- 1Metabolism and Cancer Laboratory, Centre for Cancer Research, Hudson Institute of Medical Research, Clayton, VIC, Australia
- 2Department of Molecular and Translational Sciences, Monash University, Clayton, VIC, Australia
- 3Department of Anatomy and Neuroscience, University of Melbourne and Florey Institute of Neuroscience and Mental Health, Parkville, VIC, Australia
- 4Department of Physiology, Monash University, Clayton, VIC, Australia
Local and systemic factors have been shown to drive the growth of breast cancer cells in postmenopausal obese women, who have increased risk of estrogen receptor-positive breast cancer. Estrogens, produced locally in the breast fat by the enzyme aromatase, have an important role in promoting cancer cell proliferation. Ghrelin, a 28-amino acid peptide hormone, may also influence cancer growth. This peptide is produced in the stomach and acts centrally to regulate appetite and growth hormone release. Circulating levels of ghrelin, and its unacylated form, des-acyl ghrelin, are almost always inversely correlated with obesity, and these peptide hormones have recently been shown to inhibit adipose tissue aromatase expression. Ghrelin and des-acyl ghrelin have also been shown to be produced by some tumor cells and influence tumor growth. The ghrelin/des-acyl ghrelin–cancer axis is complex, one reason being that tumor cells have been shown to express splice variants of ghrelin, and ghrelin and des-acyl ghrelin might act at receptors other than the cognate ghrelin receptor, growth hormone secretagogue receptor 1a, in tumors. Effects of ghrelin and des-acyl ghrelin on energy homeostasis may also affect tumor development and growth. This review will summarize our current understanding of the role of ghrelin and des-acyl ghrelin in hormone-dependent cancers, breast cancer in particular.
Obesity, Aromatase, and Postmenopausal Breast Cancer
Estrogens, including 17β-estradiol, play a crucial role in breast cancer. In premenopausal women, estrogens are mainly produced by the ovaries. After menopause when the ovaries stop producing measurable levels of estrogens, the stromal cells of adipose tissue, including the breast, become the main site of estrogen biosynthesis (1). In postmenopausal women, local estrogen production and circulating estrogens, suggested to be mainly adipose derived, are associated with driving the proliferation of breast tumor cells (2–7). Aromatase, encoded by the CYP19A1 gene, is the enzyme responsible for catalyzing the final and key step in estrogen biosynthesis by converting androgens into estrogens. It is expressed in steroidogenic tissues such as ovarian granulosa cells and the placenta, as well as in non-steroidogenic tissues such as bone and adipose tissue (8). The CYP19A1 gene is located on chromosome 15q21.2 and spans approximately 123 kb, with 9 coding exons (II–X) and a 93-kb regulatory region. Tissue-specific promoters are used to regulate aromatase expression by their interaction with promoter-specific first exons (9). Each of these promoters ensures a fine-tuned tissue-selective regulation of aromatase expression. For example, in normal breast adipose tissue, the majority of aromatase transcripts are derived from activation of the distal promoter, promoter I.4. This promoter is activated by glucocorticoids in the presence of class 1 cytokines such as interleukin 6, interleukin 11, leukemia inhibitory factor, and oncostatin M (OSM) via the Janus kinase-1/signal transducer and activator of transcription 3 pathway (10). Promoter I.4 is also activated by the inflammatory mediator, tumor necrosis factor alpha (TNFα), via the mitogen-activated protein kinase-AP1 pathway (10). Interestingly, promoter I.4 is also utilized in bone, where aromatase expression is increased in response to dexamethasone and OSM (11). However, in breast adipose tissue of obese women and those with breast cancer, the majority of aromatase transcripts are derived from the coordinated activation of promoters I.3 and II. A number of obesity-associated factors, including inflammatory mediator PGE2 and the adipokine leptin, have been shown to stimulate aromatase expression via these promoters (12, 13). More recently, the gut-derived hormone, ghrelin, and its unacylated form, des-acyl ghrelin, were shown to inhibit aromatase expression in adipose stromal cells (14, 15).
Ghrelin and Des-Acyl Ghrelin
Ghrelin, first discovered in the rat stomach in 1999, is the only known peptide hormone to be acylated (16, 17). The human ghrelin peptide is characterized by the level of acylation at serine-3, including non-acylated, octanoylated (C8:0), decanoylated (C10:0), and decenoylated (C10:1) (18). The rat and human ghrelin are generally acylated with an n-octanoyl group (C8:0). The structure of human and rat ghrelin differs at two amino acids (Figure 1) (16). In addition to the gut, ghrelin is also produced in small quantities by many tissues including the hypothalamus, pituitary, lung, heart, kidney, testis, pancreas, colon, ileum, jejunum, and duodenum (19–22). The ghrelin gene (GHRL) is located on chromosome 3p25-26 and encodes a mature mRNA that is 511 bp long (23–25). Ghrelin mRNA is then translated into preproghrelin, which contains 117 amino acids and can then be acylated and processed into ghrelin and obestatin (Figure 2). Ghrelin-O-acyl transferase (GOAT) is the only enzyme that has been molecularly identified to be able to acylate ghrelin (26–28). The ghrelin pro-peptide is cleaved by signal peptidase, prohormoneconvertase 1/3 (PC 1/3) (29), and carboxypeptidase-B like enzyme (18). Whereas the enzymes that cleave the obestatin peptide from the prohormone are still unknown. Preproghrelin can also be cleaved without first being acylated yielding des-acyl ghrelin and obestatin.
