- 1 Division of Pathology, Norwegian Radium Hospital, Oslo University Hospital, Oslo, Norway
- 2 Faculty of Medicine, University of Oslo, Oslo, Norway
- 3 Section of Gynecologic Oncology, Division of Gynecology and Obstetrics, Norwegian Radium Hospital, Oslo University Hospital, Oslo, Norway
- 4 Institute of Drug Research, School of Pharmacy, Faculty of Medicine, The Hebrew University of Jerusalem, Jerusalem, Israel
- 5 David R. Bloom Center for Pharmacy and the Brettler Center for Pharmacology, The Hebrew University of Jerusalem, Jerusalem, Israel
Ovarian cancer is the most lethal gynecologic malignancy, with the majority of patients dying within 5 years of diagnosis. This poor survival of patients diagnosed with this malignancy is attributed to diagnosis at advanced stage, when the tumor has metastasized, and to chemotherapy resistance, either primary or developing along tumor progression. However, ovarian carcinomas, constituting the vast majority of ovarian cancers, additionally have unique biology, one aspect of which is the ability to co-express epithelial and mesenchymal determinants. epithelial–mesenchymal transition (EMT), a physiological process by which mesenchymal cells are formed and migrate to target organs during embryogenesis, is involved in cancer cell invasion and metastasis. However, these changes do not fully occur in ovarian carcinoma, and are even reversed in tumor cells present in malignant peritoneal and pleural effusions. This review summarizes current knowledge in this area, including the characteristics of EMT related to adhesion, transcriptional regulation and chemoresistance, and their clinical relevance, as well as the recently observed regulation of EMT by microRNA.
Ovarian Carcinoma
Ovarian cancer, consisting predominantly of carcinomas, is the most lethal gynecologic cancer and currently ranks fifth in causing cancer-related deaths among women (Siegel et al., 2011). Ovarian carcinoma is treated by surgery and chemotherapy, the latter with combination of carboplatin and paclitaxel. Moderate improvement in the 5-year survival rate of ovarian carcinoma patients has been observed in recent years, due to more aggressive debulking surgery and improved chemotherapy regimens. However, the majority of patients still die of their disease, this being mainly attributed to frequent presentation at advanced stage (FIGO stage III–IV) and to primary or acquired drug resistance (Agarwal and Kaye, 2003; Cannistra, 2004; Hennessy et al., 2009).
Ovarian carcinoma and the closely related primary peritoneal carcinoma and fallopian tube carcinoma metastasize predominantly within the serosal cavities, forming multiple solid nodules on the surfaces of the abdominal cavity or organs therein, including the omentum, intestines, genital organs, spleen, and urinary bladder, as well as accumulation of peritoneal effusion fluid (ascites). The latter occurs through the combined effect of lymphatic obstruction by metastatic cancer cells, increased production of peritoneal fluid by mesothelial cells lining the peritoneal cavity and increased vascular permeability (Hirabayashi and Graham, 1970; Feldman et al., 1972; Nagy et al., 1995). The pleural space is frequently involved, either at diagnosis or at a later stage, this anatomic site being the most frequent one defining stage IV disease (Curtin et al., 1997; Bonnefoi et al., 1999; Akahira et al., 2001). Lymph node metastases and dissemination to distant organs occur, but are distinctly less common (Tsuruchi et al., 1993; Cormio et al., 1995; Sakai et al., 1997).
The common histological types of ovarian carcinoma are serous, endometrioid, clear cell, and mucinous carcinoma, as well as carcinomas of mixed type. These distinct morphological entities are growingly perceived to be tumors of different etiology, with unique genetic and phenotypic characteristics and different clinical behavior, including response to chemotherapy (Köbel et al., 2008).
Epithelial–Mesenchymal Transition
Epithelial cells are adherent cells that maintain cell–cell contact through adherens junctions, tight junctions, gap junctions, and desmosomes. During embryogenesis, epithelial cells assume mesenchymal characteristics in a process that facilitates migration through the extracellular environment (ECM) and settlement in areas of new organ formation. Several phases of this process, termed primary, secondary, and tertiary epithelial–mesenchymal transition (EMT), are crucial for formation of the different germ cell layers, structures originating from the neural tube, tubular organs (e.g., the alimentary system), and parenchymal organs developing through budding, such as the liver and pancreas. Wound healing represents another form of physiological EMT, whereas tissue fibrosis and cancer progression represent examples of pathological EMT (Thiery, 2002; Acloque et al., 2009; Thiery et al., 2009). In the context of cancer, it is hypothesized that carcinoma cells lose their epithelial characteristics and acquire certain mesenchymal properties that promote ECM invasion and distant metastasis in an EMT-like process.
Signals inducing EMT in embryogenesis and/or cancer progression are multiple and diverse and include growth factors, such as epidermal growth factor (EGF) and fibroblast growth factor (FGF), platelet-derived growth factor (PDGF), insulin-like growth factor (IGF), vascular endothelial growth factor (VEGF), hepatocyte growth factor (HGF), and transforming growth factor-β (TGF-β); stem cell factor (SCF); bone morphogenetic proteins (BMP); the Wnt signaling pathway; integrins; Notch transcription factors; prostaglandin E2 (PGE2) and cyclooxygenase-2 (COX-2); parathyroid hormone (PTH); UV; nicotine; bile acids and estrogens (Thiery et al., 2009).
The morphological alterations that cells undergo during EMT are accompanied by changes in the expression of a large number of molecules. Epithelial markers that are downregulated during this process include E-cadherin, cytokeratins, ZO-1, claudins, occludin, laminin-1, entactin, MUC1, and the microRNA 200 (miR-200) family. Molecules that are overexpressed in this process include the transcription factors Snail, Slug, Twist, Zeb1 and Zeb2/SIP1, E47, KLF8, E2.2, Goosecoid, LEF-1 and FoxC2, as well as E-cadherin, vimentin, fibronectin, miR10b, and miR21 (Kalluri and Weinberg, 2009; Thiery et al., 2009; Zeisberg and Neilson, 2009). While many of these molecules are altered in both embryonic and cancer-related EMT, tumor cells differ from embryonic cells by having lost specific target recognition, as well as by their ability to activate autocrine loops of growth signals, evade apoptosis, and elicit angiogenesis. Recent data support the role of hypoxia, inflammation, and oxidative stress, mediated through signals from the tumor microenvironment, i.e., leukocytes and myofibroblasts, as inducers of EMT (López-Novoa and Nieto, 2009). TGF-β1 appears to be a key mediator of EMT (López-Novoa and Nieto, 2009; Mamuya and Duncan, 2012), in part via its interaction with αV integrins (Mamuya and Duncan, 2012).
The following sections will discuss the expression, biological role, and clinical relevance of some of the molecules that are altered in the process of EMT in ovarian carcinoma.
Epithelial Markers Targeted by EMT
E-Cadherin
E-cadherin, the prototype member of the classical cadherin family, maintains cell polarity and normal structure in epithelial cells. E-cadherin-mediated intercellular adhesion is lost during malignant transformation and tumor progression in the majority of carcinomas (Pecina-Slaus, 2003; Cavallaro and Christofori, 2004). In classical EMT, expression of N-cadherin concomitantly occurs. However, cadherin expression patterns in the normal and neoplastic ovary are complex and do not fully follow this model. The ovarian surface epithelium (OSE), believed to be the origin of at least some ovarian carcinomas, shares histogenesis and anatomic continuity with the peritoneal mesothelium. Normal OSE with flat morphology expresses N-cadherin, but is E-cadherin negative, and the latter is expressed only in clefts and inclusion cysts, postulated to be ovarian carcinoma precursors (Hudson et al., 2008). Furthermore, while E-cadherin expression is reduced in some primary ovarian carcinomas, this protein is re-expressed in ovarian carcinoma effusions, with significantly higher levels compared to patient-matched primary carcinomas (Davidson et al., 2000a), suggesting that ovarian carcinoma cells undergo incomplete EMT. Further complicating matters is the ability of ovarian carcinoma cells to co-express E-cadherin and the EMT-associated N-cadherin (Figures 1A,B; Sivertsen et al., 2006). P-cadherin is another family member expressed in ovarian carcinoma, and was postulated to be the main cadherin member involved in tumor progression based on analysis of cadherin profiles in FIGO stage I–II tumors (Patel et al., 2003).
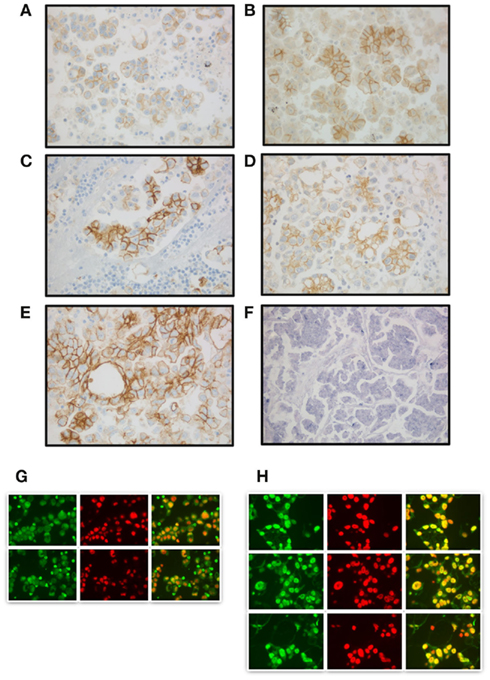
Figure 1. EMT-related molecules in ovarian serous carcinoma. (A,B) Expression of E-cadherin (A) and N-cadherin (B); (C–E) Immunostaining for claudin-1 (C), claudin-3 (D), and claudin-4 (E); (F) in situ hybridization for Snail mRNA in a primary carcinoma. NBT–BCIP as chromogen; (G,H) Snail immunofluorescence. Snail localizes to the cytoplasm in some effusions (G), whereas localization to the nucleus in seen in others, being more prominent after cells are cultured (H). Green: Snail, Red: DAPI, right column: overlay.
The clinical role of E-cadherin in ovarian carcinoma is not entirely settled, although the majority of studies suggest a prognostic role in this disease. Loss of E-cadherin protein expression was associated with poor survival in two series of 20 and 104 primary carcinomas, but these included a total of only 13 E-cadherin negative specimens (Darai et al., 1997; Faleiro-Rodrigues et al., 2004). E-cadherin protein expression in primary ovarian carcinomas and solid metastases did not differ between patients with short-term vs. long-term survival (Davidson et al., 2000b), whereas lower E-cadherin mRNA expression in ovarian carcinoma effusions correlated with poor survival (Elloul et al., 2005). In a large recent study of high-grade serous ovarian carcinomas, high expression of E-cadherin was associated with better survival, with opposite findings for N-cadherin and P-cadherin (Quattrocchi et al., 2011).
The marked changes in cadherin expression patterns in ovarian carcinoma compared to the normal ovary, as well as the anatomic site-related changes in carcinomas along tumor progression, suggest dynamic regulation at the epigenetic level, in agreement with data presented in other sections of this review. It is nevertheless questionable whether cadherins which may be associated with poor survival, such as N-cadherin, are viable therapeutic candidates, as suggested by Quattrocchi et al. (2011), in view of their frequent expression in benign host cells (e.g., normal mesothelium).
