequently used biomarker for detecting and monitoring PCa. However, there are several well-documented limitations in its reliability for predicting disease presence (14). Benign prostatic diseases, DRE, urologic instrumentation, and recent ejaculation may all cause the serum PSA to become elevated in absence of PCa (14, 53). Therefore, considerable effort has been given to identify novel tissue, serum, and urine-based biomarkers to better stratify at-risk patients.
Kallikrein-related enzymes are a family of serine proteases with high homology (54). While the kallikrein-3 gene (KLK-3, or PSA) is among the most extensively investigated in relation to PCa, expression of all 15 kallikreins can be detected in prostate tissue (55). Recently a predictive tool comprising plasma total PSA, free PSA, intact PSA, and kallikrein-2 along with patient age, DRE and biopsy history known as the 4Kscore Test has gained attention as a novel risk stratification tool for patients with elevated PSA, and possibly newly diagnosed PCa (55–57). In a prospective study by the Canary Prostate Active Surveillance Study Investigators, which evaluated more than 700 men with newly diagnosed Gleason 6 PCa on AS, the 4Kscore reliably predicted GS upgrades on the first surveillance biopsy (57). However, there was a decreased utility in the 4Kscore Test for predicting GS upgrades on subsequent biopsies, suggesting it may only be beneficial in the initial AS period (57).
A novel urinary assay-based risk score called SelectMDx by MDxHealth combines serum PSA, PSAD and clinical factors such as age and prior negative biopsy with two mRNA signatures: urinary homeobox C6 and distal-less homeobox 1 (58). This relatively low-cost assay was recently implicated as a useful diagnostic aid for appropriately selecting men for undergoing prostate biopsy (58). In the setting of a prior negative biopsy, MDxHealth developed an additional assay called ConfirmMDx (59, 60). The ConfirmMDx assay utilizes methylation analyses of various oncogenes such as Ras and Adenomatous Polyposis Coli from a patient’s prior negative biopsy tissue to estimate the likelihood of obtaining a negative repeat biopsy (59). In a multicenter study of 350 subjects, Partin et al. reported an 88% negative predictive value of detecting significant PCa at 13-month follow-up. Furthermore, their multivariate model suggested ConfirmMDx may independently predict repeat negative biopsy in this setting (60).
The Prolaris test evaluates the RNA expression of 46 genes to evaluate tumor cell growth characteristics and may help risk stratify patients with PCa (61–63). The assay measures the cell cycle progression score, and has proven prognostic value in other malignancies such as breast cancer (64). Several recent investigations revealed performing the Prolaris test in the initial diagnostic setting may reliably risk stratify patients for AS, and potentially avoid unnecessary repeat biopsies for those with low risk Prolaris scores (61, 65).
The Oncotype Dx panel is a 17-gene reverse transcription polymerase chain reaction-based diagnostic assay that has been validated as a predictor of adverse pathology following prostatectomy in men with low-risk PCa (66–69). Eure et al. prospectively studied 297 patients on AS, and those who underwent Oncotype Dx screening were more likely to remain on AS (66). The authors suggest the predictive value of Oncotype Dx may provide both patients and clinicians with better peace of mind when opting to remain on AS given additional reassurance that AS is safe given a indolent genetic profile of disease.
The Decipher gene expression assay uses 22 genes to predict the risk of developing metastases following radical prostatectomy (70, 71). While there is evidence supporting its utility in the postoperative setting, we could not identify any studies evaluating the potential benefit of using the Decipher assay for AS patients undergoing conservative management.
Metamark Genetics Inc. developed a quantitative protein-based multiplex imaging platform designated ProMark as prognostic test for PCa. ProMark’s quantitative immunofluorescence method utilizes imaging platform and measure eight biomarkers directly on sections of biopsy tissue (72). Multiple studies have tested the utility of the ProMark assay in predicting biochemical recurrence after radical prostatectomy (73, 74).
The phosphatase and tensin homolog (PTEN) is a tumor suppressor gene whose function may be lost in patients with PCa (75). As such, measuring PTEN to predict PCa severity has gained attention as a possible way to stratify patients on AS (76, 77). In a retrospective study using tissue microarray specimens of over 600 patients who underwent radical prostatectomy, loss of PTEN was significantly correlated with PCa severity (76). Lokman et al. recently demonstrated that loss of PTEN on pathological analysis of surveillance targeted biopsies may predict future GS upgrading and AS discontinuation (76).
Majority of PCa show recurrent chromosomal rearrangement which leads predominantly to the fusion of the androgen-responsive promoter elements of the TMPRSS2 gene with ETS transcription factor ERG. This gene fusion leads to overexpression of ERG in PCa (78). In a study of 265 patients on AS, Berg et al. demonstrated ERG positivity was significantly associated with progression of disease (79). Furthermore, a separate study by Berg et al. showed ERG expression remains consistent from initial biopsy through surgical removal of the prostate (80). This temporal stability suggests clinicians could obtain ERG expression just one time at any point in a patient’s disease course, thus limiting the costs of re-sampling.
The PHI is a formula that incorporates the following PSA isoforms: total PSA, free PSA, and pro-PSA (81). PCA3 is a gene that expresses noncoding RNA which is extracted from the prostate following DRE (81, 82). Both assays have gained attention as potential tools for AS patient selection and monitoring (81–84). Recently, Cantiello et al. demonstrated both PHI and PCA3 may aid in the prediction pathologically insignificant PCa (84). However, this was a retrospective observational study, and few if any studies have investigated the predictive value of these biomarkers in a prospective clinical setting (81, 84).
