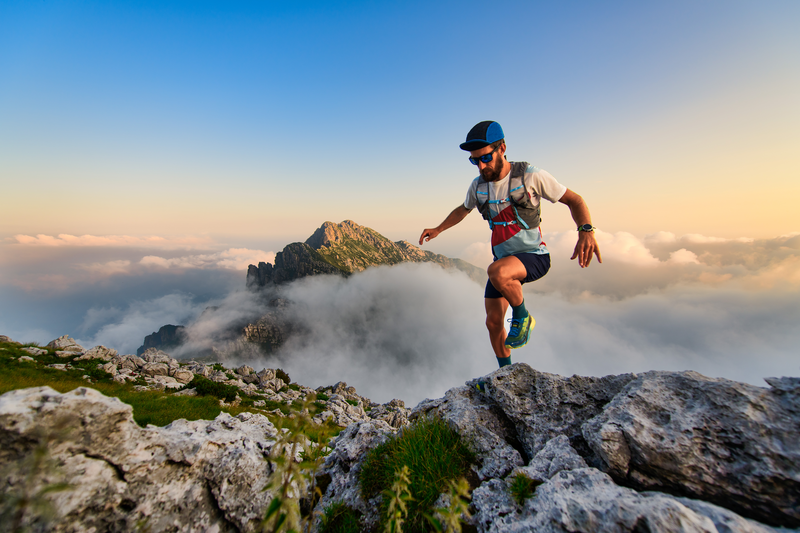
94% of researchers rate our articles as excellent or good
Learn more about the work of our research integrity team to safeguard the quality of each article we publish.
Find out more
REVIEW article
Front. Oncol. , 23 May 2014
Sec. Molecular and Cellular Oncology
Volume 4 - 2014 | https://doi.org/10.3389/fonc.2014.00114
This article is part of the Research Topic GLYCOBIOLOGY OF CANCER: CURRENT STATUS AND FUTURE DIRECTIONS View all 10 articles
A commentary has been posted on this article:
Corrigendum: Glycobiology of Neuroblastoma: Impact on Tumor Behavior, Prognosis, and Therapeutic Strategies
Neuroblastoma (NB), accounting for 10% of childhood cancers, exhibits aberrant cell-surface glycosylation patterns. There is evidence that changes in glycolipids and protein glycosylation pathways are associated to NB biological behavior. Polysialic acid (PSA) interferes with cellular adhesion, and correlates with NB progression and poor prognosis, as well as the expression of sialyltransferase STX, the key enzyme responsible for PSA synthesis. Galectin-1 and gangliosides, overexpressed and actively shedded by tumor cells, can modulate normal cells present in the tumor microenvironment, favoring angiogenesis and immunological escape. Different glycosyltransferases are emerging as tumor markers and potential molecular targets. Immunotherapy targeting disialoganglioside GD2 rises as an important treatment option. One anti-GD2 antibody (ch14.18), combined with IL-2 and GM-CSF, significantly improves survival for high-risk NB patients. This review summarizes our current knowledge on NB glycobiology, highlighting the molecular basis by which carbohydrates and protein–carbohydrate interactions impact on biological behavior and patient clinical outcome.
Neuroblastoma (NB), the most common type of solid extra-cranial tumor in children, accounts for nearly 15% of pediatric cancer-related deaths (1). It is a very complex disease, extremely heterogeneous (2), able to regress spontaneously, even without therapy, but frequently displaying very aggressive behavior, refractory to current intensive multimodal therapy. Although over the past decade advances in NB staging through identification of molecular events responsible for different clinical behavior have improved risk stratification, not enough is known about how the features of this disease relate to its underlying biology and how this can be exploited to improve clinical outcome (3).
Clinical presentation of NB depends on primary tumor localization, most of them occur within the abdomen. Approximately half of the patients present localized forms of the disease, but dissemination occurs through lymphatic and hematogenous pathways, and about 35% have regional lymph node spread at the time of diagnosis. Bone, bone marrow, and liver are the most common sites of hematogenous spread. Most NB are undifferentiated tumors, which is an important issue in outcome prediction (4). The most common focal genetic lesion in NB is MYCN (V-myc myelocytomatosis viral-related oncogene) amplification, which occurs in approximately 22% of the cases and has been largely associated with poor outcome (2). However, among patients with MYCN-amplified low-stage NB, the outcome was significantly better for patients with hyperdiploid tumors when compared to those with diploid tumors (5), suggesting that tumor-cell ploidy could potentially improve risk classification. Another genetic disorder frequently found in NB is allelic loss of 11q. Although it seems mutually exclusive with MYCN amplification, it is frequently associated to other genetic abnormalities and poor clinical outcome (6). Pediatric oncologists classically distinguished between two risk-groups: (1) The low-risk group, consisting of non-MYCN-amplified localized tumors or the metastatic form in children younger than 18 months [survival rate of up to 90%, (7)]. (2) The high-risk group, comprising all MYCN-amplified NB, regardless of stage and age of the child, plus non-MYCN-amplified disseminated NB for children older than 18 months, usually very aggressive tumors that more frequently lead to death (8). However, relapse for low-risk patients constitutes a current concern (9), hence the International Neuroblastoma Risk Group Staging System (INRGSS) has recently established a new classification based on clinical criteria and image-defined risk factors (10). It distinguishes localized stages L1 and L2, and stages M and MS as disseminated forms. Based on this classification, age at diagnosis, histology and grade of tumor differentiation, MYCN status, presence/absence of 11q aberrations, and tumor-cell ploidy, NB patients can be sorted into very low-, low-, intermediate-, and high-risk groups according to percentage of 5 years disease-free survival (11). This classification will require validation in prospective clinical studies and solving some limitations as primary tumor dimensions using anatomic imaging, definitions of metastatic site, response not measurable by anatomical imaging (bone and bone marrow), as well as metastatic disease assessment using 123I-MIBG imaging and quantification of bone marrow disease (12).
Tumor cells, particularly tumors of neuroectodermal cell origin, express high levels of gangliosides (13). Besides their expression on tumor-cell membranes, gangliosides are also shed in the tumor microenvironment and eventually circulate in the patients’ bloodstream. These molecules are recognized to have multiple effects; for example, acting as cell-surface receptors and markers, participating in intercellular communication, and modulating cell signaling, cell cycling, and cell motility (14, 15). They have been implicated in the biology of various cellular processes, and linked to the behavior of many types of tumors (16). In NB, ganglioside composition is linked to biological and clinical behavior.
Gangliosides consist of a carbohydrate chain, containing one or several sialic acid residues, and a lipid portion (ceramide backbone), which anchors the ganglioside molecule to the cell membrane (17). Ganglioside biosynthesis occurs in a sequential order of glycosylations via two major pathways designated as “a” (GM2, GM1a, and GD1a) and “b” (GD3, GD2, GD1b, GT1b, and GQ1b), from a common precursor (GM3) (Figure 1). Each ganglioside is structurally more complex than its precursor molecule, and the stepwise addition of monosaccharide or sialic acid residues in the Golgi apparatus is catalyzed by the same specific membrane-bound glycosyltransferases in both pathways (18) (Figure 1). Gangliosides can also be grouped into structurally simple (SG) and complex (CG) molecules. The enzyme GM1a/GD1b synthase (UDP-Gal:betaGlcNAc-beta-1,3-galactosyltransferase) converts its substrates, the simple gangliosides GM2 and GD2, into the corresponding initial complex ganglioside products, GM1a and GD1b (Figure 1). The key role played by this enzyme in human NB was confirmed by inducing high expression of GM1a/GD1b synthase in IMR-32 cells, which normally contain predominantly simple gangliosides, observing a rise of complex ganglioside expression, associated with reduced levels of simple gangliosides (19).
Ganglioside metabolism differs between NB tumors with different malignant potential, and may ultimately affect clinical behavior and patient outcome. It was observed that high levels of gangliosides of the “b” pathway (GD3, GD2, GD1b, GT1b, GQ1b) are predominant in infant NB compared to the same disease in older children (20). Evidence supports a role of some tumor gangliosides as prognostic indicators in NB. It is very interesting that low (≤35%) or absent expression of gangliosides of the complex “b” (CbG) pathway (GD1b, GT1b, and GQ1b) correlates with an aggressive biological phenotype in human NB tumors (21). This observation is consistent with reports in which a decreased or absent expression of two CbG subspecies, GD1b and GT1b, was linked to reduced survival in NB patients (22, 23). High expression of complex gangliosides, both complex “a” gangliosides (CaG) and CbG, has been shown to inhibit aggressive tumor-cell behavior in vitro (e.g., cellular proliferation and migration) and to enhance differentiation (24, 25). In this context, complex gangliosides have been proposed as useful biomarkers to predict clinical outcome, to stratify patients with NB for purposes of tailoring anti-cancer treatment, or to monitor effectiveness of treatment.
Retinoic acid is successfully used in maintenance therapy of disseminated NB (26). Treatment with this pharmacological agent induces a dramatic shift from synthesis of simple gangliosides toward predominant expression of structurally complex “a” and “b” pathway ganglioside molecules in some NB cell lines (27). Predominant expression of complex gangliosides can be considered a biochemical marker of increasing neuronal differentiation. The retinoic acid-induced rise of CbG expression in NB cells represents a transition into a ganglioside pattern associated with clinically less-aggressive NB tumors. These authors demonstrated that treatment with retinoic acid markedly enhances the activity of GD1b/GM1a synthase, resulting in increased expression of the complex gangliosides in NB cell lines.
When compared with normal brain tissue, NB tumors were found to overexpress the disialoganglioside GD2 (“b” pathway) (28). GD2 is a surface glycolipid antigen normally found on neurons, peripheral nerve fibers, and skin melanocytes. In NB, GD2 is expressed homogeneously and abundantly in virtually all neuroblasts and facilitates the attachment of tumor cells to the extracellular matrix (29, 30). Because of the widespread expression of GD2 in NB tissue, contrasting with the more benign tumors ganglioneuroma and ganglioneuroblastoma, GD2 is a sensitive diagnostic marker which can help to discriminate NB from other related tumors (28, 31). Expression of GD2 is an indicator of the presence of NB, and high levels of circulating GD2 have been correlated with a more rapid disease progression among patients in advanced stages of the disease (32). However, patient outcome was independent of GD2 expression in tumor tissues (21), suggesting that GD2 is useful for diagnostic purposes but not for prognostic ones. Surface GD2 antigen is an important molecular target for NB therapy and specific monoclonal antibodies have emerged as a major therapeutic development for high-risk NB patients (see below).
Gangliosides overexpressed and actively shedded by tumor cells can modulate the function of normal cells present in the tumor microenvironment (33). Several studies have demonstrated modulation of growth factor signaling pathways through the epidermal growth factor (EGF), fibroblast growth factor (FGF), platelet-derived growth factor (PDGF), Trk family, and insulin receptors (15, 34, 35). It was also found that the enrichment of human umbilical vein endothelial cell (HUVEC) membranes with purified GD1a ganglioside results in amplified VEGF-induced signaling and the associated cellular responses of proliferation and migration, important for angiogenesis (36). In addition, tumor gangliosides induce robust murine tumor angiogenesis in vivo (37). Also, tumor-derived gangliosides may promote tumor development by suppressing immune cell function and promoting immune evasion mechanisms (38). Early studies found that gangliosides, predominantly GD2, isolated from the human NB cell line LAN5, inhibit murine cellular immune responses in vivo (39, 40). The mechanisms by which gangliosides suppress tumor immunity, although not fully understood, involve the regulation of different immune cells. Tumor-derived gangliosides can inhibit tumor-specific cellular cytotoxicity, suppressing the lytic activity of CD8+ T-cells (41), as well as NK cell-mediated cytotoxicity in a Siglec-7-dependent manner (42). Dendritic cell (DC) development and function is critical in the initiation phase of any antigen-specific immune response against tumors. There is an important body of evidence indicating that NB-derived gangliosides regulate development of tumor immunity through the inhibition of DC function (43). Shurin et al. demonstrated that NB gangliosides inhibit the generation of functionally active DCs, playing a role in tumor-induced immunosuppression (44). NB gangliosides may induce inhibition of DC function causing CD40 signaling deficiency (45) and alterations in TLR signaling (46). Gangliosides promote DC population development characterized by decreased CD86 expression (costimulatory signal), and decreased interleukin-12 and interleukin-6 production. When these cells are used as antigen-presenting cells, CD4 T-cells are primed to proliferate normally, but have a defect in T helper (Th) effector cell development. This defect in Th effector cell responses is associated with the development of regulatory T-cell activity that can suppress the activation of previously primed Th effector cells in a contact-dependent manner (47). In total, these data suggest that ganglioside-exposed DC promote regulatory T-cell activity that may have long-lasting effects on the development of tumor-specific immune responses.