Claudins
The expression and clinical significance of tight junction proteins of the claudin family in ovarian carcinoma is yet another example of the discordance between classic EMT and expression patterns in this cancer. Tight junctions, located in the apical aspect of epithelial or endothelial cells, maintain cell polarity, and regulate the paracellular transport of solutes and the diffusion of proteins and lipids. Claudins contain intracellular amino and carboxy termini, four transmembrane domains, and two extracellular loops mediating intercellular interactions between family members, the latter being the binding site for Clostridium perfringens enterotoxin in claudin-3 and -4. The carboxy terminus of most claudins contains potential serine and/or threonine phosphorylation sites and a PDZ-binding motif, to which the tight junction cytoplasmic scaffolding proteins ZO-1, -2, and -3 bind (Morita et al., 1999; Tsukita et al., 2001; González-Mariscal et al., 2003; Van Itallie and Anderson, 2006).
Claudin expression is deregulated in cancer compared to normal tissue or benign tumors in a complex and organ-dependent manner (Hewitt et al., 2006), and several members of this family, including claudin-3, -4, -7, and -10, have been reported to be more highly expressed in ovarian carcinoma compared to normal OSE (Hough et al., 2000; Hibbs et al., 2004; Lu et al., 2004; Santin et al., 2004; Bignotti et al., 2006; Tassi et al., 2008). Interestingly, in vitro analysis of 4 ovarian carcinoma cell lines of different histological type showed formation of functional cell junctions only in serous ovarian carcinoma (Zhu and Sundfeldt, 2007).
In addition, claudins are significantly overexpressed in ovarian carcinoma effusions compared to other tumors affecting the serosal cavities, particularly malignant mesothelioma, and benign reactive mesothelial cells (Davidson et al., 2006; Kleinberg et al., 2007), and claudin-4 was recently shown to effectively differentiate between carcinomas of different origin, including ovarian carcinoma, and malignant mesothelioma or reactive mesothelial cells (Lonardi et al., 2011).
Claudin expression in ovarian carcinoma is anatomic site-related, as three members of this family, claudin-1, -3, and -7, are upregulated in ovarian carcinoma effusions compared to patient-matched primary carcinomas and solid metastases. In contrast, claudin-4 is highly expressed at all anatomic sites in this cancer (Figures 1C–E; Kleinberg et al., 2008).
Claudin-4-containing exosomes were found in the plasma of 32/63 ovarian carcinoma patients compared to 1/50 healthy volunteers. At a specificity of 98%, the claudin-4 and CA125 tests had sensitivities of 51 and 71%, respectively, and the two tests were strongly correlated (Li et al., 2009a). Claudin-4 was further shown to be involved in spheroid formation in ovarian carcinoma (Boylan et al., 2011), as well as in angiogenesis (Li et al., 2009b). Claudin-7 was recently shown to promote invasion of ovarian carcinoma cells in vitro, whereas migration was inhibited (Dahiya et al., 2011).
Several reports regarding the prognostic role of claudins in ovarian carcinoma have been published in recent years. Low claudin-3 protein expression was associated with a trend for poor survival in analysis of 115 primary carcinomas (Heinzelmann-Schwarz et al., 2004). In contrast, high claudin-3 expression was associated with shorter survival in both univariate and multivariate analysis in another study (Choi et al., 2007). In the above-mentioned comparative analysis of ovarian carcinoma claudin expression at different anatomic sites (Kleinberg et al., 2008), in which a total of 463 tumors were studied, higher claudin-1, -3, and -7 expression in effusions correlated with shorter overall survival (OS) and/or progression-free survival (PFS) in univariate analysis. In Cox multivariate survival analysis of the entire cohort, claudin-7 expression was an independent predictor of poor PFS, whereas claudin-3 independently predicted poor OS for patients with post-chemotherapy effusions. Claudin-5 expression was associated with high grade, advanced stage and shorter OS and PFS in an additional study (Turunen et al., 2009).
The overexpression of claudins in ovarian carcinoma compared to normal ovaries, their role in tumor biology, their upregulation in metastatic disease, and their association with poor outcome, suggest a role as therapeutic target in this disease, an approach that has been under clinical investigation in recent years (Romani et al., 2009; Saeki et al., 2009; Suzuki et al., 2009; Yuan et al., 2009; Cocco et al., 2010; Sun et al., 2011). Furthermore, claudin silencing increases the sensitivity of ovarian carcinoma cells to chemotherapy (Gao et al., 2011; Kim et al., 2011; Yoshida et al., 2011), and intraperitoneal application of C. perfringens enterotoxin leads to eradication of CD44+ ovarian cancer stem cells (CSC) in mice in vivo (Casagrande et al., 2011), providing a molecular rationale for their evaluation in this context.
Together, these data demonstrate that epithelial markers may be related to enhanced rather than reduced tumor aggressiveness in ovarian carcinoma and underscore the difficulty in applying the molecular principles of EMT to this cancer.
Transcriptional Regulators of EMT
The Snail Family
The Snail family is a regulator of mesenchyme formation in embryogenesis that is highly conserved across different species, from C. elegans and Drosophila to vertebrates, including humans. In vertebrates, it consists of three zinc finger transcription factors, Snail1 (Snail), Snail2 (Slug), and Snail3 (Smuc). Snail family members have in recent years been shown to be major regulators of EMT, but additionally modulate genes involved in cell polarity, apoptosis, cell survival, immune regulation, and CSC biology, whether directly or indirectly. Among the genes that are negatively regulated by Snail proteins during EMT are E-cadherin, cytokeratin 18, desmoplakin, MUC1, claudin, and occludin, whereas the expression of matrix metalloproteinases (MMP), the most important family of proteases mediating invasion, metastasis, and angiogenesis, as well and that of vimentin, fibronectin, and Rho GTPases is induced by Snail (Hemavathy et al., 2000; Nieto, 2002; Peinado et al., 2007; Wu and Zhou, 2010).
The transcriptional repressor activity of Snail is modulated by its expression level and intracellular localization. As EMT in general, Snail members are regulated by a large number of proteins and signaling pathways, including growth factors (TGF-β1, EGF, VEGF, FGF, PDGF), Integrin/Integrin-related kinase (ILK) signaling, NFκB, Hedgehog, Notch, HMGA2, and the Wnt/β-catenin pathway (Nieto, 2002; Peinado et al., 2007; Wu and Zhou, 2010). p21-activated kinase (Pak1), a serine/threonine protein kinase, regulates Snail through phosphorylation at Ser246, resulting in Snail accumulation in the nucleus, with resulting increase in its transcriptional activity. Cytoplasmic Snail has a short half-life, as it is targeted for degradation by glycogen synthase kinase-3β (GSK-3β; Wu and Zhou, 2010).
In experimental models of ovarian carcinoma, Snail was shown to mediate E-cadherin downregulation induced by hypoxia (Imai et al., 2003), promote tumor growth and metastasis (Jin et al., 2010), induce EMT initiated by p70 S6 kinase and bone morphogenetic protein-4 (BMP-4; Thériault et al., 2007; Pon et al., 2008), and mediate invasion (Kurrey et al., 2005). Treatment of serous ovarian carcinoma cell lines with another member of the BMP family, BMP-2, resulted in higher Snail level, increased motility, and increased scattering of cells in spheroids (Le Page et al., 2009).
As for Snail, Slug was shown to be involved in EMT and invasion (Kurrey et al., 2005; Thériault et al., 2007). In the latter study (Kurrey et al., 2005), Snail and Slug were associated with resistance to chemotherapy and radiotherapy in vitro, related to resistance to p53-induced apoptosis and accompanied by expression of CSC markers.
Knockdown of the chemokine receptor CXCR4 in ovarian carcinoma cells was recently shown to result in reduced invasion and proliferation, with downregulation of Slug and vimentin expression (Wang et al., 2011). Upregulation of Snail and MMP-2, with reduced E-cadherin level, was observed in ovarian carcinoma cells treated with 17β-Estradiol (Ding et al., 2006), with similar results reported for both Snail and Slug in another study (Park et al., 2008a).
Endothelin-1 induced EMT via ILK-mediated inhibition of GSK-3β, resulting in Snail upregulation (Rosanò et al., 2005). In a recent study by the same group, blocking of Endothelin A, the receptor for Endothelin-1 in A2780 cells in vitro resulted in sensitization to chemotherapy and reduced cell growth, with concomitant decrease in Snail levels (Rosanò et al., 2011).
Downregulation of E-cadherin expression by hydrogen peroxide was recently reported to occur via EGF, in a p38 MAPK- and Snail-dependent manner, with no involvement of Slug (Cheng et al., 2010), whereas combination of EGF and TGF-β synergistically induces both Snail and Slug and represses E-cadherin in OVCA429 cells (Xu et al., 2010). Stimulation of cultured OSE by TGF-β induces an EMT-like process, characterized by inability to form an epithelial barrier, sustained increase in Snail and E-cadherin, and transient increase in Slug level, with no effect on Twist and Zeb1 (Zhu et al., 2010). Heparin-binding EGF-like growth factor (HB-EGF), one of the ligands of EGFR, induces EMT phenotype in RMG1 clear cell carcinoma cells through increase in Snail, though not Slug, and reduced E-cadherin levels, with concomitant increase in ILK, VEGF, MMP-9, and integrin-β3 levels, and suppression of Snail expression using siRNA results in reduced HB-EGF levels (Yagi et al., 2008).
The metastasis-associated molecule MTA1 is an additional repressor of E-cadherin based on in vitro analysis of OVCAR-3 cells overexpressing MTA1, with concomitant decrease in ER-β, postulated to occur through increase in Snail and Slug levels (Dannenmann et al., 2008). SKOV-3 cells stably transfected with the glycoprotein Stanniocalcin-2, target of HIF-1, have increased motility and invasive capacity, fibroblast morphology, and higher levels of N-cadherin, vimentin, and Snail, with reduced E-cadherin, under hypoxic conditions (Law and Wong, 2010). The variant isoform of hepatocyte nuclear factor 1 (vHNF1) has the opposite effect, and knockdown of vHNF1 in ovarian carcinoma cell lines leads to reduced E-cadherin expression, whereas Snail and Slug levels are reduced in vHNF1-transfected OSE (Tomassetti et al., 2008).
Semaphorin 3E, a neural molecule involved in axon guidance, is more highly expressed in high-grade endometrioid ovarian carcinoma compared to other histological types of ovarian carcinoma, and mediates EMT via its Plexin-D1 receptors, with increased motility and nuclear expression of Snail (Tseng et al., 2011).
Recently, the authors showed that E-cadherin interferes with spheroid formation in ovarian carcinoma cells in vitro, and that Snail silencing in ES-2 clear cell carcinoma cells using Small Hairpin RNA reduces invasion and MMP-2 activity. Silencing of Pak1, known regulator of Snail, using dominant negative (DN) clones, showed that the latter had reduced attachment to ECM proteins, invasion, and MMP-2 activity compared to constitutively active (CA) and wild-type ES-2 and OVCAR-3 cells. DN Pak1 ES-2 cells additionally had reduced binding to LP9 cells, generated from benign mesothelium (Elloul et al., 2010). In analysis of clinical specimens, Pak1 protein expression was significantly higher in primary carcinomas compared to effusions, and positive correlation was found between Pak1 and Snail expression, suggesting that Pak1 may mediate downregulation of Snail expression in effusions (Elloul et al., 2010).
