- 1Department of Pathology, Stony Brook University, Stony Brook, NY, USA
- 2Department of Chemical Engineering, Faculty of Engineering, Burapha University, Chonburi, Thailand
- 3Department of Radiologic Technology, Faculty of Associated Medical Sciences, Center of Excellence for Molecular Imaging, Chiang Mai University, Chiang Mai, Thailand
- 4StatCom, Galveston, TX, USA
Although the lung is one of the target organs at risk for cancer induction from exposure to heavy ions found in space, information is insufficient on cellular/molecular responses linked to increased cancer risk. Knowledge of such events may aid in the development of new preventive measures. Furthermore, although it is known that germinal cells are sensitive to X- or γ-rays, there is little information on the effects of heavy ions on germinal cells. Our goal was to investigate in vivo effects of 1 GeV/n 48Ti ions (one of the important heavy ions found in the space environment) on somatic (lung) and germinal (testis) tissues collected at various times after a whole body irradiation of CBA/CaJ mice (0, 0.1, 0.25, or 0.5 Gy, delivered at 1 cGy/min). We hypothesized that 48Ti-ion-exposure induced damage in both tissues. Lung tissue was collected from each mouse from each treatment group at 1 week, 1 month, and 6 months postirradiation. For the testis, we collected samples at 6 months postirradiation. Hence, only late-occurring effects of 48Ti ions in the testis were studied. There were five mice per treatment group at each harvest time. We investigated inflammatory responses after exposure to 48Ti ions by measuring the levels of activated nuclear factor kappa B and selected pro-inflammatory cytokines in both tissues of the same mouse. These measurements were coupled with the quantitation of the levels of global 5-methylcytosine (5mC) and 5-hydroxymethylcytosine (5hmC). Our data clearly showed the induction of chronic inflammation in both tissues of exposed mice. A dose-dependent reduction in global 5hmC was found in the lung at all time-points and in testes collected at 6 months postirradiation. In contrast, significant increases in global 5mC were found only in lung and testes collected at 6 months postirradiation from mice exposed to 0.5 Gy of 1 GeV/n 48Ti ions. Overall, our data showed that 48Ti ions may create health risks in both lung and testicular tissues.
Introduction
Spaceflight results in unavoidable exposure of astronauts to space radiation (such as heavy ions and energetic protons) that may create potential risks for late-occurring injuries in both somatic and germinal cells/tissues. To protect astronauts, we must improve our understanding of changes at the cellular and molecular levels that are linked to increasing astronaut health risks and are valuable in developing countermeasures. In order to obtain reliable information about radiation-induced detrimental health effects, the data must be obtained using appropriate in vivo systems because in vitro systems cannot faithfully mimic the complex in vivo situation (1). Hence, appropriate whole-animal systems are critically important surrogates for assessment of health risks associated with exposure to space radiation.
The aim of this study was to improve our knowledge of in vivo biological effects of a whole body exposure to 1 GeV/n 48Ti ions (one of the important types of heavy ions found in the space environment). We used the CBA/CaJ mouse as an experimental model to study the effects of 1 GeV/n 48Ti ions on the lung (representing somatic tissue) and the testis (representing germinal tissue) of the same mouse, employing the inflammatory responses and DNA methylation endpoints. These two endpoints have not been used to evaluate the biological effects of 1 GeV/n 48Ti ions in somatic and germinal cells of the same mouse, setting our approach apart from the existing reports.
It is known that the lung is a highly radiosensitive organ (2–4) and that impairment of the immune function in the lung is one of the major concerns after exposure to low LET radiation (4–7). It also has been suggested that the lung is one of the target organs at risk for cancer induction from exposure to heavy ions found in space (8). However, very little is known about the responses of the lung to space radiation. Recently, it was found that 350 MeV/n 28Silicon (28Si) or 56Iron (56Fe) ions, which are also important heavy ions found in the space environment, induced both histological and functional injuries in the lungs of exposed C3H/HeNCrl mice (9). Furthermore, it was reported that 1 GeV/n 56Fe ions induced lung cancer in transgenic mice (the KrasLA1 mice) engineered to be susceptible to lung cancer (10, 11).
With respect to testes, deleterious effects (e.g., DNA double-strand breaks, cytogenetic effects, and mutagenesis) of X or γ rays on spermatogenesis were reported several decades ago (12–19). It is known that the testis is one of the most radiosensitive organs (20) and is more sensitive to radiation exposure than female germ cells (21). Thus, male-mediated reproductive and developmental toxicology has been a concern for decades in atomic bomb survivors and in the Sellafield nuclear plant workers (22). However, very little is known about the effects of heavy ions on testes. It was found that exposure to 2.0–8.07 Gy of 0.35 GeV/n 12Carbon (12C) ions (LET = 13 keV/μm) or to 0.3–2.0 Gy of 1 GeV/n 56Fe ions (LET = 147 keV/μm) did not increase mutation rates (assayed by the specific locus and the dominant lethal tests in Medaka fish), as compared to those exposed to 250 kVp X rays, a reference radiation (22). However, prenatal irradiation of pregnant rats to 0.1–2.0 Gy of 0.3 GeV/n 12C or to 0.1–0.5 Gy of 0.4 MeV/n 20Neon (20Ne) ions (LET = 30 keV/μm) caused abnormal testicular development and breeding activity of male offspring (23). Further, an increased level of interleukin-1β, lower number of sperms, and an abnormal tubular architecture were found in testes of C57BL/6 mice flown with the Space Shuttle Discovery for 114 days, in relation to that of the corresponding sham controls (with no spaceflight) (24). Of note, it is known that the space environment is complex. Several factors (e.g., radiation, microgravity, and reactivation of herpes virus infection) may have contributed to such changes. Hence, to reduce the uncertainties in the assessment of health risks of space radiation, further ground-based studies are required to help improve our understanding of the effects of heavy ions on germinal cells and somatic cells as well.
Currently, there is no information on the in vivo effects of 1 GeV/n 48Ti ions to the lung and the testes. Based upon the existing, but limited, information on responses to 1 GeV/n 48Ti ions (25–27), we hypothesized that 1 GeV/n 48Ti ions induced damage to these two tissues of exposed mice. To address this hypothesis, we used two biological endpoints to evaluate the effects of 1 GeV/n 48Ti ions on lung and testicular tissues of the same mouse. These endpoints were inflammatory responses and global DNA methylation, including both 5-methylcytosine (5mC) and 5-hydroxymethylcytosine (5hmC). These two biological endpoints were chosen for analyses because they are highly relevant surrogate biomarkers for assessing health risks, but they have not previously been assessed following in vivo exposure to 1 GeV/n 48Ti ions. Importantly, the induction of chronic inflammation has been reported in studies of astronauts’ blood samples (28–30).