The α-chemokine receptor, C–X–C chemokine receptor type 4 (CXCR4) and its ligand, stromal-derived-factor 1 (also known as CXCL12) are therapeutic targets in various epithelial, mesenchymal, and hematopoietic tumors (85, 86). Recent evidence suggests the CXCR4/CXCL12 interaction may be involved in PCa tumorigenesis (87, 88). In a retrospective review of patients who underwent radical prostatectomy, Goltz et al. demonstrated that aberrant CXCL12 methylation was correlated with GS and biochemical recurrence (89). They postulated that evaluating CXCL12 in the AS setting may be beneficial, but we failed to identify any studies to date that have evaluated this theory.
The detection of malignant circulating prostate cells (mCPCs) has gained attention as a novel, minimally invasive way to detect PCa status (90–92). By using immunocytochemistry and anti-PSA monoclonal antibodies, detection of plasma mCPCs may reliably identify low vs high risk PCa (92). In a retrospective study of 161 men on AS, Murray et al. demonstrated mCPC detection was superior to both the Chun nomogram and serum-free PSA for predicting the detection of clinically significant PCa (91). Using mCPC presence alone resulted in only two (<1%) missed PCa diagnoses, and both were low-grade disease.
Genome-wide association studies have implicated numerous SNPs in PCa development (93, 94). There is considerable interest in identifying SNPs associated with high risk PCa to aid in identifying patients eligible for AS (95). Recently, Kearns et al. identified a SNP associated with GS upgrading in a cohort of more than 200 patients on AS (96). Their findings warrant further investigation of the predictive insight SNPs may provide.
Active surveillance is a widely adopted strategy to monitor patients with low-risk, localized PCa, and offers both cost and quality of life benefits to patients meeting surveillance criteria. Optimally monitoring men on AS remains a challenge for clinicians due to disease variability, the lack of an overall consensus on optimal management, and the abundance of readily available diagnostic tests. A majority of AS protocols use data obtained from DREs, PSAs, and TRUS biopsies to stratify patients (3–5). Since up to 50% of all patients on AS will eventually require treatment of their cancer, and evidence suggests that some men are inappropriately placed on surveillance, improved diagnostic modalities are needed (6–11). The advent of improved MRI techniques and our expanding knowledge of genetic alterations driving PCa tumorigenesis have facilitated the development of novel diagnostic strategies for monitoring PCa.
Recent evidence suggests using mpMRI to characterize suspicious lesions is superior to currently accepted strategies, and doing so may avoid unnecessary repeat biopsies in a significant number of men (17–19, 21–23). Moreover, when used in the initial diagnostic setting, mpMRI, may actually identify more aggressive disease not found on the initial biopsy and, therefore, may keep the patient from being inappropriately placed on AS (22). Recently, the European School of Oncology formed the PCa Radiological Estimation of Change in Sequential Evaluation (PRECISE) panel to develop a consensus on mpMRI data reporting for researchers investigating cohorts of men on AS. While validation of the panel’s initial recommendations is needed, this may aid in future optimization of currently used diagnostic nomograms (52).
When prostate biopsy is indicated, the integration of MRI technology with TRUS biopsy via MRI–US fusion technology enhances the ability to sample concerning lesions with pinpoint accuracy (39–42). Recent evidence suggests that performing MRI-targeted biopsies may be just as reliable as saturation biopsies for detection of significant PCa (45–47). This would spare both patients and providers the burden of acquiring unnecessary cores, and may lessen the frequency of detecting insignificant disease (46). However, some studies suggest MRI–US fusion alone may not be adequate in the initial diagnostic setting, and standard template biopsy may be equally diagnostic in biopsy naïve men (44).
While PSA is the most commonly used serum biomarker to detect and monitor PCa, many novel genetic and epigenetic markers of PCa disease status are under investigation. Several commercially available screening panels exist, and have promise in risk stratification of men newly diagnosed with PCa (55–57, 61, 65–70). Among these, our review found some evidence supporting the use of the 4K Score, SelectMDx, ConfirmMDx Prolaris, and Oncotype Dx in both the initial diagnostic and AS milieus (57, 58, 60, 61, 65, 66).
Novel biomarkers such as PTEN, ERG, PCA-3, CXCL12 and utilization of various PSA isoforms are gaining attention as potential indicators of PCa disease (76, 79, 84, 89). Indirectly assessing PCa status in the form of both mCPC and SNP detection has also been proposed for patients in the AS setting (91, 96). While these candidate markers show promise, they are by and large still in their nascent stage of development, and further validation in a diverse prospective setting is warranted. Furthermore, the potential logistical and financial burden of performing large prospective studies using these advanced diagnostic panels should not be overlooked.
In the era of AS for men with low-risk PCa, improved strategies for proper stratification are needed to balance overtreatment with under diagnosis. mpMRI has dramatically enhanced the detection of clinically significant PCa, and may permit less-invasive surveillance strategies compared to currently accepted protocols. The advent of novel biomarkers for prediction of aggressive disease and AS failure has shown some initial promise, but further validation is warranted.