Snail expression and its clinical role has been the subject of several studies. Nuclear Snail expression was absent in epithelial and stromal cells in normal ovaries and benign ovarian tumors, and was infrequent in borderline tumors, whereas expression was found in >20% of carcinomas. It was, however, unrelated to clinicopathological parameters or survival (Tuhkanen et al., 2009). A similar increase in Snail, as well as in Slug, SIP1, and Twist levels, related to the degree of malignancy was observed by Yoshida et al., and Snail expression was inversely related to that of E-cadherin. Snail, SIP1, and Twist expression was lower in solid metastases compared to primary carcinomas, whereas Slug expression was higher, differences which were significant for Snail and Twist. In this study, Snail expression was significantly related to shorter OS, whereas Slug expression had no prognostic role (Yoshida et al., 2009a).
Analysis of E-cadherin and Snail expression in 48 primary carcinomas and 50 metastases, of which 47 were paired specimens, using immunohistochemistry showed significant association between expression of these two proteins in primary and metastatic specimens. Reduced E-cadherin in primary carcinomas and increased Snail in metastases were associated with poor OS (Blechschmidt et al., 2008). In contrast, Jin et al. reported on significantly lower E-cadherin expression in metastases in analysis of 14 patient-matched primary carcinomas and solid metastases. The authors additionally found significantly higher Snail expression in solid metastases and primary carcinomas from patients diagnosed with advanced-stage disease compared to primary carcinomas from early stage patients, and Snail was observed to be more often localized to the nucleus in advanced-stage compared to early stage tumors (Jin et al., 2010).
In another study, analysis of 25 patient-matched primary carcinomas and solid metastases for the expression of 25 signaling molecules using reverse phase protein arrays showed significant co-expression of Snail with activated EGFR(Tyr1086) and p38(Thr180/Tyr182) in primary OC and with EGFR(Tyr1086) in solid metastases. High Snail expression in primary carcinomas and high p38(Thr180/Tyr182) in metastases were associated with greater risk of death (Hipp et al., 2010).
Snail and Slug mRNA was expressed in ovarian carcinoma effusions, as well as in primary ovarian carcinomas (Figure 1F) and solid metastases, but their levels in effusions by RT-PCR were unrelated to survival (Elloul et al., 2005). Furthermore, while Snail mRNA levels in effusions were comparable to those of primary ovarian carcinomas and solid metastases, protein expression was significantly lower in effusions. Slug mRNA and protein expression was highest in solid metastases and lowest in effusions, with intermediate levels in primary carcinomas (Elloul et al., 2006). Additionally, Snail localized to the cytoplasm rather than the nucleus in uncultured ovarian carcinoma cells from some effusions (Figures 1G,H), suggesting that it is not functional in these cells (Elloul et al., 2006).
Interestingly, exposure of clinical specimens from patients diagnosed with advanced-stage ovarian carcinoma to cisplatin, as well as the OVCA433 cell line, results in reduced E-cadherin and increased Snail, Slug, Twist, and Vimentin mRNA levels, as well as the increased cell surface expression of CSC markers, which correlated with activation of the MAPK member ERK2, presenting a mechanism for development of resistance in ovarian carcinoma (Latifi et al., 2011). The involvement of E-cadherin repressors in chemotherapy response is well in agreement with an earlier study by Bani et al. (2004) in which Slug was shown to be one of the genes that are upregulated in response to Paclitaxel in ovarian carcinoma xenografts in mice by gene expression arrays.
The above-discussed data do not provide a conclusive statement regarding the clinical role of Snail and Slug in ovarian carcinoma, nor do they allow for full appreciation of the dynamics of expression along tumor progression as function of the anatomic site of the tumor, although they do suggest that primary and metastatic tumors have different expression levels of these E-cadherin repressors. Some of the data, particularly those related to effusion specimens, again suggest that ovarian carcinoma cells undergo at least partial mesenchymal-to-epithelial transition (MET), a hypothesis supported by the cytoplasmic localization of Snail in ovarian carcinoma cells in effusions.
The Basic Helix-Loop-Helix Family
The basic helix-loop-helix (bHLH) transcription factor family regulates cell fate during embryogenesis by direct binding to DNA and recruitment of coactivators or repressors, by protein sequestration and through protein–protein interactions. They are divided into three classes. The ubiquitous A class includes E2-2, HEB, and the E12/E47 isoforms of the E2A gene. The tissue-restricted B class includes Twist1 and Twist2, as well as Hand1, Hand2, Paraxis, and Scleraxis, whereas the third group, inhibitory Id proteins, lacks the ability to bind DNA (Franco et al., 2011). Twist proteins have in recent years been recognized as central actors in EMT (Vernon and LaBonne, 2004; Franco et al., 2011).
Twist expression was shown to be related to Paclitaxel resistance in vitro in comparison of the OVCA433 and OVCA432 cell lines (Wang et al., 2004), and analysis of NOS cells resistant to Paclitaxel showed relationship to EMT and Twist, as well as Snail, expression (Kajiyama et al., 2007). Twist was additionally shown to increase adhesion to mesothelial cells in vitro, thereby possibly related to peritoneal metastasis (Terauchi et al., 2007). Loss of MKK4, part of the MAPK signaling cascade, was recently shown to induce EMT in ovarian carcinoma cell lines, as evidenced by increased Twist expression and loss of E-cadherin (Yeasmin et al., 2011). In an additional study, knockdown of Twist in an ovarian carcinoma cell line with CSC characteristics results in decreased hsa-miR-199a and hsa-miR-214 levels, and increase in the level of IKKβ, part of the NFκB pathway, suggesting that Twist is an inhibitor of this pathway in this cell line (Yin et al., 2010).
In a series of clinical ovarian carcinoma of all histological types, Twist expression was associated with shorter OS and PFS, a finding that was an independent prognostic factor in multivariate analysis (Hosono et al., 2007). Similar findings were observed in a study limited to clear cell carcinomas by the same group (Kajiyama et al., 2006). Yoshida et al. (2009a) observed a trend for shorter OS for patients with tumors staining strongly for Twist. Our data indicate that Twist1, Zeb1, and Vimentin mRNA expression is higher in solid metastases compared to primary carcinomas and effusions from OC patients (Elloul et al., 2010), further attesting to the dynamic nature of EMT in OC as function of anatomic site, and suggesting a role in tumor progression.
As for Snail and Slug, these data support a role for Twist in the induction of EMT-related events in OC. The data from studies of clinical material suggests a prognostic role for this protein, but awaits confirmation from studies by other groups.
The Zeb Family
The Zeb family of transcription factors includes Zeb1 and Zeb2/SIP1. They contain zinc finger domains involved in binding target promoters and a POU-like homeodomain involved in protein–protein interactions. Zeb proteins are modulators of TGF-β responses, and are additionally able to bind the transcriptional activators p300 and pCAF. In addition to inducing EMT, Zeb proteins regulate cell cycle- and apoptosis-related proteins (Browne et al., 2010).
microRNAs are short (19–25 nucleotides) non-coding RNAs that modulate gene expression at the post-transcriptional level through binding to the 3′-UTR region of target mRNA, thereby inducing degradation of the mRNA transcript or inhibition of protein translation. Depending on whether miRNAs target oncogenes or tumor suppressor genes, they may act as tumor suppressors or oncogenes, respectively. microRNAs have been under growing focus as modulators of cancer biology in recent years (Filipowicz et al., 2008; Nelson and Weiss, 2008; Sandhu and Garzon, 2011). In ovarian carcinoma, the let-7 and miR-200 families are the most frequently deregulated microRNAs (Mezzanzanica et al., 2010).
Zeb factors negatively regulate the mir-200 family, which induces an epithelial phenotype, and are in turn negatively regulated by the latter. mir-200 factors additionally regulate TGF-β2 expression, as well as other molecules with a central role in cancer biology, including β-catenin, class III β-tubulin, and phospholipase Cγ1 (Brabletz and Brabletz, 2010). The inverse association between mir-200 and Zeb1/Zeb2, and their association with an E-cadherin-positive/Vimentin-negative phenotype was documented in analysis of the NCI-60 cell lines, and these data were supported by experiments in which mir-200 were ectopically expressed or inhibited, with resulting MET and EMT phenotype, respectively. In clinical ovarian carcinoma specimens, direct association was observed between miR-200 and E-cadherin levels (Park et al., 2008b). Chen et al. (2011) recently showed that the mir-429, a miR-200 member, induces MET in ovarian carcinoma cell lines, as evidenced by reduced Zeb1 and Zeb2 and increased E-cadherin levels.
The authors recently applied miRNA array to compare the miRNA profiles of primary ovarian carcinoma and ovarian carcinoma effusions. Analysis of 21 tumors, including 13 effusions and 8 primary carcinomas, identified three sets of miRNAs: one that was highly expressed in both primary carcinomas and effusions, one overexpressed in primary carcinomas, and one overexpressed in effusions. qPCR analysis of a validation set consisting of 45 additional tumors (30 effusions, 15 primary carcinomas) showed, as in the array set, reduced miR-145 and miR-214 and elevated let-7f, miR-182, miR-210, miR-200c, miR-222, and miR-23a levels in effusions compared to primary ovarian carcinomas. In silico target prediction programs identified potential target genes for some of the differentially expressed miRNAs. Expression of ZEB1 and c-Myc, targets of miR-200c, as well as of PAK1 and PTEN, predicted targets of miR-222, showed inverse correlations between miRNA expression levels and the levels of their predicted target genes. In addition, higher expression of the miRNA-processing molecules Ago1, Ago2, and Dicer was observed in effusions compared to primary carcinomas (Vaksman et al., 2011).
Zeb1 was induced in the OV266 ovarian carcinoma cell line in response to estrogen, and high levels of Zeb1 mRNA were found in high-grade serous ovarian carcinomas (Hurt et al., 2008). Tissue transglutaminase (TG2), as enzyme involved in protein post-translational modifications and cross-linking, which is overexpressed in ovarian carcinoma compared to the normal ovary, mediates EMT and increases metastasis in a mouse xenograft ovarian carcinoma model, and antisense silencing of TG2 results in reduced Zeb1, Zeb2, and Slug mRNA levels. TG2 induction of Zeb1 involves the NFκB pathway (Shao et al., 2009). Work by the same group recently showed that TG2 itself is induced by TGF-β, with resulting EMT, spheroid formation and metastasis (Cao et al., 2012). The scaffolding adaptor protein Gab2, involved in activation of the MAPK and PI3K signaling pathways, mediates EMT, with downregulation of E-cadherin and increased migration and invasion, through activation of PI3K and Zeb1 (Wang et al., 2012).
Comparison of the miRNA profiles of 70 clinical primary ovarian carcinoma specimens of the serous, endometrioid and clear cell types and 15 cell lines to those of OSE showed higher miR-200 and reduced Zeb expression in the ovarian carcinoma specimens (Bendoraite et al., 2010). In contrast, high Zeb2/SIP1 levels were observed in ovarian carcinoma effusions, in which higher SIP1/E-cadherin mRNA ratio is also associated with poor OS (Elloul et al., 2005). Based on these observations, Bendoraite et al. (2010) suggested that an initial MET occurs in the ovary, characterized by increased E-cadherin, high miR-200 and reduced Zeb expression, later followed by EMT in progression to ascites, during which Zeb2/SIP1 levels are elevated.