N-linked glycosylation is a highly regulated post-translational modification, which is involved in several biological processes such as protein folding and conformation, oligomerization, cell–cell interactions, and targeting proteins to sub- or extracellular locations. It was found that intercellular adhesion molecule-2 (ICAM-2) completely suppressed disseminated tumor development in vivo in a murine model of metastatic NB (48). The authors also observed that ICAM-2 suppressed NB cell motility and growth in soft agar in vitro. These effects on NB cells depended on the interaction of ICAM-2 with the cytoskeletal linker protein α-actinin. ICAM-2 has six N-linked glycosylation sites at asparagines 47, 82, 105, 153, 178, and 187. The substitution, using site-directed mutagenesis, of asparagine by alanine at glycosylation sites, was found to reduce N-glycosylated ICAM-2, displaying a significantly attenuated ability to suppress metastatic properties of NB cells (49). Anaplastic lymphoma kinase (ALK) has been identified as a major NB predisposing gene, and activating mutations have also been identified in a subset of sporadic NB tumors (50). ALK protein expression is significantly up-regulated in advanced/metastatic NB, and overexpression of either mutated or wild-type ALK, defines poor prognosis patients (51). Inhibition of ALK activity in NB cell lines has already been approached by using specific small molecules (51, 52). ALK has 16 highly conserved putative sites of N-linked glycosylation in the extracellular domain. It was demonstrated that inhibition of N-linked glycosylation impairs ALK phosphorylation and disrupts downstream pro-survival signaling, as well as cell viability, in NB cell lines harboring mutated or amplified ALK (53), suggesting that inhibition of this post-translational modification could be a promising therapeutic approach.
Cell-surface mucins are glycoproteins carrying large numbers of O-linked oligosaccharides. The most abundant form of O-linked glycosylation in higher eukaryotes, termed “mucin-type,” is initiated by the covalent linkage of an α-N-acetylgalactosamine residue (GalNAc) to the hydroxyl group of Ser/Thr residues, catalyzed by UDP-GalNAc:polypeptide-N-acetyl-galactosaminyl-transferases (GalNAc-T). GalNAc-T is a complex family of up to 20 isoenzymes characterized to date (54). Altered O-glycan profile is a hallmark of carcinomas, which expresses truncated O-glycosylated tumor-associated antigens such as Tn, sialyl-Tn, and TF (55). In contrast, the complete absence of these antigens was reported in NB (13, 56). However, some evidence suggests that O-glycosylation pathways could play an important role in NB biology. Our research group has demonstrated that GalNAc-T9 and GalNAc-T13 might be useful tumor markers associated with low or high tumor aggressiveness, respectively (57, 58) (see below). In addition, the better outcome of NB patients is also predicted by β1,3-N-acetylglucosaminyltransferase-3 (B3GNT3) expression, the enzyme responsible for extended core 1 O-glycan (T antigen) oligosaccharide synthesis (59) (see below).
Polysialic acid (PSA) is a developmentally regulated linear homopolymer of α-2-8-linked sialic acid (up to 200 residues long) located on the outer chains of N-linked oligosaccharides (60, 61). This unique glycosylation is attached mainly to the neural cell-adhesion molecule (NCAM) (62), which is expressed on cells of neuroectodermal origin and plays a pivotal role in neural tissue development and regeneration. It is well documented that the presence of the highly negatively charged PSA on NCAM reduces NCAM-mediated adhesion processes as well as NCAM-independent cell interactions, such as cadherin-mediated cell-adhesion (63, 64). There are several isoforms of NCAM due to different sizes, three of which can carry PSA: NCAM-180 and NCAM-140 (integral membrane isoforms), and NCAM-120 (isoform anchored to the plasma membrane via a glycosyl phosphoinositol) (65, 66). The mRNA encoding the 120 kDa protein species was the most abundant isoform found in the adult brain, ganglioneuromas and ganglioneuroblastomas, but the mRNA encoding the 180 kDa species was predominant in NB (67). The polysialylated form of NCAM (PSA-NCAM) is widely expressed in embryos, especially in the brain, where it augments cellular motility and assists neuron outgrowth (68). Conversely, PSA-NCAM is not present in the adult brain, other than in areas requiring synaptic regrowth, such as the hippocampus and olfactory bulb (69, 70).
Although PSA is virtually absent in most adult tissues, it is re-expressed during the progression of some malignant tumors, such as NB, rhabdomyosarcoma, non-small cell lung cancer (NSCLC), and small cell lung cancer (SCLC) (71–75). High PSA-NCAM levels have been correlated with malignant potential and poor prognosis of SCLC, NB, glioblastoma, medulloblastoma, and rhabdomyosarcoma (73, 76–81). PSA promotes tumor-cell migration in vitro and affects tumor-cell differentiation by attenuating NCAM signaling (82). It has been reported that PSA increases the motility of SCLC cells and allows cancer cells to detach from the primary tumor, thus favoring the formation of metastatic foci (76). Regarding NB, PSA has a direct impact on tumor-cell growth. The presence of PSA-NCAM has been shown to increase proliferative cell activity in vitro (83). PSA plays an important role as regulator of NB cell migration. It was demonstrated that the migratory effect is NCAM-dependent, but independent of FGF receptor activity (84). Removal of PSA from the cell-surface led to reduced proliferation and neuronal differentiation (82). In vivo studies indicate that PSA-NCAM reduces the adhesiveness of tumor cells and promotes dissemination, and its expression is also closely associated with tumor invasion and metastasis (85). In this work, five human NB cell lines (three of which PSA-NCAM-expressing) were xenografted into SCID mice. Disseminated lung micrometastases developed from the three PSA-expressing xenografts, but not from the negative-expressing tumors. In clinical tissues, PSA-NCAM expression is indicative of undifferentiated NB, correlating with aggressive advanced disease (79, 86, 87). Its expression also correlates with N-MYC amplification (86) and its serum detection is a disease marker (78).
Two polysialyltransferases, ST8SiaII (STX) and ST8SiaIV (PST) have been shown to synthesize PSA independently. While ST8SiaII is expressed predominantly during embryonic development, ST8SiaIV is the major polysialyltransferase in the adult brain (88). Polysialylation is a protein-specific reaction in which the polysialyltransferases initially recognize a protein sequence prior to effecting glycan modifications (89, 90). STX appears to specifically polysialylate NCAM, whereas PST may additionally polysialylate other glycoproteins (91). STX is the prominent enzyme in tumor cells (73, 92, 93). In a panel of NB cell lines, co-expression of PST and STX was observed, but STX levels were significantly higher than PST in most of them (94). In NB tumors, STX expression is consistently high (93) (see below). The fact that PSA promotes a more aggressive behavior in cancer cells and that STX (absent from the adult brain) is the dominant polysialyltransferase in tumors which express PSA, strongly suggests that STX could be a good therapeutic target to inhibit tumor invasion and metastasis (95). The cytidine monophosphate (CMP) is a compound capable of reducing STX-mediated polysialylation of NCAM (96). In addition, migration of IMR-32 NB cells decreased after application of the sialic acid precursor ManNProp, which interferes with polysialylation (97). However, despite the inhibitory effects of CMP and N-acyl mannosamines on PSA synthesis, they are unlikely therapeutic agents due to their unfavorable physicochemical properties. They are hydrophilic carbohydrate derivatives which lack the ability to efficiently penetrate cell membranes. Inhibitors with properties that facilitate cell penetration to the Golgi apparatus (the location of the STX) and possess robust drug metabolism and pharmacokinetic properties are still to be discovered (95).
Lectin–glycan interactions play a fundamental role regulating mechanisms implicated in tumor immune escape (98). Galectins are a family of carbohydrate-binding proteins that share a carbohydrate recognition domain for β-galactosides and are involved in cell adhesion, migration, differentiation, angiogenesis, proliferation, mRNA splicing, and apoptosis (99). Their function takes place both extracellularly, by interacting with cell-surface and extracellular matrix glycoproteins and glycolipids, as well as intracellularly, by interacting with cytoplasmic and nuclear proteins to modulate signaling pathways. Current research indicates that galectins play an important role in cancer; they contribute to neoplastic transformation, tumor-cell survival, angiogenesis, and tumor metastasis (100). In NB, galectin-1 (Gal-1) has emerged as an interesting target. It regulates complex signaling pathways involved in tumor–host interaction (101) and angiogenesis (102). Tumor secretion of Gal-1 contributes to the immunosuppressive potential of a wide range of tumors by limiting T-cell survival and impairing DC function (103–105). NB tumors, particularly those with poor prognosis, express high levels of Gal-1 (106). The neurotrophin receptor TrkB is also expressed in NB with poor prognosis, conferring invasive and metastatic potential to the tumor cells as well as enhancing therapy resistance. Blocking Gal-1 function strongly reduced the migratory and invasive capacities of TrkB expressing NB cells (106). Soluble Gal-1 was found to be actively secreted by different NB cell lines (107). The authors found that cancer-derived Gal-1 modulates T-cell and DC, resulting in increased tumor growth and metastasis. Interestingly, Gal-1 blockage efficiently inhibits primary tumor growth and metastasis, strongly suggesting that Gal-1 could be a therapeutic target in NB. Similarly, inhibition of Gal-1 function resulted in tumor rejection in other animal models (108, 109).
Clinical outcome for high-risk NB patients remains poor in spite of multimodal treatment including chemoradiotherapy, surgery, and stem cell transplantation. Because osteomedullary recurrences are frequent in patients with NB, the development of sensitive and specific methods to detect rare tumor cells in bone marrow or peripheral blood is important, both for risk assessment at diagnosis and for evaluating response to therapy. Conventional cytology, traditionally used for occult disseminated disease detection, has limited sensitivity and was improved by immunological strategies (110). However, the effectiveness of cell-surface antigen detection largely depends on epitope exposure and accessibility to antibodies, so techniques based on amplified mRNA detection have demonstrated a higher sensitivity for this purpose (111).
As stated above, cancer hallmark aberrant glycosylation is associated with differential expression of antigens as well as some enzymes, such as glycosyltransferases and glycosidases. In the personalized approach to cancer diagnosis and treatment, these enzymes are proving to have clinical relevance as cancer biomarkers for different tumors (112). In this study, we show results reported for NB patients (Table 1). GD2 synthase (β1,4-N-acetylgalactosaminyltransferase, EC 2.4.1.92), the key enzyme for GD2 synthesis, has been largely evaluated, both by immunological approaches as well as by nucleic acid techniques. The first monoclonal antibodies specific for this enzyme, which date from more than two decades ago, demonstrated a high specificity and sensibility of 0.01% for neuroblast detection in bone marrow samples (113). However, mRNA techniques (RT-PCR analysis) may further improve detection of rare NB cells, and highly sensitive and specific methods that measure GD2 synthase mRNA have been reported (111, 114). Cheung et al. communicated its clinical utility in evaluating adjuvant therapy in NB patients (115), although it seems less specific as a prognostic marker at diagnosis. In a comparative and prospective study of a consecutive patient cohort, Träger et al. analyzed the clinical significance of tyrosine hydroxylase (TH), dopa decarboxylase (DDC), and GD2 synthase transcripts (116). They found that high expression of TH and DDC, both in peripheral blood and bone marrow corresponds to metastatic NB at diagnosis, residual disease, and poor outcome, while GD2 is less specific at the mRNA level for NB detection. In the same way, the International Neuroblastoma Risk Group Task Force, in an effort to achieve a consensus on standard operating procedures in order to improve and standardize management of children with NB, agreed on the detection of rare neuroblasts in bone marrow, peripheral blood, and peripheral blood stem cells by immunocytochemistry using GD2 and by QRT-PCR for TH mRNA (117).
