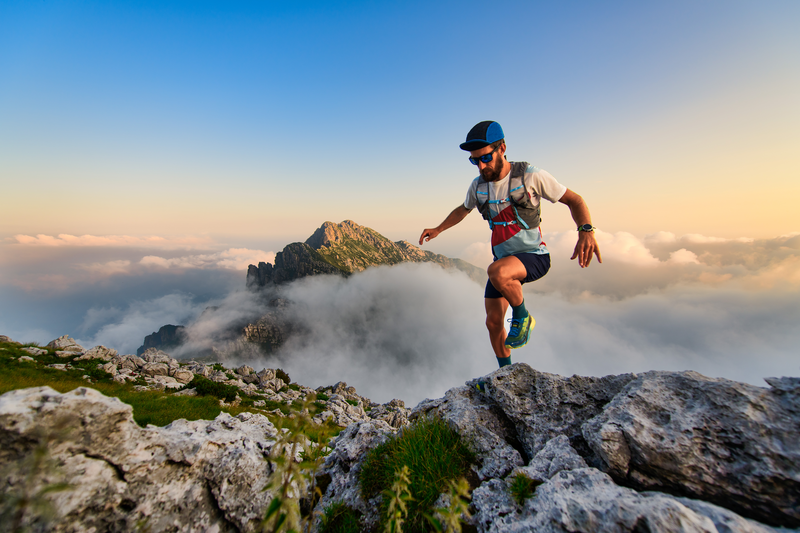
94% of researchers rate our articles as excellent or good
Learn more about the work of our research integrity team to safeguard the quality of each article we publish.
Find out more
MINI REVIEW article
Front. Oncol. , 15 April 2016
Sec. Molecular and Cellular Oncology
Volume 6 - 2016 | https://doi.org/10.3389/fonc.2016.00088
This article is part of the Research Topic Aurora kinases: classical mitotic roles, non-canonical functions and translational views View all 20 articles
Aurora A has been extensively characterized as a centrosomal kinase with essential functions during cell division including centrosome maturation and separation and spindle assembly. However, Aurora A localization is not restricted to the centrosomes and compelling evidence support the existence of specific mechanisms of activation and functions for non-centrosomal Aurora A in the dividing cell. It has been now well established that spindle assembly involves an acentrosomal RanGTP-dependent pathway that triggers microtubule assembly and organization in the proximity of the chromosomes whether centrosomes are present or not. The mechanism involves the regulation of a number of NLS-containing proteins, generically called SAFS (Spindle Assembly Factors) that exert their functions upon release from karyopherins by RanGTP. One of them, the nuclear protein TPX2 interacts with and activates Aurora A upon release from importins by RanGTP. This basic mechanism triggers the activation of Aurora A in the proximity of the chromosomes potentially translating the RanGTP signaling gradient centered on the chromosome into an Aurora A phosphorylation network. Here, we will review our current knowledge on the RanGTP-dependent TPX2 activation of Aurora A away from centrosomes: from the mechanism of activation and its functional consequences on the kinase stability and regulation to its roles in spindle assembly and cell division. We will then focus on the substrates of the TPX2-activated Aurora A having a role in microtubule nucleation, stabilization, and organization. Finally, we will briefly discuss the implications of the use of Aurora A inhibitors in anti-tumor therapies in the light of its functional interaction with TPX2.
Cell cycle progression is crucial for cell viability. During mitosis, most cellular components undergo a dramatic reorganization. In particular, the relatively stable interphase microtubule (MT) network disappears and highly dynamic MTs organize the bipolar spindle, the molecular machine that provides the support and forces for chromosome segregation. The progression and coordination of the events that drive spindle assembly and culminate with the birth of two daughter cells rely on complex regulatory networks involving several kinases. One of them is Aurora A (1), a kinase originally identified in Drosophila (2). In higher organisms, Aurora A is a member of the Aurora kinase family consisting of three serine–threonine kinases whose expression and kinase activity peak in M phase (Figure 1). Aurora kinases have essential roles during cell division and in particular in centrosome duplication and separation, spindle assembly, chromosome alignment, spindle assembly checkpoint, central spindle assembly, and cytokinesis (3–5).
Figure 1. TPX2 and Aurora A are cell cycle regulated proteins. Both proteins accumulate during G2/M and are degraded through the APC/C proteasome pathway at the end of mitosis. The relative protein levels of TPX2 (yellow triangle) and Aurora A (blue circle) in the different cell cycle phases are represented at the top. The localization of the two proteins during these cell cycle phases is represented in the drawings. In G2, TPX2 accumulates inside the nucleus whereas Aurora A accumulates at the centrosomes. During mitosis, both proteins co-localize along the spindle microtubules, and Aurora A also accumulates at the centrosomes.
The potential link between the Aurora kinases and tumor initiation and/or development has fueled the interest in understanding their function and regulation over the last years. Indeed, Aurora A gene is located in a region of chromosome 20 that is frequently overexpressed in human cancers (6, 7), and it is found in higher levels in many tumor types (8–10). Moreover, it shows oncogenic properties (3, 11, 12). Aurora A gene is also a candidate low penetrance cancer-susceptibility gene (13, 14). Aurora A is therefore considered as a potentially useful molecular therapeutic target, and several specific small molecule inhibitors are currently being tested in clinical trials (15–18).
Although the three Aurora kinases share a conserved catalytic domain, a few critical amino acid substitutions in their catalytic domains confer activator specificity. Moreover, divergent N- and C-terminal domains provide specificity at least in part through protein–protein interactions and distinct subcellular localizations during mitosis. While Aurora B and C localize to the kinetochores and the anaphase central spindle as part of the chromosomal passenger complex (19), Aurora A localizes to the centrosome throughout cell division and is often described as a centrosomal kinase (Figure 1) (20). However, Aurora A also localizes along the spindle MTs and performs essential functions unrelated to its centrosomal localization. Here, we will focus on the TPX2-dependent regulation and function of acentrosomal Aurora A during cell division.