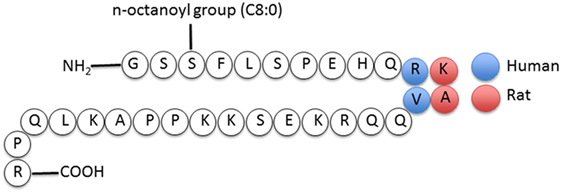
Figure 1. Structure of human and rat ghrelin (31).
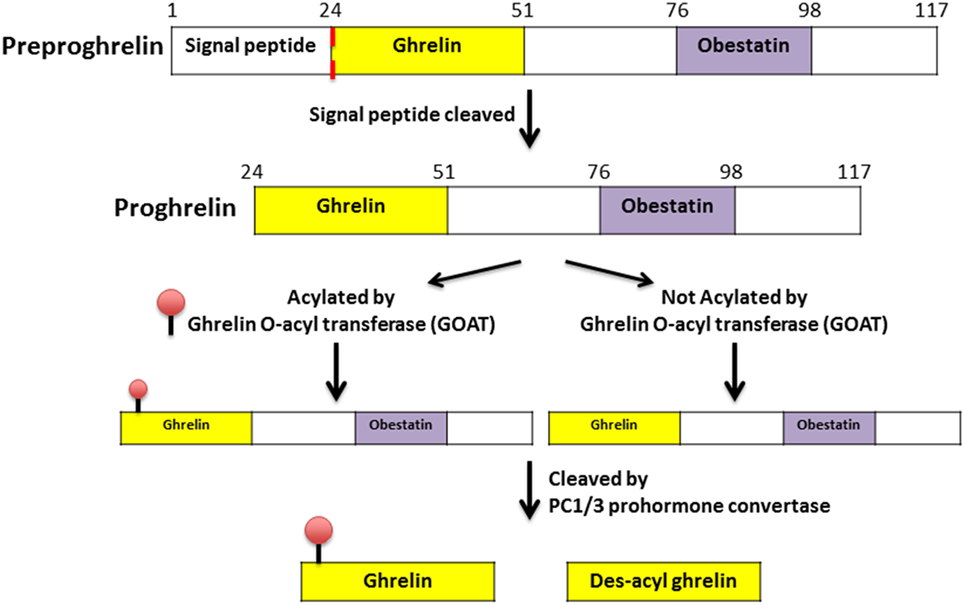
Figure 2. Processing and acylation of the GHRL gene product to yield ghrelin and des-acyl ghrelin (30).
Previous studies have demonstrated that ghrelin is the major active form of the hormone acting through the well-characterized ghrelin receptor, growth hormone secretagogue receptor 1a (GHSR1a). GHSR1a is expressed in many tissues such as the pituitary, hypothalamus, stomach, adipose tissue, bone, and prostate, as well as in numerous tumor types such as prostate, breast, and ovarian cancer. Ghrelin binding to GHSR1a leads to activation phospholipase C and generation of inositol triphosphate and diacylglycerol, leading to the stimulation of intracellular calcium release. However, studies have indicated that the mechanism of action of ghrelin in adipose stromal cells is different from the action of ghrelin via GHSR1a in the CNS (14, 32). Recent studies have demonstrated that des-acyl ghrelin is also biologically active (33) and likely acts though an unidentified alternative ghrelin receptor (14, 34, 35).
Roles of Ghrelin and Des-Acyl Ghrelin in Regulating Appetite, Energy Homeostasis, and Obesity
Although it is an orexigenic hormone, levels of ghrelin are inversely correlated with BMI in Caucasians and Pima Indians and reduced in individuals with type II diabetes (36, 37). Moreover, obese individuals have low ghrelin levels before and after meals compared with normal weight individuals (38), a finding that is consistent in obese females (39) and teenagers (40). The role of ghrelin to stimulate growth hormone release also contributes to the regulation of energy homeostasis. Rats receiving subcutaneous ghrelin (200 µg) show stimulated glucose production in the liver, effects that are inhibited by des-acyl ghrelin (41). Consistently, GOAT knockout mice have been shown to have low glucose levels after a low calorie meal compared with ghrelin- or growth hormone-treated mice (33). Different effects of ghrelin and des-acyl ghrelin are largely attributable to differences in receptor usage. Beneficial effects of des-acyl ghrelin on glucose homeostasis have led to the evaluation of AZP531, a cyclic des-acyl ghrelin analog and mimetic, in clinical trials for the treatment of type II diabetes (42). AZP531 has no agonist activity at GHSR1a.
Ghrelin, Des-Acyl Ghrelin, and Aromatase
The effect of ghrelin and des-acyl ghrelin on aromatase expression in adipose stromal cells was recently examined (14, 15). In isolated adipose stromal cells in culture, ghrelin and des-acyl ghrelin were equipotent (picomolar doses) at suppressing both the basal and PGE2-stimulated expression and activity of aromatase. GHSR1a was undetectable in these cells. Ghrelin was found to inhibit cAMP formation, suggesting that ghrelin and des-acyl ghrelin act at a receptor other than GHSR1a, where ghrelin but not des-acyl ghrelin is an agonist. The expression of aromatase in obesity and breast cancer is dependent on cAMP. Hence, decreased levels of ghrelin in obesity provide a possible mechanism for the obesity-associated increase in aromatase within the breast. Obesity is also associated with chronic low grade inflammation and an increase in macrophage-derived inflammatory mediators including TNFα and PGE2 (43, 44). Both ghrelin and des-acyl ghrelin suppress macrophage-derived inflammatory factors (15, 45), including TNFα and the expression of COX-2, the rate-limiting enzyme in prostaglandin biosynthesis. Pretreatment of macrophages with des-acyl ghrelin reduced the effect of conditioned media to stimulate aromatase expression in breast adipose stromal cells (15).