There has been increasing evidence of space-radiation-induced acute and chronic inflammation (26, 31–35), and radiation-induced aberrant DNA methylation at the global (26, 36) or specific locus levels (37–41). For the inflammatory responses, in this report, we chose to study the nuclear-factor kappa B (NF-κB) pathway because NF-κB is a key transcription factor playing a pivotal role in inflammatory responses to oxidative stress induced by several stimuli, including radiation (42). Although NF-κB is a member of the ubiquitously expressed family of the Rel-related transcription factors (43), only the activation of NF-κB/p65 was the focus of our study and referred to as NF-κB throughout the article. It also has been well recognized that NF-κB is a key transcription factor known to be part of a common network between inflammation and cancer (44–46), and that there is a close association between inflammation and cancer (44, 47–54). In addition, chronic inflammation in male germinal cells has been linked to male infertility (55–57). In addition to the levels of activated NF-κB, we measured the expression, at the protein levels, of selected NF-κB-regulated pro-inflammatory cytokines, i.e., tumor necrosis factor alpha (TNF-α), interleukin-1 beta (IL-1β), and interleukin 6 (IL-6). This is because their increased levels have been found in the liver (26) of the same exposed mice included in this present study. Furthermore, the expression of these proteins (at the gene level) was elevated in human mononuclear cells obtained from healthy adult individuals who lived near the Chernobyl Nuclear Power Plant and were chronically exposed to low-dose radiation ranging from 0.18 to 49 mSv (58).
Relating to DNA methylation, it has been well recognized that it is one of the key epigenetic events that plays a critical role in carcinogenesis, both initiation and promotion, in somatic and germinal cells (59, 60), and other untoward health outcomes (14) including male-mediated developmental toxicology (61), male infertility (62–64), and transgeneration effects (65, 66). Furthermore, a high level of 5mC (hypermethylation) has been linked to gene silencing (59, 67); while a reduction in global levels of 5hmC has been associated with cancer development (68). Since inflammatory responses and DNA methylation were analyzed in the lung and the testes of the same mouse, it is possible to investigate the differential sensitivity of these two tissues in the same mouse.
Materials and Methods
Animals
The CBA/CaJ mice included in this study were the same cohort that were used to investigate the effects of 1 GeV/n 48Ti ions on the liver previously reported (26), where the description of the CBA/CaJ mice, 48Ti-irradiation, and animal husbandry were presented. The experimental design of the study was approved by both the Brookhaven National Laboratory (BNL) and the Stony Brook University (SBU) Institutional Animal Care and Use Committee (IACUC). Of note, the CBA/CaJ mouse is known to be sensitive to the development of radiation-induced myeloid leukemia (ML) (69–76), liver cancer (hepatocellular carcinoma or HCC) (70, 75), and lung cancer (70).
Irradiation of Mice
Figure 1 is a diagram of the experimental design. Mice were exposed, whole-body, to average total-body doses of 0, 0.1, 0.25, or 0.5 Gy of 1 GeV/n 48Ti ions, delivered at a dose rate of 0.01 Gy/min by a 20 cm × 20 cm beam. Mice included in the sham-control group (i.e., those that were exposed to 0 Gy) were age-matched to exposed mice. Therefore, the age of mice in each treatment group would be similar at each sacrifice time. The exposure of mice was done at the National Aeronautics and Space Administration (NASA) Research Laboratory (NSRL) located at BNL. Details of the NSRL facility and irradiation procedure were previously provided (26, 34, 35, 77). We designated the first day after irradiation as day 1 after exposure. Mice were transported to the animal facility of SBU in a climate-controlled vehicle within 2 days postirradiation. Similar to the animal facility located in BNL, the animal facility of SBU, where sample collections were performed, is also approved by the Association for Assessment and Accreditation of Laboratory Animal Care (AAALAC), with the same light cycle (12 h light/12 h dark), temperature (21 ± 2°C), 10–15 hourly cycles of fresh air, and relative humidity (50 ± 10%).
Collection of the Lung and Testis
For the lung, groups of mice were used for sampling at 1 week, 1 month, and 6 months following the exposure to 1 GeV/n 48Ti ions. In contrast, the collection of the testis was done at 6 months postexposure only. Hence, at 6 months postirradiation, the lung and the testis were collected from the same mouse. The rational for choosing only one harvest time for the testis was because our goal was to study the effects of 48Ti ions on the stem-cell compartment of spermatogenesis. It is known that spermatogenesis is a complex biological process involving the transformation of spermatogonial stem cells (types A and B) into primary and secondary spermatocytes, round spermatids, and, eventually, spermatozoa over an extended period of time within seminiferous tubules of the testis (60, 65, 78). The duration of mouse spermatogenesis from the primitive type Asingle spermatogonial stem cells (SSCs) to mature sperms (spermatozoa) is about 52 days, but 35 days from differentiated spermatogonia to mature sperms (62, 79). Hence, the results obtained from the analyses of the testes collected at 6 months postirradiation reflect the effects of radiation on type Asingle SSCs.
From each treatment group, we collected the tissues from each mouse (five mice per dose of 48Ti ions). Briefly, the lung and testicular tissues were removed, rinsed three times with 1 mL phosphate buffered saline (PBS) each time to remove external contamination (i.e., blood), snap frozen in liquid nitrogen, and stored in a −80°C freezer until needed for protein extraction and further analyses. After thawing, the total lung tissue was homogenized using a Bullet Blender Homogenizer (Next Advance Inc., Averill Park, NY, USA). Likewise, after thawing, the epididymis was removed from each testis to obtain seminiferous tubules, which were homogenized for use in protein extraction. The protocols for protein extraction from the lung and the testis suggested by the manufacturer were followed. Then, the cell lysates from each tissue of each mouse were divided into two fractions, i.e., fractions A and B. Fraction A of the tissue lysate was used to extract proteins from nuclear and cytosolic samples using the method we previously described (26, 34, 35, 80, 81). The total protein obtained from the nuclear portion of the lysate suspension of the lung or the testis was used for measuring the levels of NF-κB, while the total protein obtained from the cytosolic portion was used for the measurements of NF-κB-regulated pro-inflammatory cytokines, i.e., TNF-α, IL-1β, and IL-6. Protein contents in the cytosolic portion and the nuclear portion of the lung or the testis were measured by the Bradford assay using a BioPhotometer (Eppendorf, Inc., Westbury, NY, USA). Fraction B of the tissue lysate was used to isolate DNA for the measurements of global 5mC and global 5hmC.
Measurement of Activated Nuclear Factor-Kappa B
As with our previous work (26, 34, 35, 81), we used the enzyme-linked immunosorbent assay (ELISA) NF-κB kits from Active Motif North America, Inc. for measuring the levels of activated NF-κB in the nuclear portions obtained from the lung and testis lysates. The assay was performed in duplicate wells for each lung or testis sample of each treatment group. The mean value of activated NF-κB levels for the tissue of each mouse was obtained and reported. Then, the mean value of ten measurements from five mice and the SE for each treatment group were obtained.
Measurement of NF-κB-Regulated Pro-Inflammatory Cytokines, Including Tumor Necrosis Factor-α, Interleukin-1β, and Interleukin-6
Coupled with the levels of activated NF-κB, we measured the expression (at the protein levels) of NF-κB-regulated pro-inflammatory cytokines, i.e., TNF-α, IL-1β, and IL-6. The rational for studying these pro-inflammatory cytokines has been presented in the Section “Introduction.” We applied the methods routinely used in our laboratory for measuring the expression levels of these selected cytokines in lung or testicular cell suspensions (the cytosolic portions) using the specific ELISA kits for TNF-α, IL-1β, and IL-6 from Biosource (Invitrogen, Carlsbad, CA, USA) (26, 34, 35, 82). The mean value and SE of each cytokine for each treatment group were calculated from the means of five mice.