ZG: data acquisition, data processing, and drafting and critical review of manuscript. JG: data processing, and drafting and critical review of manuscript. KP: data processing, and drafting and critical review of manuscript. SV: data processing, drafting and critical review of manuscript. SR-B: idea development, data acquisition, data processing, drafting and critical review of manuscript.
The authors declare that the research was conducted in the absence of any commercial or financial relationships that could be construed as a potential conflict of interest.
ADC, apparent diffusion coefficient; AS, active surveillance; CCP, cell cycle progression score; CZ, central zone; DWI, diffusion-weighted imaging; GS, Gleason score; mpMRI, multi parametric magnetic resonance imaging; MRI, magnetic resonance imaging; PCa, prostate cancer; PHI, prostate health index; PIRADS, Prostate Imaging Reporting and Data System; PSA, prostate specific antigen; PSAD, PSA density; TRUS, transrectal ultrasound.
1. Siegel RL, Miller KD, Jemal A. Cancer statistics, 2017. CA Cancer J Clin (2017) 67:7–30. doi:10.3322/caac.21387
2. Etzioni R, Gulati R, Cooperberg MR, Penson DM, Weiss NS, Thompson IM. Limitations of basing screening policies on screening trials: the US Preventive Services Task Force and Prostate Cancer Screening. Med Care (2013) 51:295–300. doi:10.1097/MLR.0b013e31827da979
3. Eggener SE, Badani K, Barocas DA, Barrisford GW, Cheng JS, Chin AI, et al. Gleason 6 prostate cancer: translating biology into population health. J Urol (2015) 194:626–34. doi:10.1016/j.juro.2015.01.126
4. Ritch CR, Graves AJ, Keegan KA, Ni S, Bassett JC, Chang SS, et al. Increasing use of observation among men at low risk for prostate cancer mortality. J Urol (2015) 193:801–6. doi:10.1016/j.juro.2014.08.102
5. Albertsen PC, Hanley JA, Fine J. 20-year outcomes following conservative management of clinically localized prostate cancer. JAMA (2005) 293:2095–101. doi:10.1001/jama.293.17.2095
6. Tosoian JJ, Mamawala M, Epstein JI, Landis P, Wolf S, Trock BJ, et al. Intermediate and longer-term outcomes from a prospective active-surveillance program for favorable-risk prostate cancer. J Clin Oncol (2015) 33:3379–85. doi:10.1200/JCO.2015.62.5764
7. Leyh-Bannurah SR, Abou-Haidar H, Dell’Oglio P, Schiffmann J, Tian Z, Heinzer H, et al. Primary Gleason pattern upgrading in contemporary patients with D’Amico low-risk prostate cancer: implications for future biomarkers and imaging modalities. BJU Int (2017) 119:692–9. doi:10.1111/bju.13570
8. Frye TP, George AK, Kilchevsky A, Maruf M, Siddiqui MM, Kongnyuy M, et al. Magnetic resonance imaging-transrectal ultrasound guided fusion biopsy to detect progression in patients with existing lesions on active surveillance for low and intermediate risk prostate cancer. J Urol (2017) 197:640–6. doi:10.1016/j.juro.2016.08.109
9. Berglund RK, Masterson TA, Vora KC, Eggener SE, Eastham JA, Guillonneau BD. Pathological upgrading and up staging with immediate repeat biopsy in patients eligible for active surveillance. J Urol (2008) 180:1964–7; discussion 7–8. doi:10.1016/j.juro.2008.07.051
10. Freedland SJ, Kane CJ, Amling CL, Aronson WJ, Terris MK, Presti JC Jr, et al. Upgrading and downgrading of prostate needle biopsy specimens: risk factors and clinical implications. Urology (2007) 69:495–9. doi:10.1016/j.urology.2006.10.036
11. Thaxton CS, Loeb S, Roehl KA, Kan D, Catalona WJ. Treatment outcomes of radical prostatectomy in potential candidates for 3 published active surveillance protocols. Urology (2010) 75:414–8. doi:10.1016/j.urology.2009.07.1353
12. Oberlin DT, Casalino DD, Miller FH, Meeks JJ. Dramatic increase in the utilization of multiparametric magnetic resonance imaging for detection and management of prostate cancer. Abdom Radiol (NY) (2017) 42:1255–8. doi:10.1007/s00261-016-0975-5
13. Almeida GL, Petralia G, Ferro M, Ribas CA, Detti S, Jereczek-Fossa BA, et al. Role of multi-parametric magnetic resonance image and PIRADS score in patients with prostate cancer eligible for active surveillance according PRIAS criteria. Urol Int (2016) 96:459–69. doi:10.1159/000444197
14. Stephan C, Rittenhouse H, Hu X, Cammann H, Jung K. Prostate-specific antigen (PSA) screening and new biomarkers for prostate cancer (PCa). EJIFCC (2014) 25:55–78.