Ghrelin in Cancer
Ghrelin has been implicated in a multitude of cancers including colorectal, pituitary, head and neck, esophageal, liver, gastrointestinal, lung, neuroendocrine, non-Hodgkin lymphoma, pancreatic, thyroid, ovarian, prostate, and breast (46). Ligand-binding assays performed on non-tumoral and neoplastic breast tissue confirmed that the ghrelin analog, hexarelin, was capable of binding to breast cancers, with highest affinity demonstrated for well-differentiated invasive breast carcinomas (47). Interestingly, the ligand was displaced by a number of ghrelin analogs, as well as des-acyl ghrelin, suggesting that ghrelin and des-acyl ghrelin may act on breast cancer cells. This provides further evidence for the presence of alternate ghrelin receptors.
In a study of 53 female patients, including women with benign ovarian tumors and those with ovarian cancer, plasma concentrations of acyl ghrelin were significantly higher compared to the control population, whereas there was no difference between cancer and control groups when total ghrelin plasma levels were compared (48). Due to a lack of scientific evidence for the presence of GHSR1a in human ovaries, the authors suggested that there is likely no direct linkage between ghrelin levels and ovarian cancer development (48). However, now that other receptors for ghrelin are known to exist, this conclusion might be revised. In prostate cancer, a study of 30 patients with benign prostate hyperplasia and 50 controls found no association between the levels of ghrelin and cancer development or progression (49). However, a study by Malendowicz et al. demonstrated that acyl ghrelin and the ratio of acyl ghrelin to total ghrelin were significantly higher in 18 prostate cancer patients compared with 12 benign prostate hyperplasia controls. Total ghrelin plasma levels were similar between prostate cancer and the control group (50).
To date, few studies have examined circulating ghrelin levels in women with breast cancer or with a history of breast cancer (51, 52). However, these focused on effects of therapies, including chemotherapy and isoflavonoids, on circulating ghrelin levels as opposed to relating blood levels with cancer risk. Numerous studies have demonstrated that SNPs in the ghrelin gene are associated with breast cancer risk (53–57), and a study in women with low Native American ancestry also demonstrated that SNPs in the ghrelin gene are associated with breast cancer-specific mortality (58). However, in the absence of functional data, it is difficult to assign causality to these findings.
In some hormone-dependent cancers, ghrelin has been shown to have pro-proliferative effects, whereas in others, it had anti-proliferative effects. Thus, heterogeneity among cancers extends to their responses to ghrelin and des-acyl ghrelin.
Complexity of the Ghrelin Axis in Cancer
In vitro studies have demonstrated that ghrelin can have both proliferative and anti-proliferative effects. Ghrelin stimulates the growth of various cancer cell lines derived from the endometrium (59), prostate (60, 61), and breast (62). Conversely, ghrelin has also been shown to inhibit the growth of small cell lung carcinoma (63), prostate (64, 65), and breast cancer cell lines (47). In the estrogen receptor (ER) + human breast cancer cell line, MCF7, ghrelin at concentrations of 0–1,000 nM had no effect on cell proliferation (62), whereas Cassoni et al. demonstrated that ghrelin, des-acyl ghrelin, as well as a number of ghrelin mimetics, significantly inhibited cell proliferation (47). This effect was also seen in ER + T47D and the triple-negative breast cancer cell line MDA-MB-231, while other groups demonstrated that ghrelin at 10 and 100 nM stimulates MDA-MB-231 cell proliferation (62, 66). Differences in culture conditions or cell lines used may have contributed to these discrepancies. In particular, the addition of 4-(2-aminoethyl) benzene-sulphonyl fluoride hydrochloride (AEBSF) in the study by Jeffery et al. may have prevented the conversion of ghrelin to des-acyl ghrelin, while the effects observed in response to ghrelin in the absence of AEBSF in the Cassoni et al. paper may have in fact been responses to des-acyl ghrelin.
In addition to its well-characterized expression in the stomach, ghrelin is also produced at many other sites, including breast tumors. Breast cancer patients with tumors that express ghrelin have a 2.5 to 3 times lower risk of recurrence or breast cancer-associated death compared to those lacking expression of ghrelin (67). The ghrelin gene can also be alternatively spliced and the transcript variants have been described in breast and prostate cancer (68, 69). In breast, a variant of the ghrelin gene product that includes intron 1 (In1-variant) with a conserved first 12 amino acids and different C-terminus, was detected in tissues where GOAT was expressed and elevated in tumor tissue compared to normal. Although GHSR1a mediates many of the endocrine functions of ghrelin, GHSR1a is absent in several breast cancer cell lines, including MCF7 and MDA-MB-231 cells, and these cells respond to des-acyl ghrelin in vitro (47). Moreover, we have recently shown that ghrelin and des-acyl ghrelin inhibit aromatase expression in the absence of GHSR1a, suggesting that ghrelin may act though an unidentified alternative receptor in the breast (14). Another GHSR isoform, GHSR1b, has been identified. It has a truncated, 5-transmembrane, structure and has been proposed to be a non-functional form of GHSR. GHSR1b has been found to be expressed in lung cancer cell lines, including LC319 and PC14 (70), in the epithelium of breast cancer tissues and in breast cancer cell lines, including MDA-MB-231, MCF7, T47D, and MDA-MB-435 (62, 68). It has also been detected in benign prostatic hyperplasia (65), human erythroleukemic cell lines (71), colorectal cancer cells (72), the human adrenal cortex (73), and in benign and malignant adrenocortical tumors (74). Interestingly, GHSR1a and GHSR1b are not expressed in all tissues and tumors where ghrelin is expressed. Therefore, the roles of ghrelin and des-acyl ghrelin differ between cancers. To exploit the potent inhibitory (anti-proliferative) effects that they sometimes exert will require characterization of individual cancers and a personalized approach to treatment with compounds that mimic ghrelin or des-acyl ghrelin’s tumor inhibiting effects.