Measurement of Global 5-Methylcytosine and 5-Hydroxymethylcytosine
The methods for DNA isolation from mouse tissues have previously been presented (26, 36). Commercially available ELISA kits for the detection of global 5mC and 5hmC (Zymo Research, Inc., Irvine, CA, USA) were used to measure the percentage of global 5mC and 5hmC in the DNA samples isolated from lung or testicular tissues. The levels of global 5mC and 5hmC were measured using a microplate spectrophotometer (Molecular Devices) at 405 nm. The % 5mC and % 5hmC was calculated from a standard curve generated using the control DNA set provided by the manufacturer. The measurements of 5mC and 5hmC in the DNA sample from each tissue of each mouse were done in duplicate (using 200 ng of DNA per well). Then, the mean value of global 5mC and global 5hmC for each mouse were obtained. Finally, the mean value of ten measurements and SE of global 5mC and global 5hmC for each treatment group were calculated from the means of five mice.
Statistical Analyses
We expressed levels of each biological endpoint as mean ± SE. For each tissue, the mean value for each assay of each mouse was used as a single datum point for statistical analyses. At each harvest time, an ANOVA method appropriate for a one-factor experiment (i.e., dose of 1 GeV/n 48Ti ions) was used to assess the significance of the radiation dose. Further, the Student’s t-test was used, independently, to evaluate statistical differences in the mean values between each exposed group and the corresponding sham-control group. A P-value of ≤0.05 was considered as statistically significant.
Results
Figures 2–7 show the effects of various doses of 1 GeV/n 48Ti ions on the lung and testicular tissues of exposed CBA/CaJ mice. P values (Student’s t-test) shown in each figure indicate statistically significant levels between exposed and sham-control groups. Tables 1 and 2 show the results of the ANOVA for the lung and testicular tissues, respectively.

Figure 2. Levels of activated NF-ĸB (±SE) in lung tissues collected at 1 week (A), 1 month (B), 6 months (C), and in testicular tissues collected at 6 months (D) from CBA/CaJ mice after a whole body exposure to various doses of 1 GeV/n 48Ti ions. P values (Student’s t-test) indicate significant differences in the levels of NF-κB between exposed and corresponding sham control groups.

Figure 3. Levels of TNF-α (±SE) in lung tissues collected at 1 week (A), 1 month (B), 6 months (C), and in testicular tissues collected at 6 months (D) from CBA/CaJ mice after a whole body exposure to various doses of 1 GeV/n 48Ti ions. P values (Student’s t-test) indicate significant differences in the levels of TNF-α between exposed and corresponding sham control groups.

Figure 4. Levels of IL-1β (±SE) in lung tissues collected at 1 week (A), 1 month (B), 6 months (C), and in testicular tissues collected at 6 months (D) from CBA/CaJ mice after a whole body exposure to various doses of 1 GeV/n 48Ti ions. P values (Student’s t-test) indicate significant differences in the levels of IL-1β between exposed and corresponding sham control groups.

Figure 5. Levels of IL-6 (±SE) in lung tissues collected at 1 week (A), 1 month (B), 6 months (C), and in testicular tissues collected at 6 months (D) from CBA/CaJ mice after a whole body exposure to various doses of 1 GeV/n 48Ti ions. P values (Student’s t-test) indicate significant differences in the levels of IL-6 between exposed and corresponding sham control groups.

Figure 6. Levels of 5mC (±SE) in lung tissues collected at 1 week (A), 1 month (B), 6 months (C), and in testicular tissues collected at 6 months (D) from CBA/CaJ mice after a whole body exposure to various doses of 1 GeV/n 48Ti ions. P values (Student’s t-test) indicate significant differences in the levels of 5mC between exposed and corresponding sham control groups.

Figure 7. Levels of 5hmC (±SE) in lung tissues collected at 1 week (A), 1 month (B), 6 months (C), and in testicular tissues collected at 6 months (D) from CBA/CaJ mice after a whole body exposure to various doses of 1 GeV/n 48Ti ions. P values (Student’s t-test) indicate significant differences in the levels of 5hmC between exposed and corresponding sham control groups.
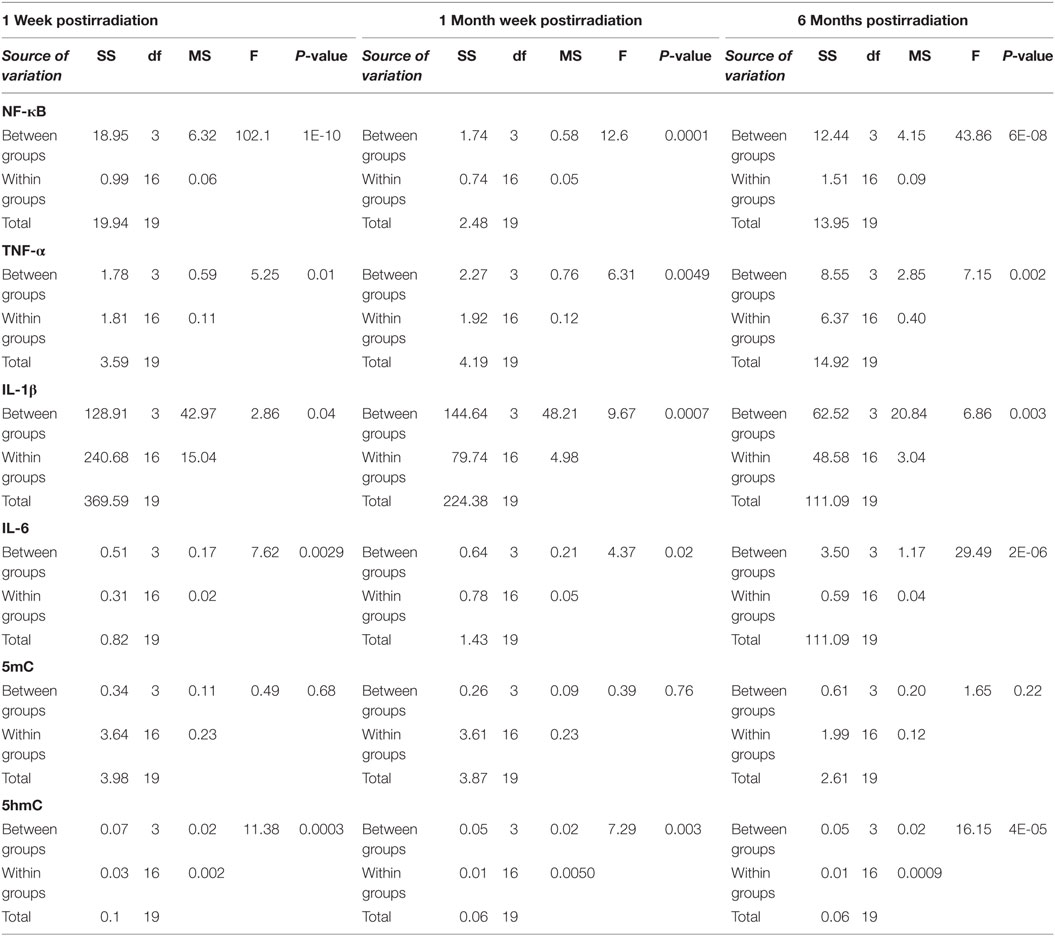
Table 1. Analysis of variance results for lung tissues collected at 1 week, 1 month, and 6 months postirradiation, respectively (SS, sum of squares; df, degree of freedom; MS, mean of squares; F, F-statistic).