However, despite the increased sensitivity of those methods, it is clear that not all patients with disseminated disease are detected, reflecting limitations in the procedures. This could be due to analysis of a small volume of sample and also to limitations as a consequence of tumor heterogeneity, which could possibly be solved by multi-marker assays. Therefore, research for novel specific markers is warranted and investigators are working in this direction. Cheung et al. hypothesize that sialyltransferase STX (ST8SiaII), the key enzyme for PSA synthesis, can potentially be a sensitive marker for metastatic NB (93). As stated above, PSA is critical for cellular adhesion, neuronal migration, and tumor metastasis, and it is highly expressed in many human cancers, including NB. Since the enzyme STX has restricted expression in postnatal tissues, including spleen and leukocytes, and because of its up-regulation during dedifferentiation in a number of human cancers, it could be a potential marker for metastatic tumor cells in blood or bone marrow. The authors optimized a quantitative RT-PCR in order to evaluate STX expression in tumor samples, cell lines, and bone marrow from a cohort of high-risk NB patients enrolled in a post-induction immunotherapy protocol utilizing anti-GD2 antibody 3F8. Patient follow-up demonstrated that STX positivity and its transcript level were highly prognostic for PFS (p < 0.0005) (93). However, like TH and GD2 synthase, the utility of STX as a single marker is constrained by the inherent heterogeneity of human NB, so its utility is expected to improve if combined into a marker panel.
N-acetylglucosaminyltransferase V (GnT-V) is one of the most relevant glycosyltransferases associated to tumor invasion and metastasis. Previous studies demonstrated that an increased amount of β1,6-branched oligosaccharides, formed by the action of GnT-V, is correlated with metastatic potential (118). This enzyme has been associated to tumor progression in human breast and colon neoplasia (119), and could be a prognostic marker in human colorectal carcinoma (120, 121). However, for lung cancer, Dosaka-Akita et al. reported that the lower expression of GnT-V is associated with shorter survival and poor prognosis (122). Regarding NB, assessing GnT-V mRNA expression by real-time PCR, a significant correlation was observed between higher expression levels and a favorable prognosis in a cohort of 126 patients (123). GnT-V-knockdown in NB cells showed a tendency to escape from retinoic acid-induced apoptosis, supporting the notion that a higher expression of GnT-V is correlated with a favorable prognosis of NB patients.
Novel techniques in marker discovery, such as genome-wide gene expression array analyses, have been successfully applied to NB, leading to identification of useful markers for minimal residual disease diagnosis (124) and prognostic markers (125, 126). Using the same strategy, we studied the transcriptome profiles of malignant neuroblasts established from the human MYCN-amplified IGR-N-91 model (127). Comparative gene expression profiles obtained with Agilent oligo microarrays, between primary tumor versus bone marrow metastatic cell lines, revealed a set of 107 differentially expressed genes in the metastatic neuroblasts (58). Up-regulated genes were involved in chemoresistance, cell motility, and neuronal structure/signaling, and surprisingly, the most strongly up-regulated gene was GALNT13, followed by ABCB1, whose higher expression has been previously described (127, 128) and highlights the fact that acquired drug resistance is an important cause of NB treatment failure. GALNT13 encodes the UDP-GalNAc:polypeptide GalNAc-transferase-13 (GalNAc-T13), described as specifically expressed in neuronal tissue (129), belonging to the enzyme family which catalyzes the first step of mucin-type O-glycosylation (54). Our analysis of GALNT13 mRNA transcripts in various NB cell lines, as well as in favorable and high-risk neuroblastic tumors, showed that GALNT13 is highly expressed in more aggressive neuroblasts but is not correlated with MYCN amplification and/or expression. Looking for a potential marker for NB disseminated cells, bone marrow samples harvested at diagnosis of NB patients with different stages of the disease were analyzed for GALNT13 expression, and compared to other proposed markers (TH, GD2 synthase, DDC, and conventional cytology). Overall survival by Kaplan–Meier analysis demonstrated the best correlation with a poor clinical outcome for GALNT13 expression (58). We then proposed GalNAc-T13 as a new informative marker for the molecular diagnosis of BM involvement and the follow-up of minimal residual disease in NB patients. However, to elucidate the biological role of GALNT13 in NB, further work is needed to analyze the molecular mechanisms that regulate this gene’s expression and to identify the enzyme acceptor substrates potentially involved in metastatic activity of these cells.
Performing a GALNTs expression profile in several NB cell lines, we observed that a few enzymes showed an opposite expression pattern in some of them. GALNT9, described by Toba et al. as restricted to the brain (130), was found expressed in neuroblasts derived from the primary tumor in the experimental model IGR-N-91, but not in neuroblasts derived from bone marrow metastases (57). The expression profile in different NB cell lines was restricted to substrate adherent (S)-type ones, exhibiting weaker tumorigenesis, invasiveness, and metastatic properties (131), while it was always negative in more aggressive neuronal (N)-type cell lines. When a tumor cohort from 122 NB patients was analyzed, GALNT9 expression was associated with high overall survival, independently of the standard risk-stratification covariates, and it was significantly associated with disease-free survival for patients currently classified as low risk (57). GALNT9 expression is probably a marker for more mature stages of neuroblastic tumor cells, which are associated with less aggressivity. This isoenzyme belongs to a subfamily among GALNT genes, together with GALNT8, GALNT18, and GALNT19, and exhibits significant sequence differences from the other members of the GALNT family, and also a low activity in a small number of peptides (54). This fact, together with the opposite expression of GALNT13, up-regulated in more aggressive neuroblasts, lead to the hypothesis that these enzymes could have biological relevance in NB behavior. They could be candidates for a panel of tumor markers to be included in large prospective cooperative multi-center studies, performed according to the INRG standard operating procedures (117).
Another interesting glycosyltransferase, β1,3-N-acetylglucos aminyltransferase-3 (B3GNT3), is a member of the β3GlcNAc-T family, composed of at least eight isoenzymes, and responsible for adding GlcNAc to core 1 (T antigen) in a β1,3 linkage, forming extended core 1 oligosaccharides (132). This enzyme is expressed in lymphocytes and neutrophils, and could contribute to the expression of core 1-derived O-glycans, which play an essential role in E-selectin adhesion (133). It has been demonstrated, in a colon cancer experimental model, that E- and P-selectin are essential protagonists in cell–cell adhesion by means of glycosylated ligands in tumor cell-surface, which are responsible for lung metastatic development (134). Gakhar et al. suggested a similar mechanism as responsible for the attachment of glycoproteins on the surface of prostate cancer circulating tumor cells, acting as functional ligands for E-selectin expressed on endothelial cells (135). However, contrary to what one might expect, in NB, B3GNT3 expression has been correlated with favorable clinical outcome (59). Increased B3GNT3 expression in NB tumor tissues correlated well with the histological grade of differentiation, while undifferentiated tumors remain more frequently negative. Univariate and multivariate analyses revealed that positive B3GNT3 expression in tumor tissues predicted a better survival of NB patients, independently of other prognostic markers (59). In the same work, cell line experiments expressing and knocking down the enzyme demonstrated that B3GNT3 expression significantly correlated with suppression of core 1 expression, as well as malignant phenotypes including migration and invasion.
β1,4-N-acetylgalactosaminyltransferase III (B4GALNT3) cloned by Sato et al., has been described as expressed in stomach, colon, and testis (136). This enzyme can transfer GalNAc residues to non-reducing terminal GlcNAc-β leading the synthesis of GalNAcβ1–4GlcNAc (also known as LacdiNAc or LDN), which is a unique terminal structure in the outer chain moieties of human N-glycans (137), and also in O-linked oligosaccharide structures (138). The largest amount of B4GALNT3 transcripts were found in gastric tissues, followed by colon, testis, and adrenal glands (136). Gastric expression of B4GALNT3 was found regulated by cellular differentiation (139). In the human colon, Huang et al. reported that B4GALNT3 is up-regulated in primary tumors comparing with the normal mucosa (140). They performed in vitro and in vivo experiments showing that overexpression of this enzyme increases malignant phenotype of colon cancer cells, and these phenotypic changes are associated with enhanced integrin and mitogen-activated protein kinase (MAPK) signaling, suggesting that B4GALNT3 may play a crucial role in promoting malignant behavior of colon cancer (140). However, the same research team has recently published that B4GALNT3 overexpression in colorectal cancer cells suppressed cell migration, invasion, and adhesion, while B4GALNT3 knockdown enhanced malignant cell phenotypes promoting cell migration and invasion (141). Surprisingly, a similar situation was found for NB. Firstly, Hsu et al. communicated that B4GALNT3 expression positively correlates with the differentiation status of NB, predicting a favorable prognosis for patients and suppressing the malignant phenotype in cell lines experiments via decreasing β1-integrin signaling (142). However, opposite results were reported 2 years later by the same group and for the same cohort of patients, showing B4GALNT3 expression in NB tumors correlating with advanced clinical stage, unfavorable histology, and lower survival rate (143). In face of this controversy, the role of B4GALNT3 in NB is not clear, and further work is necessary to elucidate the real clinical significance of the enzyme in tumor behavior and subjacent molecular mechanisms.
The choice of NB treatment depends on patient risk group stratification. While some carefully selected cases with favorable features could benefit from observation alone (144), usually high-risk patients are subject to multimodal aggressive therapeutic plans (including surgery, chemotherapy, radiotherapy, and immunotherapy) (145). Clinical outcome for high-risk groups remains poor due to frequent recurrence of osteomedullary disease. The identification of newer tumor targets and immunotherapy strategies may generate novel therapeutic approaches which could combine to improve survival and cure rates. Rational approaches to target ALK and MYCN, which arise from the two most common and potentially significant genetic alterations in NB, are emerging and we must expect that some therapeutic options will become available in the near future (146).