Obestatin and Cancer
Obestatin has been implicated in numerous cancers including gastric, ovarian, thyroid, invasive breast, and prostate cancers.
Exogenous obestatin stimulates KATO-III gastric cancer cell proliferation via MAPK, ERK1/2-dependent pathways (75). In gastric cancer, obestatin binds to GPR39 leading to the formation of a GPR39/β-arresin/Src complex, which leads to the activation of AKT signaling via EGFR transactivation (76). Obestatin in GPR39-bearing gastric cancer cells stimulates epithelial–mesenchymal transition and angiogenesis, as well as affecting morphology, migration, invasion, and proliferation of these cells (77). Furthermore, obestatin causes anti-proliferative effects in TT medullary thyroid carnicinoma cells as well as in pancreatic neuroendocrine tumor cells (78).
With regard to the relationship between circulating peptides and cancer, both obestatin and ghrelin levels were found to be elevated in benign and malignant ovarian cancers (48). No significant associations between circulating obestatin levels and prostate cancer were observed (50).
Expression of obestatin has also been examined in thyroid cancer where levels where found to be elevated in nodular goiter (benign thyroid tumor), but reduced in medullary cancer (malignant thyroid tumor) (79). Conversely, ghrelin levels increased with malignancy. The authors suggested that the ghrelin gene may be alternatively spliced or that ghrelin peptide production may be independently regulated.
In invasive breast cancer and similar to findings relating to ghrelin, the expression of obestatin is weakly correlated with low histological grade, ER positivity, small tumor size, and low proliferative index (67). However, the expression of obestatin did not predict survival.
Conclusion
Obesity-related breast cancers are largely estrogen dependent. Increased expression of aromatase in obese/inflamed breast adipose tissue contributes to the cancers. In some cases, reduced levels of ghrelin and des-acyl ghrelin may release estrogen biosynthesis and proliferation from inhibition. The ability of ghrelin and des-acyl ghrelin to reduce estrogen production and breast cancer growth may support their use, or ghrelin/des-acyl ghrelin mimetics, as therapeutics for suitably characterized cancers in the future.
Author Contributions
Conception of article, drafting, and review (CA, JF, and KB).
Conflict of Interest Statement
The authors declare that the research was conducted in the absence of any commercial or financial relationships that could be construed as a potential conflict of interest.
Funding
This work was supported by the Victorian Government Operational Infrastructure Support Program. KB is supported by the Mavis Robertson Fellowship from the National Breast Cancer Foundation (NBCF; ECF-16-004), by an NBCF Novel Concept Award (NC-14-011), and by NHMRC project grant (GNT1061800). CA is supported by an Endocrine Society of Australia Research Higher Degree Scholarship.
References
1. Ackerman GE, Smith ME, Mendelson CR, Macdonald PC, Simpson ER. Aromatization of androstenedione by human adipose tissue stromal cells in monolayer culture. J Clin Endocrinol Metab (1981) 53:412–7. doi:10.1210/jcem-53-2-412
2. Thomas HV, Key TJ, Allen DS, Moore JW, Dowsett M, Fentiman IS, et al. A prospective study of endogenous serum hormone concentrations and breast cancer risk in post-menopausal women on the island of Guernsey. Br J Cancer (1997) 76:401–5. doi:10.1038/bjc.1997.398
3. Toniolo PG, Levitz M, Zeleniuch-Jacquotte A, Banerjee S, Koenig KL, Shore RE, et al. A prospective study of endogenous estrogens and breast cancer in postmenopausal women. J Natl Cancer Inst (1995) 87:190–7. doi:10.1093/jnci/87.3.190
4. Gunnarsson C, Hellqvist E, Stal O. 17beta-Hydroxysteroid dehydrogenases involved in local oestrogen synthesis have prognostic significance in breast cancer. Br J Cancer (2005) 92:547–52. doi:10.1038/sj.bjc.6602375
5. Larionov AA, Berstein LM, Miller WR. Local uptake and synthesis of oestrone in normal and malignant postmenopausal breast tissues. J Steroid Biochem Mol Biol (2002) 81:57–64. doi:10.1016/S0960-0760(02)00047-X
6. O’Neill JS, Elton RA, Miller WR. Aromatase activity in adipose tissue from breast quadrants: a link with tumour site. Br Med J (Clin Res Ed) (1988) 296:741–3. doi:10.1136/bmj.296.6624.741
7. Bulun SE, Price TM, Aitken J, Mahendroo MS, Simpson ER. A link between breast cancer and local estrogen biosynthesis suggested by quantification of breast adipose tissue aromatase cytochrome P450 transcripts using competitive polymerase chain reaction after reverse transcription. J Clin Endocrinol Metab (1993) 77:1622–8. doi:10.1210/jcem.77.6.8117355
8. Simpson ER, Mahendroo MS, Means GD, Kilgore MW, Hinshelwood MM, Graham-Lorence S, et al. Aromatase cytochrome P450, the enzyme responsible for estrogen biosynthesis. Endocr Rev (1994) 15:342–55. doi:10.1210/er.15.3.342
9. Mahendroo MS, Mendelson CR, Simpson ER. Tissue-specific and hormonally controlled alternative promoters regulate aromatase cytochrome P450 gene expression in human adipose tissue. J Biol Chem (1993) 268:19463–70.