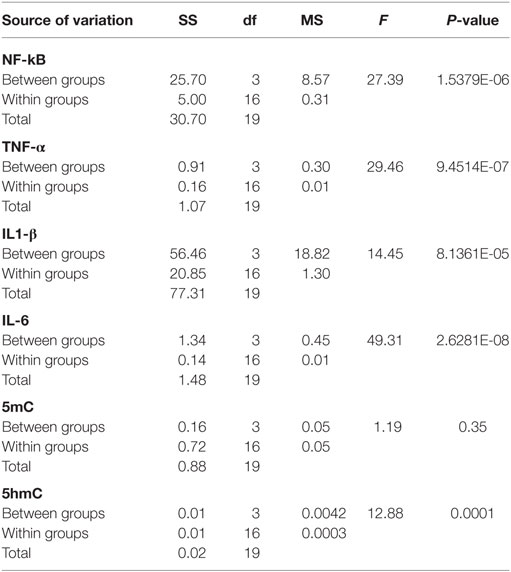
Table 2. Analysis of variance results for testicular tissues collected at 6 months postirradiation (SS, sum of squares; df, degree of freedom; MS, mean of squares; F, F-statistic).
Activated Nuclear Factor-Kappa B
There were dose-dependent increases in the levels of activated NF-κB in lung tissues from all exposed groups (ANOVA, P < 0.01), regardless of the harvest time (Figures 2A–C). Of note, there is a fluctuation in the levels of activated NF-κB in lung tissues collected from sham control mice (in particular in those collected at 1 month postirradiation). However, the factors contributing to this temporal change are unknown. We also detected a dose-dependent increase in the levels of activated NF-κB in the testicular tissues (ANOVA, P < 0.01) at 6 months postirradiation (as shown in Figure 2D).
Tumor Necrosis Factor-α
Similar to the levels of activated NF-κB, there were dose-dependent increases in the level of TNF-α in lung tissues collected at all time-points (ANOVA, P < 0.01), as shown in Figures 3A–C. Likewise, at 6 months postirradiation, a dose-dependent increase (ANOVA, P < 0.01) in the levels of IL-6 was found in testicular tissues collected at 6 months postirradiation (Figure 3D).
Interleukin-1β and Interleukin-6
Clearly, dose-dependent increases in the levels of IL-1β (Figures 4A–C) and IL-6 (Figures 5A–C) were observed in lung tissues collected at 1 week, 1 month, and 6 months postirradiation (ANOVA, P < 0.01), respectively. Of note, similar to activated NF-κB, there was a fluctuation in the levels of IL-1β in lung tissues of sham control mice, in particular in those collected at 1 month postirradiation. The cause of such fluctuation remains unidentified. Likewise, dose-dependent increases in the levels of IL-1β (Figure 4D) and IL-6 (Figure 5D) in testicular tissues collected at 6 months postirradiation (ANOVA, P < 0.01) were evident.
5 -Methylcytosine and 5-Hydroxymethylcytosine
Figures 6A–C show the effects of 48Ti ions on the levels of global 5mC in lung tissues of exposed mice. There was a trend of increased levels of global 5mC in the lung tissues of exposed mice, in relation to those of the corresponding sham controls. However, such increases were not statistically different, except in the lung tissues collected at 6 months from mice exposed to the highest dose of 1 GeV/n 48Ti ions. A similar finding was found in testicular tissues collected at 6 months postirradiation (Figure 6D).
In contrast, Figures 7A–C show significant dose-dependent decreases in the levels of 5hmC in the lungs of exposed mice (ANOVA, P < 0.05) at 1 week, 1 month, and 6 months, respectively. The decreases in 5hmC levels in lung tissues of exposed mice relative to the corresponding sham controls were: 1.33-, 1.48-, and 1.88-fold at 1 week postirradiation; 1.29-, 1.58-, and 2.29-fold at 1 month postirradiation; 1.06-, 1.30-, and 1.38-fold at 6 months postirradiation. Likewise, there was a dose-dependent reduction in the levels of global 5hmC in testicular tissues collected at 6 months postirradiation as shown in Figure 7D.
Discussion
Our data are the first to report the presence of chronic inflammation and altered levels of global 5hmC in the lung and testicular tissues of CBA/CaJ mice after a whole body exposure to 1 GeV/n 48Ti ions at low doses and a low dose-rate relevant to what is found in space, i.e., 0.1–0.5 Gy (delivered at 0.01 Gy/min). Our data also indicated that only 0.5 Gy (the highest dose used in our study) of 1 GeV/n 48Ti ions induced significant increases in the levels of global 5mC in both tissues of the same mouse. The magnitude of the effects of 48Ti ions on each tissue is similar. Since these two endpoints were detected in both tissues of the same mouse, it is plausible to speculate that there is a connection between chronic inflammation and altered DNA methylation. The information obtained from our study is important because these two in vivo endpoints are the hallmarks of cancer (52, 53, 68) and several types of male germ-cell disturbance (55–57, 62, 63, 83, 84). Hence, our findings provide an important foundation for future studies in which an association between molecular changes and the histopathological, pathological and/or functional damage in the lung and the testes, including the incidence of lung or testicular cancer, can be achieved. Of note, in future studies, it is important to measure the levels of activated NF-ĸB and related pro-inflammatory cytokines not only in tissues but also in plasma obtained from the same mice. The obtained information will help to determine whether there is a correlation between chronic inflammation in tissues and the levels of circulating cytokines, which should have clinical implications.
The approach we used in this study has allowed the investigation of the kinetics of effects of 1 GeV/n 48Ti ions on the lung, not only as a function of radiation dose but also time after exposure, since lung tissues were collected at various times up to 6 months postirradiation. We observed dose- and time-dependent increases in the levels of activated NF-κB and expression of NF-κB-related pro-inflammatory cytokines (i.e., TNF-α, IL-1β, and IL-6). Our data indicate that 48Ti-ion-exposure induces disturbances of cytokine production, reflecting chronic inflammation and an impairment of the immune system. Relating to the kinetics of the levels of global 5mC and 5hmC in the lung, our data indicated no significant change in the levels of global 5mC, except a significant increase in lung tissues collected at 6 months postirradiation from mice exposed to 0.5 Gy of 48Ti ions. In contrast, there were significant dose-dependent decreases in the levels of global 5hmC at all harvest time-points. Such findings were similar to those detected in the liver collected from the same mouse previously reported (26). Hence, our data suggest that the loss of global 5hmC is a significant response to 48Ti-ion-irradiation, regardless of tissue type. It also is reasonable to hypothesize that chronic inflammation enhances radiation-induced loss of global 5hmC and vice versa.
