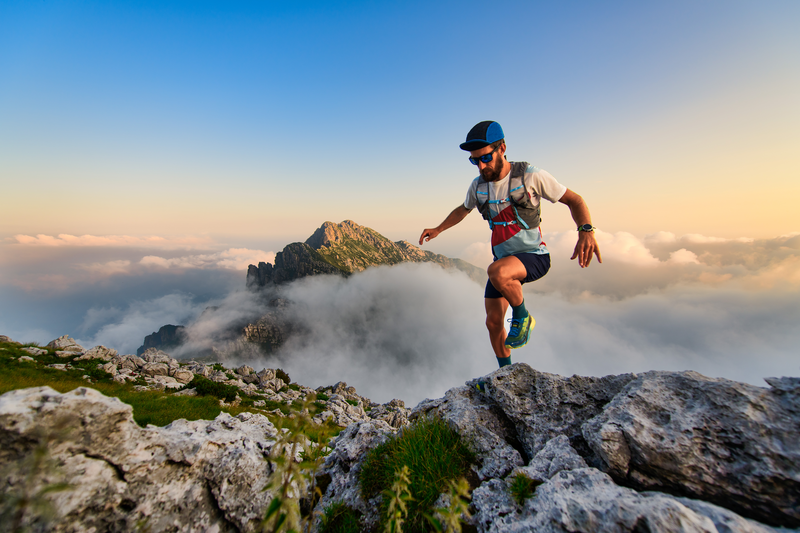
95% of researchers rate our articles as excellent or good
Learn more about the work of our research integrity team to safeguard the quality of each article we publish.
Find out more
MINI REVIEW article
Front. Oncol. , 17 February 2016
Sec. Molecular and Cellular Oncology
Volume 6 - 2016 | https://doi.org/10.3389/fonc.2016.00033
This article is part of the Research Topic Glycosylation Changes in Cancer: An Innovative Frontier at the Interface of Cancer and Glycobiology View all 16 articles
The term “cancer” refers to a group of diseases involving abnormal cell growth with the potential to invade or spread to other parts of the body. Epithelial–mesenchymal transition (EMT), a process whereby epithelial cells lose their cell polarity and cell–cell adhesion ability, and acquire migratory and invasive properties to gain mesenchymal phenotype, is an important step leading to tumor metastasis. Glycans, such as N-glycans, O-glycans, and glycosphingolipids, are involved in numerous biological processes, including inflammation, virus/bacteria–host interactions, cell–cell interactions, morphogenesis, and cancer development and progression. Aberrant expression of glycans has been observed in several EMT models, and the functional roles of such glycans in cancer development and progression has been investigated. We summarize here recent research progress regarding the functions of glycans in cancer cells undergoing EMT. Better understanding of the mechanisms underlying aberrant glycan patterns in EMT and cancer will facilitate the development of such glycans as cancer biomarkers or as targets in design and synthesis of anti-tumor drugs.
The term “cancer” refers to a group of diseases involving abnormal cell growth with the potential to invade or spread to other parts of the body. Functioning of proteins in both normal and cancer cells is maintained by post-translational modifications (PTSs), which include phosphorylation, ubiquitination, methylation, N-acetylation, and glycosylation. Glycosylation is the most commonly occurring of these PTMs, and is involved in many biological processes.
Glycosylation of proteins (“glycoproteins”) in eukaryotic cells is classified on the basis of various linkages of glycans to protein core regions; e.g., N-linked glycan (GlcNAc linkage to Asn), O-linked glycan (O-GalNAc linkage to Ser/Thr), and other types of “O-linked glycan” (e.g., O-linked mannose, O-linked GlcNAc). Coupling of glycans to lipids results in molecules termed “glycosphingolipids” (GSLs) (Figure 1). Glycans participate in numerous biological processes, including signal transduction, inflammation, virus/bacteria–host interactions, cell–cell interactions, and cancer development and progression (1–3).