BRCA1-associated ovarian carcinoma cell lines were recently shown to be characterized by a high rate of hypermethylation of p75, a p53-related gene, leading to abrogated binding of its transcriptional repressor Zeb1, with resulting higher p75 levels, leading to apoptosis. Similar findings were observed in clinical specimens when BRCA1-related tumors were compared to those with normal BRCA1. Interestingly, this hypermethylation was lost in recurrent tumors, suggesting a mechanism for chemoresistance in this ovarian carcinoma subset (Ibrahim et al., 2010).
The full biological significance related to the interaction between Zeb family members and miRNAs in ovarian carcinoma awaits elucidation, as is the reason for the opposing alterations in the expression level of Zeb1 and Zeb2/SIP1 in effusions compared to solid lesions. The clinical role of Zeb family members in ovarian carcinoma is similarly undecided at present.
Other Studies
The role of miRs other than miR-200 in regulating EMT in ovarian carcinoma has been investigated in several studies. miR-187, as well as miR-200a, was shown to be overexpressed in ovarian carcinoma cell lines compared to OSE. miR-187 negatively regulated disabled homolog-2 (Dab2), a tumor suppressor gene that induces EMT, as evidenced by measurement of E-cadherin and Vimentin levels, and its levels were inversely related to those of Dab2 protein in clinical specimens. Higher miR-187 levels were associated with better OS in clinical ovarian carcinoma specimens, an observation retained in multivariate analysis (Chao et al., 2012). EGFR was shown to repress the expression of miR-125a, negative regulator of EMT, through PEA3, member of the Ets transcription family, and AT-rich interactive domain 3B (ARID3B), regulator of mesenchymal cell development in the embryo, was identified as a target of miR-125a (Cowden Dahl et al., 2009). Parenthetically, PEA3 was reported to be a marker of poor survival in ovarian carcinoma (Davidson et al., 2003, 2004). Another member of the PEA3 sub-family of Ets factors, ETV5, is upregulated in ovarian tumors compared to normal ovaries, and induces EMT and mediates cell survival and proliferation in ovarian carcinoma cell lines (Llauradó et al., 2012), supporting the role of this family in mediating EMT in this cancer.
Secreted frizzled-related protein 5 (SFRP5), a Wnt antagonist, is hypermethylated in ovarian carcinoma, and the latter is associated with less favorable response to chemotherapy. Silencing of SFRP5 through methylation induces EMT through Twist and activates AKT2 in vitro, which may contribute to chemoresistance in clinical ovarian carcinoma (Su et al., 2010). Chemoresistance in ovarian carcinoma cell lines was additionally shown to be related to EGFR activation, with involvement of ERK and Janus kinases (Jak) signaling and induction of EMT via Snail (Yue et al., 2012).
HMGA2, a high mobility group AT hook (HMGA) protein, belongs to a family of non-histone nuclear proteins involved in chromatin remodeling and gene transcription, and is overexpressed in many cancers, including ovarian carcinoma (Fedele and Fusco, 2010; Mahajan et al., 2010). HMGA2 overexpression transforms OSE cells, leading to tumor formation in a xenograft model in mice, accompanied by reduced E-cadherin and increased Vimentin expression. Global gene expression array analysis comparing OSE overexpressing HMGA2 with control cells showed significantly altered levels of 36 genes, including downregulation of LUM, coding for the tumor suppressor Lumican, which inhibits EMT (Wu et al., 2011).
Antibody-mediated knockdown of CA 125 resulted in EMT-related changes in OVCAR-3 cells, as evidenced by reduced E-cadherin and CK18 and increased N-cadherin and Vimentin expression, with in vitro increase in motility, migration and invasion, EGFR, ERK1/2 and AKT activation, and increased MMP-2 and MMP-9 expression and activity (Comamala et al., 2011).
Neutrophil gelatinase-associated lipocalin (NGAL), a protein stored in neutrophil granules which binds MMP-9, is more highly expressed in ovarian tissue as function of the degree of malignancy, with highest expression in carcinomas. It is more highly expressed in ovarian carcinoma cell lines with epithelial features compared to those with mesenchymal features, and its levels are reduced in cells undergoing EMT (Lim et al., 2007).
MUC4, a mucin that is widely expressed on ovarian carcinoma cells in both solid lesions and effusion specimens (Davidson et al., 2006, 2007), is yet another molecule shown to induce EMT. Ectopic MUC4 overexpression in SKOV-3 cells results in reduced E-cadherin and CK18 expression and increase in Twist1, Twist2, Snail, FAK, and MAPK signaling cascade proteins, as well as in the formation of larger tumors and increased metastatic ability in nude mice (Ponnusamy et al., 2010).
Concluding Comments
Ovarian carcinoma remains a highly lethal disease despite better understanding of its molecular characteristics and growing efforts to apply targeted therapy as an additional modality complementing surgery and chemotherapy. Among the leading causes for our failure to achieve better results in treating ovarian carcinoma patients is the remarkable heterogeneity of the disease along tumor progression, and particularly upon comparison of primary carcinomas, effusion specimens, and solid metastases. Profound molecular alterations expectedly occur when tumors become chemoresistant. In the context of EMT, ovarian carcinoma defies the general characteristics of this process already at its evolution, as evidenced by studies of cadherin expression profiles in OSE, inclusion cysts and carcinomas (Ahmed et al., 2007), the former two still believed by many, including the author of this paper, to be the main precursor of serous ovarian carcinoma. Nevertheless, a recent pathway analysis of data from nine gene profiling array studies in which ovarian carcinoma resistance to platinum-based chemotherapy was studied implicated the TGF-β pathway, which affects both EMT and stemness, as one of the main drivers of chemoresistance in ovarian carcinoma (Helleman et al., 2010). EMT, including in relation to TGF-β signaling, was similarly a main driver of poor survival of ovarian carcinoma patients in a review summarizing gene expression array analyses, in which 108 of 154 genes selected by the authors were EMT-related (Yoshida et al., 2009b).
The data presented in this review suggest that a large number of molecules may induce EMT in ovarian carcinoma cells in vitro through activation of several repressors of epithelial phenotype, in particular E-cadherin. However, the relevance of these data to patient material in terms of clinical behavior, prognosis, and therapeutic intervention is not entirely clear at present. Spindle cells which may indicate transformation from epithelial to mesenchymal phenotype are not frequently observed in clinical specimens of ovarian carcinomas, with the exception of carcinosarcomas, tumors that constitute a separate entity diagnostically and clinically. There is therefore little probability that ovarian carcinoma cells undergo full EMT. The fact that serous carcinoma, the most common histological type of this tumor, frequently expresses EMT-related markers such as N-cadherin and Vimentin, evidence of its close histogenetic relationship to the mesothelium, further confounds matters.
As in other areas of research, drawing a unifying statement from the studies performed to date is also made difficult by the inclusion of different histological types of ovarian carcinoma in the majority of reports, as these have been increasingly regarded to represent different disease entities, both biologically and clinically (Köbel et al., 2008; Prat, 2012). Further difficulties are related to the study of protein vs. mRNA, different methodology, different endpoints (e.g., analyses of similarities vs. differences between primary and metastatic tumors) and the analysis of small cohorts in some of the studies.
Tables 1 and 2 provide an overview of the data gathered in analysis of clinical ovarian carcinoma specimens to date, with respect to anatomic site-related differences in expression of EMT-related molecules and their prognostic role. These suggest the following:
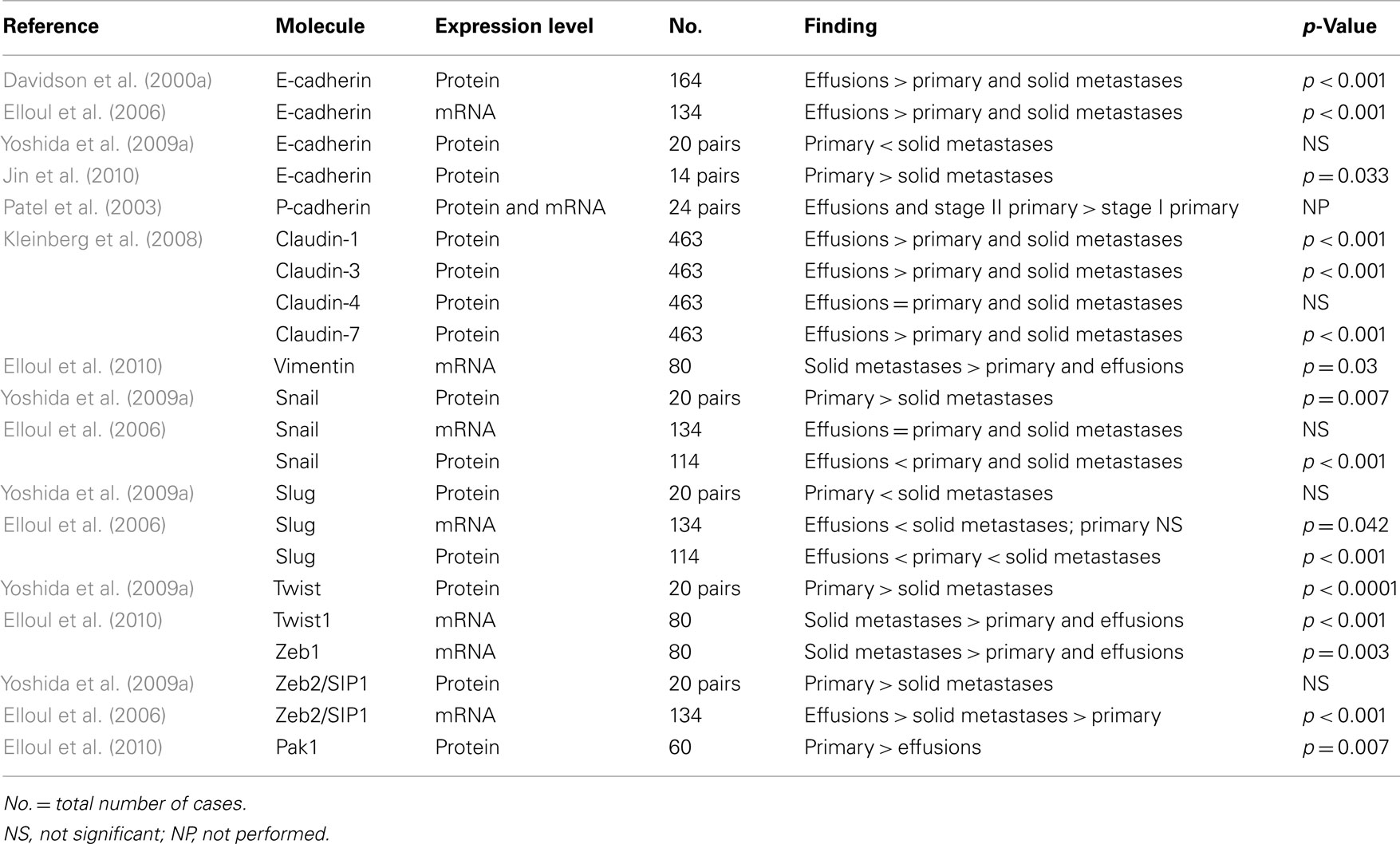
Table 1. Molecules related to epithelial-to-mesenchymal transition in clinical ovarian carcinoma specimens – association with disease progression.