Our study is the first to report the levels of global 5hmC in the lung of mice exposed to radiation. Of note, the focus of previous studies on the effects of radiation, both low and high LET, has been on specific loci of 5mC (38, 39, 41, 85–88). We included the levels of global 5hmC because it is currently recognized that a reduction in global 5hmC is a biomarker for cancer (68). Taken together, our data suggest that a reduction in the level of global 5hmC may be a better hallmark of radiation exposure than an increased level of global 5mC. In the future, it will be important to conduct studies to determine the genome-wide profiling of 5hmC/5mC to reveal the affected regions of the genome so that, in turn, the identification of affected genes will be possible.
Regarding the testes collected at 6 months from the same mouse from which the lung tissue was collected for our study, the data clearly showed that there were dose-dependent increases in the levels of activated NF-κB and expression of NF-κB-regulated pro-inflammatory cytokines (i.e., TNF-α, IL-1β, and IL-6). These findings represent the effects of 1 GeV/n 48Ti ions on the primitive type of spermatogonial stem cells (SSCs), i.e., type Asingle SSCs. The induced damage arising from exposed SSCs is highly relevant for genetic risk assessment since SSCs are capable of self-renewal and differentiation into spermatocytes and mature sperm. Hence, any induced damage in the SSC compartment, if not repaired, will be carried onto the next generation and will adversely impact self-renewal, proliferation, and differentiation. In contrast, the damage that is induced in other male germ cell stages (e.g., spermatocytes, where cell divisions, both in meiosis and mitosis, take place) will affect progenies that are conceived shortly after irradiation. Our new data are important because it has been well recognized that SSCs are responsible for long-term effects of radiation on fertility (15, 89). Further, it was suggested that inflammation in SSCs leads to failure of testicular androgen and sperm production, resulting in infertility (90), and testicular cancer (91). Thus, exposure to 1 GeV/n 48Ti ions may lead to health risks associated with the male reproductive system. At 6 months postirradiation, the effects of 1 GeV/n 48Ti ions on levels of global 5mC and 5hmC in the testes of exposed mice are similar to those found in the lung of the same mouse. As mentioned previously, altered DNA methylation plays an important role in male infertility (62–64), germ cell tumors (59, 60), and transgeneration effects (65, 78). Hence, our data provide critical information for conducting further studies to investigate the potential induction of these untoward outcomes on male germinal cells from exposure to 1 GeV/n 48Ti ions.
In summary, our results provide new information on in vivo biological responses to 48Ti ions. Our new data show that 1 GeV/n 48Ti ions (at doses ranging from 0.1 to 0.5 Gy, delivered at 0.01 Gy/min) can induce chronic inflammation, and a persistence of altered DNA methylation (at the global level) in lung and testicular tissues of exposed CBA/CaJ mice. Importantly, our findings provide an important foundation for further investigations on the genes/proteins involved in 48Ti-ion-induced chronic inflammation and altered DNA methylation. Knowing such detailed molecular markers for health risks from exposure to heavy ions would not only greatly improve radiation protection guidance for astronauts (or cancer patients receiving heavy-ion radiation therapy) but would also provide significantly valuable insight for developing biological countermeasures.
Author Contributions
KR was responsible for the study concept and design; critical revision of the manuscript for important intellectual content. WJ was responsible for acquisition of the data. MT was responsible for acquisition of the data. CG was responsible for acquisition of the data. LH was responsible for acquisition of the data. EW was responsible for statistical analyses and critical revision of the manuscript for important intellectual content.
Conflict of Interest Statement
The authors declare that the research was conducted in the absence of any commercial or financial relationships that could be construed as a potential conflict of interest.
Acknowledgments
This research was supported in part by the National Aeronautics and Space Administration (NASA) Grant # NNX11AK91G and the Department of Pathology, Stony Brook University, Stony Brook, NY, USA. We thank Dr. Peter Guida and his team for logistic support, MaryAnn Petry and her staff at Brookhaven Laboratory Animal Facilities (BLAF) for their assistance in animal handling. We also thank Drs. Adam Rusek and Michael Sivertz for dosimetry support.
References
1. Nestor C, Ottaviano R, Reinhardt D, Cruickshanks H, Mjoseng H, McPherson R, et al. Rapid reprogramming of epigenetic and transcriptional profiles in mammalian culture systems. Genome Biol (2015) 16(1):11. doi:10.1186/s13059-014-0576-y
2. Coggle JE, Lambert BE, Moores SR. Radiation effects in the lung. Environ Health Perspect (1986) 70:261–91. doi:10.1289/ehp.8670261
3. Marks LB, Yu X, Vujaskovic Z, Small W, Folz R, Anscher MS. Radiation-induced lung injury. Semin Radiat Oncol (2003) 13(3):333–45. doi:10.1016/S1053-4296(03)00034-1
4. Hill RP. Radiation effects on the respiratory system. Br J Radiol (2005) 27(Suppl 1):75–81. doi:10.1259/bjr/34124307
5. Finkelstein JN, Johnston CJ, Baggs R, Rubin P. Early alterations in extracellular matrix and transforming growth factor [beta] gene expression in mouse lung indicative of late radiation fibrosis. Int J Radiat Oncol Biol Phys (1994) 28(3):621–31. doi:10.1016/0360-3016(94)90187-2
6. Hong JH, Chiang CS, Tsao CY, Lin PY, McBride WH, Wu CJ. Rapid induction of cytokine gene expression in the lung after single and fractionated doses of radiation. Int J Radiat Biol (1999) 75(11):1421–7. doi:10.1080/095530099139287
7. Rübe CE, Wilfert F, Palm J, König J, Burdak-Rothkamm S, Liu L, et al. Irradiation induces a biphasic expression of pro-inflammatory cytokines in the lung. Strahlenther Onkol (2004) 180(7):442–8. doi:10.1007/s00066-004-1265-7