Immunotherapy is intended to redirect the immune system to target tumors and tumor-associated antigens, leading to the elimination of malignant cells. Multiple forms of immunotherapy are being used in clinics, including monoclonal antibodies targeting tumor cell-surface antigens or disrupting the normal checkpoints that inhibit anti-tumor immune responses, cytokines that modify innate or adaptive immunity, tumor vaccines, as well as adoptive cellular therapies (147–151). There is increasing data regarding more efficient immunotherapeutic protocols designed based on cancer-associated glycan structures (152). NB provides an attractive target for immunotherapy as it expresses certain glycan antigens not widely detected in non-embryonic tissues, such as GD2 and O-acetyl GD2 (153). In fact, the National Cancer Institute pilot program for the prioritization of the most important cancer antigens ranks GD2 as 12 out of 75 potential targets for cancer therapy (154). Anti-GD2 antibodies have been actively tested over the past two decades in clinical trials for NB, and have emerged as a major therapeutic development for high-risk cases, with proven safety and efficacy (155, 156). In particular, four anti-GD2 antibodies (3F8, hu3F8, ch14.18, and hu14.18) have been extensively tested in clinics (157). The murine IgG3 monoclonal antibody (MAb) 3F8 was the first well-characterized anti-GD2 antibody (158). Its efficacy in treating NB was initially described in 1987 in the report of a Phase 1 trial which included patients with refractory high-risk NB (159). MAb 3F8 mediates highly efficient antibody-dependent cell-mediated cytotoxicity (ADCC) of NB in the presence of human natural killer (NK) cells and granulocytes in vitro (160–162). Moreover, it induces complement-mediated cytotoxicity (CMC), because NB cells lack decay-accelerating factor CD55 (163) and homologous restriction factor CD59 (164). IL-2 and GM-CSF were shown to enhance ADCC in vitro by activating cytotoxic NK cells and neutrophils (160, 165, 166). When combined with the cytokine GM-CSF and 13-cis-retinoic acid, 3F8 induced >60% long-term survival among high-risk patients with metastatic disease, treated at first remission (167). MAb 3F8 was recently humanized (hu3F8) (168) and is currently in Phase I trials (157). MAb 14.G2a is an IgG2a class switch variant of MAb 14.18, originally isolated as an IgG3 isotype (169). 14.G2a showed higher in vitro and in vivo ADCC than 14.18 and was subsequently modified for clinical development. MAb 14.G2a was chimerized to form ch14.18 and humanized to form hu14.18 (named after the original mouse 14.18 IgG3 isotype) (170). A phase III randomized trial showed that ch14.18, when combined with GM-CSF and interleukin-2, in high-risk NB patients, was associated with significantly improved survival compared to standard therapy after a 2-year follow-up period (171).
Several approaches have been developed to enhance anti-tumor efficacy of anti-GD2 monoclonal antibodies and fragments: immunocytokines (172), immunotoxins (173), antibody drug conjugates (174), radiolabeled antibodies (175), targeted nanoparticles (176), T-cell engaging bispecific antibodies, and chimeric antigen receptors (CARs) (157). Although the CAR technology is still at an early stage, clinical trials have already shown significant anti-tumor activity in NB patients. In this approach, antibody and cell-based immunotherapy of cancer has converged in the development of engineered T-cells which express the antigen binding site of a MAb (commonly an antibody-derived single-chain fragment) coupled with the intracellular signaling portion of the T-cell receptor (TCR) (177, 178). A key advantage is that CARs target native, rather than processed antigens; consequently, their function is not hampered by HLA downregulation, frequently observed in human cancer (179). CAR-T-cells targeting cancer-associated ganglioside antigens such as GD2 (180) or GD3 (181) have been developed. A chimeric GD2-specific receptor on T lymphocytes exhibited in vitro anti-melanoma activity and increased survival of mice xenografted with a human melanoma cell line (182). Cytotoxic T lymphocytes (CTLs) expressing a chimeric GD2-specific receptor were generated using the Epstein-Barr virus (183). Infusion of these genetically modified cells (CAR-CTL anti-GD2) was associated with tumor regression or necrosis in half of the tested patients (183). After that, Louis et al. reported complete remission of 3 out of 11 patients with active disease treated with CAR-CTL anti-GD2 infusions, on a long-term clinical and immunologic follow-up (184).
Different molecules involved in NB glycobiology play key roles in tumor growth and are potential targets for anti-tumor therapy (Figure 2). It is well-established that gangliosides and PSA-NCAM impact in the aggressiveness of NB cells as well as in the patients’ clinical outcome. Some evidence suggests that enzymes which catalyze O-glycan biosynthesis could play a role regulating NB behavior, but the molecular basis of these observations remain to be established. Some aspects of NB glycobiology do not only affect the tumor-cell phenotype (e.g., proliferation, differentiation, and adhesion), but also contribute to local microenvironment and immune response control. For example, NB-derived gangliosides inhibit the generation of functionally active DCs, leading to an increase of Treg cells inside the tumor, which suppress effective anti-tumor responses. In the same way, Gal-1 secretion by NB cells stimulates tumor growth by inducing local immunosuppressive activities and angiogenesis. This is relevant because Gal-1 has been characterized as a promising target for therapy in cancer models, using synthetic and natural inhibitors. Virtually all NB cells express ganglioside GD2. This is a relevant antigen for anti-tumor immunotherapy strategies because it is highly expressed in NB cell-surface, and the blood–brain barrier limits the side effects due to normal expression in neuronal cells. Anti-GD2 antibody (ch14.18) combined with IL-2 and GM-CSF represent the latest major therapeutic advance for high-risk NB in the last decade. This immunotherapy was demonstrated to be safe as well as a key component to achieve cure or long-term remission in patients with residual disease. In addition, recent clinical success has underscored the potential of NB immunotherapy based on the adoptive cell transfer of engineered T lymphocytes (CAR-CTL anti-GD2) in order to mediate strong and durable clinical responses. Several approaches may further enhance anti-tumor activity and persistence of circulating CAR-modified cells. Safe transfer of CAR-based immunotherapy into clinical practice could represent a potential alternative to conventional treatment options for NB patients.
Figure 2. Schematic representation of NB glycobiology impact on tumor growth and anti-tumor therapy. Treatment with retinoic acid markedly enhances the activity of GD1b/GM1a synthase, resulting in increased expression of complex gangliosides, associated with less-aggressive tumors. NB gangliosides promote dendritic cell (DC) to develop with decreased costimulatory signals and IL-12 production. These DC promote differentiation of Th0 cells toward regulatory T-cells (Treg). Gal-1 secreted by NB also contributes to the immunosuppressive tumor microenvironment, limiting T-cell survival and impairing DC function. Both, gangliosides and Gal-1 contribute to tumor angiogenesis. The presence of polysialic acid (PSA) on NCAM reduces NCAM-mediated adhesion processes promoting NB cell migration. The fact that STX is the dominant polysialyltransferase for PSA biosynthesis in NB suggests that this enzyme could be a good therapeutic target. GD2 is a relevant antigen for NB immunotherapy. Anti-tumor activity of anti-GD2 antibodies is mediated by antibody-dependent cell-mediated cytotoxicity (ADCC) in the presence of human natural killer (NK) cells and granulocytes, as well as by complement-mediated cytotoxicity (CMC). Anti-GD2 chimeric antigen receptor T-cells (CAR-T-cells) activity could induce NB tumor regression.
The authors declare that the research was conducted in the absence of any commercial or financial relationships that could be construed as a potential conflict of interest.
We thank all present and past members of our group and our collaborators who contributed to the results reported in this manuscript, especially Dr. Jean Bénard. Work in the author’s laboratory was supported by Programa Grupos de Investigación (CSIC, Universidad de la República, Uruguay) and it was partially funded by FOCEM (MERCOSUR Structural Convergence Fund), COF 03/11.
1. Park JR, Eggert A, Caron H. Neuroblastoma: biology, prognosis, and treatment. Hematol Oncol Clin North Am (2010) 24:65–86. doi: 10.1016/j.hoc.2009.11.011
2. Brodeur GM. Neuroblastoma: biological insights into a clinical enigma. Nat Rev Cancer (2003) 3:203–16. doi:10.1038/nrc1014
3. Navarro S, Piqueras M, Villamón E, Yáñez Y, Balaguer J, Cañete A, et al. New prognostic markers in neuroblastoma. Expert Opin Med Diagn (2012) 6:555–67. doi:10.1517/17530059.2012.704018
4. Shimada H, Umehara S, Monobe Y, Hachitanda Y, Nakagawa A, Goto S, et al. International neuroblastoma pathology classification for prognostic evaluation of patients with peripheral neuroblastic tumors: a report from the Children’s Cancer Group. Cancer (2001) 92:2451–61. doi:10.1002/1097-0142(20011101)92:9<2451::AID-CNCR1595>3.0.CO;2-S
5. Bagatell R, Beck-Popovic M, London WB, Zhang Y, Pearson AD, Matthay KK, et al. Significance of MYCN amplification in international neuroblastoma staging system stage 1 and 2 neuroblastoma: a report from the International Neuroblastoma Risk Group database. J Clin Oncol (2009) 27:365–70. doi:10.1200/JCO.2008.17.9184
6. Carén H, Kryh H, Nethander M, Sjöberg RM, Träger C, Nilsson S, et al. High-risk neuroblastoma tumors with 11q-deletion display a poor prognostic, chromosome instability phenotype with later onset. Proc Natl Acad Sci U S A (2010) 107:4323–8. doi:10.1073/pnas.0910684107
7. Rubie H, De Bernardi B, Gerrard M, Canete A, Ladenstein R, Couturier J, et al. Excellent outcome with reduced treatment in infants with nonmetastatic and unresectable neuroblastoma without MYCN amplification: results of the prospective INES 99.1. J Clin Oncol (2011) 29:449–55. doi:10.1200/JCO.2010.29.5196
8. Canete A, Gerrard M, Rubie H, Castel V, Di Cataldo A, Munzer C, et al. Poor survival for infants with MYCN-amplified metastatic neuroblastoma despite intensified treatment: the International Society of Paediatric Oncology European Neuroblastoma Experience. J Clin Oncol (2009) 27:1014–9. doi:10.1200/JCO.2007.14.5839
9. Strother DR, London WB, Schmidt ML, Brodeur GM, Shimada H, Thorner P, et al. Outcome after surgery alone or with restricted use of chemotherapy for patients with low-risk neuroblastoma: results of Children’s Oncology Group study P9641. J Clin Oncol (2012) 30:1842–8. doi:10.1200/JCO.2011.37.9990
10. Monclair T, Brodeur GM, Ambros PF, Brisse HJ, Cecchetto G, Holmes K, et al. The International Neuroblastoma Risk Group (INRG) staging system: an INRG Task Force report. J Clin Oncol (2009) 27:298–303. doi:10.1200/JCO.2008.16.6876
11. Cohn SL, Pearson AD, London WB, Monclair T, Ambros PF, Brodeur GM, et al. The International Neuroblastoma Risk Group (INRG) classification system: an INRG Task Force report. J Clin Oncol (2009) 27:289–97. doi:10.1200/JCO.2008.16.6785
12. Park JR, Bagatell R, London WB, Maris JM, Cohn SL, Mattay KM, et al. Children’s Oncology Group’s 2013 blueprint for research: neuroblastoma. Pediatr Blood Cancer (2013) 60:985–93. doi:10.1002/pbc.24433
13. Hakomori S. Tumor-associated carbohydrate antigens defining tumor malignancy: basis for development of anti-cancer vaccines. Adv Exp Med Biol (2001) 491:369–402. doi:10.1007/978-1-4615-1267-7_24
14. Allende ML, Proia RL. Lubricating cell signaling pathways with gangliosides. Curr Opin Struct Biol (2002) 12:587–92. doi:10.1016/S0959-440X(02)00376-7
15. Todeschini AR, Hakomori SI. Functional role of glycosphingolipids and gangliosides in control of cell adhesion, motility, and growth, through glycosynaptic microdomains. Biochim Biophys Acta (2008) 1780:421–33. doi:10.1016/j.bbagen.2007.10.008
16. Hakomori S. Tumor malignancy defined by aberrant glycosylation and sphingo(glyco)lipid metabolism. Cancer Res (1996) 56:5309–18.
17. Ledeen RW, Yu RK. Gangliosides: structure, isolation, and analysis. Methods Enzymol (1982) 83:139–91. doi:10.1016/0076-6879(82)83012-7
18. van Echten G, Sandhoff K. Ganglioside metabolism. Enzymology, topology, and regulation. J Biol Chem (1993) 268:5341–4.