The activity of Aurora A is regulated by phosphorylation–dephosphorylation (21, 22). In particular, the autophosphorylation of Thr288 (in humans), a residue residing within the activation loop of the catalytic domain, has been described as critical for kinase activity (11). In addition, other kinases may phosphorylate Thr288, and in vitro assays showed that PKA phosphorylates Aurora A on at least three residues, including Thr288 (11, 22). Specific anti-Phosho-Thr288 antibodies have been useful to monitor when and where Aurora A is active in tissue culture cells, revealing that the kinase is activated at the centrosomes and the spindle microtubules proximal to the poles during prometaphase and metaphase (23). However, some controversy regarding Aurora A activation has recently emerged because phosphorylation on Thr288 alone was shown to be insufficient for the kinase to adopt a fully active conformation (24). On the other hand, there is evidence that activation may occur in the absence of Thr288 phosphorylation (see below).
Aurora A activation can also be triggered through allosteric interactions with a number of proteins such as Ajuba, Bora, protein phosphatase inhibitor-2, nucleophosmin, and PAK (25–29). A specific mechanism drives Aurora A activation in a RanGTP-dependent manner in dividing cells (21, 30, 31) (Figure 2A). This mechanism involves TPX2, a cell cycle regulated nuclear protein essential for chromosome and RanGTP-dependent MT nucleation (32, 33) and bipolar spindle assembly whether centrosomes are present or not (34–36) (Figure 1). TPX2 release from importins is triggered by RanGTP in the proximity of the chromosomes and enables its interaction with Aurora A thereby promoting its local activation in a centrosome-independent manner (34).
Figure 2. (A) Schematic representation of the RanGTP-dependent interaction between TPX2 and Aurora A in the proximity of the chromosomes. The exchange factor RCC1 associated with the chromosomes generates a peak of RanGTP that releases TPX2 from the importin alpha and beta complex. TPX2 can then bind to Aurora A, promoting its autophosphorylation on Thr288 and kinase activation (blue color). The phosphatase PP1 can inactivate TPX2 free active Aurora A (mainly at the centrosome, gray color) through dephosphorylation but not the TPX2-activated Aurora A (blue color). (B) Direct consequences of the TPX2–Aurora A interaction. (C) Schematic representation of the mechanism driving acentrosomal RanGTP MT nucleation triggered by the complex TPX2–Aurora A. The TPX2–Aurora A complex associates with another specific complex containing XRHAMM-NEDD1–γ-TurC. In this macro complex the activated Aurora A phosphorylates NEDD1 at Ser405, an essential prerequisite for MT nucleation.
The interaction between TPX2 and Aurora A has several important functional consequences including the targeting of Aurora A to the spindle microtubules (37) and the assembly of spindles of the correct length that faithfully segregate chromosomes (38). Mechanistically it drives the activation of Aurora A (21, 30) through a direct interaction between the catalytic domain of Aurora A and the first 43 residues of TPX2 in humans (39 residues in Xenopus) (30, 37). Despite the high degree of conservation between the catalytic domains of Aurora A and B, the interaction between Aurora A and TPX2 is highly specific. Indeed, a single amino acid difference in the catalytic domain of Aurora B is sufficient to impair its interaction with TPX2 (39).
Structural studies showed that the binding of TPX2 to Aurora A promotes a conformational change in its catalytic domain involving the reorganization of the activation segment, providing a good binding platform for substrates (30). This also triggers Aurora A autophosphorylation at Thr288 in human cells (Thr295 in Xenopus laevis) (21) contributing to its activation (Figure 2A). Although it has been shown that TPX2 can fully activate Aurora A in the absence of Thr288 phosphorylation (40), other authors have proposed that Aurora A Thr288 phosphorylation and TPX2 binding act synergistically for the full kinase activation (41).
The conformational change induced by the binding of TPX2 to Aurora A results in the change in position of Thr288 that moves it into a buried position inaccessible to inactivating phosphatases (30). Therefore, TPX2 not only activates Aurora A but it “locks” the kinase into an active conformation that cannot be readily inactivated by PP1 like TPX2 free Aurora A (Figure 2A). Interestingly, the phosphatase PP6 was recently shown to specifically target the Aurora A–TPX2 complex triggering the dephosphorylation of the protected Thr288 thereby regulating Aurora A activity and consequently, spindle formation (42).
Finally, TPX2 protects Aurora A from degradation that occurs under normal conditions at the end of mitosis through the cdh1 activated APC/C proteasome pathway (43). Certainly, TPX2 depletion promotes a premature decrease of Aurora A levels in prometaphase (44) (Figure 2B).
Other functional implications of the TPX2–Aurora A interaction may derive from the phosphorylation of TPX2 itself. Indeed, TPX2 is a substrate of Aurora A. Xenopus Aurora A phosphorylates TPX2 on three serine residues (Ser48, Ser90, and Ser94) (21, 45). In HeLa cells Aurora A phosphorylated TPX2 was shown to control mitotic spindle length (46). However, the specific function of the Aurora A-dependent phosphorylation of TPX2 is still not entirely clear. In addition, TPX2 phosphorylation by the essential mitotic kinase polo-like kinase 1 (Plk1) was reported to increase its ability to activate Aurora A (47) while ckd1/2-dependent TPX2 phosphorylation was shown to regulate TPX2 localization impacting spindle assembly via Aurora A and Eg5 (48).
TPX2 and Aurora A both perform essential functions during cell division although not all of them are dependent on their interaction. Aurora A null mouse embryos, similar to TPX2 ablation, are embryonic lethal failing to undergo the morula-blastocyst transition due to defects in mitosis (49–51).