8. Cucinotta FA. Space radiation risks for astronauts on multiple international space station missions. PLoS One (2014) 9(4):e96099. doi:10.1371/journal.pone.0096099
9. Christofidou-Solomidou M, Pietrofesa RA, Arguiri E, Schweitzer KS, Berdyshev EV, McCarthy M, et al. Space radiation-associated lung injury in a murine model. Amer J Physiol Lung Cell Mol Physiol (2015) 308(5):L416–28. doi:10.1152/ajplung.00260.2014
10. Shay JW, Cucinotta FA, Sulzman FM, Coleman CN, Minna JD. From mice and men to earth and space: joint NASA-NCI workshop on lung cancer risk resulting from space and terrestrial radiation. Cancer Res (2011) 71(22):6926–9. doi:10.1158/0008-5472.CAN-11-2546
11. Kim SB, Kaisani A, Shay JW. Risk assessment of space radiation-induced invasive cancer in mouse models of lung and colorectal cancer. J Radiat Res (2014) 55(Suppl 1):i46–7. doi:10.1093/jrr/rrt149
12. van Buul PP. Dose-response relationship for radiation-induced translocations in somatic and germ cells of mice. Mutat Res (1977) 45(1):61–8. doi:10.1016/0027-5107(77)90043-4
13. Vaiserman AM, Mekhova LV, Koshel NM, Voitenko VP. Cancer incidence and mortality after low-dosage radiation exposure: epidemiological aspects. Biophysics (2011) 56(2):371–80. doi:10.1134/S000635091102031X
14. Vaiserman AM. Epigenetic programming by early-life stress: evidence from human populations. Dev Dyn (2015) 244(3):254–65. doi:10.1002/dvdy.24211
15. Hacker-Klom U. Long term effects of ionizing radiation on mouse spermatogenesis. Acta Radiol Oncol (1985) 24(4):363–7. doi:10.3109/02841868509136066
16. Hamer G, Roepers-Gajadien HL, van Duyn-Goedhart A, Gademan IS, Kal HB, van Buul PPW, et al. DNA double-strand breaks and γ-H2AX signaling in the testis. Biol Reprod (2003) 68(2):628–34. doi:10.1095/biolreprod.102.008672
17. Cordelli E, Eleuteri P, Grollino MG, Benassi B, Blandino G, Bartoleschi C, et al. Direct and delayed X-ray-induced DNA damage in male mouse germ cells. Environ Mol Mutagen (2012) 53(6):429–39. doi:10.1002/em.21703
18. Spalding JF, Wellnitz JM, Schweitzer WH. Effects of rapid massive doses of gamma-rays on the testes and germ cells of the rat. Radiat Res (1957) 7(1):65–70. doi:10.2307/3570555
19. Generoso WM, Cain KT, Cacheiro NL, Cornett CV, Gossle DG. Response of mouse spermatogonial stem cells to X-ray induction of heritable reciprocal translocations. Mutat Res (1984) 126(2):177–87. doi:10.1016/0027-5107(84)90060-5
20. Ory C, Ugolin N, Hofman P, Schlumberger M, Likhtarev IA, Chevillard S. Comparison of transcriptomic signature of post-chernobyl and postradiotherapy thyroid tumors. Thyroid (2013) 23(11):1390–400. doi:10.1089/thy.2012.0318
21. Hall EJ, editor. Radiobiology for the Radiologist. 2000 ed. Philadelphia: Lippincott Williams & Wilkins (2000).
22. Shimada A, Shima A, Nojima K, Seino Y, Setlow RB. Germ cell mutagenesis in medaka fish after exposures to high-energy cosmic ray nuclei: a human model. Proc Natl Acad Sci U S A (2005) 102(17):6063–7. doi:10.1073/pnas.0500895102
23. Verdelli C, Forno I, Vaira V, Corbetta S. Epigenetic alterations in human parathyroid tumors. Endocrine (2015) 49(2):324–32. doi:10.1007/s12020-015-0555-4
24. Masini MA, Albi E, Barmo C, Bonfiglio T, Bruni L, Canesi L, et al. The impact of long-term exposure to space environment on adult mammalian organisms: a study on mouse thyroid and testis. PLoS One (2012) 7(4):e35418. doi:10.1371/journal.pone.0035418
25. Jain MR, Li M, Chen W, Liu T, de Toledo SM, Pandey BN, et al. In vivo space radiation-induced non-targeted responses: late effects on molecular signaling in mitochondria. Curr Mol Pharmacol (2011) 4(2):106–14. doi:10.2174/1874467211104020106
26. Jangiam W, Tungjai M, Rithidech K. Induction of chronic oxidative stress, chronic inflammation and aberrant patterns of DNA methylation in the liver of titanium-exposed CBA/CaJ mice. Int J Radiat Biol (2015) 91(5):389–98. doi:10.3109/09553002.2015.1001882
27. Rabin BM, Shukitt-Hale B, Joseph JA, Carrihill-Knoll KL, Carey AN, Cheng V. Relative effectiveness of different particles and energies in disrupting behavioral performance. Radiat Environ Biophys (2007) 46(2):173–7. doi:10.1007/s00411-006-0071-2
28. Crucian BE, Stowe RP, Pierson DL, Sams CF. Immune system dysregulation following short- vs long-duration spaceflight. Aviat Space Environ Med (2008) 79(9):835–43. doi:10.3357/ASEM.2276.2008
29. Sonnenfeld G, Shearer W. Immune function during space flight. Nutrition (2002) 18(10):899–903. doi:10.1016/S0899-9007(02)00903-6
30. Crucian B, Stowe RP, Mehta S, Quiriarte H, Pierson D, Sams C. Alterations in adaptive immunity persist during long-duration spaceflight. NPJ Microgravity (2015) 1:15013. doi:10.1038/npjmgrav.2015.13
31. Gridley DS, Miller GM, Pecaut MJ. Radiation and primary response to lipopolysaccharide: bone marrow-derived cells and susceptible organs. In Vivo (2007) 21(3):453–61.
32. Gridley DS, Pecaut MJ, Nelson GA. Total-body irradiation with high-LET particles: acute and chronic effects on the immune system. Amer J Physiol Regul Integr Comp Physiol (2002) 282(3):R677–88. doi:10.1152/ajpregu.00435.2001
33. Gridley DS, Rizvi A, Makinde AY, Luo-Owen X, Mao XW, Tian J, et al. Space-relevant radiation modifies cytokine profiles, signaling proteins and Foxp3+ T cells. Int J Radiat Biol (2013) 89(1):26–35. doi:10.3109/09553002.2012.715792
34. Rithidech K, Reungpatthanaphong P, Honikel L, Rusek A, Simon S. Dose-rate effects of protons on in vivo activation of nuclear factor-kappa B and cytokines in mouse bone marrow cells. Radiat Environ Biophys (2010) 49:405–19. doi:10.1007/s00411-010-0295-z
35. Tungjai M, Whorton E, Rithidech K. Persistence of apoptosis and inflammatory responses in the heart and bone marrow of mice following whole-body exposure to 28Silicon (28Si) ions. Radiat Environ Biophys (2013) 52(3):339–50. doi:10.1007/s00411-013-0479-4
36. Rithidech KN, Honikle LM, Reungpathanaphong P, Tungjai M, Jangiam W, Whorton EB. Late-occurring chromosome aberrations and global DNA methylation in hematopoietic stem/progenitor cells of CBA/CaJ mice exposed to silicon (28Si) ions. Mutat Res (2015) 781:22–31. doi:10.1016/j.mrfmmm.2015.09.001