We review here the roles of glycans in cancer cells undergoing epithelial–mesenchymal transition (EMT), a fundamental biological phenomenon that occurs during early embryonic development, tissue repair, and cancer metastasis.
The EMT process is an important step in promotion of tumor metastasis, whereby epithelial cells lose cell polarity and cell–cell adhesion ability, and acquire migratory and invasive properties to gain mesenchymal phenotype (Figure 1) (4). Activation of EMT has been implicated in acquisition of malignant phenotypes by epithelial cancer cells (5). Malignant carcinoma cells often display a mesenchymal phenotype and express “mesenchymal markers,” such as N-cadherin, vimentin, fibroblast-specific protein 1 (FSP1), and desmin. Such carcinoma cells acquire migratory and invasive properties, and subsequently undergo steps in the invasion/metastasis process (5–7).
Multiple signals are responsible for induction of EMT in cancer cells; in particular, hepatocyte growth factor (HGF), epidermal growth factor (EGF), and transforming growth factor-β (TGF-β) (5, 8). Several in vitro EMT models have been developed for elucidation of molecular mechanisms underlying the association between inflammation and cancer progression. TGF-β was originally described as an inducer of EMT in normal mammary epithelial cells (9), and later in other types of cancer cells (10, 11). The signaling pathways of ERK/MAPK, Jagged/Notch, WNT/GSK3/β-catenin, NF-kB, and PI3K clearly cooperate with TGF-β to play a role in cancer metastasis (12). HGF and other inducers can induce cells to undergo changes in morphology that favor a mesenchymal phenotype characteristic of EMT (13, 14). In vitro and in vivo models continue to be developed as useful tools for addressing crucial, yet-unanswered questions in EMT research.
Glycosylation is catalyzed by glycosyltransferases and glycosidases. Over 200 glycosyltransferase genes have been identified. These enzymes produce distinctive types of glycans with strict substrate specificity. Aberrant glycosylation, associated with expression of specific glycosyltransferases, is a hallmark of cancer, and reflects cancer-specific cellular changes.
Changes in N-glycan patterns and the related glycosyltransferases are important in understanding the role of EMT and adhesive properties of cancer cells. Xu et al. reported decreased expression of β1,4-N-acetylglucosaminyltransferase III (GnT-III) and its product (bisecting N-glycans) in a TGF-β1-induced EMT model (15). GnT-III modified E-cadherin and inhibited β-catenin translocation into the cytoplasm and nucleus resulting from cell–cell contact (15). Inhibition of N-acetylglucosaminyltransferase V (GnT-V) expression prevented liver fibrosis and suppressed TGF-β1-induced EMT in hepatocytes by reversal of EMT markers (16). Yang et al. (17) found that fucosyltransferase IV (FUT4) activated PI3K/Akt and NF-κB signaling systems and facilitated acquisition of a mesenchymal phenotype during EMT. FUT8, the only enzyme that catalyzes α1,6-fucosylation in mammals, was up-regulated during EMT through transactivation of β-catenin/lymphoid enhancer-binding factor-1 (LEF-1) (18). E-cadherin with enhanced core fucosylation (through FUT8 overexpression) in giant lung carcinoma cell line 95C reduced Src phosphorylation and inhibited cell migration, whereas E-cadherin with low core fucosylation activated Src and induced an EMT-like process (19). Increased expression of β-galactoside α2,6-sialyltranferase 1 (ST6GAL1), which adds terminal α2,6-sialylation to N-glycans, has been observed in a variety of carcinomas and in a TGF-β-induced EMT model. Up-regulation of ST6GAL1 contributed to EMT through a non-Smad signaling pathway (20). Du et al. analyzed dynamic changes of sialylation in TGF-β1-induced EMT in human keratinocyte HaCaT cells. Sialylation was found to be down-regulated during EMT, and then reverted (up-regulated) in the mesenchymal state following EMT. Global inhibition of sialylation by a fluorinated analog of sialic acid promoted the EMT process (21).