The functional consequences of Aurora A activation by TPX2 in the dividing cell have to be examined in the context of the function and regulation of TPX2 during cell division. In Xenopus egg extract and in mammalian cells TPX2 is essential for acentrosomal MT assembly driven by the chromosome-dependent RanGTP pathway in M phase (33, 52). In turn, this pathway is essential for the assembly of a functional spindle that can drive faithful chromosome segregation to the daughter cells (35, 53–55).
Ran cycles between an inactive GDP-bound state and an active GTP-bound state, which is controlled by regulatory proteins. The Ran exchange factor RCC1 localizes to the mitotic chromosomes whereas other factors that promote RanGTPase activity (RanGAP1 and RanBP1) are cytosolic. This promotes the formation of a RanGTP gradient centered on the chromosomes that has been directly visualized in Xenopus egg extracts (56–58) and in mammalian cells (59, 60). In the dividing cell, RanGTP provides a spatial signal that triggers MT assembly in the proximity of the chromosomes and their organization into a bipolar spindle [reviewed in Ref. (61)]. In mammalian cells the system may however be more complex since it has been shown that components of the Ran system, including RanGTP, localize to the centrosome and play an important role in MT nucleation (62–64). One essential target of the RanGTP pathway away from the centrosome is the nuclear protein TPX2. Work performed in Xenopus egg extracts showed that RanGTP promotes the dissociation of TPX2 from inhibitory interactions with importin-α/β in the vicinity of chromosomes (52, 65). This release enables the interaction of TPX2 with Aurora A leading to its activation. Therefore, it is tempting to speculate that the RanGTP gradient translates into an Aurora A-dependent phosphorylation signaling network.
Some functional implications of the TPX2-dependent interaction with and activation of Aurora A have been recently uncovered through the characterization of the mechanism underlying RanGTP-dependent acentrosomal MT nucleation in Xenopus egg extract (32). In higher eukaryotes MT nucleation is driven by the γ-tubulin ring complex (γ-TuRC), a multi-subunit complex consisting of multiple copies of γ-tubulin and a number of associated proteins named as gamma-tubulin complex proteins (GCPs) (66, 67). Together with the adaptor protein NEDD1, γ-TuRC is required for all the MT nucleation pathways described in mitosis (68, 69). Another specific requirement for the RanGTP pathway is TPX2 (65). Recently, we showed that RanGTP promotes the association of TPX2 with a XRHAMM-NEDD1– γ-TuRC complex that includes Aurora A. We also showed that within this complex the TPX2-activated Aurora A phosphorylates NEDD1 on Ser405 an essential step for RanGTP-dependent MT nucleation (32, 70) (Figure 2C).
Another RanGTP-dependent protein complex containing TPX2 and Aurora A was previously identified in Xenopus egg extract (71) and shown to be required for RanGTP-dependent MT organization. This complex includes the tetramic plus-end directed motor Eg5, XMAP215 and the RanGTP target HURP. In Xenopus egg extract and in mammalian cells, TPX2 regulates Eg5 activity through a direct interaction (72, 73). Although Aurora A phosphorylates Eg5 (74) no function for this phosphorylation in spindle formation was identified in Xenopus egg extracts (75). On the other hand, HURP is necessary for K-fiber stabilization in mammalian cells (76–78) and its phosphorylation by Aurora A is required for MT binding (79). Altogether these data suggest that some proteins may be specific substrates of the TPX2-Aurora A complex. However, further work is needed to test this idea.
The dual role of TPX2 in activating and localizing Aurora A to the spindle microtubules through an allosteric interaction is not unique. For example, besides the classical activation of the MAPK p38α by MAPKK, p38α can be activated by TAB1 [transforming growth factor-β-activated protein kinase 1 (TAK1)-binding protein 1] as well (80). The binding of TAB1 to p38α promotes its autophosphorylation and consequently, its activation. Concerning the targeting role, a similar mechanism is at play for the A-kinase anchoring proteins (AKAPs). AKAPs bind directly PKA and recruit it to specific subcellular localizations where the kinase activity is required (81). AKAPs also function as scaffold proteins to facilitate the formation of multiprotein complexes. TPX2 may also provide a scaffolding activity. It may have a critical role for the recruitment of the MT nucleation complex and NEDD1 phosphorylation by Aurora A. Similarly, it may also act as a scaffold for the HURP containing complex whose formation and function depends on Aurora A activity, and consequently on TPX2 (71).
Aurora kinases are found in a wide range of organisms from yeast to humans and they have conserved functions during cell division. TPX2 orthologs have also been identified in a variety of genera and different kingdoms. Interestingly, the tpx2 knockout mice display severe developmental defects and embryonic lethality (82) and similar phenotypes were described for a tpx2 knockout in Arabidopsis thaliana (83).
Aurora A was identified in Drosophila. However, it is only recently that Ssp1/Mei-38 was proposed to be a putative TPX2 ortholog (84). Although the effects of loss-of-function of this protein are less severe than in the case of human TPX2, Ssp1/Mei-38 shows similar localization to spindle microtubules. Moreover, it also contains a sequence conserved in human TPX2 that confers the microtubule-binding and bundling activities. However, Ssp1/Mei-38 lacks an Aurora A binding domain suggesting that it does not fulfill the same role as the vertebrate TPX2 during cell division and therefore it may not be a true TPX2 ortholog.
Caenorhabditis elegans has two Aurora-like kinases. A putative ortholog of TPX2 was recently identified and named TPX2-like protein (TPXL-1) (85). Although TPXL-1 activates and localizes Aurora A to the mitotic spindle and not to the centrosome, it actually does not share other essential features and functions of TPX2 like its RanGTP regulation and its role in microtubule nucleation (86).
These data suggest that different evolutionary modules may exist to control the localization and activation of the Aurora kinases during cell division. Vertebrates seem to have developed a unique module to integrate the control of localization and activation of Aurora A by the chromosomal RanGTP-dependent pathway through a single interacting protein, TPX2.