37. Götze S, Schumacher EC, Kordes C, Häussinger D. Epigenetic changes during hepatic stellate cell activation. PLoS One (2015) 10(6):e0128745. doi:10.1371/journal.pone.0128745
38. Lima F, Ding D, Goetz W, Yang AJ, Baulch JE. High LET 56Fe ion irradiation induces tissue-specific changes in DNA methylation in the mouse. Environ Mol Mutagen (2014) 55(3):266–77. doi:10.1002/em.21832
39. Nzabarushimana E, Miousse IR, Shao L, Chang J, Allen AR, Turner J, et al. Long-term epigenetic effects of exposure to low doses of 56Fe in the mouse lung. J Radiat Res (2014) 55(4):823–8. doi:10.1093/jrr/rru010
40. Aypar U, Morgan WF, Baulch JE. Radiation-induced epigenetic alterations after low and high LET irradiations. Mutat Res (2011) 707(1–2):24–33. doi:10.1016/j.mrfmmm.2010.12.003
41. Miousse IR, Shao L, Chang J, Feng W, Wang Y, Allen AR, et al. Exposure to low-dose 56Fe-ion radiation induces long-term epigenetic alterations in mouse bone marrow hematopoietic progenitor and stem cells. Radiat Res (2014) 182(1):92–101. doi:10.1667/RR13580.1
43. Verma IM, Stevenson JK, Schwarz EM, Van Antwerp D, Miyamoto S. Rel/NF-kappa B/I kappa B family: intimate tales of association and dissociation. Genes Develop (1995) 9(22):2723–35. doi:10.1101/gad.9.22.2723
44. Karin M, Greten FR. NF-[kappa]B: linking inflammation and immunity to cancer development and progression. Nat Rev Immunol (2005) 5(10):749–59. doi:10.1038/nri1703
45. Pikarsky E, Porat RM, Stein I, Abramovitch R, Amit S, Kasem S, et al. NF-[kappa]B functions as a tumour promoter in inflammation-associated cancer. Nature (2004) 431(7007):461–6. doi:10.1038/nature02924
46. Dolcet X, Llobet D, Pallares J, Matias-Guiu X. NF-kB in development and progression of human cancer. Virchows Archiv (2005) 446(5):475–82. doi:10.1007/s00428-005-1264-9
47. Coussens LM, Werb Z. Inflammation and cancer. Nature (2002) 420(6917):860–7. doi:10.1038/nature01322
48. Takahashi H, Ogata H, Nishigaki R, Broide DH, Karin M. Tobacco smoke promotes lung tumorigenesis by triggering IKK[beta]- and JNK1-dependent inflammation. Cancer Cell (2010) 17(1):89–97. doi:10.1016/j.ccr.2009.12.008
49. Grivennikov SI, Greten FR, Karin M. Immunity, inflammation, and cancer. Cell (2010) 140(6):883–99. doi:10.1016/j.cell.2010.01.025
50. Schetter AJ, Heegaard NHH, Harris CC. Inflammation and cancer: interweaving microRNA, free radical, cytokine and p53 pathways. Carcinogenesis (2010) 31(1):37–49. doi:10.1093/carcin/bgp272
51. Li Q, Withoff S, Verma IM. Inflammation-associated cancer: NF-[kappa]B is the lynchpin. Trends Immunol (2005) 26(6):318–25. doi:10.1016/j.it.2005.04.003
52. Colotta F, Allavena P, Sica A, Garlanda C, Mantovani A. Cancer-related inflammation, the seventh hallmark of cancer: links to genetic instability. Carcinogenesis (2009) 30(7):1073–81. doi:10.1093/carcin/bgp127
53. Hanahan D, Weinberg Robert A. Hallmarks of cancer: the next generation. Cell (2011) 144(5):646–74. doi:10.1016/j.cell.2011.02.013
54. Lee JM, Yanagawa J, Peebles KA, Sharma S, Mao JT, Dubinett SM. Inflammation in lung carcinogenesis: new targets for lung cancer chemoprevention and treatment. Crit Rev Oncol Hematol (2008) 66(3):208–17. doi:10.1016/j.critrevonc.2008.01.004
55. Eggert-Kruse W, Boit R, Rohr G, Aufenanger J, Hund M, Strowitzki T. Relationship of seminal plasma interleukin (IL)-8 and IL-6 with semen quality. Hum Reprod (2001) 16(3):517–28. doi:10.1093/humrep/16.3.517
56. Haidl F, Haidl G, Oltermann I, Allam JP. Seminal parameters of chronic male genital inflammation are associated with disturbed sperm DNA integrity. Andrologia (2015) 47(4):464–9. doi:10.1111/and.12408
57. Schuppe HC, Meinhardt A, Allam JP, Bergmann M, Weidner W, Haidl G. Chronic orchitis: a neglected cause of male infertility? Andrologia (2008) 40(2):84–91. doi:10.1111/j.1439-0272.2008.00837.x
58. Albanese J, Martens K, Karkanitsa LV, Schreyer SK, Dainiak N. Multivariate analysis of low-dose radiation-associated changes in cytokine gene expression profiles using microarray technology. Exp Hematol (2007) 35(4 Suppl 1):47–54. doi:10.1016/j.exphem.2007.01.012
59. Feinberg AP, Ohlsson R, Henikoff S. The epigenetic progenitor origin of human cancer. Nat Rev Genet (2006) 7(1):21–33. doi:10.1038/nrg1748
60. Rijlaarsdam MA, Tax DMJ, Gillis AJM, Dorssers LCJ, Koestler DC, de Ridder J, et al. Genome wide DNA methylation profiles provide clues to the origin and pathogenesis of germ cell tumors. PLoS One (2015) 10(4):e0122146. doi:10.1371/journal.pone.0122146
61. Anderson D, Schmid TE, Baumgartner A. Male-mediated developmental toxicity. Asian J Androl (2014) 16(1):81–8. doi:10.4103/1008-682X.122342
62. Cisneros FJ. DNA methylation and male infertility. Front Biosci (2004) 1(9):1189–200. doi:10.2741/1332
63. Rajender S, Avery K, Agarwal A. Epigenetics, spermatogenesis and male infertility. Mutat Res (2011) 727(3):62–71. doi:10.1016/j.mrrev.2011.04.002
64. Wang X-X, Sun B-F, Jiao J, Chong Z-C, Chen Y-S, Wang X-L, et al. Genome-wide 5-hydroxymethylcytosine modification pattern is a novel epigenetic feature of globozoospermia. Oncotarget (2015) 6(9):6535–43. doi:10.18632/oncotarget.3163
65. Soubry A, Hoyo C, Jirtle RL, Murphy SK. A paternal environmental legacy: evidence for epigenetic inheritance through the male germ line. Bioessays (2014) 36(4):359–71. doi:10.1002/bies.201300113
66. Siklenka K, Erkek S, Godmann M, Lambrot R, McGraw S, Lafleur C, et al. Disruption of histone methylation in developing sperm impairs offspring health transgenerationally. Science (2015) 350(6261):aab2006. doi:10.1126/science.aab2006
67. Bird A. DNA methylation patterns and epigenetic memory. Genes Dev (2002) 16:6–12. doi:10.1101/gad.947102
68. Pfeifer G, Kadam S, Jin S-G. 5-Hydroxymethylcytosine and its potential roles in development and cancer. Epigenet Chromatin (2013) 6(1):631–41. doi:10.1186/1756-8935-6-10
69. Bouffler SD, Breckon G, Cox R. Chromosomal mechanisms in murine radiation acute myeloid leukaemogenesis. Carcinogenesis (1996) 17(4):655–9. doi:10.1093/carcin/17.4.655
70. Cronkite EP, Bond VP. Radiation Leukemic-Prediction of Low Dose Effects. Upton: NCI Report, Contract Y01-CO 10712 (1988).