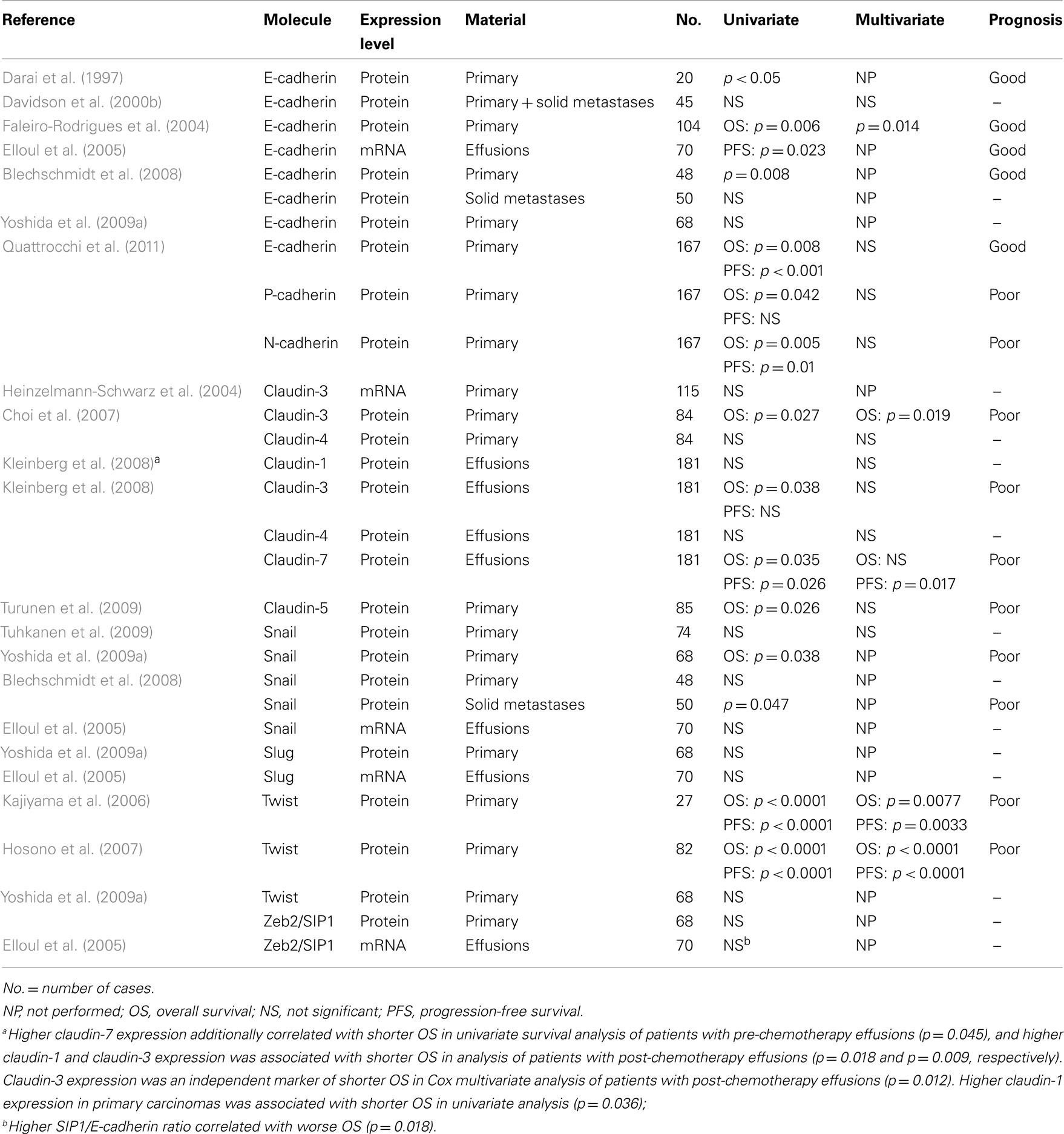
Table 2. Molecules related to epithelial-to-mesenchymal transition in clinical ovarian carcinoma specimens – prognostic relevance.
1. Ovarian carcinoma cells in effusions have significantly different expression profile of EMT-related molecules, with higher levels of E-cadherin and SIP1 and reduced expression of Snail, Slug, Twist, and Zeb1 compared to solid lesions. Data with respect to differences between primary carcinomas and solid metastases are less consistent, with the sole possible exception being the observation that E-cadherin expression is lowest in primary carcinomas.
2. E-cadherin expression is associated with longer survival in the majority of studies, while the opposite is true for its transcriptional suppressors, for claudins and for other cadherins.
Studies of metastatic disease in clinical specimens are nevertheless still too few in this context, and as metastatic tumors are those that eventually lead to the death of the patients, such studies are the ultimate test of relevance and should be the ones to decide the clinical role of EMT-related molecules considered as possible candidates for targeted therapy in OC.
The solution to the above-detailed shortcomings is in performing studies of large tumor series, which include each only one histological type of ovarian carcinoma, with well-characterized cohorts with respect to clinical data, in which a broad panel of EMT-related markers will be analyzed. Analyses should be performed for primary carcinomas, effusions, and solid metastases, preferably in patient-matched specimens. This is most easily achieved applying immunohistochemistry, but can also be performed at the mRNA level. Only this approach will enable us to reach conclusive observations with respect to the occurrence and clinical significance of EMT in ovarian carcinoma.
Conflict of Interest Statement
The authors declare that the research was conducted in the absence of any commercial or financial relationships that could be construed as a potential conflict of interest.
Acknowledgments
Studies by the authors of this review were supported by the Inger and John Fredriksen Foundation for Ovarian Cancer Research, and by the Norwegian Cancer Society and the Research Foundation at the Norwegian Radium Hospital.
References
Acloque, H., Adams, M. S., Fishwick, K., Bronner-Fraser, M., and Nieto, M. A. (2009). Epithelial-mesenchymal transitions: the importance of changing cell state in development and disease. J. Clin. Invest. 119, 1438–1449.
Agarwal, R., and Kaye, S. B. (2003). Ovarian cancer: strategies for overcoming resistance to chemotherapy. Nat. Rev. Cancer 3, 502–516.
Ahmed, N., Thompson, E. W., and Quinn, M. A. (2007). Epithelial-mesenchymal interconversions in normal ovarian surface epithelium and ovarian carcinomas: an exception to the norm. J. Cell. Physiol. 213, 581–588.
Akahira, J. I., Yoshikawa, H., Shimizu, Y., Tsunematsu, R., Hirakawa, T., Kuramoto, H., Shiromizu, K., Kuzuya, K., Kamura, T., Kikuchi, Y., Kodama, S., Yamamoto, K., and Sato, S. (2001). Prognostic factors of stage IV epithelial ovarian cancer: a multicenter retrospective study. Gynecol. Oncol. 81, 398–403.
Bani, M. R., Nicoletti, M. I., Alkharouf, N. W., Ghilardi, C., Petersen, D., Erba, E., Sausville, E. A., Liu, E. T., and Giavazzi, R. (2004). Gene expression correlating with response to paclitaxel in ovarian carcinoma xenografts. Mol. Cancer Ther. 3, 111–121.
Bendoraite, A., Knouf, E. C., Garg, K. S., Parkin, R. K., Kroh, E. M., O’Briant, K. C., Ventura, A. P., Godwin, A. K., Karlan, B. Y., Drescher, C. W., Urban, N., Knudsen, B. S., and Tewari, M. (2010). Regulation of miR-200 family microRNAs and ZEB transcription factors in ovarian cancer: evidence supporting a mesothelial-to-epithelial transition. Gynecol. Oncol. 116, 117–125.
Bignotti, E., Tassi, R. A., Calza, S., Ravaggi, A., Romani, C., Rossi, E., Falchetti, M., Odicino, F. E., Pecorelli, S., and Santin, A. D. (2006). Differential gene expression profiles between tumor biopsies and short-term primary cultures of ovarian serous carcinomas: identification of novel molecular biomarkers for early diagnosis and therapy. Gynecol. Oncol. 103, 405–416.
Blechschmidt, K., Sassen, S., Schmalfeldt, B., Schuster, T., Höfler, H., and Becker, K. F. (2008). The E-cadherin repressor Snail is associated with lower overall survival of ovarian cancer patients. Br. J. Cancer 98, 489–495.
Bonnefoi, H., A’Hern, R. P., Fisher, C., Macfarlane, V., Barton, D., Blake, P., Shepherd, J. H., and Gore, M. E. (1999). Natural history of stage IV epithelial ovarian cancer. J. Clin. Oncol. 17, 765–775.
Boylan, K. L., Misemer, B., Derycke, M. S., Andersen, J. D., Harrington, K. M., Kalloger, S. E., Gilks, C. B., Pambuccian, S. E., and Skubitz, A. P. (2011). Claudin 4 is differentially expressed between ovarian cancer subtypes and plays a role in spheroid formation. Int. J. Mol. Sci. 12, 334–358.
Brabletz, S., and Brabletz, T. (2010). The ZEB/miR-200 feedback loop – a motor of cellular plasticity in development and cancer? EMBO Rep. 11, 670–677.
Browne, G., Sayan, A. E., and Tulchinsky, E. (2010). ZEB proteins link cell motility with cell cycle control and cell survival in cancer. Cell Cycle 9, 886–891.
Cao, L., Shao, M., Schilder, J., Guise, T., Mohammad, K. S., and Matei, D. (2012). Tissue transglutaminase links TGF-β, epithelial to mesenchymal transition and a stem cell phenotype in ovarian cancer. Oncogene. doi:10.1038/onc.2011.429. [Epub ahead of print].
Casagrande, F., Cocco, E., Bellone, S., Richter, C. E., Bellone, M., Todeschini, P., Siegel, E., Varughese, J., Arin-Silasi, D., Azodi, M., Rutherford, T. J., Pecorelli, S., Schwartz, P. E., and Santin, A. D. (2011). Eradication of chemotherapy-resistant CD44+ human ovarian cancer stem cells in mice by intraperitoneal administration of Clostridium perfringens enterotoxin. Cancer 117, 5519–5528.
Cavallaro, U., and Christofori, G. (2004). Cell adhesion and signalling by cadherins and Ig-CAMs in cancer. Nat. Rev. Cancer 4, 118–132.
Chao, A., Lin, C. Y., Lee, Y. S., Tsai, C. L., Wei, P. C., Hsueh, S., Wu, T. I., Tsai, C. N., Wang, C. J., Chao, A. S., Wang, T. H., and Lai, C. H. (2012). Regulation of ovarian cancer progression by microRNA-187 through targeting disabled homolog-2. Oncogene 31, 764–775.
Chen, J., Wang, L., Matyunina, L. V., Hill, C. G., and McDonald, J. F. (2011). Overexpression of miR-429 induces mesenchymal-to-epithelial transition (MET) in metastatic ovarian cancer cells. Gynecol. Oncol. 121, 200–205.
Cheng, J. C., Klausen, C., and Leung, P. C. (2010). Hydrogen peroxide mediates EGF-induced down-regulation of E-cadherin expression via p38 MAPK and snail in human ovarian cancer cells. Mol. Endocrinol. 24, 1569–1580.
Choi, Y. L., Kim, J., Kwon, M. J., Choi, J. S., Kim, T. J., Bae, D. S., Koh, S. S., In, Y. H., Park, Y. W., Kim, S. H., Ahn, G., and Shin, Y. K. (2007). Expression profile of tight junction protein claudin 3 and claudin 4 in ovarian serous adenocarcinoma with prognostic correlation. Histol. Histopathol. 22, 1185–1195.