Aurora A and TPX2 are overexpressed in several types of tumors and have been implicated at different levels in cancer. Although the mechanism underlying the role of TPX2 and Aurora A in tumorigenesis may be at least in part independent, there are data to suggest a role for the complex. TPX2 was initially identified as a proliferation marker with a potential role in human cancer (87). It is indeed overexpressed in many tumor types (88). High levels of Aurora A were detected in many cancer types including prostate cancer, gastric carcinoma, breast carcinoma (89), ovarian cancer, laryngeal carcinoma, bladder cancer, and pancreatic carcinoma, among others (90). Moreover, both genes are part of the chromosomal instability signature that was found to predict clinical outcome for different cancers with TPX2 having the highest CIN score (91).
Interestingly, both TPX2 and Aurora A genes are located on chromosome 20q, whose amplification is found in tumors and moreover, co-expression of TPX2 and Aurora A has been observed in some tumors (92). For instance, Aurora A and TPX2 were found overexpressed in lung cancer cells (93), different colon cancers (94, 95) and neuroblastoma (96). Based on the correlation of co-expression it was in fact proposed that TPX2 and Aurora A might act as a functional unit (90). Interestingly, a mutant of Aurora A (S155R), that is unable to interact with TPX2, has been identified in colon cancer (97), suggesting that the misregulation of Aurora A localization and/or activity may also be deleterious for the cell. It is also interesting to note in this context that the tumor suppressor p53 is regulated by both TPX2 and Aurora A in Xenopus (98).
Some data suggest that the increased levels of Aurora A in various tumors may be the consequence of protein stabilization rather than gene amplification. Indeed, phosphorylation of Aurora A Ser51 inhibits its degradation via the cdh1 activated ubiquitin ligase APC/C at the end of mitosis and Aurora A constitutively phosphorylated at Ser51 was shown to be present in neck and head cancer tissues with Aurora A overexpression (99). Although no direct connection has been reported yet, it is interesting to note here that TPX2 protects Aurora A from degradation potentially contributing to the maintenance of high levels.
The clear implications of Aurora A in cancer have promoted the intensive search for small molecule inhibitors for their potential therapeutic use (100). Some of them already show interesting potential in clinical trials but a further optimization may be required. Targeting specifically the TPX2-activated Aurora A may open new strategies in cancer therapy.
All authors listed, have made substantial, direct, and intellectual contribution to the work, and approved it for publication.
The authors declare that the research was conducted in the absence of any commercial or financial relationships that could be construed as a potential conflict of interest.
We want to thank the members of Vernos lab for critical discussions on the various aspects of the RanGTP pathway.
Work in the Vernos lab was supported by the Spanish ministry grants BFU2009-10202 and BFU2012-37163. We also acknowledge support of the Spanish Ministry of Economy and Competitiveness, “Centro de Excelencia Severo Ochoa 2013-2017,” SEV-2012-0208.
γTuRC, γ-tubulin ring complex; APC/C, anaphase-promoting complex; K-Fiber, kinetochore-fiber; MAP, microtubule-associated protein; MT, microtubule; NLS, nuclear localization signal; SAF, spindle assembly factor.
1. Nigg EA. Mitotic kinases as regulators of cell division and its checkpoints. Nat Rev Mol Cell Biol (2001) 2:21–32. doi: 10.1038/35048096
2. Glover DM, Leibowitz MH, McLean DA, Parry H. Mutations in aurora prevent centrosome separation leading to the formation of monopolar spindles. Cell (1995) 81:95–105. doi:10.1016/0092-8674(95)90374-7
3. Bischoff JR, Anderson L, Zhu Y, Mossie K, Ng L, Souza B, et al. A homologue of Drosophila aurora kinase is oncogenic and amplified in human colorectal cancers. EMBO J (1998) 17:3052–65. doi:10.1093/emboj/17.11.3052
4. Lioutas A, Vernos I. Aurora A: working from dawn to dusk in mitosis. Cell Cycle (2014) 13:499–500. doi:10.4161/cc.27781
5. Vader G, Lens SMA. The Aurora kinase family in cell division and cancer. Biochim Biophys Acta (2008) 1786:60–72. doi:10.1016/j.bbcan.2008.07.003
6. Giet R, Petretti C, Prigent C. Aurora kinases, aneuploidy and cancer, a coincidence or a real link? Trends Cell Biol (2005) 15:241–50. doi:10.1016/j.tcb.2005.03.004
7. Marumoto T, Zhang D, Saya H. Aurora-A – a guardian of poles. Nat Rev Cancer (2005) 5:42–50. doi:10.1038/nrc1526
8. Katsha A, Belkhiri A, Goff L, El-Rifai W. Aurora kinase A in gastrointestinal cancers: time to target. Mol Cancer (2015) 14:106. doi:10.1186/s12943-015-0375-4
9. Mehra R, Serebriiskii IG, Burtness B, Astsaturov I, Golemis EA. Aurora kinases in head and neck cancer. Lancet Oncol (2013) 14:e425–35. doi:10.1016/S1470-2045(13)70128-1
10. Nikonova AS, Astsaturov I, Serebriiskii IG, Dunbrack RL, Golemis EA. Aurora A kinase (AURKA) in normal and pathological cell growth. Cell Mol Life Sci (2013) 70:661–87. doi:10.1007/s00018-012-1073-7