15. Moher D, Liberati A, Tetzlaff J, Altman DG, Group P. Preferred reporting items for systematic reviews and meta-analyses: the PRISMA statement. Int J Surg (2010) 8:336–41. doi:10.1016/j.ijsu.2010.02.007
16. Klein J, de Gorski A, Benamran D, Vallee JP, De Perrot T, Wirth GJ, et al. Transrectal ultrasound-guided prostate biopsy for cancer detection: performance of 2D-, 3D- and 3D-MRI fusion targeted techniques. Urol Int (2017) 98:7–14. doi:10.1159/000452250
17. Salami SS, Ben-Levi E, Yaskiv O, Turkbey B, Villani R, Rastinehad AR. Risk stratification of prostate cancer utilizing apparent diffusion coefficient value and lesion volume on multiparametric MRI. J Magn Reson Imaging (2017) 45:610–6. doi:10.1002/jmri.25363
18. Henderson DR, de Souza NM, Thomas K, Riches SF, Morgan VA, Sohaib SA, et al. Nine-year follow-up for a study of diffusion-weighted magnetic resonance imaging in a prospective prostate cancer active surveillance cohort. Eur Urol (2016) 69:1028–33. doi:10.1016/j.eururo.2015.10.010
19. Kim TH, Jeong JY, Lee SW, Kim CK, Park BK, Sung HH, et al. Diffusion-weighted magnetic resonance imaging for prediction of insignificant prostate cancer in potential candidates for active surveillance. Eur Radiol (2015) 25:1786–92. doi:10.1007/s00330-014-3566-2
20. Marin L, Ezziane M, Comperat E, Mozer P, Cancel-Tassin G, Cote JF, et al. Comparison of semi-automated and manual methods to measure the volume of prostate cancer on magnetic resonance imaging. Diagn Interv Imaging (2017) 98:423–8. doi:10.1016/j.diii.2017.02.004
21. Porpiglia F, Cantiello F, De Luca S, Manfredi M, Veltri A, Russo F, et al. In-parallel comparative evaluation between multiparametric magnetic resonance imaging, prostate cancer antigen 3 and the prostate health index in predicting pathologically confirmed significant prostate cancer in men eligible for active surveillance. BJU Int (2016) 118:527–34. doi:10.1111/bju.13318
22. Ouzzane A, Renard-Penna R, Marliere F, Mozer P, Olivier J, Barkatz J, et al. Magnetic resonance imaging targeted biopsy improves selection of patients considered for active surveillance for clinically low risk prostate cancer based on systematic biopsies. J Urol (2015) 194:350–6. doi:10.1016/j.juro.2015.02.2938
23. Radtke JP, Kuru TH, Bonekamp D, Freitag MT, Wolf MB, Alt CD, et al. Further reduction of disqualification rates by additional MRI-targeted biopsy with transperineal saturation biopsy compared with standard 12-core systematic biopsies for the selection of prostate cancer patients for active surveillance. Prostate Cancer Prostatic Dis (2016) 19:283–91. doi:10.1038/pcan.2016.16
24. Dianat SS, Carter HB, Pienta KJ, Schaeffer EM, Landis PK, Epstein JI, et al. Magnetic resonance-invisible versus magnetic resonance-visible prostate cancer in active surveillance: a preliminary report on disease outcomes. Urology (2015) 85:147–53. doi:10.1016/j.urology.2014.06.085
25. Grey AD, Chana MS, Popert R, Wolfe K, Liyanage SH, Acher PL. Diagnostic accuracy of magnetic resonance imaging (MRI) prostate imaging reporting and data system (PI-RADS) scoring in a transperineal prostate biopsy setting. BJU Int (2015) 115:728–35. doi:10.1111/bju.12862
26. Venderink W, van Luijtelaar A, Bomers JG, van der Leest M, Hulsbergen-van de Kaa C, Barentsz JO, et al. Results of targeted biopsy in men with magnetic resonance imaging lesions classified equivocal, likely or highly likely to be clinically significant prostate cancer. Eur Urol (2017). doi:10.1016/j.eururo.2017.02.021
27. Nougaret S, Robertson N, Golia Pernicka J, Molinari N, Hotker AM, Ehdaie B, et al. The performance of PI-RADSv2 and quantitative apparent diffusion coefficient for predicting confirmatory prostate biopsy findings in patients considered for active surveillance of prostate cancer. Abdom Radiol (NY) (2017) 42:1968–74. doi:10.1007/s00261-017-1086-7
28. Barentsz JO, Richenberg J, Clements R, Choyke P, Verma S, Villeirs G, et al. ESUR prostate MR guidelines 2012. Eur Radiol (2012) 22:746–57. doi:10.1007/s00330-011-2377-y
29. Weinreb JC, Barentsz JO, Choyke PL, Cornud F, Haider MA, Macura KJ, et al. PI-RADS prostate imaging – reporting and data system: 2015, version 2. Eur Urol (2016) 69:16–40. doi:10.1016/j.eururo.2015.08.052
30. Hoeks CM, Somford DM, van Oort IM, Vergunst H, Oddens JR, Smits GA, et al. Value of 3-T multiparametric magnetic resonance imaging and magnetic resonance-guided biopsy for early risk restratification in active surveillance of low-risk prostate cancer: a prospective multicenter cohort study. Invest Radiol (2014) 49:165–72. doi:10.1097/RLI.0000000000000008