Many groups have utilized glycomic techniques (mass spectrometry, glycogene microarray, and lectin microarray analysis) to evaluate aberrant N-glycosylation in tumor progression, particularly during EMT (22, 23). Li et al. used a lectin microarray to analyze cell surface protein glycosylation in an HGF-induced EMT model in hepatocellular carcinoma cells. Consistent with the microarray findings, mRNA levels of glycosyltransferase genes involved in N-glycan synthesis (e.g., GnT-III) were reduced, whereas mRNA levels of GnT-V, FUT8, and β3GalT5 were increased (14). In a study of TGF-β-stimulated breast epithelial cells, Tan et al. (22) observed elevated levels of high-mannose-type N-glycans, but reduced levels of antennary N-glycans, fucosylation, and bisecting GlcNAc N-glycans. Expression of seven N-glycan-related genes was significantly altered, and the products of these genes (e.g., ALG9, MGAT3, MGAT4B) evidently contributed to the alteration of N-glycans. Guo et al. (23) used a combination of mass spectrometry, lectin microarray, and GlycoV4 oligonucleotide microarray analysis to demonstrate altered expression of five N-glycan-related genes and corresponding glycan structures in TGF-β-stimulated bladder epithelial cells.
Structural changes of O-linked glycosylation have been correlated with tumor development and progression in only a few cases (24–26). Overexpression of MUC1 O-glycans was observed in breast, prostate, ovary, and pancreatic cancer cells (27). Altered MUC1 expression participates in the EMT process by interacting with β-catenin to activate the transcription factor SNAIL (28, 29). Overexpression of the polypeptide N-acetylgalactosaminyltransferase 6 (GALNT6), which is involved in the initial step of O-glycosylation, disrupted acinar morphogenesis and produced cellular changes similar to those of EMT in normal mammary epithelial MCF10A cells. O-glycosylated fibronectin (FN) was stabilized by GALNT6 and further facilitated the acinar disruption (30). Freire-de-Lima et al. (31) found that oncofetal FN (onfFN), a FN isoform recognized by mAb FDC6 in fetal and cancer tissues (27), was up-regulated in TGFβ-induced EMT (31). FDC6 reactivity required O-glycan addition at a specific Thr, GalNAc-T3, and/or GalNAc-T6 of onfFN in EMT process, whereas inhibition of GalNAc-T3 and GalNAc-T6 of onfFN suppressed the EMT process. In a follow-up study, only TGF-β-treated cells expressed a substantial amount of onfFN, and only O-glycosylated onfFN induced EMT-related events (32). Hyperglycemic conditions induced EMT in human lung adenocarcinoma A549 cells, and also increased onfFN protein levels, thereby promoting up-regulation of mRNA levels for ppGalNAc-T6 and the IIICS domain of FN (33). The molecular mechanisms triggered by this unusual glycoprotein remain unknown; however, O-glycosylated onfFN appears to be a possible target for anti-cancer drug development.
Glycosphingolipids mediate cell adhesion and modulate cell growth through their effects on growth factor receptor tyrosine kinases (34, 35). Some GSLs, particularly gangliosides (sialic acid-containing GSLs), control cell motility through interactions with integrins and tetraspanin CD9 or CD82 (36, 37). The gangliosides GM2, GD2, and GD3 are highly expressed in various human tumors (melanomas, gliomas, neuroblastomas), but are absent or weakly expressed in normal tissues (38, 39). Guan et al. (40) investigated the roles of GSLs in phenotypic changes of cell adhesion, motility, and growth during EMT. Specific GSLs were shown to participate in EMT in human and mouse mammary epithelial cells. Changes in GSL patterns in these cells with and without TGF-β treatment were compared, and levels of Gg4 and GM2 were found to be greatly reduced or depleted by TGF-β. In a follow-up study (41), the functional role of Gg4 in EMT was further elucidated using normal mouse mammary gland epithelial cells (NMuMG) in which EMT was induced by hypoxia or CoCl2 treatment. Both treatments caused reduced levels of Gg4 and UDP-Gal:β1-3galactosyltransferase-4 (β3GalT4) mRNA, similarly to TGF-β-induced EMT.