19. Dong L, Liu Y, Colberg-Poley AM, Kaucic K, Ladisch S. Induction of GM1a/GD1b synthase triggers complex ganglioside expression and alters neuroblastoma cell behavior; a new tumor cell model of ganglioside function. Glycoconj J (2011) 28:137–47. doi:10.1007/s10719-011-9330-9
20. Kaucic K, Etue N, LaFleur B, Woods W, Ladisch S. Neuroblastomas of infancy exhibit a characteristic ganglioside pattern. Cancer (2001) 91:785–93. doi:10.1002/1097-0142(20010215)91:4<785::AID-CNCR1065>3.0.CO;2-R
21. Hettmer S, Malott C, Woods W, Ladisch S, Kaucic K. Biological stratification of human neuroblastoma by complex ‘B’ pathway ganglioside expression. Cancer Res (2003) 63:7270–6.
22. Schengrund CL, Repman MA, Shochat SJ. Ganglioside composition of human neuroblastomas. Correlation with prognosis. A Pediatric Oncology Group Study. Cancer (1985) 56:2640–6. doi:10.1002/1097-0142(19851201)56:11<2640::AID-CNCR2820561118>3.0.CO;2-W
24. Leskawa KC, Hogan EL. Quantitation of the in vitro neuroblastoma response to exogenous, purified gangliosides. J Neurosci Res (1985) 13:539–50. doi:10.1002/jnr.490130409
25. Mirkin BL, Clark SH, Zhang C. Inhibition of human neuroblastoma cell proliferation and EGF receptor phosphorylation by gangliosides GM1, GM3, GD1A and GT1B. Cell Prolif (2002) 35:105–15. doi:10.1046/j.1365-2184.2002.00228.x
26. Matthay KK, Villablanca JG, Seeger RC, Stram DO, Harris RE, Ramsay NK, et al. Treatment of high-risk neuroblastoma with intensive chemotherapy, radiotherapy, autologous bone marrow transplantation, and 13-cisretinoic acid. Children’s Cancer Group. N Engl J Med (1999) 341:1165–73. doi:10.1056/NEJM199910143411601
27. Hettmer S, McCarter R, Ladisch S, Kaucic K. Alterations in neuroblastoma ganglioside synthesis by induction of GD1b synthase by retinoic acid. Br J Cancer (2004) 91:389–97. doi:10.1038/sj.bjc.6601914
28. Wu ZL, Schwartz E, Seeger R, Ladisch S. Expression of GD2 ganglioside by untreated primary human neuroblastomas. Cancer Res (1986) 46:440–3.
29. Modak S, Cheung NK. Disialoganglioside directed immunotherapy of neuroblastoma. Cancer Invest (2007) 25:67–77. doi:10.1080/07357900601130763
30. Cheresh DA, Pierschbacher MD, Herzig MA, Mujoo K. Disialogangliosides GD2 and GD3 are involved in the attachment of human melanoma and neuroblastoma cells to extracellular matrix proteins. J Cell Biol (1986) 102:688–96. doi:10.1083/jcb.102.3.688
31. Li R, Ladisch S. Shedding of human neuroblastoma gangliosides. Biochim Biophys Acta (1991) 1083:57–64. doi:10.1016/0005-2760(91)90124-Z
32. Valentino L, Moss T, Olson E, Wang HJ, Elashoff R, Ladisch S. Shed tumor gangliosides and progression of human neuroblastoma. Blood (1990) 75:1564–7.
33. Olshefski R, Ladisch S. Intercellular transfer of shed tumor cell gangliosides. FEBS Lett (1996) 386:11–4. doi:10.1016/0014-5793(96)00392-4
34. Hakomori S. The glycosynapse. Proc Natl Acad Sci U S A (2002) 99:225–32. doi:10.1073/pnas.012540899
35. Kaucic K, Liu Y, Ladisch S. Modulation of growth factor signaling by gangliosides: positive or negative? Methods Enzymol (2006) 417:168–85. doi:10.1016/S0076-6879(06)17013-5
36. Liu Y, McCarthy J, Ladisch S. Membrane ganglioside enrichment lowers the threshold for vascular endothelial cell angiogenic signaling. Cancer Res (2006) 66:10408–14. doi:10.1158/0008-5472.CAN-06-1572
37. Liu Y, Wondimu A, Yan S, Bobb D, Ladisch S. Tumor gangliosides accelerate murine tumor angiogenesis. Angiogenesis (2013). doi:10.1007/s10456-013-9403-4
38. Potapenko M, Shurin GV, de León J. Gangliosides as immunomodulators. Adv Exp Med Biol (2007) 601:195–203. doi:10.1007/978-0-387-72005-0_20
39. Li R, Gage D, McKallip R, Ladisch S. Structural characterization and in vivo immunosuppressive activity of neuroblastoma GD2. Glycoconj J (1996) 13:385–9. doi:10.1007/BF00731471
40. Li R, Ladisch S. Abrogation of shedding of immunosuppressive neuroblastoma gangliosides. Cancer Res (1996) 56:4602–5.
41. Lee HC, Wondimu A, Liu Y, Ma JS, Radoja S, Ladisch S. Ganglioside inhibition of CD8+ T cell cytotoxicity: interference with lytic granule trafficking and exocytosis. J Immunol (2012) 189:3521–7. doi:10.4049/jimmunol.1201256
42. Nicoll G, Avril T, Lock K, Furukawa K, Bovin N, Crocker PR. Ganglioside GD3 expression on target cells can modulate NK cell cytotoxicity via siglec-7-dependent and -independent mechanisms. Eur J Immunol (2003) 33:1642–8. doi:10.1002/eji.200323693
43. Redlinger RE Jr, Mailliard RB, Barksdale EM Jr. Neuroblastoma and dendritic cell function. Semin Pediatr Surg (2004) 13:61–71. doi:10.1053/j.sempedsurg.2003.09.009
44. Shurin GV, Shurin MR, Bykovskaia S, Shogan J, Lotze MT, Barksdale EM Jr. Neuroblastoma-derived gangliosides inhibit dendritic cell generation and function. Cancer Res (2001) 61:363–9.
45. Walker SR, Redlinger RE Jr, Barksdale EM Jr. Neuroblastoma-induced inhibition of dendritic cell IL-12 production via abrogation of CD40 expression. J Pediatr Surg (2005) 40:244–9. doi:10.1016/j.jpedsurg.2004.09.050
46. Shen W, Stone K, Jales A, Leitenberg D, Ladisch S. Inhibition of TLR activation and up-regulation of IL-1R-associated kinase-M expression by exogenous gangliosides. J Immunol (2008) 180:4425–32. doi:10.4049/jimmunol.180.7.4425
47. Jales A, Falahati R, Mari E, Stemmy EJ, Shen W, Southammakosane C, et al. Ganglioside-exposed dendritic cells inhibit T-cell effector function by promoting regulatory cell activity. Immunology (2011) 132:134–43. doi:10.1111/j.1365-2567.2010.03348.x
48. Yoon KJ, Phelps DA, Bush RA, Remack JS, Billups CA, Khoury JD. ICAM-2 expression mediates a membrane-actin link, confers a nonmetastatic phenotype and reflects favorable tumor stage or histology in neuroblastoma. PLoS One (2008) 3:e3629. doi:10.1371/journal.pone.0003629
49. Feduska JM, Garcia PL, Brennan SB, Bu S, Council LN, Yoon KJ. N-glycosylation of ICAM-2 is required for ICAM-2-mediated complete suppression of metastatic potential of SK-N-AS neuroblastoma cells. BMC Cancer (2013) 13:261. doi:10.1186/1471-2407-13-261
50. Mossé YP, Laudenslager M, Longo L, Cole KA, Wood A, Attiyeh EF, et al. Identification of ALK as a major familial neuroblastoma predisposition gene. Nature (2008) 455:930–5. doi:10.1038/nature07261
51. Passoni L, Longo L, Collini P, Coluccia AM, Bozzi F, Podda M, et al. Mutation-independent anaplastic lymphoma kinase overexpression in poor prognosis neuroblastoma patients. Cancer Res (2009) 69:7338–46. doi:10.1158/0008-5472.CAN-08-4419
52. George RE, Sanda T, Hanna M, Fröhling S, Luther W, Zhang J, et al. Activating mutations in ALK provide a therapeutic target in neuroblastoma. Nature (2008) 455:975–8. doi:10.1038/nature07397
53. Del Grosso F, De Mariano M, Passoni L, Luksch R, Tonini GP, Longo L. Inhibition of N-linked glycosylation impairs ALK phosphorylation and disrupts pro-survival signaling in neuroblastoma cell lines. BMC Cancer (2011) 11:525. doi:10.1186/1471-2407-11-525
54. Bennett EP, Mandel U, Clausen H, Gerken TA, Fritz TA, Tabak LA. Control of mucin-type O-glycosylation: a classification of the polypeptide GalNAc-transferase gene family. Glycobiology (2012) 22:736–56. doi:10.1093/glycob/cwr182
55. Dube DH, Bertozzi CR. Glycans in cancer and inflammation – potential for therapeutics and diagnostics. Nat Rev Drug Discov (2005) 4:477–88. doi:10.1038/nrd1751
56. Zhang S, Zhang HS, Cordon-Cardo C, Reuter VE, Singhal AK, Lloyd KO, et al. Selection of tumor antigens as targets for immune attack using immunohistochemistry: II. Blood group-related antigens. Int J Cancer (1997) 73:50–6. doi:10.1002/(SICI)1097-0215(19970926)73:1<42::AID-IJC8>3.0.CO;2-1
57. Berois N, Gattolliat CH, Barrios E, Capandeguy L, Douc-Rasy S, Valteau-Couanet D, et al. GALNT9 gene expression is a prognostic marker in neuroblastoma patients. Clin Chem (2013) 59:225–33. doi:10.1373/clinchem.2012.192328
58. Berois N, Blanc E, Ripoche H, Mergui X, Trajtenberg F, Cantais S, et al. ppGalNAc-T13: a new molecular marker of bone marrow involvement in neuroblastoma. Clin Chem (2006) 52:1701–12. doi:10.1373/clinchem.2006.067975
59. Ho WL, Che MI, Chou CH, Chang HH, Jeng YM, Hsu WM, et al. B3GNT3 expression suppresses cell migration and invasion and predicts favorable outcomes in neuroblastoma. Cancer Sci (2013) 104:1600–8. doi:10.1111/cas.12294
60. Finne J, Finne U, Deagostini-Bazin H, Goridis C. Occurrence of alpha 2-8 linked polysialosyl units in a neural cell adhesion molecule. Biochem Biophys Res Commun (1983) 112:482–7. doi:10.1016/0006-291X(83)91490-0
61. Santoni MJ, Goridis C, Fontecilla-Camps JC. Molecular modelling of the immunoglobulin-like domains of the neural cell adhesion molecule (NCAM): implications for the positioning of functionally important sugar side chains. J Neurosci Res (1988) 20:304–10. doi:10.1002/jnr.490200304
62. Mühlenhoff M, Eckhardt M, Gerardy-Schahn R. Polysialic acid: three-dimensional structure, biosynthesis and function. Curr Opin Struct Biol (1998) 8:558–64. doi:10.1016/S0959-440X(98)80144-9
63. Sadoul R, Hirn M, Deagostini-Bazin H, Rougon G, Goridis C. Adult and embryonic mouse neural cell adhesion molecules have different binding properties. Nature (1983) 304:347–9. doi:10.1038/304347a0
64. Johnson CP, Fujimoto I, Rutishauser U, Leckband DE. Direct evidence that neural cell adhesion molecule (NCAM) polysialylation increases intermembrane repulsion and abrogates adhesion. J Biol Chem (2005) 280:137–45. doi:10.1074/jbc.M410216200
65. Franceschini I, Angata K, Ong K, Hong A, Doherty P, Fukuda M. Polysialyltransferase ST8Sia II (STX) polysialylates all of the major isoforms of NCAM and facilitates neurite outgrowth. Glycobiology (2001) 11:231–9. doi:10.1093/glycob/11.3.231
66. Gascon E, Vutskits L, Kiss JK. Polysialic acid-neural cell adhesion molecule in brain plasticity: from synapses to integration of new neurons. Brain Res Rev (2007) 56:101–18. doi:10.1016/j.brainresrev.2007.05.014
67. Winter C, Pawel B, Seiser E, Zhao H, Raabe E, Wang Q, et al. Neural cell adhesion molecule (NCAM) isoform expression is associated with neuroblastoma differentiation status. Pediatr Blood Cancer (2008) 51:10–6. doi:10.1002/pbc.21475
68. Hildebrandt H, Muhlenhoff M, Weinhold B, Gerardy-Schahn R. Dissecting polysialic acid and NCAM functions in brain development. J Neurochem (2007) 103(Suppl 1):56–64. doi:10.1111/j.1471-4159.2007.04716.x
69. Rutishauser U, Landmesser L. Polysialic acid in the vertebrate nervous system: a promoter of plasticity in cell-cell interactions. Trends Neurosci (1996) 19:422–7. doi:10.1016/0166-2236(96)10041-2
70. Oltmann-Norden I, Galuska SP, Hildebrandt H, Geyer R, Gerardy-Schahn R, Geyer H, et al. Impact of the polysialyltransferases ST8SiaII and ST8SiaIV on polysialic acid synthesis during postnatal mouse brain development. J Biol Chem (2008) 283:1463–71. doi:10.1074/jbc.M708463200
71. Lipinski M, Hirsch MR, Deagostini-Bazin H, Yamada O, Tursz T, Goridis C. Characterization of neural cell adhesion molecules (NCAM) expressed by Ewing and neuroblastoma cell lines. Int J Cancer (1987) 40:81–6. doi:10.1002/ijc.2910400115
72. Figarella-Branger DF, Durbec PL, Rougon GN. Differential spectrum of expression of neural cell adhesion molecule isoforms and L1 adhesion molecules on human neuroectodermal tumors. Cancer Res (1990) 50:6364–70.