31. Stamatakis L, Siddiqui MM, Nix JW, Logan J, Rais-Bahrami S, Walton-Diaz A, et al. Accuracy of multiparametric magnetic resonance imaging in confirming eligibility for active surveillance for men with prostate cancer. Cancer (2013) 119:3359–66. doi:10.1002/cncr.28216
32. Lai WS, Gordetsky JB, Thomas JV, Nix JW, Rais-Bahrami S. Factors predicting prostate cancer upgrading on magnetic resonance imaging-targeted biopsy in an active surveillance population. Cancer (2017) 123:1941–8. doi:10.1002/cncr.30548
33. Vargas HA, Hotker AM, Goldman DA, Moskowitz CS, Gondo T, Matsumoto K, et al. Updated prostate imaging reporting and data system (PIRADS v2) recommendations for the detection of clinically significant prostate cancer using multiparametric MRI: critical evaluation using whole-mount pathology as standard of reference. Eur Radiol (2016) 26:1606–12. doi:10.1007/s00330-015-4015-6
34. Tan WP, Mazzone A, Shors S, Antoine N, Ekbal S, Khare N, et al. Central zone lesions on magnetic resonance imaging: should we be concerned? Urol Oncol (2017) 35:31.e7–e12. doi:10.1016/j.urolonc.2016.08.006
35. Cohen RJ, Shannon BA, Phillips M, Moorin RE, Wheeler TM, Garrett KL. Central zone carcinoma of the prostate gland: a distinct tumor type with poor prognostic features. J Urol (2008) 179:1762–7; discussion 7. doi:10.1016/j.juro.2008.01.017
36. Vargas HA, Akin O, Franiel T, Goldman DA, Udo K, Touijer KA, et al. Normal central zone of the prostate and central zone involvement by prostate cancer: clinical and MR imaging implications. Radiology (2012) 262:894–902. doi:10.1148/radiol.11110663
37. Pinto PA, Chung PH, Rastinehad AR, Baccala AA Jr, Kruecker J, Benjamin CJ, et al. Magnetic resonance imaging/ultrasound fusion guided prostate biopsy improves cancer detection following transrectal ultrasound biopsy and correlates with multiparametric magnetic resonance imaging. J Urol (2011) 186:1281–5. doi:10.1016/j.juro.2011.05.078
38. Okoro C, George AK, Siddiqui MM, Rais-Bahrami S, Walton-Diaz A, Shakir NA, et al. Magnetic resonance imaging/transrectal ultrasonography fusion prostate biopsy significantly outperforms systematic 12-core biopsy for prediction of total magnetic resonance imaging tumor volume in active surveillance patients. J Endourol (2015) 29:1115–21. doi:10.1089/end.2015.0027
39. Panebianco V, Barchetti F, Sciarra A, Ciardi A, Indino EL, Papalia R, et al. Multiparametric magnetic resonance imaging vs. standard care in men being evaluated for prostate cancer: a randomized study. Urol Oncol (2015) 33:17.e1–e7. doi:10.1016/j.urolonc.2014.09.013
40. Da Rosa MR, Milot L, Sugar L, Vesprini D, Chung H, Loblaw A, et al. A prospective comparison of MRI-US fused targeted biopsy versus systematic ultrasound-guided biopsy for detecting clinically significant prostate cancer in patients on active surveillance. J Magn Reson Imaging (2015) 41:220–5. doi:10.1002/jmri.24710
41. Nassiri N, Margolis DJ, Natarajan S, Sharma DS, Huang J, Dorey FJ, et al. Targeted biopsy to detect Gleason score upgrading during active surveillance for men with low versus intermediate risk prostate cancer. J Urol (2017) 197:632–9. doi:10.1016/j.juro.2016.09.070
42. Abdi H, Pourmalek F, Zargar H, Walshe T, Harris AC, Chang SD, et al. Multiparametric magnetic resonance imaging enhances detection of significant tumor in patients on active surveillance for prostate cancer. Urology (2015) 85:423–8. doi:10.1016/j.urology.2014.09.060
43. Siddiqui MM, Rais-Bahrami S, Turkbey B, George AK, Rothwax J, Shakir N, et al. Comparison of MR/ultrasound fusion-guided biopsy with ultrasound-guided biopsy for the diagnosis of prostate cancer. JAMA (2015) 313:390–7. doi:10.1001/jama.2014.17942
44. Marliere F, Puech P, Benkirane A, Villers A, Lemaitre L, Leroy X, et al. The role of MRI-targeted and confirmatory biopsies for cancer upstaging at selection in patients considered for active surveillance for clinically low-risk prostate cancer. World J Urol (2014) 32:951–8. doi:10.1007/s00345-014-1314-5
45. Li YH, Elshafei A, Li J, Hatem A, Zippe CD, Fareed K, et al. Potential benefit of transrectal saturation prostate biopsy as an initial biopsy strategy: decreased likelihood of finding significant cancer on future biopsy. Urology (2014) 83:714–8. doi:10.1016/j.urology.2013.12.029
46. Pepe P, Cimino S, Garufi A, Priolo G, Russo GI, Giardina R, et al. Detection rate for significant cancer at confirmatory biopsy in men enrolled in active surveillance protocol: 20 cores vs 30 cores vs MRI/TRUS fusion prostate biopsy. Arch Ital Urol Androl (2016) 88:300–3. doi:10.4081/aiua.2016.4.300
47. Galosi AB, Maselli G, Sbrollini G, Donatelli G, Montesi L, Talle M, et al. Cognitive zonal fusion biopsy of the prostate: original technique between target and saturation. Arch Ital Urol Androl (2016) 88:292–5. doi:10.4081/aiua.2016.4.292