Following the initial 2009 report of GSL involvement in EMT, several studies described the effects of altered GSL expression on EMT processes. Kim et al. (42) reported significantly increased expression of GM3 and GM3 synthase mRNA in TGF-β1-induced EMT of human lens epithelial cells. Inhibition of GM3 and GM3 synthase expression in the TGF-β1-stimulated cells resulted in reduced cell migration and EMT-related signaling, through interaction with TGF-β receptors. Sarkar et al. (43) showed that inhibition of GD3 synthase, an enzyme involved in GD2 biosynthesis, blocked initiation and maintenance of the EMT process. Findings from these and other studies clearly indicate that aberrant GSLs associated with tumor progression play various different roles in initiation or suppression of EMT.
Glycans often function as essential biosynthesis precursors or as structural elements that become attached to proteins or lipids to form glycoproteins or glycolipids. Most glycan complexes are present on cell surfaces, where they play essential roles in cell–cell interactions, signal transduction, receptor activation, cell adhesion, and other physiological processes. The EMT process is closely associated with glycosylation changes, but our knowledge of the molecular mechanisms that underlie altered glycan patterns in cancer cells undergoing EMT is quite limited. We have reviewed here some specific N-glycans, O-glycans, and GSLs known to be altered during EMT, and effects of those glycans and their glycosyltransferases on processes, such as cell proliferation and migration (summary in Table 1). Advanced methods in cancer research will help us elucidate the above-mentioned molecular mechanisms. Integrated glycomic techniques, as used in some of the studies reviewed here, will accelerate the discovery of new glycan markers and the development of novel diagnostic and therapeutic approaches for preventing tumor progression. By better understanding the mechanisms of aberrant glycan patterns, we will more efficiently identify cancer markers and targets for design and synthesis of anti-tumor drugs.
FG designed the manuscript. XL, XW, ZT, and SC wrote the manuscript.
The authors declare that the research was conducted in the absence of any commercial or financial relationships that could be construed as a potential conflict of interest.
Studies by the authors mentioned here were supported by the Natural Science Foundation of Jiangsu Province, China (No. BK2012113), Jiangsu Province Recruiting Plan for High-level, Innovative and Entrepreneurial Talents, Jiangsu Province “Six Summit Talent” Foundation (2013-SWYY-019), and the 111 Project (No. 111-2-06). We are grateful to Dr. S. Anderson for English editing of the manuscript.