10. Zhao Y, Nichols JE, Bulun SE, Mendelson CR, Simpson ER. Aromatase P450 gene expression in human adipose tissue. Role of a Jak/STAT pathway in regulation of the adipose-specific promoter. J Biol Chem (1995) 270:16449–57. doi:10.1074/jbc.270.27.16449
11. Watanabe M, Simpson ER, Pathirage N, Nakajin S, Clyne CD. Aromatase expression in the human fetal osteoblastic cell line SV-HFO. J Mol Endocrinol (2004) 32:533–45. doi:10.1677/jme.0.0320533
12. Brown KA, Mcinnes KJ, Hunger NI, Oakhill JS, Steinberg GR, Simpson ER. Subcellular localization of cyclic AMP-responsive element binding protein-regulated transcription coactivator 2 provides a link between obesity and breast cancer in postmenopausal women. Cancer Res (2009) 69:5392–9. doi:10.1158/0008-5472.CAN-09-0108
13. Zhao Y, Agarwal VR, Mendelson CR, Simpson ER. Transcriptional regulation of CYP19 gene (aromatase) expression in adipose stromal cells in primary culture. J Steroid Biochem Mol Biol (1997) 61:203–10. doi:10.1016/S0960-0760(97)80013-1
14. Docanto MM, Yang F, Callaghan B, AU CC, Ragavan R, Wang X, et al. Ghrelin and des-acyl ghrelin inhibit aromatase expression and activity in human adipose stromal cells: suppression of cAMP as a possible mechanism. Breast Cancer Res Treat (2014) 147:193–201. doi:10.1007/s10549-014-3060-1
15. Au CC, Docanto MM, Zahid H, Raffaelli FM, Ferrero RL, Furness JB, et al. Des-acyl ghrelin inhibits the capacity of macrophages to stimulate the expression of aromatase in breast adipose stromal cells. J Steroid Biochem Mol Biol (2016). doi:10.1016/j.jsbmb.2016.07.005
16. Kojima M, Hosoda H, Date Y, Nakazato M, Matsuo H, Kangawa K. Ghrelin is a growth-hormone-releasing acylated peptide from stomach. Nature (1999) 402:656–60. doi:10.1038/45230
17. Ariyasu H, Takaya K, Tagami T, Ogawa Y, Hosoda K, Akamizu T, et al. Stomach is a major source of circulating ghrelin, and feeding state determines plasma ghrelin-like immunoreactivity levels in humans. J Clin Endocrinol Metab (2001) 86:4753–8. doi:10.1210/jcem.86.10.7885
18. Hosoda H, Kojima M, Mizushima T, Shimizu S, Kangawa K. Structural divergence of human ghrelin. Identification of multiple ghrelin-derived molecules produced by post-translational processing. J Biol Chem (2003) 278:64–70. doi:10.1074/jbc.M205366200
19. Gnanapavan S, Kola B, Bustin SA, Morris DG, McGee P, Fairclough P, et al. The tissue distribution of the mRNA of ghrelin and subtypes of its receptor, GHS-R, in humans. J Clin Endocrinol Metab (2002) 87:2988. doi:10.1210/jcem.87.6.8739
20. Ghelardoni S, Carnicelli V, Frascarelli S, Ronca-Testoni S, Zucchi R. Ghrelin tissue distribution: comparison between gene and protein expression. J Endocrinol Invest (2006) 29:115–21. doi:10.1007/BF03344083
21. Korbonits M, Bustin SA, Kojima M, Jordan S, Adams EF, Lowe DG, et al. The expression of the growth hormone secretagogue receptor ligand ghrelin in normal and abnormal human pituitary and other neuroendocrine tumors. J Clin Endocrinol Metab (2001) 86:881–7. doi:10.1210/jcem.86.2.7190
22. Date Y, Kojima M, Hosoda H, Sawaguchi A, Mondal MS, Suganuma T, et al. Ghrelin, a novel growth hormone-releasing acylated peptide, is synthesized in a distinct endocrine cell type in the gastrointestinal tracts of rats and humans. Endocrinology (2000) 141:4255–61. doi:10.1210/en.141.11.4255
23. Kanamoto N, Akamizu T, Tagami T, Hataya Y, Moriyama K, Takaya K, et al. Genomic structure and characterization of the 5’-flanking region of the human ghrelin gene. Endocrinology (2004) 145:4144–53. doi:10.1210/en.2003-1718
24. Seim I, Collet C, Herington AC, Chopin LK. Revised genomic structure of the human ghrelin gene and identification of novel exons, alternative splice variants and natural antisense transcripts. BMC Genomics (2007) 8:298. doi:10.1186/1471-2164-8-298
25. Nakai N, Kaneko M, Nakao N, Fujikawa T, Nakashima K, Ogata M, et al. Identification of promoter region of ghrelin gene in human medullary thyroid carcinoma cell line. Life Sci (2004) 75:2193–201. doi:10.1016/j.lfs.2004.04.028
26. Seim I, Jeffery PL, de Amorim L, Walpole CM, Fung J, Whiteside EJ, et al. Ghrelin O-acyltransferase (GOAT) is expressed in prostate cancer tissues and cell lines and expression is differentially regulated in vitro by ghrelin. Reprod Biol Endocrinol (2013) 11:70. doi:10.1186/1477-7827-11-70
27. Gutierrez JA, Solenberg PJ, Perkins DR, Willency JA, Knierman MD, Jin Z, et al. Ghrelin octanoylation mediated by an orphan lipid transferase. Proc Natl Acad Sci U S A (2008) 105:6320–5. doi:10.1073/pnas.0800708105
28. Yang J, Brown MS, Liang G, Grishin NV, Goldstein JL. Identification of the acyltransferase that octanoylates ghrelin, an appetite-stimulating peptide hormone. Cell (2008) 132:387–96. doi:10.1016/j.cell.2008.01.017