73. Tanaka F, Otake Y, Nakagawa T, Kawano Y, Miyahara R, Li M, et al. Expression of polysialic acid and STX, a human polysialyltransferase, is correlated with tumor progression in non-small cell lung cancer. Cancer Res (2000) 60:3072–80.
74. Glüer S, Schelp C, von Schweinitz D, Gerardy-Schahn R. Polysialylated neural cell adhesion molecule in childhood rhabdomyosarcoma. Pediatr Res (1998) 43:145–7. doi:10.1203/00006450-199804001-00861
75. Moolenaar CE, Muller EJ, Schol DJ, Figdor CG, Bock E, Bitter-Suermann D, et al. Expression of neural cell adhesion molecule-related sialoglycoprotein in small cell lung cancer and neuroblastoma cell lines H69 and CHP-212. Cancer Res (1990) 50:1102–6.
76. Scheidegger EP, Lackie PM, Papay J, Roth J. In vitro and in vivo growth of clonal sublines of human small cell lung carcinoma is modulated by polysialic acid of the neural cell adhesion molecule. Lab Invest (1994) 70:95–106.
77. Figarella-Branger D, Dubois C, Chauvin P, Devictor B, Gentet JC, Rougon G. Correlation between polysialic-neural cell adhesion molecule levels in CSF and medulloblastoma outcomes. J Clin Oncol (1996) 14:2066–72.
78. Glüer S, Schelp C, Madry N, von Schweinitz D, Eckhardt M, Gerardy-Schahn R. Serum polysialylated neural cell adhesion molecule in childhood neuroblastoma. Br J Cancer (1998) 78:106–10. doi:10.1038/bjc.1998.450
79. Glüer S, Schelp C, Gerardy-Schahn R, von Schweinitz D. Polysialylated neural cell adhesion molecule as a marker for differential diagnosis in pediatric tumors. J Pediatr Surg (1998) 33:1516–20. doi:10.1016/S0022-3468(98)90488-2
80. Daniel L, Durbec P, Gautherot E, Rouvier E, Rougon G, Figarella-Branger D. A nude mice model of human rhabdomyosarcoma lung metastases for evaluating the role of polysialic acids in the metastatic process. Oncogene (2001) 20:997–1004. doi:10.1038/sj.onc.1204176
81. Amoureux MC, Coulibaly B, Chinot O, Loundou A, Metellus P, Rougon G, et al. Polysialic acid neural cell adhesion molecule (PSA-NCAM) is an adverse prognosis factor in glioblastoma, and regulates olig2 expression in glioma cell lines. BMC Cancer (2010) 10:91. doi:10.1186/1471-2407-10-91
82. Seidenfaden R, Krauter A, Schertzinger F, Gerardy-Schahn R, Hildebrandt H. Polysialic acid directs tumor cell growth by controlling heterophilic neural cell adhesion molecule interactions. Mol Cell Biol (2003) 23:5908–18. doi:10.1128/MCB.23.16.5908-5918.2003
83. Hildebrandt H, Becker C, Glüer S, Rösner H, Gerardy-Schahn R, Rahmann H. Polysialic acid on the neural cell adhesion molecule correlates with expression of polysialyltransferases and promotes neuroblastoma cell growth. Cancer Res (1998) 58:779–84.
84. Eggers K, Werneburg S, Schertzinger A, Abeln M, Schiff M, Scharenberg MA, et al. Polysialic acid controls NCAM signals at cell-cell contacts to regulate focal adhesion independent from FGF receptor activity. J Cell Sci (2011) 124:3279–91. doi:10.1242/jcs.084863
85. Valentiner U, Mühlenhoff M, Lehmann U, Hildebrandt H, Schumacher U. Expression of the neural cell adhesion molecule and polysialic acid in human neuroblastoma cell lines. Int J Oncol (2011) 39:417–24. doi:10.3892/ijo.2011.1038
86. Glüer S, Zense M, Radtke E, von Schweinitz D. Polysialylated neural cell adhesion molecule in childhood ganglioneuroma and neuroblastoma of different histological grade and clinical stage. Langenbecks Arch Surg (1998) 383:340–4. doi:10.1007/s004230050145
87. Korja M, Jokilammi A, Salmi TT, Kalimo H, Pelliniemi TT, Isola J, et al. Absence of polysialylated NCAM is an unfavorable prognostic phenotype for advanced stage neuroblastoma. BMC Cancer (2009) 9:57. doi:10.1186/1471-2407-9-57
88. Angata K, Nakayama J, Fredette B, Chong K, Ranscht B, Fukuda M. Human STX polysialyltransferase forms the embryonic form of the neural cell adhesion molecule. Tissue-specific expression, neurite outgrowth, and chromosomal localization in comparison with another polysialyltransferase, PST. J Biol Chem (1997) 272:7182–90. doi:10.1074/jbc.272.11.7182
89. Thompson MG, Foley DA, Swartzentruber KG, Colley KJ. Sequences at the interface of the fifth immunoglobulin domain and first fibronectin type III repeat of the neural cell adhesion molecule are critical for its polysialylation. J Biol Chem (2011) 286:4525–34. doi:10.1074/jbc.M110.200386
90. Thompson MG, Foley DA, Colley KJ. The polysialyltransferases interact with sequences in two domains of the neural cell adhesion molecule to allow its polysialylation. J Biol Chem (2013) 288:7282–93. doi:10.1074/jbc.M112.438374
91. Kojima N, Tachida Y, Tsuji S. Two polysialic acid synthases, mouse ST8Sia II and IV, synthesize different degrees of polysialic acids on different substrate glycoproteins in mouse neuroblastoma Neuro2a cells. J Biochem (1997) 122:1265–73. doi:10.1093/oxfordjournals.jbchem.a021893
92. Scheidegger EP, Sternberg LR, Roth J, Lowe JB. A human STX cDNA confers polysialic acid expression in mammalian cells. J Biol Chem (1995) 270:22685–8. doi:10.1074/jbc.270.39.22685
93. Cheung IY, Vickers A, Cheung NK. Sialyltransferase STX (ST8SiaII): a novel molecular marker of metastatic neuroblastoma. Int J Cancer (2006) 119:152–6. doi:10.1002/ijc.21789
94. Seidenfaden R, Gerardy-Schahn R, Hildebrandt H. Control of NCAM polysialylation by the differential expression of polysialyltransferases ST8SiaII and ST8SiaIV. Eur J Cell Biol (2000) 79:680–8. doi:10.1078/0171-9335-00093
95. Falconer RA, Errington RJ, Shnyder SD, Smith PJ, Patterson LH. Polysialyltransferase: a new target in metastatic cancer. Curr Cancer Drug Targets (2012) 12:925–39. doi:10.2174/156800912803251225
96. Al-Saraireh YM, Sutherland M, Springett BR, Freiberger F, Ribeiro Morais G, Loadman PM, et al. Pharmacological inhibition of polysialyltransferase ST8SiaII modulates tumour cell migration. PLoS One (2013) 8:e73366. doi:10.1371/journal.pone.0073366
97. Seifert A, Glanz D, Glaubitz N, Horstkorte R, Bork K. Polysialylation of the neural cell adhesion molecule: interfering with polysialylation and migration in neuroblastoma cells. Arch Biochem Biophys (2012) 524:56–63. doi:10.1016/j.abb.2012.04.011
98. Rabinovich GA, Croci DO. Regulatory circuits mediated by lectin-glycan interactions in autoimmunity and cancer. Immunity (2012) 36:322–35. doi:10.1016/j.immuni.2012.03.004
99. Yang RY, Rabinovich G, Liu FT. Galectins: structure, function and therapeutic potential. Expert Rev Mol Med (2008) 10:e17. doi:10.1017/S1462399408000719
100. Liu FT, Rabinovich GA. Galectins as modulators of tumour progression. Nat Rev Cancer (2005) 5:29–41. doi:10.1038/nrc1527
101. Ito K, Stannard K, Gabutero E, Clark AM, Neo SY, Onturk S, et al. Galectin-1 as a potent target for cancer therapy: role in the tumor microenvironment. Cancer Metastasis Rev (2012) 31:763–78. doi:10.1007/s10555-012-9388-2
102. Croci DO, Cerliani JP, Dalotto-Moreno T, Méndez-Huergo SP, Mascanfroni ID, Dergan-Dylon S, et al. Glycosylation-dependent lectin-receptor interactions preserve angiogenesis in anti-VEGF refractory tumors. Cell (2014) 156:744–58. doi:10.1016/j.cell.2014.01.043
103. Juszczynski P, Ouyang J, Monti S, Rodig SJ, Takeyama K, Abramson J, et al. The AP1-dependent secretion of galectin-1 by Reed Sternberg cells fosters immune privilege in classical Hodgkin lymphoma. Proc Natl Acad Sci U S A (2007) 104:13134–9. doi:10.1073/pnas.0706017104
104. Banh A, Zhang J, Cao H, Bouley DM, Kwok S, Kong C, et al. Tumor galectin-1 mediates tumor growth and metastasis through regulation of T-cell apoptosis. Cancer Res (2011) 71:4423–31. doi:10.1158/0008-5472.CAN-10-4157
105. Kuo PL, Hung JY, Huang SK, Chou SH, Cheng DE, Jong YJ, et al. Lung cancer-derived galectin-1 mediates dendritic cell anergy through inhibitor of DNA binding 3/IL-10 signaling pathway. J Immunol (2011) 186:1521–30. doi:10.4049/jimmunol.1002940
106. Cimmino F, Schulte JH, Zollo M, Koster J, Versteeg R, Iolascon A, et al. Galectin-1 is a major effector of TrkB-mediated neuroblastoma aggressiveness. Oncogene (2009) 28:2015–23. doi:10.1038/onc.2009.70
107. Soldati R, Berger E, Zenclussen AC, Jorch G, Lode HN, Salatino M, et al. Neuroblastoma triggers an immunoevasive program involving galectin-1-dependent modulation of T cell and dendritic cell compartments. Int J Cancer (2012) 131:1131–41. doi:10.1002/ijc.26498
108. Rubinstein N, Alvarez M, Zwirner NW, Toscano MA, Ilarregui JM, Bravo A, et al. Targeted inhibition of galectin-1 gene expression in tumor cells results in heightened T cell-mediated rejection; a potential mechanism of tumor immune privilege. Cancer Cell (2004) 5:241–51. doi:10.1016/S1535-6108(04)00024-8
109. Dalotto-Moreno T, Croci DO, Cerliani JP, Martinez-Allo VC, Dergan-Dylon S, Méndez-Huergo SP, et al. Targeting galectin-1 overcomes breast cancer-associated immunosuppression and prevents metastatic disease. Cancer Res (2013) 73:1107–17. doi:10.1158/0008-5472.CAN-12-2418
110. Ambros PF, Mehes G, Ambros IM, Ladenstein R. Disseminated tumor cells in the bone marrow – chances and consequences of microscopical detection methods. Cancer Lett (2003) 197:29–34. doi:10.1016/S0304-3835(03)00078-8
111. Hoon DS, Kuo CT, Wen S, Wang H, Metelitsa L, Reynolds CP, et al. Ganglioside GM2/GD2 synthetase mRNA is a marker for detection of infrequent neuroblastoma cells in bone marrow. Am J Pathol (2001) 159:493–500. doi:10.1016/S0002-9440(10)61721-X
112. Meany DL, Chan DW. Aberrant glycosylation associated with enzymes as cancer biomarkers. Clin Proteomics (2011) 8:7. doi:10.1186/1559-0275-8-7
113. Cheung NK, Von Hoff DD, Strandjord SE, Coccia PF. Detection of neuroblastoma cells in bone marrow using GD2 specific monoclonal antibodies. J Clin Oncol (1986) 4:363–9.