11. Littlepage LE, Wu H, Andresson T, Deanehan JK, Amundadottir LT, Ruderman JV. Identification of phosphorylated residues that affect the activity of the mitotic kinase Aurora-A. Proc Natl Acad Sci U S A (2002) 99:15440–5. doi:10.1073/pnas.202606599
12. Zhou H, Kuang J, Zhong L, Kuo WL, Gray JW, Sahin A, et al. Tumour amplified kinase STK15/BTAK induces centrosome amplification, aneuploidy and transformation. Nat Genet (1998) 20:189–93. doi:10.1038/2496
13. Ewart-Toland A, Briassouli P, de Koning JP, Mao J-H, Yuan J, Chan F, et al. Identification of Stk6/STK15 as a candidate low-penetrance tumor-susceptibility gene in mouse and human. Nat Genet (2003) 34:403–12. doi:10.1038/ng1220
14. Ewart-Toland A, Dai Q, Gao Y-T, Nagase H, Dunlop MG, Farrington SM, et al. Aurora-A/STK15 T+91A is a general low penetrance cancer susceptibility gene: a meta-analysis of multiple cancer types. Carcinogenesis (2005) 26:1368–73. doi:10.1093/carcin/bgi085
15. Falchook GS, Bastida CC, Kurzrock R. Aurora kinase inhibitors in oncology clinical trials: current state of the progress. Semin Oncol (2015) 42:832–48. doi:10.1053/j.seminoncol.2015.09.022
16. Keen N, Taylor S. Aurora-kinase inhibitors as anticancer agents. Nat Rev Cancer (2004) 4:927–36. doi:10.1038/nrc1502
17. Niu H, Manfredi M, Ecsedy JA. Scientific rationale supporting the clinical development strategy for the investigational Aurora A kinase inhibitor alisertib in cancer. Front Oncol (2015) 5:189. doi:10.3389/fonc.2015.00189
18. Sausville EA. Aurora kinases dawn as cancer drug targets. Nat Med (2004) 10:234–5. doi:10.1038/nm0304-234
19. Sasai K, Katayama H, Stenoien DL, Fujii S, Honda R, Kimura M, et al. Aurora-C kinase is a novel chromosomal passenger protein that can complement Aurora-B kinase function in mitotic cells. Cell Motil Cytoskeleton (2004) 59:249–63. doi:10.1002/cm.20039
20. Sardon T, Peset I, Petrova B, Vernos I. Dissecting the role of Aurora A during spindle assembly. EMBO J (2008) 27:2567–79. doi:10.1038/emboj.2008.224
21. Eyers PA, Erikson E, Chen LG, Maller JL. A novel mechanism for activation of the protein kinase Aurora A. Curr Biol (2003) 13:691–7. doi:10.1016/S
22. Walter AO, Seghezzi W, Korver W, Sheung J, Lees E. The mitotic serine/threonine kinase Aurora2/AIK is regulated by phosphorylation and degradation. Oncogene (2000) 19:4906–16. doi:10.1038/sj.onc.1203847
23. Ohashi S, Sakashita G, Ban R, Nagasawa M, Matsuzaki H, Murata Y, et al. Phospho-regulation of human protein kinase Aurora-A: analysis using anti-phospho-Thr288 monoclonal antibodies. Oncogene (2006) 25:7691–702. doi:10.1038/sj.onc.1209754
24. Burgess SG, Bayliss R. The structure of C290A:C393A Aurora A provides structural insights into kinase regulation. Acta Crystallogr F Struct Biol Commun (2015) 71:315–9. doi:10.1107/S2053230X15002290
25. Hirota T, Kunitoku N, Sasayama T, Marumoto T, Zhang D, Nitta M, et al. Aurora-A and an interacting activator, the LIM protein Ajuba, are required for mitotic commitment in human cells. Cell (2003) 114:585–98. doi:10.1016/S0092-8674(03)00642-1
26. Hutterer A, Berdnik D, Wirtz-Peitz F, Žigman M, Schleiffer A, Knoblich JA. Mitotic activation of the kinase Aurora-A requires its binding partner Bora. Dev Cell (2006) 11:147–57. doi:10.1016/j.devcel.2006.06.002
27. Reboutier D, Troadec M, Cremet J, Fukasawa K, Prigent C. Nucleophosmin/B23 activates Aurora A at the centrosome through phosphorylation of serine 89. J Cell Biol (2012) 197:19–26. doi:10.1083/jcb.201107134
28. Satinover DL, Leach CA, Stukenberg PT, Brautigan DL. Activation of Aurora-A kinase by protein phosphatase inhibitor-2, a bifunctional signaling protein. Proc Natl Acad Sci U S A (2004) 101:8625–30. doi:10.1073/pnas.0402966101
29. Zhao Z-S, Lim JP, Ng Y-W, Lim L, Manser E. The GIT-associated kinase PAK targets to the centrosome and regulates Aurora-A. Mol Cell (2005) 20:237–49. doi:10.1016/j.molcel.2005.08.035
30. Bayliss R, Sardon T, Vernos I, Conti E. Structural basis of Aurora-A activation by TPX2 at the mitotic spindle. Mol Cell (2003) 12:851–62. doi:10.1016/S1097-2765(03)00392-7
31. Tsai M-Y, Wiese C, Cao K, Martin OC, Donovan P, Ruderman J, et al. A Ran signalling pathway mediated by the mitotic kinase Aurora A in spindle assembly. Nat Cell Biol (2003) 5:242–8. doi:10.1038/ncb936
32. Scrofani J, Sardon T, Meunier S, Vernos I. Microtubule nucleation in mitosis by a RanGTP-dependent protein complex. Curr Biol (2015) 25:131–40. doi:10.1016/j.cub.2014.11.025
33. Tulu US, Fagerstrom C, Ferenz NP, Wadsworth P. Molecular requirements for kinetochore-associated microtubule formation in mammalian cells. Curr Biol (2006) 16:536–41. doi:10.1016/j.cub.2006.01.060
34. Brunet S, Dumont J, Lee KW, Kinoshita K, Hikal P, Gruss OJ, et al. Meiotic regulation of TPX2 protein levels governs cell cycle progression in mouse oocytes. PLoS One (2008) 3:e3338. doi:10.1371/journal.pone.0003338