29. Zhu X, Cao Y, Voogd K, Steiner DF. On the processing of proghrelin to ghrelin. J Biol Chem (2006) 281:38867–70. doi:10.1074/jbc.M607955200
30. Frago LM, Baquedano E, Argente J, Chowen JA. Neuroprotective actions of ghrelin and growth hormone secretagogues. Front Mol Neurosci (2011) 4:23. doi:10.3389/fnmol.2011.00023
31. Kojima M, Kangawa K. Ghrelin: structure and function. Physiol Rev (2005) 85:495–522. doi:10.1152/physrev.00012.2004
32. Cowley MA, Smith RG, Diano S, Tschop M, Pronchuk N, Grove KL, et al. The distribution and mechanism of action of ghrelin in the CNS demonstrates a novel hypothalamic circuit regulating energy homeostasis. Neuron (2003) 37:649–61. doi:10.1016/S0896-6273(03)00063-1
33. Zhao TJ, Liang G, LI RL, Xie X, Sleeman MW, Murphy AJ, et al. Ghrelin O-acyltransferase (GOAT) is essential for growth hormone-mediated survival of calorie-restricted mice. Proc Natl Acad Sci U S A (2010) 107:7467–72. doi:10.1073/pnas.1002271107
34. Delhanty PJ, Neggers SJ, van der Lely AJ. Mechanisms in endocrinology: ghrelin: the differences between acyl- and des-acyl ghrelin. Eur J Endocrinol (2012) 167:601–8. doi:10.1530/EJE-12-0456
35. Callaghan B, Furness JB. Novel and conventional receptors for ghrelin, desacyl-ghrelin, and pharmacologically related compounds. Pharmacol Rev (2014) 66:984–1001. doi:10.1124/pr.113.008433
36. Tschop M, Weyer C, Tataranni PA, Devanarayan V, Ravussin E, Heiman ML. Circulating ghrelin levels are decreased in human obesity. Diabetes (2001) 50:707–9. doi:10.2337/diabetes.50.4.707
37. Shiiya T, Nakazato M, Mizuta M, Date Y, Mondal MS, Tanaka M, et al. Plasma ghrelin levels in lean and obese humans and the effect of glucose on ghrelin secretion. J Clin Endocrinol Metab (2002) 87:240–4. doi:10.1210/jcem.87.1.8129
38. le Roux CW, Patterson M, Vincent RP, Hunt C, Ghatei MA, Bloom SR. Postprandial plasma ghrelin is suppressed proportional to meal calorie content in normal-weight but not obese subjects. J Clin Endocrinol Metab (2005) 90:1068–71. doi:10.1210/jc.2004-1216
39. Stock S, Leichner P, Wong AC, Ghatei MA, Kieffer TJ, Bloom SR, et al. Ghrelin, peptide YY, glucose-dependent insulinotropic polypeptide, and hunger responses to a mixed meal in anorexic, obese, and control female adolescents. J Clin Endocrinol Metab (2005) 90:2161–8. doi:10.1210/jc.2004-1251
40. Mittelman SD, Klier K, Braun S, Azen C, Geffner ME, Buchanan TA. Obese adolescents show impaired meal responses of the appetite-regulating hormones ghrelin and PYY. Obesity (Silver Spring) (2010) 18:918–25. doi:10.1038/oby.2009.499
41. Gauna C, Delhanty PJ, Hofland LJ, Janssen JA, Broglio F, Ross RJ, et al. Ghrelin stimulates, whereas des-octanoyl ghrelin inhibits, glucose output by primary hepatocytes. J Clin Endocrinol Metab (2005) 90:1055–60. doi:10.1210/jc.2004-1069
42. Allas S, Delale T, Ngo N, Julien M, Sahakian P, Ritter J, et al. Safety, tolerability, pharmacokinetics and pharmacodynamics of AZP-531, a first-in-class analogue of unacylated ghrelin, in healthy and overweight/obese subjects and subjects with type 2 diabetes. Diabetes Obes Metab (2016) 18:868–74. doi:10.1111/dom.12675
43. Morris PG, Hudis CA, Giri D, Morrow M, Falcone DJ, Zhou XK, et al. Inflammation and increased aromatase expression occur in the breast tissue of obese women with breast cancer. Cancer Prev Res (Phila) (2011) 4:1021–9. doi:10.1158/1940-6207.CAPR-11-0110
44. Subbaramaiah K, Howe LR, Bhardwaj P, DU B, Gravaghi C, Yantiss RK, et al. Obesity is associated with inflammation and elevated aromatase expression in the mouse mammary gland. Cancer Prev Res (Phila) (2011) 4:329–46. doi:10.1158/1940-6207.CAPR-10-0381
45. Waseem T, Duxbury M, Ito H, Ashley SW, Robinson MK. Exogenous ghrelin modulates release of pro-inflammatory and anti-inflammatory cytokines in LPS-stimulated macrophages through distinct signaling pathways. Surgery (2008) 143:334–42. doi:10.1016/j.surg.2007.09.039
46. Sever S, White DL, Garcia JM. Is there an effect of ghrelin/ghrelin analogs on cancer? A systematic review. Endocr Relat Cancer (2016) 23:R393–409. doi:10.1530/ERC-16-0130
47. Cassoni P, Papotti M, Ghe C, Catapano F, Sapino A, Graziani A, et al. Identification, characterization, and biological activity of specific receptors for natural (ghrelin) and synthetic growth hormone secretagogues and analogs in human breast carcinomas and cell lines. J Clin Endocrinol Metab (2001) 86:1738–45. doi:10.1210/jcem.86.4.7402
48. Markowska A, Ziolkowska A, Jaszczynska-Nowinka K, Madry R, Malendowicz LK. Elevated blood plasma concentrations of active ghrelin and obestatin in benign ovarian neoplasms and ovarian cancers. Eur J Gynaecol Oncol (2009) 30:518–22.