1. Varki A. Nothing in glycobiology makes sense, except in the light of evolution. Cell (2006) 126:841–5. doi: 10.1016/j.cell.2006.08.022
2. Bucior I, Burger MM. Carbohydrate-carbohydrate interactions in cell recognition. Curr Opin Struct Biol (2004) 14:631–7. doi:10.1016/j.sbi.2004.08.006
3. Dwek RA. Glycobiology: toward understanding the function of sugars. Chem Rev (1996) 96:683–720. doi:10.1021/cr940283b
4. Chaffer CL, Weinberg RA. A perspective on cancer cell metastasis. Science (2011) 331:1559–64. doi:10.1126/science.1203543
5. Thiery JP. Epithelial-mesenchymal transitions in tumour progression. Nat Rev Cancer (2002) 2:442–54. doi:10.1038/nrc822
6. Yang J, Weinberg RA. Epithelial-mesenchymal transition: at the crossroads of development and tumor metastasis. Dev Cell (2008) 14:818–29. doi:10.1016/j.devcel.2008.05.009
7. Fidler IJ, Poste G. The “seed and soil” hypothesis revisited. Lancet Oncol (2008) 9:808. doi:10.1016/S1470-2045(08)70201-8
8. Jechlinger M, Grunert S, Beug H. Mechanisms in epithelial plasticity and metastasis: insights from 3D cultures and expression profiling. J Mammary Gland Biol Neoplasia (2002) 7:415–32. doi:10.1023/A:1024090116451
9. Miettinen PJ, Ebner R, Lopez AR, Derynck R. TGF-beta induced transdifferentiation of mammary epithelial cells to mesenchymal cells: involvement of type I receptors. J Cell Biol (1994) 127:2021–36. doi:10.1083/jcb.127.6.2021
10. Song J. EMT or apoptosis: a decision for TGF-beta. Cell Res (2007) 17:289–90. doi:10.1038/cr.2007.25
11. Zavadil J, Bottinger EP. TGF-beta and epithelial-to-mesenchymal transitions. Oncogene (2005) 24:5764–74. doi:10.1038/sj.onc.1208927
12. Kalluri R, Weinberg RA. The basics of epithelial-mesenchymal transition. J Clin Invest (2009) 119:1420–8. doi:10.1172/JCI39104
13. Farrell J, Kelly C, Rauch J, Kida K, Garcia-Munoz A, Monsefi N, et al. HGF induces epithelial-to-mesenchymal transition by modulating the mammalian hippo/MST2 and ISG15 pathways. J Proteome Res (2014) 13:2874–86. doi:10.1021/pr5000285
14. Li S, Mo C, Peng Q, Kang X, Sun C, Jiang K, et al. Cell surface glycan alterations in epithelial mesenchymal transition process of Huh7 hepatocellular carcinoma cell. PLoS One (2013) 8:e71273. doi:10.1371/journal.pone.0071273
15. Xu Q, Isaji T, Lu Y, Gu W, Kondo M, Fukuda T, et al. Roles of N-acetylglucosaminyltransferase III in epithelial-to-mesenchymal transition induced by transforming growth factor beta1 (TGF-beta1) in epithelial cell lines. J Biol Chem (2012) 287:16563–74. doi:10.1074/jbc.M111.262154
16. Liu J, Zhang Z, Tu X, Liu J, Zhang H, Zhang J, et al. Knockdown of N-acetylglucosaminyl transferase V ameliorates hepatotoxin-induced liver fibrosis in mice. Toxicol Sci (2013) 135:144–55. doi:10.1093/toxsci/kft135
17. Yang X, Liu S, Yan Q. Role of fucosyltransferase IV in epithelial-mesenchymal transition in breast cancer cells. Cell Death Dis (2013) 4:e735. doi:10.1038/cddis.2013.241
18. Chen CY, Jan YH, Juan YH, Yang CJ, Huang MS, Yu CJ, et al. Fucosyltransferase 8 as a functional regulator of nonsmall cell lung cancer. Proc Natl Acad Sci U S A (2013) 110:630–5. doi:10.1073/pnas.1220425110
19. Shao K, Chen ZY, Gautam S, Deng NH, Zhou Y, Wu XZ. Posttranslational modification of E-cadherin by core fucosylation regulates Src activation and induces epithelial-mesenchymal transition-like process in lung cancer cells. Glycobiology (2016) 26:142–54. doi:10.1093/glycob/cwv089