48. Walton Diaz A, Shakir NA, George AK, Rais-Bahrami S, Turkbey B, Rothwax JT, et al. Use of serial multiparametric magnetic resonance imaging in the management of patients with prostate cancer on active surveillance. Urol Oncol (2015) 33:202.e1–e7. doi:10.1016/j.urolonc.2015.01.023
49. Siddiqui MM, Truong H, Rais-Bahrami S, Stamatakis L, Logan J, Walton-Diaz A, et al. Clinical implications of a multiparametric magnetic resonance imaging based nomogram applied to prostate cancer active surveillance. J Urol (2015) 193:1943–9. doi:10.1016/j.juro.2015.01.088
50. Felker ER, Wu J, Natarajan S, Margolis DJ, Raman SS, Huang J, et al. Serial magnetic resonance imaging in active surveillance of prostate cancer: incremental value. J Urol (2016) 195:1421–7. doi:10.1016/j.juro.2015.11.055
51. Rais-Bahrami S, Turkbey B, Rastinehad AR, Walton-Diaz A, Hoang AN, Siddiqui MM, et al. Natural history of small index lesions suspicious for prostate cancer on multiparametric MRI: recommendations for interval imaging follow-up. Diagn Interv Radiol (2014) 20:293–8. doi:10.5152/dir.2014.13319
52. Moore CM, Giganti F, Albertsen P, Allen C, Bangma C, Briganti A, et al. Reporting magnetic resonance imaging in men on active surveillance for prostate cancer: the PRECISE Recommendations-A Report of a European School of Oncology Task Force. Eur Urol (2017) 71:648–55. doi:10.1016/j.eururo.2016.06.011
53. Polascik TJ, Oesterling JE, Partin AW. Prostate specific antigen: a decade of discovery – what we have learned and where we are going. J Urol (1999) 162:293–306. doi:10.1016/S0022-5347(05)68543-6
54. Avgeris M, Mavridis K, Scorilas A. Kallikrein-related peptidases in prostate, breast, and ovarian cancers: from pathobiology to clinical relevance. Biol Chem (2012) 393:301–17. doi:10.1515/hsz-2011-0260
55. Punnen S, Pavan N, Parekh DJ. Finding the Wolf in Sheep’s Clothing: the 4Kscore is a novel blood test that can accurately identify the risk of aggressive prostate cancer. Rev Urol (2015) 17:3–13.
56. Borque-Fernando A, Esteban-Escano LM, Rubio-Briones J, Lou-Mercade AC, Garcia-Ruiz R, Tejero-Sanchez A, et al. A preliminary study of the ability of the 4Kscore test, the prostate cancer prevention trial-risk calculator and the European Research Screening Prostate-Risk Calculator for Predicting High-Grade Prostate Cancer. Actas Urol Esp (2016) 40:155–63. doi:10.1016/j.acuroe.2016.02.004
57. Lin DW, Newcomb LF, Brown MD, Sjoberg DD, Dong Y, Brooks JD, et al. Evaluating the Four Kallikrein Panel of the 4Kscore for prediction of high-grade prostate cancer in men in the canary prostate active surveillance study. Eur Urol (2017) 72(3):448–54. doi:10.1016/j.eururo.2016.11.017
58. Dijkstra S, Govers TM, Hendriks RJ, Schalken JA, Van Criekinge W, Van Neste L, et al. Cost-effectiveness of a new urinary biomarker-based risk score compared to standard of care in prostate cancer diagnostics – a decision analytical model. BJU Int (2017) 120(5):659–65. doi:10.1111/bju.13861
59. Bostrom PJ, Bjartell AS, Catto JW, Eggener SE, Lilja H, Loeb S, et al. Genomic predictors of outcome in prostate cancer. Eur Urol (2015) 68:1033–44. doi:10.1016/j.eururo.2015.04.008
60. Partin AW, Van Neste L, Klein EA, Marks LS, Gee JR, Troyer DA, et al. Clinical validation of an epigenetic assay to predict negative histopathological results in repeat prostate biopsies. J Urol (2014) 192:1081–7. doi:10.1016/j.juro.2014.04.013
61. AUA. Cell-Cycle Progression Score Provides Significant Prognostic Information in Patients With Gleason Score < 7. The ASCO Post: ASCO (2016).
62. Cuzick J, Swanson GP, Fisher G, Brothman AR, Berney DM, Reid JE, et al. Prognostic value of an RNA expression signature derived from cell cycle proliferation genes in patients with prostate cancer: a retrospective study. Lancet Oncol (2011) 12:245–55. doi:10.1016/S1470-2045(10)70295-3
63. Cooperberg MR, Simko JP, Cowan JE, Reid JE, Djalilvand A, Bhatnagar S, et al. Validation of a cell-cycle progression gene panel to improve risk stratification in a contemporary prostatectomy cohort. J Clin Oncol (2013) 31:1428–34. doi:10.1200/JCO.2012.46.4396
64. Wang Y, Klijn JG, Zhang Y, Sieuwerts AM, Look MP, Yang F, et al. Gene-expression profiles to predict distant metastasis of lymph-node-negative primary breast cancer. Lancet (2005) 365:671–9. doi:10.1016/S0140-6736(05)70933-8
65. Arsov C, Jankowiak F, Hiester A, Rabenalt R, Quentin M, Schimmoller L, et al. Prognostic value of a cell-cycle progression score in men with prostate cancer managed with active surveillance after MRI-guided prostate biopsy – a pilot study. Anticancer Res (2014) 34:2459–66.