Cocco, E., Casagrande, F., Bellone, S., Richter, C. E., Bellone, M., Todeschini, P., Holmberg, J. C., Fu, H. H., Montagna, M. K., Mor, G., Schwartz, P. E., Arin-Silasi, D., Azoudi, M., Rutherford, T. J., Abu-Khalaf, M., Pecorelli, S., and Santin, A. D. (2010). Clostridium perfringens enterotoxin carboxy-terminal fragment is a novel tumor-homing peptide for human ovarian cancer. BMC Cancer 10, 349. doi:10.1186/1471-2407-10-349
Comamala, M., Pinard, M., Thériault, C., Matte, I., Albert, A., Boivin, M., Beaudin, J., Piché, A., and Rancourt, C. (2011). Downregulation of cell surface CA125/MUC16 induces epithelial-to-mesenchymal transition and restores EGFR signalling in NIH:OVCAR3 ovarian carcinoma cells. Br. J. Cancer 104, 989–999.
Cormio, G., Maneo, A., Parma, G., Pittelli, M. R., Miceli, M. D., and Bonazzi, C. (1995). Central nervous system metastases in patients with ovarian carcinoma. A report of 23 cases and literature review. Ann. Oncol. 6, 571–574.
Cowden Dahl, K. D., Dahl, R., Kruichak, J. N., and Hudson, L. G. (2009). The epidermal growth factor receptor responsive miR-125a represses mesenchymal morphology in ovarian cancer cells. Neoplasia 11, 1208–1215.
Curtin, J. P., Malik, R., Venkatraman, E. S., Barakat, R. R., and Hoskins, W. J. (1997). Stage IV ovarian cancer: impact of surgical debulking. Gynecol. Oncol. 64, 9–12.
Dahiya, N., Becker, K. G., Wood, W. H. III, Zhang, Y., and Morin, P. J. (2011). Claudin-7 is frequently overexpressed in ovarian cancer and promotes invasion. PLoS ONE 6, e22119. doi:10.1371/journal.pone.0022119
Dannenmann, C., Shabani, N., Friese, K., Jeschke, U., Mylonas, I., and Brüning, A. (2008). The metastasis-associated gene MTA1 is upregulated in advanced ovarian cancer, represses ERbeta, and enhances expression of oncogenic cytokine GRO. Cancer Biol. Ther. 7, 1460–1467.
Darai, E., Scoazec, J. Y., Walker-Combrouze, F., Mlika-Cabanne, N., Feldmann, G., Madelenat, P., and Potet, F. (1997). Expression of cadherins in benign, borderline, and malignant ovarian epithelial tumors: a clinicopathologic study of 60 cases. Hum. Pathol. 28, 922–928.
Davidson, B., Baekelandt, M., and Shih, I. M. (2007). MUC4 is upregulated in ovarian carcinoma effusions and differentiates carcinoma cells from mesothelial cells. Diagn. Cytopathol. 35, 756–760.
Davidson, B., Berner, A., Nesland, J. M., Risberg, B., Berner, H. S., Tropé, C. G., Kristensen, G. B., Bryne, M., and Flørenes, V. A. (2000a). E-cadherin and alpha-, beta-, and gamma-catenin protein expression is up-regulated in ovarian carcinoma cells in serous effusions. J. Pathol. 192, 460–469.
Davidson, B., Gotlieb, W. H., Ben-Baruch, G., Nesland, J. M., Bryne, M., Goldberg, I., Kopolovic, J., and Berner, A. (2000b). E-cadherin complex protein expression and survival in ovarian carcinoma. Gynecol. Oncol. 79, 362–371.
Davidson, B., Goldberg, I., Gotlieb, W. H., Kopolovic, J., Ben-Baruch, G., and Reich, R. (2003). PEA3 is the second Ets family transcription factor involved in tumor progression in ovarian carcinoma. Clin. Cancer Res. 9, 1412–1419.
Davidson, B., Goldberg, I., Reich, R., Tell, L., Baekelandt, M., Kristensen, G. B., Berner, A., and Kopolovic, J. (2004). The clinical role of the PEA3 transcription factor in ovarian and breast carcinoma in effusions. Clin. Exp. Metastasis 21, 191–199.
Davidson, B., Zhang, Z., Kleinberg, L., Li, M., Flørenes, V. A., Wang, T. L., and Shih, I. M. (2006). Gene expression signatures differentiate ovarian/peritoneal serous carcinoma from diffuse malignant peritoneal mesothelioma. Clin. Cancer Res. 12, 5944–5950.
Ding, J. X., Feng, Y. J., Yao, L. Q., Yu, M., Jin, H. Y., and Yin, L. H. (2006). The reinforcement of invasion in epithelial ovarian cancer cells by 17 beta-estradiol is associated with up-regulation of Snail. Gynecol. Oncol. 103, 623–630.
Elloul, S., Elstrand, M. B., Nesland, J. M., Tropé, C. G., Kvalheim, G., Goldberg, I., Reich, R., and Davidson, B. (2005). Snail, Slug, and Smad-interacting protein 1 as novel parameters of disease aggressiveness in metastatic ovarian and breast carcinoma. Cancer 103, 1631–1643.
Elloul, S., Silins, I., Tropé, C. G., Benshushan, A., Davidson, B., and Reich, R. (2006). Expression of E-cadherin transcriptional regulators in ovarian carcinoma. Virchows Arch. 449, 520–528.
Elloul, S., Vaksman, O., Stavnes, H. T., Tropé, C. G., Davidson, B., and Reich, R. (2010). Mesenchymal-to-epithelial transition determinants as characteristics of ovarian carcinoma effusions. Clin. Exp. Metastasis 27, 161–172.
Faleiro-Rodrigues, C., Macedo-Pinto, I., Pereira, D., and Lopes, C. S. (2004). Prognostic value of E-cadherin immunoexpression in patients with primary ovarian carcinomas. Ann. Oncol. 15, 1535–1542.
Feldman, G. B., Knapp, R. C., Order, S. E., and Hellman, S. (1972). The role of lymphatic obstruction in the formation of ascites in a murine ovarian carcinoma. Cancer Res. 32, 1663–1666.
Filipowicz, W., Bhattacharyya, S. N., and Sonenberg, N. (2008). Mechanisms of post-transcriptional regulation by microRNAs: are the answers in sight? Nat. Rev. Genet. 9, 102–114.
Franco, H. L., Casasnovas, J., Rodríguez-Medina, J. R., and Cadilla, C. L. (2011). Redundant or separate entities? – roles of Twist1 and Twist2 as molecular switches during gene transcription. Nucleic Acids Res. 39, 1177–1186. doi:10.1186/1471-2407-6-186
Gao, Z., Xu, X., McClane, B., Zeng, Q., Litkouhi, B., Welch, W. R., Berkowitz, R. S., Mok, S. C., and Garner, E. I. (2011). C terminus of Clostridium perfringens enterotoxin downregulates CLDN4 and sensitizes ovarian cancer cells to Taxol and Carboplatin. Clin. Cancer Res. 17, 1065–1074.
González-Mariscal, L., Betanzos, A., Nava, P., and Jaramillo, B. E. (2003). Tight junction proteins. Prog. Biophys. Mol. Biol. 81, 1–44.
Heinzelmann-Schwarz, V. A., Gardiner-Garden, M., Henshall, S. M., Scurry, J., Scolyer, R. A., Davies, M. J., Heinzelmann, M., Kalish, L. H., Bali, A., Kench, J. G., Edwards, L. S., Vanden Bergh, P. M., Hacker, N. F., Sutherland, R. L., and O’Brien, P. M. (2004). Overexpression of the cell adhesion molecules DDR1, claudin 3, and Ep-CAM in metaplastic ovarian epithelium and ovarian cancer. Clin. Cancer Res. 10, 4427–4436.
Helleman, J., Smid, M., Jansen, M. P., van der Burg, M. E., and Berns, E. M. (2010). Pathway analysis of gene lists associated with platinum-based chemotherapy resistance in ovarian cancer: the big picture. Gynecol. Oncol. 117, 170–176.
Hemavathy, K., Ashraf, S. I., and Ip, Y. T. (2000). Snail/Slug family of repressors: slowly going into the fast lane of development and cancer. Gene 257, 1–12.
Hewitt, K. J., Agarwal, R., and Morin, P. J. (2006). The claudin gene family: expression in normal and neoplastic tissues. BMC Cancer 6, 186.
Hibbs, K., Skubitz, K. M., Pambuccian, S. E., Casey, R. C., Burleson, K. M., Oegema, T. R. Jr., Thiele, J. J., Grindle, S. M., Bliss, R. L., and Skubitz, A. P. (2004). Differential gene expression in ovarian carcinoma: identification of potential biomarkers. Am. J. Pathol. 165, 397–414.
Hipp, S., Berg, D., Ergin, B., Schuster, T., Hapfelmeier, A., Walch, A., Avril, S., Schmalfeldt, B., Höfler, H., and Becker, K. F. (2010). Interaction of Snail and p38 mitogen-activated protein kinase results in shorter overall survival of ovarian cancer patients. Virchows Arch. 457, 705–713.
Hirabayashi, K., and Graham, J. (1970). The genesis of ascites in ovarian cancer. Am. J. Obstet. Gynecol. 106, 492–497.
Hosono, S., Kajiyama, H., Terauchi, M., Shibata, K., Ino, K., Nawa, A., and Kikkawa, F. (2007). Expression of Twist increases the risk for recurrence and for poor survival in epithelial ovarian carcinoma patients. Br. J. Cancer 96, 314–320.
Hough, C. D., Sherman-Baust, C. A., Pizer, E. S., Montz, F. J., Im, D. D., Rosenshein, N. B., Cho, K. R., Riggins, G. J., and Morin, P. J. (2000). Large-scale serial analysis of gene expression reveals genes differentially expressed in ovarian cancer. Cancer Res. 60, 6281–6287.
Hudson, L. G., Zeineldin, R., and Stack, M. S. (2008). Phenotypic plasticity of neoplastic ovarian epithelium: unique cadherin profiles in tumor progression. Clin. Exp. Metastasis 25, 643–655.
Hurt, E. M., Saykally, J. N., Anose, B. M., Kalli, K. R., and Sanders, M. M. (2008). Expression of the ZEB1 (deltaEF1) transcription factor in human: additional insights. Mol. Cell. Biochem. 318, 89–99.
Ibrahim, N., He, L., Leong, C. O., Xing, D., Karlan, B. Y., Swisher, E. M., Rueda, B. R., Orsulic, S., and Ellisen, L. W. (2010). BRCA1-associated epigenetic regulation of p73 mediates an effector pathway for chemosensitivity in ovarian carcinoma. Cancer Res. 70, 7155–7165.
Imai, T., Horiuchi, A., Wang, C., Oka, K., Ohira, S., Nikaido, T., and Konishi, I. (2003). Hypoxia attenuates the expression of E-cadherin via up-regulation of SNAIL in ovarian carcinoma cells. Am. J. Pathol. 163, 1437–1447.
Jin, H., Yu, Y., Zhang, T., Zhou, X., Zhou, J., Jia, L., Wu, Y., Zhou, B. P., and Feng, Y. (2010). Snail is critical for tumor growth and metastasis of ovarian carcinoma. Int. J. Cancer 126, 2102–2111.