35. Gruss OJ, Wittmann M, Yokoyama H, Pepperkok R, Kufer TA, Silljé HHW, et al. Chromosome-induced microtubule assembly mediated by TPX2 is required for spindle formation in HeLa cells. Nat Cell Biol (2002) 4:871–9. doi:10.1038/ncb870
36. Ma N, Titus J, Gable A, Ross JL, Wadsworth P. TPX2 regulates the localization and activity of Eg5 in the mammalian mitotic spindle. J Cell Biol (2011) 195:87–98. doi:10.1083/jcb.201106149
37. Kufer TA, Silljé HHW, Körner R, Gruss OJ, Meraldi P, Nigg EA. Human TPX2 is required for targeting Aurora-A kinase to the spindle. J Cell Biol (2002) 158:617–23. doi:10.1083/jcb.200204155
38. Bird AW, Hyman AA. Building a spindle of the correct length in human cells requires the interaction between TPX2 and Aurora A. J Cell Biol (2008) 182:289–300. doi:10.1083/jcb.200802005
39. Bayliss R, Sardon T, Ebert J, Lindner D, Vernos I, Conti E. Determinants for Aurora-A activation and Aurora-B discrimination by TPX2. Cell Cycle (2004) 3:404–7. doi:10.4161/cc.3.4.777
40. Zorba A, Buosi V, Kutter S, Kern N, Pontiggia F, Cho YJ, et al. Molecular mechanism of Aurora A kinase autophosphorylation and its allosteric activation by TPX2. Elife (2014) 2014:1–24. doi:10.7554/eLife.02667.001
41. Dodson CA, Bayliss R. Activation of Aurora-A kinase by protein partner binding and phosphorylation are independent and synergistic. J Biol Chem (2012) 287:1150–7. doi:10.1074/jbc.M111.312090
42. Zeng K, Bastos RN, Barr FA, Gruneberg U. Protein phosphatase 6 regulates mitotic spindle formation by controlling the T-loop phosphorylation state of Aurora A bound to its activator TPX2. J Cell Biol (2010) 191:1315–32. doi:10.1083/jcb.201008106
43. Carmena M, Earnshaw WC. The cellular geography of aurora kinases. Nat Rev Mol Cell Biol (2003) 4:842–54. doi:10.1038/nrm1245
44. Giubettini M, Asteriti IA, Scrofani J, De Luca M, Lindon C, Lavia P, et al. Control of Aurora-A stability through interaction with TPX2. J Cell Sci (2011) 124:113–22. doi:10.1242/jcs.075457
45. Eyers PA, Maller JL. Regulation of Xenopus Aurora A Activation by TPX2. J Biol Chem (2004) 279:9008–15. doi:10.1074/jbc.M312424200
46. Fu J, Bian M, Xin G, Deng Z, Luo J, Guo X, et al. TPX2 phosphorylation maintains metaphase spindle length by regulating microtubule flux. J Cell Biol (2015) 210:373–83. doi:10.1083/jcb.201412109
47. Eckerdt F, Pascreau G, Phistry M, Lewellyn AL, DePaoli-Roach AA, Maller JL. Phosphorylation of TPX2 by Plx1 enhances activation of Aurora A. Cell Cycle (2009) 8:2413–9. doi:10.4161/cc.8.15.9086
48. Shim SY, De Castro IP, Neumayer G, Wang J, Park SK, Sanada K, et al. Phosphorylation of targeting protein for Xenopus kinesin-like protein 2 (TPX2) at threonine 72 in spindle assembly. J Biol Chem (2015) 290:9122–34. doi:10.1074/jbc.M114.591545
49. Cowley DO, Rivera-Pérez JA, Schliekelman M, He YJ, Oliver TG, Lu L, et al. Aurora-A kinase is essential for bipolar spindle formation and early development. Mol Cell Biol (2009) 29:1059–71. doi:10.1128/MCB.01062-08
50. Lu L-Y, Wood JL, Ye L, Minter-Dykhouse K, Saunders TL, Yu X, et al. Aurora A is essential for early embryonic development and tumor suppression. J Biol Chem (2008) 283:31785–90. doi:10.1074/jbc.M805880200
51. Sasai K, Parant JM, Brandt ME, Carter J, Adams HP, Stass SA, et al. Targeted disruption of Aurora A causes abnormal mitotic spindle assembly, chromosome misalignment and embryonic lethality. Oncogene (2008) 27:4122–7. doi:10.1038/onc.2008.47
52. Gruss OJ, Carazo-Salas RE, Schatz CA, Guarguaglini G, Kast J, Wilm M, et al. Ran induces spindle assembly by reversing the inhibitory effect of importin alpha on TPX2 activity. Cell (2001) 104:83–93. doi:10.1016/S0092-8674(01)00193-3
53. Cavazza T, Vernos I. The RanGTP pathway: from nucleo-cytoplasmic transport to spindle assembly and beyond. Front Cell Dev Biol (2016) 3:82. doi:10.3389/fcell.2015.00082
54. Meunier S, Vernos I. K-fibre minus ends are stabilized by a RanGTP-dependent mechanism essential for functional spindle assembly. Nat Cell Biol (2011) 13:1406–14. doi:10.1038/ncb2372
55. Walczak CE, Heald R. Mechanisms of mitotic spindle assembly and function. Int Rev Cytol (2008) 265:111–58. doi:10.1016/S0074-7696(07)65003-7
56. Caudron M, Bunt G, Bastiaens P, Karsenti E. Spatial coordination of spindle assembly by chromosome-mediated signaling gradients. Science (2005) 309:1373–6. doi:10.1126/science.1115964
57. Clarke PR. Cell biology. A gradient signal orchestrates the mitotic spindle. Science (2005) 309:1334–5. doi:10.1126/science.1117842
58. Kalab P, Weis K, Heald R. Visualization of a Ran-GTP gradient in interphase and mitotic Xenopus egg extracts. Science (2002) 295:2452–6. doi:10.1126/science.1068798
59. Kalab P, Pralle A, Isacoff EY, Heald R, Weis K. Analysis of a RanGTP-regulated gradient in mitotic somatic cells. Nature (2006) 440:697–701. doi:10.1038/nature04589
60. Li H-Y, Zheng Y. Phosphorylation of RCC1 in mitosis is essential for producing a high RanGTP concentration on chromosomes and for spindle assembly in mammalian cells. Genes Dev (2004) 18:512–27. doi:10.1101/gad.1177304
61. Clarke PR, Zhang C. Spatial and temporal coordination of mitosis by Ran GTPase. Nat Rev Mol Cell Biol (2008) 9:464–77. doi:10.1038/nrm2410