114. Lo Piccolo MS, Cheung NKV, Cheung IY. GD2 synthase: a new molecular marker for detecting neuroblastoma. Cancer (2001) 92:924–31. doi:10.1002/1097-0142(20010815)92:4<924::AID-CNCR1402>3.0.CO;2-O
115. Cheung IY, Lo Piccolo MS, Kushner BH, Cheung NK. Early molecular response of marrow disease to biologic therapy is highly prognostic in neuroblastoma. J Clin Oncol (2003) 21:3853–8. doi:10.1200/JCO.2003.11.077
116. Träger C, Vernby A, Kullman A, Ora I, Kogner P, Kågedal B. mRNAs of tyrosine hydroxylase and dopa decarboxylase but not of GD2 synthase are specific for neuroblastoma minimal disease and predicts outcome for children with high-risk disease when measured at diagnosis. Int J Cancer (2008) 123:2849–55. doi:10.1002/ijc.23846
117. Beiske K, Burchill SA, Cheung IY, Hiyama E, Seeger RC, Cohn SL, et al. Consensus criteria for sensitive detection of minimal neuroblastoma cells in bone marrow, blood and stem cell preparations by immunocytology and QRT-PCR: recommendations by the International Neuroblastoma Risk Group Task Force. Br J Cancer (2009) 100:1627–37. doi:10.1038/sj.bjc.6605029
118. Dennis JW, Laferte S, Waghorne C, Breitman ML, Kerbel RS. Beta 1–6 branching of Asn-linked oligosaccharides is directly associated with metastasis. Science (1987) 236:582–5. doi:10.1126/science.2953071
119. Fernandes B, Sagman U, Auger M, Demetrio M, Dennis JW. Beta 1–6 branched oligosaccharides as a marker of tumor progression in human breast and colon neoplasia. Cancer Res (1991) 51:718–23.
120. Seelentag WK, Li WP, Schmitz SF, Metzger U, Aeberhard P, Heitz PU, et al. Prognostic value of beta1,6-branched oligosaccharides in human colorectal carcinoma. Cancer Res (1998) 58:5559–64.
121. Murata K, Miyoshi E, Kameyama M, Ishikawa O, Kabuto T, Sasaki Y, et al. Expression of N-acetylglucosaminyltransferase V in colorectal cancer correlates with metastasis and poor prognosis. Clin Cancer Res (2000) 6:1772–7.
122. Dosaka-Akita H, Miyoshi E, Suzuki O, Itoh T, Katoh H, Taniguchi N. Expression of N-acetylglucosaminyltransferase V is associated with prognosis and histology in nonsmall cell lung cancers. Clin Cancer Res (2004) 10:1773–9. doi:10.1158/1078-0432.CCR-1047-3
123. Inamori K, Gu J, Ohira M, Kawasaki A, Nakamura Y, Nakagawa T, et al. High expression of N-acetylglucosaminyltransferase V in favorable neuroblastomas: involvement of its effect on apoptosis. FEBS Lett (2006) 580:627–32. doi:10.1016/j.febslet.2005.12.089
124. Cheung IY, Feng Y, Gerald W, Cheung NK. Exploiting gene expression profiling to identify novel minimal residual disease markers of neuroblastoma. Clin Cancer Res (2008) 14:7020–7. doi:10.1158/1078-0432.CCR-08-0541
125. Takita J, Ishii M, Tsutsumi S, Tanaka Y, Kato K, Toyoda Y, et al. Gene expression profiling and identification of novel prognostic marker genes in neuroblastoma. Genes Chromosomes Cancer (2004) 40:120–32. doi:10.1002/gcc.20021
126. Fischer M, Oberthuer A, Brors B, Kahlert Y, Skowron M, Voth H, et al. Differential expression of neuronal genes defines subtypes of disseminated neuroblastoma with favorable and unfavorable outcome. Clin Cancer Res (2006) 12:5118–28. doi:10.1158/1078-0432.CCR-06-0985
127. Ferrandis E, Da Silva J, Riou G, Bénard J. Coactivation of the MDR1 and MYCN in human neuroblastoma during the metastatic process in the nude mouse. Cancer Res (1994) 54:2256–61.
128. Blanc E, Goldschneider D, Ferrandis E, Barrois M, Le Roux G, Leonce S, et al. MYCN enhances P-gp/MDR1 gene expression in the human metastatic neuroblastoma IGR-N-91 model. Am J Pathol (2003) 163:321–31. doi:10.1016/S0002-9440(10)63656-5
129. Zhang Y, Iwasaki H, Wang H, Kudo T, Kalka TB, Hennet T, et al. Cloning and characterization of a new human UDP-N-acetyl-alpha-D-galactosamine:polypeptide N-acetylgalactosaminyltransferase, designated pp-GalNAc-T13, that is specifically expressed in neurons and synthesizes GalNAc alpha-serine/threonine antigen. J Biol Chem (2003) 278:573–84. doi:10.1074/jbc.M203094200
130. Toba S, Tenno M, Konishi M, Mikami T, Itoh N, Kurosaka A. Brain-specific expression of a novel human UDP-GalNAc:polypeptide N-acetylgalactosaminyltransferase (GalNAc-T9). Biochim Biophys Acta (2000) 1493:264–8. doi:10.1016/S0167-4781(00)00180-9
131. Piacentini M, Piredda L, Starace DT, Annicchiarico-Petruzzelli M, Mattei M, Oliverio S, et al. Differential growth of N- and S-type human neuroblastoma cells xenografted into SCID mice. Correlation with apoptosis. J Pathol (1996) 180:415–22. doi:10.1002/(SICI)1096-9896(199612)180:4<415::AID-PATH684>3.0.CO;2-A
132. Mitoma J, Petryniak B, Hiraoka N, Yeh JC, Lowe JB, Fukuda M. Extended core 1 and core 2 branched O-glycans differentially modulate sialyl Lewis X-type L-selectin ligand activity. J Biol Chem (2003) 278:9953–61. doi:10.1074/jbc.M212756200
133. Yago T, Fu J, McDaniel JM, Miner JJ, McEver RP, Xia L. Core 1-derived O-glycans are essential E-selectin ligands on neutrophils. Proc Natl Acad Sci U S A (2010) 107:9204–9. doi:10.1073/pnas.1003110107
134. Köhler S, Ullrich S, Richter U, Schumacher U. E-/P-selectins and colon carcinoma metastasis: first in vivo evidence for their crucial role in a clinically relevant model of spontaneous metastasis formation in the lung. Br J Cancer (2010) 102:602–9. doi:10.1038/sj.bjc.6605492
135. Gakhar G, Navarro VN, Jurish M, Lee GY, Tagawa ST, Akhtar NH, et al. Circulating tumor cells from prostate cancer patients interact with E-selectin under physiologic blood flow. PLoS One (2013) 8:e85143. doi:10.1371/journal.pone.0085143
136. Sato T, Gotoh M, Kiyohara K, Kameyama A, Kubota T, Kikuchi N, et al. Molecular cloning and characterization of a novel human β1,4-N-acetylgalactosaminyltransferase, β4GalNAc-T3, responsible for the synthesis of N,N′-diacetyllactosediamine, galNAc β1-4GlcNAc. J Biol Chem (2003) 278:47534–44. doi:10.1074/jbc.M308857200
137. Kawar ZS, Haslam SM, Morris HR, Dell A, Cummings RD. Novel poly-GalNAcbeta1-4GlcNAc (LacdiNAc) and fucosylated poly-LacdiNAc N-glycans from mammalian cells expressing beta1,4-N-acetylgalactosaminyltransferase and alpha1,3-fucosyltransferase. J Biol Chem (2005) 280:12810–919. doi:10.1074/jbc.M414273200
138. Fiete D, Beranek M, Baenziger JU. Peptide-specific transfer of N-acetylgalactosamine to O-linked glycans by the glycosyltransferases β1,4-N-acetylgalactosaminyl transferase 3 (β4GalNAc-T3) and β4GalNAc-T4. J Biol Chem (2012) 287:29204–12. doi:10.1074/jbc.M112.371880
139. Ikehara Y, Sato T, Niwa T, Nakamura S, Gotoh M, Ikehara SK, et al. Apical Golgi localization of N,N’-diacetyllactosediamine synthase, beta4GalNAc-T3, is responsible for LacdiNAc expression on gastric mucosa. Glycobiology (2006) 16:777–85. doi:10.1093/glycob/cwl005
140. Huang J, Liang JT, Huang HC, Shen TL, Chen HY, Lin NY, et al. Beta1,4-N-acetylgalactosaminyltransferase III enhances malignant phenotypes of colon cancer cells. Mol Cancer Res (2007) 5:543–52. doi:10.1158/1541-7786.MCR-06-0431
141. Chen CH, Wang SH, Liu CH, Wu YL, Wang WJ, Huang J, et al. β-1,4-galactosyltransferase III suppresses β1 integrin mediated invasive phenotypes and negatively correlates with metastasis in colorectal cancer. Carcinogenesis (2014). doi:10.1093/carcin/bgu007
142. Hsu WM, Che MI, Liao YF, Chang HH, Chen CH, Huang YM, et al. B4GALNT3 expression predicts a favorable prognosis and suppresses cell migration and invasion via β1 integrin signaling in neuroblastoma. Am J Pathol (2011) 179:1394–404. doi:10.1016/j.ajpath.2011.05.025
143. Chang HH, Chen CH, Chou CH, Liao YF, Huang MJ, Chen YH, et al. β-1,4-galactosyltransferase III enhances invasive phenotypes via β1-integrin and predicts poor prognosis in neuroblastoma. Clin Cancer Res (2013) 19:1705–16. doi:10.1158/1078-0432.CCR-12-2367
144. De Bernardi B, Gerrard M, Boni L, Rubie H, Cañete A, Di Cataldo A, et al. Excellent outcome with reduced treatment for infants with disseminated neuroblastoma without MYCN gene amplification. J Clin Oncol (2009) 27:1034–40. doi:10.1200/JCO.2008.17.5877
145. Modak S, Cheung NK. Neuroblastoma: therapeutic strategies for a clinical enigma. Cancer Treat Rev (2010) 36:307–17. doi:10.1016/j.ctrv.2010.02.006
146. Barone G, Anderson J, Pearson AD, Petrie K, Chesler L. New strategies in neuroblastoma: therapeutic targeting of MYCN and ALK. Clin Cancer Res (2013) 19:5814–21. doi:10.1158/1078-0432.CCR-13-0680
147. Ott PA, Hodi FS, Robert C. CTLA-4 and PD-1/PD-L1 blockade: new immunotherapeutic modalities with durable clinical benefit in melanoma patients. Clin Cancer Res (2013) 19:5300–9. doi:10.1158/1078-0432.CCR-13-0143
148. Baxevanis CN, Papamichail M, Perez SA. Therapeutic cancer vaccines: a long and winding road to success. Expert Rev Vaccines (2014) 13:131–44. doi:10.1586/14760584.2014.852961
149. Darcy PK, Neeson P, Yong CS, Kershaw MH. Manipulating immune cells for adoptive immunotherapy of cancer. Curr Opin Immunol (2014) 27C:46–52. doi:10.1016/j.coi.2014.01.008
150. Kyi C, Postow MA. Checkpoint blocking antibodies in cancer immunotherapy. FEBS Lett (2014) 588:368–76. doi:10.1016/j.febslet.2013.10.015
151. Zigler M, Shir A, Levitzki A. Targeted cancer immunotherapy. Curr Opin Pharmacol (2013) 13:504–10. doi:10.1016/j.coph.2013.04.003
152. Freire T, Osinaga E. The sweet side of tumor immunotherapy. Immunotherapy (2012) 4:719–34. doi:10.2217/imt.12.58
153. Cheung NK, Dyer MA. Neuroblastoma: developmental biology, cancer genomics and immunotherapy. Nat Rev Cancer (2013) 13:397–411. doi:10.1038/nrc3526
154. Cheever MA, Allison JP, Ferris AS, Finn OJ, Hastings BM, Hecht TT, et al. The prioritization of cancer antigens: a national cancer institute pilot project for the acceleration of translational research. Clin Cancer Res (2009) 15:5323–37. doi:10.1158/1078-0432.CCR-09-0737
155. Matthay KK, George RE, Yu AL. Promising therapeutic targets in neuroblastoma. Clin Cancer Res (2012) 18:2740–53. doi:10.1158/1078-0432.CCR-11-1939
156. Parsons K, Bernhardt B, Strickland B. Targeted immunotherapy for high-risk neuroblastoma – the role of monoclonal antibodies. Ann Pharmacother (2013) 47:210–8. doi:10.1345/aph.1R353
157. Ahmed M, Cheung NK. Engineering anti-GD2 monoclonal antibodies for cancer immunotherapy. FEBS Lett (2014) 588:288–97. doi:10.1016/j.febslet.2013.11.030
158. Cheung NK, Saarinen UM, Neely JE, Landmeier B, Donovan D, Coccia PF. Monoclonal antibodies to a glycolipid antigen on human neuroblastoma cells. Cancer Res (1985) 45:2642–9.