20. Lu J, Isaji T, Im S, Fukuda T, Hashii N, Takakura D, et al. beta-Galactoside alpha2,6-sialyltranferase 1 promotes transforming growth factor-beta-mediated epithelial-mesenchymal transition. J Biol Chem (2014) 289:34627–41. doi:10.1074/jbc.M114.593392
21. Du J, Hong S, Dong L, Cheng B, Lin L, Zhao B, et al. Dynamic sialylation in transforming growth factor-beta (TGF-beta)-induced epithelial to mesenchymal transition. J Biol Chem (2015) 290:12000–13. doi:10.1074/jbc.M115.636969
22. Tan Z, Lu W, Li X, Yang G, Guo J, Yu H, et al. Altered N-Glycan expression profile in epithelial-to-mesenchymal transition of NMuMG cells revealed by an integrated strategy using mass spectrometry and glycogene and lectin microarray analysis. J Proteome Res (2014) 13:2783–95. doi:10.1021/pr401185z
23. Guo J, Li X, Tan Z, Lu W, Yang G, Guan F. Alteration of N-glycans and expression of their related glycogenes in the epithelial-mesenchymal transition of HCV29 bladder epithelial cells. Molecules (2014) 19:20073–90. doi:10.3390/molecules191220073
24. Baldus SE, Engelmann K, Hanisch FG. MUC1 and the MUCs: a family of human mucins with impact in cancer biology. Crit Rev Clin Lab Sci (2004) 41:189–231. doi:10.1080/10408360490452040
25. Pinto R, Carvalho AS, Conze T, Magalhaes A, Picco G, Burchell JM, et al. Identification of new cancer biomarkers based on aberrant mucin glycoforms by in situ proximity ligation. J Cell Mol Med (2012) 16:1474–84. doi:10.1111/j.1582-4934.2011.01436.x
26. Pinho S, Marcos NT, Ferreira B, Carvalho AS, Oliveira MJ, Santos-Silva F, et al. Biological significance of cancer-associated sialyl-Tn antigen: modulation of malignant phenotype in gastric carcinoma cells. Cancer Lett (2007) 249:157–70. doi:10.1016/j.canlet.2006.08.010
27. Freire-de-Lima L. Sweet and sour: the impact of differential glycosylation in cancer cells undergoing epithelial-mesenchymal transition. Front Oncol (2014) 4:59. doi:10.3389/fonc.2014.00059
28. Gnemmi V, Bouillez A, Gaudelot K, Hemon B, Ringot B, Pottier N, et al. MUC1 drives epithelial-mesenchymal transition in renal carcinoma through Wnt/beta-catenin pathway and interaction with SNAIL promoter. Cancer Lett (2014) 346:225–36. doi:10.1016/j.canlet.2013.12.029
29. Roy LD, Sahraei M, Subramani DB, Besmer D, Nath S, Tinder TL, et al. MUC1 enhances invasiveness of pancreatic cancer cells by inducing epithelial to mesenchymal transition. Oncogene (2011) 30:1449–59. doi:10.1038/onc.2010.526
30. Park JH, Katagiri T, Chung S, Kijima K, Nakamura Y. Polypeptide N-acetylgalactosaminyltransferase 6 disrupts mammary acinar morphogenesis through O-glycosylation of fibronectin. Neoplasia (2011) 13:320–6. doi:10.1593/neo.101440
31. Freire-de-Lima L, Gelfenbeyn K, Ding Y, Mandel U, Clausen H, Handa K, et al. Involvement of O-glycosylation defining oncofetal fibronectin in epithelial-mesenchymal transition process. Proc Natl Acad Sci U S A (2011) 108:17690–5. doi:10.1073/pnas.1115191108
32. Ding Y, Gelfenbeyn K, Freire-de-Lima L, Handa K, Hakomori S. Induction of epithelial-mesenchymal transition with O-glycosylated oncofetal fibronectin. FEBS Lett (2012) 586:1813–20. doi:10.1016/j.febslet.2012.05.020
33. Alisson-Silva F, Freire-de-Lima L, Donadio JL, Lucena MC, Penha L, Sa-Diniz JN, et al. Increase of O-glycosylated oncofetal fibronectin in high glucose-induced epithelial-mesenchymal transition of cultured human epithelial cells. PLoS One (2013) 8:e60471. doi:10.1371/journal.pone.0060471