62. Ciciarello M, Mangiacasale R, Thibier C, Guarguaglini G, Marchetti E, Di Fiore B, et al. Importin beta is transported to spindle poles during mitosis and regulates Ran-dependent spindle assembly factors in mammalian cells. J Cell Sci (2004) 117:6511–22. doi:10.1242/jcs.01569
63. Di Fiore B, Ciciarello M, Mangiacasale R, Palena A, Tassin A-M, Cundari E, et al. Mammalian RanBP1 regulates centrosome cohesion during mitosis. J Cell Sci (2003) 116:3399–411. doi:10.1242/jcs.00624
64. Lavia P. The GTPase RAN regulates multiple steps of the centrosome life cycle. Chromosome Res (2016) 24:53–65. doi:10.1007/s10577-015-9514-4
65. Gruss OJ, Vernos I. The mechanism of spindle assembly: functions of Ran and its target TPX2. J Cell Biol (2004) 166:949–55. doi:10.1083/jcb.200312112
66. Kollman JM, Merdes A, Mourey L, Agard DA. Microtubule nucleation by γ-tubulin complexes. Nat Rev Mol Cell Biol (2011) 12:709–21. doi:10.1038/nrm3209
67. Zheng Y, Wong ML, Alberts B, Mitchison TJ. Nucleation of microtubule assembly by a gamma-tubulin-containing ring complex. Nature (1995) 378:578–83. doi:10.1038/378578a0
68. Moudjou M, Bordes N, Paintrand M, Bornens M. Gamma-Tubulin in mammalian cells: the centrosomal and the cytosolic forms. J Cell Sci (1996) 109(Pt 4):875–87.
69. Teixidó-Travesa N, Roig J, Lüders J. The where, when and how of microtubule nucleation – one ring to rule them all. J Cell Sci (2012) 125:4445–56. doi:10.1242/jcs.106971
70. Pinyol R, Scrofani J, Vernos I. The role of NEDD1 phosphorylation by Aurora A in chromosomal microtubule nucleation and spindle function. Curr Biol (2013) 23:143–9. doi:10.1016/j.cub.2012.11.046
71. Koffa MD, Casanova CM, Santarella R, Köcher T, Wilm M, Mattaj IW. HURP is part of a Ran-dependent complex involved in spindle formation. Curr Biol (2006) 16:743–54. doi:10.1016/j.cub.2006.03.056
72. Eckerdt F, Eyers PA, Lewellyn AL, Prigent C, Maller JL. Spindle pole regulation by a discrete Eg5-interacting domain in TPX2. Curr Biol (2008) 18:519–25. doi:10.1016/j.cub.2008.02.077
73. Balchand SK, Mann BJ, Titus J, Ross JL, Wadsworth P. TPX2 inhibits Eg5 by interactions with both motor and microtubule. J Biol Chem (2015) 290:17367–79. doi:10.1074/jbc.M114.612903
74. Giet R, Uzbekov R, Cubizolles F, Le Guellec K, Prigent C. The Xenopus laevis aurora-related protein kinase pEg2 associates with and phosphorylates the kinesin-related protein XlEg5. J Biol Chem (1999) 274:15005–13. doi:10.1074/jbc.274.21.15005
75. Cahu J, Olichon A, Hentrich C, Schek H, Drinjakovic J, Zhang C, et al. Phosphorylation by Cdk1 increases the binding of Eg5 to microtubules in vitro and in Xenopus egg extract spindles. PLoS One (2008) 3:e3936. doi:10.1371/journal.pone.0003936
76. Santarella R, Koffa MD, Tittmann P, Gross H, Hoenger A. HURP wraps microtubule ends with an additional tubulin sheet that has a novel conformation of tubulin. J Mol Biol (2007) 365:1587–95. doi:10.1016/j.jmb.2006.10.064
77. Silljé HHW, Nagel S, Körner R, Nigg EA. HURP is a Ran-importin β-regulated protein that stabilizes kinetochore microtubules in the vicinity of chromosomes. Curr Biol (2006) 16:731–42. doi:10.1016/j.cub.2006.02.070
78. Wong J, Lerrigo R, Jang C-Y, Fang G. Aurora A regulates the activity of HURP by controlling the accessibility of its microtubule-binding domain. Mol Biol Cell (2008) 19:2083–91. doi:10.1091/mbc.E07
79. Yu C-TR, Hsu J-M, Lee Y-CG, Tsou A-P, Chou C-K, Huang CYF. Phosphorylation and stabilization of HURP by Aurora-A: implication of HURP as a transforming target of Aurora-A. Mol Cell Biol (2005) 25:5789–800. doi:10.1128/MCB.25.14.5789-5800.2005
80. Ge B, Gram H, Di Padova F, Huang B, New L, Ulevitch RJ, et al. MAPKK-independent activation of p38alpha mediated by TAB1-dependent autophosphorylation of p38alpha. Science (2002) 295:1291–4. doi:10.1126/science.1067289
81. Calejo AI, Taskén K. Targeting protein–protein interactions in complexes organized by A kinase anchoring proteins. Front Pharmacol (2015) 6:192. doi:10.3389/fphar.2015.00192
82. Aguirre-Portolés C, Bird AW, Hyman A, Cañamero M, Pérez de Castro I, Malumbres M. Tpx2 controls spindle integrity, genome stability, and tumor development. Cancer Res (2012) 72:1518–28. doi:10.1158/0008-5472.CAN-11-1971
83. Vos JW, Pieuchot L, Evrard J-L, Janski N, Bergdoll M, de Ronde D, et al. The plant TPX2 protein regulates prospindle assembly before nuclear envelope breakdown. Plant Cell (2008) 20:2783–97. doi:10.1105/tpc.107.056796
84. Goshima G. Identification of a TPX2-like microtubule-associated protein in Drosophila. PLoS One (2011) 6(11):e28120. doi:10.1371/journal.pone.0028120
85. Özlü N, Srayko M, Kinoshita K, Habermann B, O’Toole ET, Müller-Reichert T, et al. An essential function of the C. elegans ortholog of TPX2 is to localize activated Aurora A kinase to mitotic spindles. Dev Cell (2005) 9:237–48. doi:10.1016/j.devcel.2005.07.002
87. Heidebrecht HJ, Buck F, Steinmann J, Sprenger R, Wacker HH, Parwaresch R. p100: a novel proliferation-associated nuclear protein specifically restricted to cell cycle phases S, G2, and M. Blood (1997) 90:226–33.