Kajiyama, H., Hosono, S., Terauchi, M., Shibata, K., Ino, K., Yamamoto, E., Nomura, S., Nawa, A., and Kikkawa, F. (2006). Twist expression predicts poor clinical outcome of patients with clear cell carcinoma of the ovary. Oncology 71, 394–401.
Kajiyama, H., Shibata, K., Terauchi, M., Yamashita, M., Ino, K., Nawa, A., and Kikkawa, F. (2007). Chemoresistance to paclitaxel induces epithelial-mesenchymal transition and enhances metastatic potential for epithelial ovarian carcinoma cells. Int. J. Oncol. 31, 277–283.
Kalluri, R., and Weinberg, R. A. (2009). The basics of epithelial-mesenchymal transition. J. Clin. Invest. 119, 1420–1428.
Kim, C. J., Lee, J. W., Choi, J. J., Choi, H. Y., Park, Y. A., Jeon, H. K., Sung, C. O., Song, S. Y., Lee, Y. Y., Choi, C. H., Kim, T. J., Lee, J. H., Kim, B. G., and Bae, D. S. (2011). High claudin-7 expression is associated with a poor response to platinum-based chemotherapy in epithelial ovarian carcinoma. Eur. J. Cancer 47, 918–925.
Kleinberg, L., Holth, A., Fridman, E., Schwartz, I., Shih, I. M., and Davidson, B. (2007). The diagnostic role of claudins in serous effusions. Am. J. Clin. Pathol. 127, 928–937.
Kleinberg, L., Holth, A., Trope’, C. G., Reich, R., and Davidson, B. (2008). Claudin upregulation in ovarian carcinoma effusions is associated with poor survival. Hum. Pathol. 39, 747–757.
Köbel, M., Kalloger, S. E., Boyd, N., McKinney, S., Mehl, E., Palmer, C., Leung, S., Bowen, N. J., Ionescu, D. N., Rajput, A., Prentice, L. M., Miller, D., Santos, J., Swenerton, K., Gilks, C. B., and Huntsman, D. (2008). Ovarian carcinoma subtypes are different diseases: implications for biomarker studies. PLoS Med. 5, e232. doi:10.1371/journal.pmed.0050232
Kurrey, N. K., Amit, K., and Bapat, S. A. (2005). Snail and Slug are major determinants of ovarian cancer invasiveness at the transcription level. Gynecol. Oncol. 97, 155–165.
Latifi, A., Abubaker, K., Castrechini, N., Ward, A. C., Liongue, C., Dobill, F., Kumar, J., Thompson, E. W., Quinn, M. A., Findlay, J. K., and Ahmed, N. (2011). Cisplatin treatment of primary and metastatic epithelial ovarian carcinomas generates residual cells with mesenchymal stem cell-like profile. J. Cell. Biochem. 112, 2850–2864.
Law, A. Y., and Wong, C. K. (2010). Stanniocalcin-2 promotes epithelial-mesenchymal transition and invasiveness in hypoxic human ovarian cancer cells. Exp. Cell Res. 316, 3425–3434.
Le Page, C., Puiffe, M. L., Meunier, L., Zietarska, M., de Ladurantaye, M., Tonin, P. N., Provencher, D., and Mes-Masson, A. M. (2009). BMP-2 signaling in ovarian cancer and its association with poor prognosis. J. Ovarian Res. 2, 4.
Li, J., Sherman-Baust, C. A., Tsai-Turton, M., Bristow, R. E., Roden, R. B., and Morin, P. J. (2009a). Claudin-containing exosomes in the peripheral circulation of women with ovarian cancer. BMC Cancer 9, 244. doi:10.1186/1471-2407-9-244
Li, J., Chigurupati, S., Agarwal, R., Mughal, M. R., Mattson, M. P., Becker, K. G., Wood, W. H. III, Zhang, Y., and Morin, P. J. (2009b). Possible angiogenic roles for claudin-4 in ovarian cancer. Cancer Biol. Ther. 8, 1806–1814.
Lim, R., Ahmed, N., Borregaard, N., Riley, C., Wafai, R., Thompson, E. W., Quinn, M. A., and Rice, G. E. (2007). Neutrophil gelatinase-associated lipocalin (NGAL) an early-screening biomarker for ovarian cancer: NGAL is associated with epidermal growth factor-induced epithelio-mesenchymal transition. Int. J. Cancer 120, 2426–2434.
Llauradó, M., Abal, M., Castellví, J., Cabrera, S., Gil-Moreno, A., Pérez-Benavente, A., Colás, E., Doll, A., Dolcet, X., Matias-Guiu, X., Vazquez-Levin, M., Reventós, J., and Ruiz, A. (2012). ETV5 transcription factor is overexpressed in ovarian cancer and regulates cell adhesion in ovarian cancer cells. Int. J. Cancer 130, 1532–1543.
Lonardi, S., Manera, C., Marucci, R., Santoro, A., Lorenzi, L., and Facchetti, F. (2011). Usefulness of Claudin 4 in the cytological diagnosis of serosal effusions. Diagn. Cytopathol. 39, 313–317.
López-Novoa, J. M., and Nieto, M. A. (2009). Inflammation and EMT: an alliance towards organ fibrosis and cancer progression. EMBO Mol. Med. 1, 303–314.
Lu, K. H., Patterson, A. P., Wang, L., Marquez, R. T., Atkinson, E. N., Baggerly, K. A., Ramoth, L. R., Rosen, D. G., Liu, J., Hellstrom, I., Smith, D., Hartmann, L., Fishman, D., Berchuck, A., Schmandt, R., Whitaker, R., Gershenson, D. M., Mills, G. B., and Bast, R. C. Jr. (2004). Selection of potential markers for epithelial ovarian cancer with gene expression arrays and recursive descent partition analysis. Clin. Cancer Res. 10, 3291–3300.
Mahajan, A., Liu, Z., Gellert, L., Zou, X., Yang, G., Lee, P., Yang, X., and Wei, J. J. (2010). HMGA2: a biomarker significantly over-expressed in high grade ovarian serous carcinoma. Mod. Pathol. 23, 673–681.
Mamuya, F. A., and Duncan, M. K. (2012). αV integrins and TGF-β induced EMT; a circle of regulation. J. Cell Mol. Med. 16, 445–455.
Mezzanzanica, D., Bagnoli, M., De Cecco, L., Valeri, B., and Canevari, S. (2010). Role of microRNAs in ovarian cancer pathogenesis and potential clinical implications. Int. J. Biochem. Cell Biol. 42, 1262–1272.
Morita, K., Furuse, M., Fujimoto, K., and Tsukita, S. (1999). Claudin multigene family encoding four-transmembrane domain protein components of tight junction strands. Proc. Natl. Acad. Sci. U.S.A. 96, 511–516.
Nagy, J. A., Masse, E. M., Herzberg, K. T., Meyers, M. S., Yeo, K. T., Yeo, T. K., Sioussat, T. M., and Dvorak, H. F. (1995). Pathogenesis of ascites tumor growth: vascular permeability factor, vascular hyperpermeability, and ascites tumor accumulation. Cancer Res. 55, 360–368.
Nelson, K. M., and Weiss, G. J. (2008). MicroRNAs and cancer: past, present, and potential future. Mol. Cancer Ther. 7, 3655–3660.
Nieto, M. A. (2002). The snail superfamily of zinc-finger transcription factors. Nat. Rev. Mol. Cell Biol. 3, 155–166.
Park, S. H., Cheung, L. W., Wong, A. S., and Leung, P. C. (2008a). Estrogen regulates Snail and Slug in the down-regulation of E-cadherin and induces metastatic potential of ovarian cancer cells through estrogen receptor alpha. Mol. Endocrinol. 22, 2085–2098.
Park, S. M., Gaur, A. B., Lengyel, E., and Peter, M. E. (2008b). The miR-200 family determines the epithelial phenotype of cancer cells by targeting the E-cadherin repressors ZEB1 and ZEB2. Genes Dev. 22, 894–907.
Patel, I. S., Madan, P., Getsios, S., Bertrand, M. A., and MacCalman, C. D. (2003). Cadherin switching in ovarian cancer progression. Int. J. Cancer 106, 172–177.
Pecina-Slaus, N. (2003). Tumor suppressor gene E-cadherin and its role in normal and malignant cells. Cancer Cell Int. 3, 17.
Peinado, H., Olmeda, D., and Cano, A. (2007). Snail, Zeb and bHLH factors in tumour progression: an alliance against the epithelial phenotype? Nat. Rev. Cancer 7, 415–428.
Pon, Y. L., Zhou, H. Y., Cheung, A. N., Ngan, H. Y., and Wong, A. S. (2008). p70 S6 kinase promotes epithelial to mesenchymal transition through snail induction in ovarian cancer cells. Cancer Res. 68, 6524–6532.
Ponnusamy, M. P., Lakshmanan, I., Jain, M., Das, S., Chakraborty, S., Dey, P., and Batra, S. K. (2010). MUC4 mucin-induced epithelial to mesenchymal transition: a novel mechanism for metastasis of human ovarian cancer cells. Oncogene 29, 5741–5754.
Prat, J. (2012). Ovarian carcinomas: five distinct diseases with different origins, genetic alterations, and clinicopathological features. Virchows Arch. 460, 237–249.
Quattrocchi, L., Green, A. R., Martin, S., Durrant, L., and Deen, S. (2011). The cadherin switch in ovarian high-grade serous carcinoma is associated with disease progression. Virchows Arch. 459, 21–29.
Romani, C., Comper, F., Bandiera, E., Ravaggi, A., Bignotti, E., Tassi, R. A., Pecorelli, S., and Santin, A. D. (2009). Development and characterization of a human single-chain antibody fragment against claudin-3: a novel therapeutic target in ovarian and uterine carcinomas. Am. J. Obstet. Gynecol. 201, 70.e1–70.e9.
Rosanò, L., Cianfrocca, R., Spinella, F., Di Castro, V., Nicotra, M. R., Lucidi, A., Ferrandina, G., Natali, P. G., and Bagnato, A. (2011). Acquisition of chemoresistance and EMT phenotype is linked with activation of the endothelin A receptor pathway in ovarian carcinoma cells. Clin. Cancer Res. 17, 2350–2360.
Rosanò, L., Spinella, F., Di Castro, V., Nicotra, M. R., Dedhar, S., de Herreros, A. G., Natali, P. G., and Bagnato, A. (2005). Endothelin-1 promotes epithelial-to-mesenchymal transition in human ovarian cancer cells. Cancer Res. 65, 11649–11657.
Saeki, R., Kondoh, M., Kakutani, H., Tsunoda, S., Mochizuki, Y., Hamakubo, T., Tsutsumi, Y., Horiguchi, Y., and Yagi, K. (2009). A novel tumor-targeted therapy using a claudin-4-targeting molecule. Mol. Pharmacol. 76, 918–926.
Sakai, K., Kamura, T., Hirakawa, T., Saito, T., Kaku, T., and Nakano, H. (1997). Relationship between pelvic lymph node involvement and other disease sites in patients with ovarian cancer. Gynecol. Oncol. 65, 164–168.
Sandhu, S., and Garzon, R. (2011). Potential applications of microRNAs in cancer diagnosis, prognosis, and treatment. Semin. Oncol. 38, 781–787.