159. Cheung NK, Lazarus H, Miraldi FD, Abramowsky CR, Kallick S, Saarinen UM, et al. Ganglioside GD2 specific monoclonal antibody 3F8: a phase I study in patients with neuroblastoma and malignant melanoma. J Clin Oncol (1987) 5:1430–40.
160. Kushner BH, Cheung NK. GM-CSF enhances 3F8 monoclonal antibody-dependent cellular cytotoxicity against human melanoma and neuroblastoma. Blood (1989) 73:1936–41.
161. Kushner BH, Cheung NK. Clinically effective monoclonal antibody 3F8 mediates nonoxidative lysis of human neuroectodermal tumor cells by polymorphonuclear leukocytes. Cancer Res (1991) 51:4865–70.
162. Kushner BH, Cheung NK. Absolute requirement of CD11/CD18 adhesion molecules, FcRII and the phosphatidylinositol-linked FcRIII for monoclonal antibody-mediated neutrophil antihuman tumor cytotoxicity. Blood (1992) 79:1484–90.
163. Cheung NK, Walter EI, Smith-Mensah WH, Ratnoff WD, Tykocinski ML, Medof ME. Decay-accelerating factor protects human tumor cells from complement-mediated cytotoxicity in vitro. J Clin Invest (1988) 81:1122–8. doi:10.1172/JCI113426
164. Chen S, Caragine T, Cheung NK, Tomlinson S. CD59 expressed on a tumor cell surface modulates decay-accelerating factor expression and enhances tumor growth in a rat model of human neuroblastoma. Cancer Res (2000) 60:3013–8.
165. Hank JA, Robinson RR, Surfus J, Mueller BM, Reisfeld RA, Cheung NK, et al. Augmentation of antibody dependent cell mediated cytotoxicity following in vivo therapy with recombinant interleukin 2. Cancer Res (1990) 50:5234–9.
166. Barker E, Reisfeld RA. A mechanism for neutrophil-mediated lysis of human neuroblastoma cells. Cancer Res (1993) 53:362–7.
167. Cheung NK, Cheung IY, Kushner BH, Ostrovnaya I, Chamberlain E, Kramer K, et al. Murine anti-GD2 monoclonal antibody 3F8 combined with granulocyte-macrophage colony-stimulating factor and 13-cis-retinoic acid in high-risk patients with stage 4 neuroblastoma in first remission. J Clin Oncol (2012) 30:3264–70. doi:10.1200/JCO.2011.41.3807
168. Cheung NK, Guo H, Hu J, Tassev DV, Cheung IY. Humanizing murine IgG3 anti-GD2 antibody m3F8 substantially improves antibody dependent cell-mediated cytotoxicity while retaining targeting in vivo. Oncoimmunology (2012) 1:477–86. doi:10.4161/onci.19864
169. Mujoo K, Kipps TJ, Yang HM, Cheresh DA, Wargalla U, Sander DJ, et al. Functional properties and effect on growth suppression of human neuroblastoma tumors by isotype switch variants of monoclonal antiganglioside GD2 antibody 14.18. Cancer Res (1989) 49:2857–61.
170. Gillies SD, Lo KM, Wesolowski J. High-level expression of chimeric antibodies using adapted cDNA variable region cassettes. J Immunol Methods (1989) 125:191–202. doi:10.1016/0022-1759(89)90093-8
171. Yu AL, Gilman AL, Ozkaynak MF, London WB, Kreissman SG, Chen HX, et al. Anti-GD2 antibody with GM-CSF, interleukin-2, and isotretinoin for neuroblastoma. N Engl J Med (2010) 363:1324–34. doi:10.1056/NEJMoa0911123
172. Yang RK, Kalogriopoulos NA, Rakhmilevich AL, Ranheim EA, Seo S, Kim K, et al. Intratumoral hu14.18-IL-2 (IC) induces local and systemic antitumor effects that involve both activated T and NK cells as well as enhanced IC retention. J Immunol (2012) 189:2656–64. doi:10.4049/jimmunol.1200934
173. Tur MK, Sasse S, Stöcker M, Djabelkhir K, Huhn M, Matthey B, et al. An anti-GD2 single chain Fv selected by phage display and fused to Pseudomonas exotoxin A develops specific cytotoxic activity against neuroblastoma derived cell lines. Int J Mol Med (2001) 8:579–84. doi:10.3892/ijmm.8.5.579
174. Lode HN, Reisfeld RA, Handgretinger R, Nicolaou KC, Gaedicke G, Wrasidlo W. Targeted therapy with a novel enediyene antibiotic calicheamicin theta(I)1 effectively suppresses growth and dissemination of liver metastases in a syngeneic model of murine neuroblastoma. Cancer Res (1998) 58:2925–8.
175. Vavere AL, Butch ER, Dearling JL, Packard AB, Navid F, Shulkin BL, et al. 64Cu-p-NH2-Bn-DOTA-hu14.18K322A, a PET radiotracer targeting neuroblastoma and melanoma. J Nucl Med (2012) 53:1772–8. doi:10.2967/jnumed.112.104208
176. Tivnan A, Orr WS, Gubala V, Nooney R, Williams DE, McDonagh C, et al. Inhibition of neuroblastoma tumor growth by targeted delivery of microRNA-34a using anti-disialoganglioside GD2 coated nanoparticles. PLoS One (2012) 7:e38129. doi:10.1371/journal.pone.0038129
177. Sadelain M, Riviere I, Brentjens R. Targeting tumours with genetically enhanced T lymphocytes. Nat Rev Cancer (2003) 3:35–45. doi:10.1038/nrc971
178. Cheadle EJ, Gornall H, Baldan V, Hanson V, Hawkins RE, Gilham DE. CAR T cells: driving the road from the laboratory to the clinic. Immunol Rev (2014) 257:91–106. doi:10.1111/imr.12126
179. Dotti G, Gottschalk S, Savoldo B, Brenner MK. Design and development of therapies using chimeric antigen receptor-expressing T cells. Immunol Rev (2014) 257:107–26. doi:10.1111/imr.12131
180. Rossig C, Bollard C, Nuchtern J, Merchant D, Brenner M. Targeting of GD2-positive tumor cells by human T lymphocytes engineered to express chimeric T-cell receptor genes. Int J Cancer (2001) 94:228–36. doi:10.1002/ijc.1457
181. Yun C, Nolan K, Beecham E, Reisfeld R, Junghans R. Targeting of T lymphocytes to melanoma cells through chimeric anti-GD3 immunoglobulin T-cell receptors. Neoplasia (2000) 2:449–59. doi:10.1038/sj.neo.7900108
182. Yvon E, Del Vecchio M, Savoldo B, Hoyos V, Dutour A, Anichini A, et al. Immunotherapy of metastatic melanoma using genetically engineered GD2-specific T cells. Clin Cancer Res (2009) 15:5852–60. doi:10.1158/1078-0432.CCR-08-3163
183. Pule MA, Savoldo B, Myers GD, Rossig C, Russell HV, Dotti G, et al. Virus-specific T cells engineered to coexpress tumor-specific receptors: persistence and antitumor activity in individuals with neuroblastoma. Nat Med (2008) 14:1264–70. doi:10.1038/nm.1882
Keywords: neuroblastoma, glycosylation, gangliosides, polysialic acid, galectin-1, glycosyltransferases, immunotherapy
Citation: Berois N and Osinaga E (2014) Glycobiology of neuroblastoma: impact on tumor behavior, prognosis, and therapeutic strategies. Front. Oncol. 4:114. doi: 10.3389/fonc.2014.00114
Received: 18 March 2014; Accepted: 02 May 2014;
Published online: 23 May 2014.
Edited by:
Adriane Regina Todeschini, Universidade Federal do Rio de Janeiro, BrazilReviewed by:
Igor C. Almeida, University of Texas at El Paso, USACopyright: © 2014 Berois and Osinaga. This is an open-access article distributed under the terms of the Creative Commons Attribution License (CC BY). The use, distribution or reproduction in other forums is permitted, provided the original author(s) or licensor are credited and that the original publication in this journal is cited, in accordance with accepted academic practice. No use, distribution or reproduction is permitted which does not comply with these terms.
*Correspondence: Eduardo Osinaga, Laboratorio de Glicobiología e Inmunología Tumoral, Institut Pasteur de Montevideo, Mataojo 2020, Montevideo CP 11400, Uruguay e-mail:ZW9zaW5hZ2FAZm1lZC5lZHUudXk=
Disclaimer: All claims expressed in this article are solely those of the authors and do not necessarily represent those of their affiliated organizations, or those of the publisher, the editors and the reviewers. Any product that may be evaluated in this article or claim that may be made by its manufacturer is not guaranteed or endorsed by the publisher.
Research integrity at Frontiers
Learn more about the work of our research integrity team to safeguard the quality of each article we publish.