88. Neumayer G, Belzil C, Gruss OJ, Nguyen MD. TPX2: of spindle assembly, DNA damage response, and cancer. Cell Mol Life Sci (2014) 71:3027–47. doi:10.1007/s00018-014-1582-7
89. Tanaka T, Kimura M, Matsunaga K, Fukada D, Mori H, Okano Y. Centrosomal kinase AIK1 is overexpressed in invasive ductal carcinoma of the breast. Cancer Res (1999) 59:2041–4.
90. Asteriti IA, Rensen WM, Lindon C, Lavia P, Guarguaglini G. The Aurora-A/TPX2 complex: a novel oncogenic holoenzyme? Biochim Biophys Acta (2010) 1806:230–9. doi:10.1016/j.bbcan.2010.08.001
91. Carter SL, Eklund AC, Kohane IS, Harris LN, Szallasi Z. A signature of chromosomal instability inferred from gene expression profiles predicts clinical outcome in multiple human cancers. Nat Genet (2006) 38:1043–8. doi:10.1038/ng1861
92. Pérez de Castro I, Malumbres M. Mitotic stress and chromosomal instability in cancer: the case for TPX2. Genes Cancer (2013) 3:721–30. doi:10.1177/1947601912473306
93. Kadara H, Lacroix L, Behrens C, Solis L, Gu X, Lee JJ, et al. Identification of gene signatures and molecular markers for human lung cancer prognosis using an in vitro lung carcinogenesis system. Cancer Prev Res (Phila) (2009) 2:702–11. doi:10.1158/1940-6207.CAPR-09-0084
94. Sillars-Hardebol AH, Carvalho B, Tijssen M, Beliën JAM, de Wit M, Delis-van Diemen PM, et al. TPX2 and AURKA promote 20q amplicon-driven colorectal adenoma to carcinoma progression. Gut (2012) 61:1568–75. doi:10.1136/gutjnl-2011-301153
95. Takahashi Y, Sheridan P, Niida A, Sawada G, Uchi R, Mizuno H, et al. The AURKA/TPX2 axis drives colon tumorigenesis cooperatively with MYC. Ann Oncol (2015) 26:935–42. doi:10.1093/annonc/mdv034
96. Ooi WF, Re A, Sidarovich V, Canella V, Arseni N, Adami V, et al. Segmental chromosome aberrations converge on overexpression of mitotic spindle regulatory genes in high-risk neuroblastoma. Genes Chromosomes Cancer (2012) 51:545–56. doi:10.1002/gcc.21940
97. Bibby RA, Tang C, Faisal A, Drosopoulos K, Lubbe S, Houlston R, et al. A cancer-associated aurora A mutant is mislocalized and misregulated due to loss of interaction with TPX2. J Biol Chem (2009) 284:33177–84. doi:10.1074/jbc.M109.032722
98. Pascreau G, Eckerdt F, Lewellyn AL, Prigent C, Maller JL. Phosphorylation of p53 is regulated by TPX2-Aurora A in Xenopus oocytes. J Biol Chem (2009) 284:5497–505. doi:10.1074/jbc.M805959200
99. Kitajima S, Kudo Y, Ogawa I, Tatsuka M, Kawai H, Pagano M, et al. Constitutive phosphorylation of aurora-a on ser51 induces its stabilization and consequent overexpression in cancer. PLoS One (2007) 2:e944. doi:10.1371/journal.pone.0000944
Keywords: Aurora A kinase, TPX2, spindle, RanGTP, microtubule, cell division, importin, phosphorylation
Citation: Garrido G and Vernos I (2016) Non-centrosomal TPX2-Dependent Regulation of the Aurora A Kinase: Functional Implications for Healthy and Pathological Cell Division. Front. Oncol. 6:88. doi: 10.3389/fonc.2016.00088
Received: 08 January 2016; Accepted: 29 March 2016;
Published: 15 April 2016
Edited by:
Ignacio Perez De Castro, Spanish National Rare Diseases Research Institute, SpainReviewed by:
Giulia Guarguaglini, National Research Council, ItalyCopyright: © 2016 Garrido and Vernos. This is an open-access article distributed under the terms of the Creative Commons Attribution License (CC BY). The use, distribution or reproduction in other forums is permitted, provided the original author(s) or licensor are credited and that the original publication in this journal is cited, in accordance with accepted academic practice. No use, distribution or reproduction is permitted which does not comply with these terms.
*Correspondence: Isabelle Vernos, aXNhYmVsbGUudmVybm9zQGNyZy5ldQ==
Disclaimer: All claims expressed in this article are solely those of the authors and do not necessarily represent those of their affiliated organizations, or those of the publisher, the editors and the reviewers. Any product that may be evaluated in this article or claim that may be made by its manufacturer is not guaranteed or endorsed by the publisher.
Research integrity at Frontiers
Learn more about the work of our research integrity team to safeguard the quality of each article we publish.