66. Eure G, Germany R, Given R, Lu R, Shindel AW, Rothney M, et al. Use of a 17-gene prognostic assay in contemporary urologic practice: results of an interim analysis in an observational cohort. Urology (2017) 107:67–75. doi:10.1016/j.urology.2017.02.052
67. Brand TC, Zhang N, Crager MR, Maddala T, Dee A, Sesterhenn IA, et al. Patient-specific meta-analysis of 2 clinical validation studies to predict pathologic outcomes in prostate cancer using the 17-gene genomic prostate score. Urology (2016) 89:69–75. doi:10.1016/j.urology.2015.12.008
68. Cullen J, Rosner IL, Brand TC, Zhang N, Tsiatis AC, Moncur J, et al. A biopsy-based 17-gene genomic prostate score predicts recurrence after radical prostatectomy and adverse surgical pathology in a racially diverse population of men with clinically low- and intermediate-risk prostate cancer. Eur Urol (2015) 68:123–31. doi:10.1016/j.eururo.2014.11.030
69. Klein EA, Cooperberg MR, Magi-Galluzzi C, Simko JP, Falzarano SM, Maddala T, et al. A 17-gene assay to predict prostate cancer aggressiveness in the context of Gleason grade heterogeneity, tumor multifocality, and biopsy undersampling. Eur Urol (2014) 66:550–60. doi:10.1016/j.eururo.2014.05.004
70. Marrone M, Potosky AL, Penson D, Freedman AN. A 22 gene-expression assay, decipher(R) (GenomeDx Biosciences) to predict five-year risk of metastatic prostate cancer in men treated with radical prostatectomy. PLoS Curr (2015) 7. doi:10.1371/currents.eogt.761b81608129ed61b0b48d42c04f92a4
71. Klein EA, Haddad Z, Yousefi K, Lam LL, Wang Q, Choeurng V, et al. Decipher genomic classifier measured on prostate biopsy predicts metastasis risk. Urology (2016) 90:148–52. doi:10.1016/j.urology.2016.01.012
72. Blume-Jensen P, Berman DM, Rimm DL, Shipitsin M, Putzi M, Nifong TP, et al. Development and clinical validation of an in situ biopsy-based multimarker assay for risk stratification in prostate cancer. Clin Cancer Res (2015) 21:2591–600. doi:10.1158/1078-0432.CCR-14-2603
73. Saad F, Latour M, Lattouf JB, Widmer H, Zorn KC, Mes-Masson AM, et al. Biopsy based proteomic assay predicts risk of biochemical recurrence after radical prostatectomy. J Urol (2017) 197:1034–40. doi:10.1016/j.juro.2016.09.116
74. Peabody JW, DeMaria LM, Tamondong-Lachica D, Florentino J, Czarina Acelajado M, Ouenes O, et al. Impact of a protein-based assay that predicts prostate cancer aggressiveness on urologists’ recommendations for active treatment or active surveillance: a randomized clinical utility trial. BMC Urol (2017) 17:51. doi:10.1186/s12894-017-0243-1
75. Li J, Yen C, Liaw D, Podsypanina K, Bose S, Wang SI, et al. PTEN, a putative protein tyrosine phosphatase gene mutated in human brain, breast, and prostate cancer. Science (1997) 275:1943–7. doi:10.1126/science.275.5308.1943
76. Lokman U, Erickson AM, Vasarainen H, Rannikko AS, Mirtti T. PTEN loss but not ERG expression in diagnostic biopsies is associated with increased risk of progression and adverse surgical findings in men with prostate cancer on active surveillance. Eur Urol Focus (2017). doi:10.1016/j.euf.2017.03.004
77. Troyer DA, Jamaspishvili T, Wei W, Feng Z, Good J, Hawley S, et al. A multicenter study shows PTEN deletion is strongly associated with seminal vesicle involvement and extracapsular extension in localized prostate cancer. Prostate (2015) 75:1206–15. doi:10.1002/pros.23003
78. Powell IJ, Dyson G, Chinni SR, Bollig-Fischer A. Considering race and the potential for ERG expression as a biomarker for prostate cancer. Per Med (2014) 11:409–12. doi:10.2217/pme.14.26
79. Berg KD, Vainer B, Thomsen FB, Roder MA, Gerds TA, Toft BG, et al. ERG protein expression in diagnostic specimens is associated with increased risk of progression during active surveillance for prostate cancer. Eur Urol (2014) 66:851–60. doi:10.1016/j.eururo.2014.02.058
80. Berg KD, Brasso K, Thomsen FB, Roder MA, Holten-Rossing H, Toft BG, et al. ERG protein expression over time: from diagnostic biopsies to radical prostatectomy specimens in clinically localised prostate cancer. J Clin Pathol (2015) 68:788–94. doi:10.1136/jclinpath-2015-202894
81. Ward JF, Eggener SE. Active surveillance monitoring: the role of novel biomarkers and imaging. Asian J Androl (2015) 17:882–4; discussion 3. doi:10.4103/1008-682X.156858
82. Lin DW, Newcomb LF, Brown EC, Brooks JD, Carroll PR, Feng Z, et al. Urinary TMPRSS2:ERG and PCA3 in an active surveillance cohort: results from a baseline analysis in the Canary Prostate Active Surveillance Study. Clin Cancer Res (2013) 19:2442–50. doi:10.1158/1078-0432.CCR-12-3283