71. Peng Y, Brown N, Finnon R, Warner CL, Liu X, Genik PC, et al. Radiation leukemogenesis in mice: loss of PU.1 on chromosome 2 in CBA and C57BL/6 mice after irradiation with 1 GeV/nucleon 56Fe ions, X rays or γ rays. Part I. Experimental observations. Radiat Res (2009) 171(4):474–83. doi:10.1667/RR1547.1
72. Rithidech K, Bond VP, Cronkite EP, Thompson MH, Bullis JE. Hypermutability of mouse chromosome 2 during the development of x-ray-induced murine myeloid leukemia. Proc Natl Acad Sci U S A (1995) 92(4):1152–6. doi:10.1073/pnas.92.4.1152
73. Rithidech K, Dunn JJ, Roe BA, Gordon CR, Cronkite EP. Evidence for two commonly deleted regions on mouse chromosome 2 in gamma ray-induced acute myeloid leukemic cells. Exp Hematol (2002) 30(6):564–70. doi:10.1016/S0301-472X(02)00799-3
74. Rithidech KN, Cronkite EP, Bond VP. Advantages of the CBA mouse in leukemogenesis research. Blood Cells Mol Dis (1999) 25(1):38–45. doi:10.1006/bcmd.1999.0225
75. Weil MM, Bedford JS, Bielefeldt-Ohmann H, Ray FA, Genik PC, Ehrhart EJ, et al. Incidence of acute myeloid leukemia and hepatocellular carcinoma in mice irradiated with 1 GeV/nucleon 56Fe ions. Radiat Res (2009) 172(2):213–9. doi:10.1667/RR1648.1
76. Verbiest T, Bouffler S, Nutt SL, Badie C. PU.1 downregulation in murine radiation-induced acute myeloid leukaemia (AML): from molecular mechanism to human AML. Carcinogenesis (2015) 36(4):413–9. doi:10.1093/carcin/bgv016
77. Rithidech KN, Honikel LM, Reungpatthanaphong P, Tungjai M, Golightly M, Whorton EB. Effects of 100 MeV protons delivered at 0.5 or 1 cGy/min on the in vivo induction of early and delayed chromosomal damage. Mutat Res (2013) 756(1–2):127–40. doi:10.1016/j.mrgentox.2013.06.001
78. Kanherkar RR, Bhatia-Dey N, Csoka AB. Epigenetics across the human lifespan. Front Cell Dev Biol (2014) 2:49. doi:10.3389/fcell.2014.00049
79. Baulch JE, Aypar U, Waters KM, Yang AJ, Morgan WF. Genetic and epigenetic changes in chromosomally stable and unstable progeny of irradiated cells. PLoS One (2014) 9(9):e107722. doi:10.1371/journal.pone.0107722
80. Rithidech K, Tungjai M, Arbab E, Simon SR. Activation of NF-kappa B in bone marrow cells of BALB/cJ mice following exposure in vivo to low doses of 137Cs gamma rays. Radiat Environ Biophys (2005) 44(2):139–43. doi:10.1007/s00411-005-0004-5
81. Rithidech KN, Tungjai M, Reungpatthanaphong P, Honikel L, Simon SR. Attenuation of oxidative damage and inflammatory responses by apigenin given to mice after irradiation. Mutat Res (2012) 749(1–2):29–38. doi:10.1016/j.mrgentox.2012.08.001
82. Rithidech K, Tungjai M, Reungpatthanaphong P, Honikel L, Sanford SR. Attenuation of oxidative damage and inflammatory responses by apigenin given to mice after irradiation. Mutat Res (2012) 749(1–2):29–38. doi:10.1016/j.mrgentox.2012.08.001
83. Curley JP, Mashoodh R, Champagne FA. Epigenetics and the origins of paternal effects. Horm Behav (2011) 59(3):306–14. doi:10.1016/j.yhbeh.2010.06.018
84. Aston KI, Uren PJ, Jenkins TG, Horsager A, Cairns BR, Smith AD, et al. Aberrant sperm DNA methylation predicts male fertility status and embryo quality. Fertil Steril (2015) 104(6):1388–97.e5. doi:10.1016/j.fertnstert.2015.08.019
85. Goetz W, Morgan MNM, Baulch JE. The effect of radiation quality on genomic DNA methylation profiles in irradiated human cell lines. Radiat Res (2011) 175(5):575–87. doi:10.1667/RR2390.1
86. Koturbash I, Pogribny I, Kovalchuk O. Stable loss of global DNA methylation in the radiation-target tissue – a possible mechanism contributing to radiation carcinogenesis? Biochem Biophys Res Commun (2005) 337(2):526–33. doi:10.1016/j.bbrc.2005.09.084
87. Kovalchuk O, Burke P, Besplug J, Slovack M, Filkowski J, Pogribny I. Methylation changes in muscle and liver tissues of male and female mice exposed to acute and chronic low-dose X-ray-irradiation. Mutat Res (2004) 548(1–2):75–84. doi:10.1016/j.mrfmmm.2003.12.016
88. Pogribny I, Raiche J, Slovack M, Kovalchuk O. Dose-dependence, sex- and tissue-specificity, and persistence of radiation-induced genomic DNA methylation changes. Biochem Biophys Res Commun (2004) 320(4):1253–61. doi:10.1016/j.bbrc.2004.06.081
89. Meistrich ML. Effects of chemotherapy and radiotherapy on spermatogenesis in humans. Fertil Steril (2013) 100(5):1180–6. doi:10.1016/j.fertnstert.2013.08.010
90. Hedger MP. Toll-like receptors and signalling in spermatogenesis and testicular responses to inflammation – a perspective. J Reprod Immunol (2011) 88(2):130–41. doi:10.1016/j.jri.2011.01.010
Keywords: titanium ions, chronic inflammation, NF-ĸB, pro-inflammatory cytokines, lung, testes, 5-methylcytosine, 5-hydroxymethylcytosine
Citation: Rithidech KN, Jangiam W, Tungjai M, Gordon C, Honikel L and Whorton EB (2016) Induction of Chronic Inflammation and Altered Levels of DNA Hydroxymethylation in Somatic and Germinal Tissues of CBA/CaJ Mice Exposed to 48Ti Ions. Front. Oncol. 6:155. doi: 10.3389/fonc.2016.00155
Received: 28 October 2015; Accepted: 10 June 2016;
Published: 27 June 2016
Edited by:
Marco Durante, University of Trento, ItalyReviewed by:
Nicole Averbeck, GSI Helmholtzzentrum für Schwerionenforschung, GermanyPaula Vertino, Emory University, USA
Copyright: © 2016 Rithidech, Jangiam, Tungjai, Gordon, Honikel and Whorton. This is an open-access article distributed under the terms of the Creative Commons Attribution License (CC BY). The use, distribution or reproduction in other forums is permitted, provided the original author(s) or licensor are credited and that the original publication in this journal is cited, in accordance with accepted academic practice. No use, distribution or reproduction is permitted which does not comply with these terms.
*Correspondence: Kanokporn Noy Rithidech, a2Fub2twb3JuLnJpdGhpZGVjaCYjeDAwMDQwO3N0b255YnJvb2ttZWRpY2luZS5lZHU=