Santin, A. D., Zhan, F., Bellone, S., Palmieri, M., Cane, S., Bignotti, E., Anfossi, S., Gokden, M., Dunn, D., Roman, J. J., O’Brien, T. J., Tian, E., Cannon, M. J., Shaughnessy, J. Jr., and Pecorelli, S. (2004). Gene expression profiles in primary ovarian serous papillary tumors and normal ovarian epithelium: identification of candidate molecular markers for ovarian cancer diagnosis and therapy. Int. J. Cancer 112, 14–25.
Shao, M., Cao, L., Shen, C., Satpathy, M., Chelladurai, B., Bigsby, R. M., Nakshatri, H., and Matei, D. (2009). Epithelial-to-mesenchymal transition and ovarian tumor progression induced by tissue transglutaminase. Cancer Res. 69, 9192–9201.
Siegel, R., Ward, E., Brawley, O., and Jemal, A. (2011). Cancer statistics, 2011. CA Cancer J. Clin. 61, 212–236.
Sivertsen, S., Berner, A., Michael, C. W., Bedrossian, C., and Davidson, B. (2006). Cadherin expression in ovarian carcinoma and malignant mesothelioma cell effusions. Acta Cytol. 50, 603–607.
Su, H. Y., Lai, H. C., Lin, Y. W., Liu, C. Y., Chen, C. K., Chou, Y. C., Lin, S. P., Lin, W. C., Lee, H. Y., and Yu, M. H. (2010). Epigenetic silencing of SFRP5 is related to malignant phenotype and chemoresistance of ovarian cancer through Wnt signaling pathway. Int. J. Cancer 127, 555–567.
Sun, C., Yi, T., Song, X., Li, S., Qi, X., Chen, X., Lin, H., He, X., Li, Z., Wei, Y., and Zhao, X. (2011). Efficient inhibition of ovarian cancer by short hairpin RNA targeting claudin-3. Oncol. Rep. 26, 193–200.
Suzuki, M., Kato-Nakano, M., Kawamoto, S., Furuya, A., Abe, Y., Misaka, H., Kimoto, N., Nakamura, K., Ohta, S., and Ando, H. (2009). Therapeutic antitumor efficacy of monoclonal antibody against Claudin-4 for pancreatic and ovarian cancers. Cancer Sci. 100, 1623–1630.
Tassi, R. A., Bignotti, E., Falchetti, M., Ravanini, M., Calza, S., Ravaggi, A., Bandiera, E., Facchetti, F., Pecorelli, S., and Santin, A. D. (2008). Claudin-7 expression in human epithelial ovarian cancer. Int. J. Gynecol. Cancer 18, 1262–1271.
Terauchi, M., Kajiyama, H., Yamashita, M., Kato, M., Tsukamoto, H., Umezu, T., Hosono, S., Yamamoto, E., Shibata, K., Ino, K., Nawa, A., Nagasaka, T., and Kikkawa, F. (2007). Possible involvement of TWIST in enhanced peritoneal metastasis of epithelial ovarian carcinoma. Clin. Exp. Metastasis 24, 329–339.
Thériault, B. L., Shepherd, T. G., Mujoomdar, M. L., and Nachtigal, M. W. (2007). BMP4 induces EMT and Rho GTPase activation in human ovarian cancer cells. Carcinogenesis 28, 1153–1162.
Thiery, J. P. (2002). Epithelial-mesenchymal transitions in tumour progression. Nat. Rev. Cancer 2, 442–454.
Thiery, J. P., Acloque, H., Huang, R. Y., and Nieto, M. A. (2009). Epithelial-mesenchymal transitions in development and disease. Cell 139, 871–890.
Tomassetti, A., De Santis, G., Castellano, G., Miotti, S., Mazzi, M., Tomasoni, D., Van Roy, F., Carcangiu, M. L., and Canevari, S. (2008). Variant HNF1 modulates epithelial plasticity of normal and transformed ovary cells. Neoplasia 10, 1481–1492. [3 pages following 1492].
Tseng, C. H., Murray, K. D., Jou, M. F., Hsu, S. M., Cheng, H. J., and Huang, P. H. (2011). Sema3E/plexin-D1 mediated epithelial-to-mesenchymal transition in ovarian endometrioid cancer. PLoS ONE 6, e19396. doi:10.1371/journal.pone.0019396
Tsukita, S., Furuse, M., and Itoh, M. (2001). Multifunctional strands in tight junctions. Nat. Rev. Mol. Cell Biol. 2, 285–293.
Tsuruchi, N., Kamura, T., Tsukamoto, N., Akazawa, K., Saito, T., Kaku, T., To, N., and Nakano, H. (1993). Relationship between paraaortic lymph node involvement and intraperitoneal spread in patients with ovarian cancer – a multivariate analysis. Gynecol. Oncol. 49, 51–55.
Tuhkanen, H., Soini, Y., Kosma, V. M., Anttila, M., Sironen, R., Hämäläinen, K., Kukkonen, L., Virtanen, I., and Mannermaa, A. (2009). Nuclear expression of Snail1 in borderline and malignant epithelial ovarian tumours is associated with tumour progression. BMC Cancer 9, 289. doi:10.1186/1471-2407-9-289
Turunen, M., Talvensaari-Mattila, A., Soini, Y., and Santala, M. (2009). Claudin-5 overexpression correlates with aggressive behavior in serous ovarian adenocarcinoma. Anticancer Res. 29, 5185–5189.
Vaksman, O., Tuft Stavnes, H., Kærn, J., Trope’, C. G., Davidson, B., and Reich, R. (2011). miRNA profiling along tumor progression in ovarian carcinoma. J. Cell. Mol. Med. 15, 1593–1602.
Van Itallie, C. M., and Anderson, J. M. (2006). Claudins and epithelial paracellular transport. Annu. Rev. Physiol. 68, 403–429.
Vernon, A. E., and LaBonne, C. (2004). Tumor metastasis: a new twist on epithelial-mesenchymal transitions. Curr. Biol. 14, R719–R721.
Wang, J., Cai, J., Han, F., Yang, C., Tong, Q., Cao, T., Wu, L., and Wang, Z. (2011). Silencing of CXCR4 blocks progression of ovarian cancer and depresses canonical Wnt signaling pathway. Int. J. Gynecol. Cancer 21, 981–987.
Wang, X., Ling, M. T., Guan, X. Y., Tsao, S. W., Cheung, H. W., Lee, D. T., and Wong, Y. C. (2004). Identification of a novel function of TWIST, a bHLH protein, in the development of acquired taxol resistance in human cancer cells. Oncogene 23, 474–482.
Wang, Y., Sheng, Q., Spillman, M. A., Behbakht, K., and Gu, H. (2012). Gab2 regulates the migratory behaviors and E-cadherin expression via activation of the PI3K pathway in ovarian cancer cells. Oncogene. doi:10.1038/onc.2011.435. [Epub ahead of print].
Wu, J., Liu, Z., Shao, C., Gong, Y., Hernando, E., Lee, P., Narita, M., Muller, W., Liu, J., and Wei, J. J. (2011). HMGA2 overexpression-induced ovarian surface epithelial transformation is mediated through regulation of EMT genes. Cancer Res. 71, 349–359.
Xu, Z., Jiang, Y., Steed, H., Davidge, S., and Fu, Y. (2010). TGFβ and EGF synergistically induce a more invasive phenotype of epithelial ovarian cancer cells. Biochem. Biophys. Res. Commun. 401, 376–381.
Yagi, H., Yotsumoto, F., and Miyamoto, S. (2008). Heparin-binding epidermal growth factor-like growth factor promotes transcoelomic metastasis in ovarian cancer through epithelial-mesenchymal transition. Mol. Cancer Ther. 7, 3441–3451.
Yeasmin, S., Nakayama, K., Rahman, M. T., Rahman, M., Ishikawa, M., Katagiri, A., Iida, K., Nakayama, N., and Miyazaki, K. (2011). Loss of MKK4 expression in ovarian cancer: a potential role for the epithelial to mesenchymal transition. Int. J. Cancer 128, 94–104.
Yin, G., Chen, R., Alvero, A. B., Fu, H. H., Holmberg, J., Glackin, C., Rutherford, T., and Mor, G. (2010). TWISTing stemness, inflammation and proliferation of epithelial ovarian cancer cells through MIR199A2/214. Oncogene 29, 3545–3553.
Yoshida, H., Sumi, T., Zhi, X., Yasui, T., Honda, K., and Ishiko, O. (2011). Claudin-4: a potential therapeutic target in chemotherapy-resistant ovarian cancer. Anticancer Res. 31, 1271–1277.
Yoshida, J., Horiuchi, A., Kikuchi, N., Hayashi, A., Osada, R., Ohira, S., Shiozawa, T., and Konishi, I. (2009a). Changes in the expression of E-cadherin repressors, Snail, Slug, SIP1, and Twist, in the development and progression of ovarian carcinoma: the important role of Snail in ovarian tumorigenesis and progression. Med. Mol. Morphol. 42, 82–91.
Yoshida, S., Furukawa, N., Haruta, S., Tanase, Y., Kanayama, S., Noguchi, T., Sakata, M., Yamada, Y., Oi, H., and Kobayashi, H. (2009b). Expression profiles of genes involved in poor prognosis of epithelial ovarian carcinoma: a review. Int. J. Gynecol. Cancer 19, 992–997.
Yuan, X., Lin, X., Manorek, G., Kanatani, I., Cheung, L. H., Rosenblum, M. G., and Howell, S. B. (2009). Recombinant CPE fused to tumor necrosis factor targets human ovarian cancer cells expressing the claudin-3 and claudin-4 receptors. Mol. Cancer Ther. 8, 1906–1915.
Yue, P., Zhang, X., Paladino, D., Sengupta, B., Ahmad, S., Holloway, R. W., Ingersoll, S. B., and Turkson, J. (2012). Hyperactive EGF receptor, Jaks and Stat3 signaling promote enhanced colony-forming ability, motility and migration of cisplatin-resistant ovarian cancer cells. Oncogene. doi:10.1038/onc.2011.409. [Epub ahead of print].
Zeisberg, M., and Neilson, E. G. (2009). Biomarkers for epithelial-mesenchymal transitions. J. Clin. Invest. 119, 1429–1437.
Zhu, Y., Nilsson, M., and Sundfeldt, K. (2010). Phenotypic plasticity of the ovarian surface epithelium: TGF-beta 1 induction of epithelial to mesenchymal transition (EMT) in vitro. Endocrinology 151, 5497–5505.
Keywords: ovarian carcinoma, epithelial–mesenchymal transition, mesenchymal–epithelial transition, metastasis, tumor progression, chemotherapy, prognosis
Citation: Davidson B, Tropé CG and Reich R (2012) Epithelial–mesenchymal transition in ovarian carcinoma. Front. Oncol. 2:33. doi: 10.3389/fonc.2012.00033
Received: 07 January 2012; Accepted: 21 March 2012;
Published online: 10 April 2012.
Edited by:
Nicolas Wentzensen, National Cancer Institute, USAReviewed by:
Britton Trabert, National Institutes of Health, USAIe-Ming Shih, Johns Hopkins Medical Institutions, USA
Copyright: © 2012 Davidson, Tropé and Reich. This is an open-access article distributed under the terms of the Creative Commons Attribution Non Commercial License, which permits non-commercial use, distribution, and reproduction in other forums, provided the original authors and source are credited.
*Correspondence: Ben Davidson, Division of Pathology, Norwegian Radium Hospital, Oslo University Hospital, N-0310 Oslo, Norway. e-mail:YmVuZEBtZWRpc2luLnVpby5ubw==