49. Mungan NA, Eminferzane S, Mungan AG, Yesilli C, Seckiner I, Can M, et al. Diagnostic value of serum ghrelin levels in prostate cancer. Urol Int (2008) 80:245–8. doi:10.1159/000127334
50. Malendowicz W, Ziolkowska A, Szyszka M, Kwias Z. Elevated blood active ghrelin and unaltered total ghrelin and obestatin concentrations in prostate carcinoma. Urol Int (2009) 83:471–5. doi:10.1159/000251190
51. Russo F, Linsalata M, Clemente C, D’attoma B, Orlando A, Campanella G, et al. The effects of fluorouracil, epirubicin, and cyclophosphamide (FEC60) on the intestinal barrier function and gut peptides in breast cancer patients: an observational study. BMC Cancer (2013) 13:56. doi:10.1186/1471-2407-13-56
52. Nikander E, Tiitinen A, Laitinen K, Tikkanen M, Ylikorkala O. Effects of isolated isoflavonoids on lipids, lipoproteins, insulin sensitivity, and ghrelin in postmenopausal women. J Clin Endocrinol Metab (2004) 89:3567–72. doi:10.1210/jc.2003-032229
53. Dossus L, Mckay JD, Canzian F, Wilkening S, Rinaldi S, Biessy C, et al. Polymorphisms of genes coding for ghrelin and its receptor in relation to anthropometry, circulating levels of IGF-I and IGFBP-3, and breast cancer risk: a case-control study nested within the European Prospective Investigation into Cancer and Nutrition (EPIC). Carcinogenesis (2008) 29:1360–6. doi:10.1093/carcin/bgn083
54. Pabalan NA, Seim I, Jarjanazi H, Chopin LK. Associations between ghrelin and ghrelin receptor polymorphisms and cancer in Caucasian populations: a meta-analysis. BMC Genet (2014) 15:118. doi:10.1186/s12863-014-0118-3
55. Wagner K, Hemminki K, Grzybowska E, Klaes R, Burwinkel B, Bugert P, et al. Polymorphisms in genes involved in GH1 release and their association with breast cancer risk. Carcinogenesis (2006) 27:1867–75. doi:10.1093/carcin/bgl036
56. Feigelson HS, Teras LR, Diver WR, Tang W, Patel AV, Stevens VL, et al. Genetic variation in candidate obesity genes ADRB2, ADRB3, Ghrl, HSD11B1, IRS1, IRS2, and SHC1 and risk for breast cancer in the Cancer Prevention Study II. Breast Cancer Res (2008) 10:R57. doi:10.1186/bcr2114
57. Slattery ML, Lundgreen A, Hines L, Wolff RK, Torres-Mejia G, Baumgartner KN, et al. Energy homeostasis genes and breast cancer risk: the influence of ancestry, body size, and menopausal status, the breast cancer health disparities study. Cancer Epidemiol (2015) 39:1113–22. doi:10.1016/j.canep.2015.08.012
58. Pellatt AJ, Lundgreen A, Wolff RK, Hines L, John EM, Slattery ML. Energy homeostasis genes and survival after breast cancer diagnosis: the Breast Cancer Health Disparities Study. Cancer Causes Control (2016) 27:47–57. doi:10.1007/s10552-015-0681-6
59. Fung JN, Seim I, Wang D, Obermair A, Chopin LK, Chen C. Expression and in vitro functions of the ghrelin axis in endometrial cancer. Horm Cancer (2010) 1:245–55. doi:10.1007/s12672-010-0047-1
60. Jeffery PL, Herington AC, Chopin LK. Expression and action of the growth hormone releasing peptide ghrelin and its receptor in prostate cancer cell lines. J Endocrinol (2002) 172:R7–11. doi:10.1677/joe.0.172R007
61. Yeh AH, Jeffery PL, Duncan RP, Herington AC, Chopin LK. Ghrelin and a novel preproghrelin isoform are highly expressed in prostate cancer and ghrelin activates mitogen-activated protein kinase in prostate cancer. Clin Cancer Res (2005) 11:8295–303. doi:10.1158/1078-0432.CCR-05-0443
62. Jeffery PL, Murray RE, Yeh AH, Mcnamara JF, Duncan RP, Francis GD, et al. Expression and function of the ghrelin axis, including a novel preproghrelin isoform, in human breast cancer tissues and cell lines. Endocr Relat Cancer (2005) 12:839–50. doi:10.1677/erc.1.00984
63. Cassoni P, Allia E, Marrocco T, Ghe C, Ghigo E, Muccioli G, et al. Ghrelin and cortistatin in lung cancer: expression of peptides and related receptors in human primary tumors and in vitro effect on the H345 small cell carcinoma cell line. J Endocrinol Invest (2006) 29:781–90. doi:10.1007/BF03347371
64. Diaz-Lezama N, Hernandez-Elvira M, Sandoval A, Monroy A, Felix R, Monjaraz E. Ghrelin inhibits proliferation and increases T-type Ca2+ channel expression in PC-3 human prostate carcinoma cells. Biochem Biophys Res Commun (2010) 403:24–9. doi:10.1016/j.bbrc.2010.10.100