83. Tosoian JJ, Loeb S, Feng Z, Isharwal S, Landis P, Elliot DJ, et al. Association of [-2]proPSA with biopsy reclassification during active surveillance for prostate cancer. J Urol (2012) 188:1131–6. doi:10.1016/j.juro.2012.06.009
84. Cantiello F, Russo GI, Cicione A, Ferro M, Cimino S, Favilla V, et al. PHI and PCA3 improve the prognostic performance of PRIAS and Epstein criteria in predicting insignificant prostate cancer in men eligible for active surveillance. World J Urol (2016) 34:485–93. doi:10.1007/s00345-015-1643-z
85. Darash-Yahana M, Pikarsky E, Abramovitch R, Zeira E, Pal B, Karplus R, et al. Role of high expression levels of CXCR4 in tumor growth, vascularization, and metastasis. FASEB J (2004) 18:1240–2. doi:10.1096/fj.03-0935fje
86. Zlotnik A. New insights on the role of CXCR4 in cancer metastasis. J Pathol (2008) 215:211–3. doi:10.1002/path.2350
87. Gladson CL, Welch DR. New insights into the role of CXCR4 in prostate cancer metastasis. Cancer Biol Ther (2008) 7:1849–51. doi:10.4161/cbt.7.11.7218
88. Hsiao JJ, Ng BH, Smits MM, Wang J, Jasavala RJ, Martinez HD, et al. Androgen receptor and chemokine receptors 4 and 7 form a signaling axis to regulate CXCL12-dependent cellular motility. BMC Cancer (2015) 15:204. doi:10.1186/s12885-015-1201-5
89. Goltz D, Holmes EE, Gevensleben H, Sailer V, Dietrich J, Jung M, et al. CXCL12 promoter methylation and PD-L1 expression as prognostic biomarkers in prostate cancer patients. Oncotarget (2016) 7:53309–20. doi:10.18632/oncotarget.10786
90. Murray NP, Reyes E, Tapia P, Orellana N, Duenas R, Fuentealba C, et al. Diagnostic performance of malignant prostatic cells detection in blood for early detection of prostate cancer: comparison to prostatic biopsy. Arch Esp Urol (2011) 64:961–71.
91. Murray NP, Reyes E, Orellana N, Fuentealba C, Jacob O. Head to head comparison of the Chun Nomogram, Percentage Free PSA and primary circulating prostate cells to predict the presence of prostate cancer at repeat biopsy. Asian Pac J Cancer Prev (2016) 17:2941–6.
92. Murray NP, Reyes E, Fuentealba C, Orellana N, Jacob O. Primary circulating prostate cells are not detected in men with low grade small volume prostate cancer. J Oncol (2014) 2014:612674. doi:10.1155/2014/612674
93. Kim ST, Cheng Y, Hsu FC, Jin T, Kader AK, Zheng SL, et al. Prostate cancer risk-associated variants reported from genome-wide association studies: meta-analysis and their contribution to genetic variation. Prostate (2010) 70:1729–38. doi:10.1002/pros.21208
94. Grin B, Loeb S, Roehl K, Cooper PR, Catalona WJ, Helfand BT. A rare 8q24 single nucleotide polymorphism (SNP) predisposes North American men to prostate cancer and possibly more aggressive disease. BJU Int (2015) 115:101–5. doi:10.1111/bju.12847
95. Dall’Era MA, Klotz L. Active surveillance for intermediate-risk prostate cancer. Prostate Cancer Prostatic Dis (2017) 20:1–6. doi:10.1038/pcan.2016.51
Keywords: prostatic adenocarcinoma, multiparametric MRI, cancer imaging, cancer genetics, cancer epigenetics
Citation: Glaser ZA, Gordetsky JB, Porter KK, Varambally S and Rais-Bahrami S (2017) Prostate Cancer Imaging and Biomarkers Guiding Safe Selection of Active Surveillance. Front. Oncol. 7:256. doi: 10.3389/fonc.2017.00256
Received: 01 September 2017; Accepted: 12 October 2017;
Published: 30 October 2017
Edited by:
Guillaume Ploussard, IUCT-O, FranceReviewed by:
Alan Dal Pra, University of Bern, SwitzerlandCopyright: © 2017 Glaser, Gordetsky, Porter, Varambally and Rais-Bahrami. This is an open-access article distributed under the terms of the Creative Commons Attribution License (CC BY). The use, distribution or reproduction in other forums is permitted, provided the original author(s) or licensor are credited and that the original publication in this journal is cited, in accordance with accepted academic practice. No use, distribution or reproduction is permitted which does not comply with these terms.
*Correspondence: Soroush Rais-Bahrami, c3JhaXNiYWhyYW1pQHVhYm1jLmVkdQ==
Disclaimer: All claims expressed in this article are solely those of the authors and do not necessarily represent those of their affiliated organizations, or those of the publisher, the editors and the reviewers. Any product that may be evaluated in this article or claim that may be made by its manufacturer is not guaranteed or endorsed by the publisher.
Research integrity at Frontiers
Learn more about the work of our research integrity team to safeguard the quality of each article we publish.