34. Fenderson BA, Zehavi U, Hakomori S. A multivalent lacto-N-fucopentaose III-lysyllysine conjugate decompacts preimplantation mouse embryos, while the free oligosaccharide is ineffective. J Exp Med (1984) 160:1591–6. doi:10.1084/jem.160.5.1591
35. Handa K, Takatani-Nakase T, Larue L, Stemmler MP, Kemler R, Hakomori S. Le(x) glycan mediates homotypic adhesion of embryonal cells independently from E-cadherin: a preliminary note. Biochem Biophys Res Commun (2007) 358:247–52. doi:10.1016/j.bbrc.2007.04.101
36. Todeschini AR, Dos Santos JN, Handa K, Hakomori S. Ganglioside GM2-tetraspanin CD82 complex inhibits Met and its cross-talk with integrins, providing a basis for control of cell motility through glycosynapse. J Biol Chem (2007) 282:8123–33. doi:10.1074/jbc.M611407200
37. Todeschini AR, Dos Santos JN, Handa K, Hakomori S. Ganglioside GM2/GM3 complex affixed on silica nanospheres strongly inhibits cell motility through CD82/cMet-mediated pathway. Proc Natl Acad Sci U S A (2008) 105:1925–30. doi:10.1073/pnas.0709619104
38. Lloyd KO, Old LJ. Human monoclonal antibodies to glycolipids and other carbohydrate antigens: dissection of the humoral immune response in cancer patients. Cancer Res (1989) 49:3445–51.
39. Hakomori S. Tumor malignancy defined by aberrant glycosylation and sphingo(glyco)lipid metabolism. Cancer Res (1996) 56:5309–18.
40. Guan F, Handa K, Hakomori S. Specific glycosphingolipids mediate epithelial-to-mesenchymal transition of human and mouse epithelial cell lines. Proc Natl Acad Sci U S A (2009) 106:7461–6. doi:10.1073/pnas.0902368106
41. Guan F, Schaffer L, Handa K, Hakomori S. Functional role of gangliotetraosylceramide in epithelial-to-mesenchymal transition process induced by hypoxia and by TGF-β. FASEB J (2010) 24:4889–903. doi:10.1096/fj.10-162107
42. Kim SJ, Chung TW, Choi HJ, Kwak CH, Song KH, Suh SJ, et al. Ganglioside GM3 participates in the TGF-beta1-induced epithelial-mesenchymal transition of human lens epithelial cells. Biochem J (2013) 449:241–51. doi:10.1042/BJ20120189
Keywords: glycan, cancer, epithelial–mesenchymal transition, glycosylation, glycosyltransferases
Citation: Li X, Wang X, Tan Z, Chen S and Guan F (2016) Role of Glycans in Cancer Cells Undergoing Epithelial–Mesenchymal Transition. Front. Oncol. 6:33. doi: 10.3389/fonc.2016.00033
Received: 22 December 2015; Accepted: 01 February 2016;
Published: 17 February 2016
Edited by:
Leonardo Freire-de-Lima, Federal University of Rio de Janeiro, BrazilReviewed by:
Leonardo Marques Da Fonseca, Federal University of Rio de Janeiro, BrazilCopyright: © 2016 Li, Wang, Tan, Chen and Guan. This is an open-access article distributed under the terms of the Creative Commons Attribution License (CC BY). The use, distribution or reproduction in other forums is permitted, provided the original author(s) or licensor are credited and that the original publication in this journal is cited, in accordance with accepted academic practice. No use, distribution or reproduction is permitted which does not comply with these terms.
*Correspondence: Feng Guan, ZmVuZ2d1YW5AamlhbmduYW4uZWR1LmNu
Disclaimer: All claims expressed in this article are solely those of the authors and do not necessarily represent those of their affiliated organizations, or those of the publisher, the editors and the reviewers. Any product that may be evaluated in this article or claim that may be made by its manufacturer is not guaranteed or endorsed by the publisher.
Research integrity at Frontiers
Learn more about the work of our research integrity team to safeguard the quality of each article we publish.