65. Cassoni P, Ghe C, Marrocco T, Tarabra E, Allia E, Catapano F, et al. Expression of ghrelin and biological activity of specific receptors for ghrelin and des-acyl ghrelin in human prostate neoplasms and related cell lines. Eur J Endocrinol (2004) 150:173–84. doi:10.1530/eje.0.1500173
66. Chambers AF. MDA-MB-435 and M14 cell lines: identical but not M14 melanoma? Cancer Res (2009) 69:5292–3. doi:10.1158/0008-5472.CAN-09-1528
67. Gronberg M, Fjallskog ML, Jirstrom K, Janson ET. Expression of ghrelin is correlated to a favorable outcome in invasive breast cancer. Acta Oncol (2012) 51:386–93. doi:10.3109/0284186X.2011.631576
68. Gahete MD, Cordoba-Chacon J, Hergueta-Redondo M, Martinez-Fuentes AJ, Kineman RD, Moreno-Bueno G, et al. A novel human ghrelin variant (In1-ghrelin) and ghrelin-O-acyltransferase are overexpressed in breast cancer: potential pathophysiological relevance. PLoS One (2011) 6:e23302. doi:10.1371/journal.pone.0023302
69. Seim I, Lubik AA, Lehman ML, Tomlinson N, Whiteside EJ, Herington AC, et al. Cloning of a novel insulin-regulated ghrelin transcript in prostate cancer. J Mol Endocrinol (2013) 50:179–91. doi:10.1530/JME-12-0150
70. Takahashi K, Furukawa C, Takano A, Ishikawa N, Kato T, Hayama S, et al. The neuromedin U-growth hormone secretagogue receptor 1b/neurotensin receptor 1 oncogenic signaling pathway as a therapeutic target for lung cancer. Cancer Res (2006) 66:9408–19. doi:10.1158/0008-5472.CAN-06-1349
71. De Vriese C, Gregoire F, de Neef P, Robberecht P, Delporte C. Ghrelin is produced by the human erythroleukemic HEL cell line and involved in an autocrine pathway leading to cell proliferation. Endocrinology (2005) 146:1514–22. doi:10.1210/en.2004-0964
72. Waseem T, Javaid-Ur R, Ahmad F, Azam M, Qureshi MA. Role of ghrelin axis in colorectal cancer: a novel association. Peptides (2008) 29:1369–76. doi:10.1016/j.peptides.2008.03.020
73. Carraro G, Albertin G, Abudukerimu A, Aragona F, Nussdorfer GG. Growth hormone secretagogue receptor subtypes 1a and 1b are expressed in the human adrenal cortex. Int J Mol Med (2004) 13:295–8.
74. Barzon L, Pacenti M, Masi G, Stefani AL, Fincati K, Palu G. Loss of growth hormone secretagogue receptor 1a and overexpression of type 1b receptor transcripts in human adrenocortical tumors. Oncology (2005) 68:414–21. doi:10.1159/000086983
75. Pazos Y, Alvarez CJ, Camina JP, Casanueva FF. Stimulation of extracellular signal-regulated kinases and proliferation in the human gastric cancer cells KATO-III by obestatin. Growth Factors (2007) 25:373–81. doi:10.1080/08977190801889313
76. Alvarez CJ, Lodeiro M, Theodoropoulou M, Camina JP, Casanueva FF, Pazos Y. Obestatin stimulates Akt signalling in gastric cancer cells through beta-arrestin-mediated epidermal growth factor receptor transactivation. Endocr Relat Cancer (2009) 16:599–611. doi:10.1677/ERC-08-0192
77. Alen BO, Leal-Lopez S, Alen MO, Viano P, Garcia-Castro V, Mosteiro CS, et al. The role of the obestatin/GPR39 system in human gastric adenocarcinomas. Oncotarget (2016) 7:5957–71. doi:10.18632/oncotarget.6718
78. Volante M, Rosas R, Ceppi P, Rapa I, Cassoni P, Wiedenmann B, et al. Obestatin in human neuroendocrine tissues and tumours: expression and effect on tumour growth. J Pathol (2009) 218:458–66. doi:10.1002/path.2551
Keywords: obesity, breast cancer, estrogen, aromatase, ghrelin, des-acyl ghrelin
Citation: Au CC, Furness JB and Brown KA (2017) Ghrelin and Breast Cancer: Emerging Roles in Obesity, Estrogen Regulation, and Cancer. Front. Oncol. 6:265. doi: 10.3389/fonc.2016.00265
Received: 26 September 2016; Accepted: 12 December 2016;
Published: 09 January 2017
Edited by:
Robin Anderson, Peter MacCallum Cancer Centre, AustraliaReviewed by:
Katie L. Powell, University of Sydney, AustraliaTakayuki Ueno, Kyorin University, Japan
Copyright: © 2017 Au, Furness and Brown. This is an open-access article distributed under the terms of the Creative Commons Attribution License (CC BY). The use, distribution or reproduction in other forums is permitted, provided the original author(s) or licensor are credited and that the original publication in this journal is cited, in accordance with accepted academic practice. No use, distribution or reproduction is permitted which does not comply with these terms.
*Correspondence: Kristy A. Brown, a3Jpc3R5LmJyb3duJiN4MDAwNDA7aHVkc29uLm9yZy5hdQ==