- 1 Swedish Radiosurgery Center, Swedish Medical Center, Seattle, WA, USA
- 2 Swedish Cancer Center, Swedish Medical Center, Seattle, WA, USA
- 3 Swedish First Hill Diagnostic Imaging Center, Swedish Medical Center, Seattle, WA, USA
We evaluate the CyberKnife (Accuray Incorporated, Sunnyvale, CA, USA) for non-invasive delivery of accelerated partial breast irradiation (APBI) in early breast cancer patients. Between 6/2009 and 5/2011, nine patients were treated with CyberKnife APBI. Normal tissue constraints were imposed as outlined in the National Surgical Adjuvant Breast and Bowel Project B-39/Radiation Therapy Oncology Group 0413 (NSABP/RTOG) Protocol (Vicini and White, 2007). Patients received a total dose of 30 Gy in five fractions (group 1, n = 2) or 34 Gy in 10 fractions (group 2, n = 7) delivered to the planning treatment volume (PTV) defined as the clinical target volume (CTV) +2 mm. The CTV was defined as either the lumpectomy cavity plus 10 mm (n = 2) or 15 mm (n = 7). The cavity was defined by a T2-weighted non-contrast breast MRI fused to a planning non-contrast thoracic CT. The CyberKnife Synchrony system tracked gold fiducials sutured into the cavity wall during lumpectomy. Treatments started 4–5 weeks after lumpectomy. The mean PTV was 100 cm3 (range, 92–108 cm3) and 105 cm3 (range, 49–241 cm3) and the mean PTV isodose prescription line was 70% for groups 1 and 2, respectively. The mean percent of whole breast reference volume receiving 100 and 50% of the dose (V100 and V50) for group 1 was 11% (range, 8–13%) and 23% (range, 16–30%) and for group 2 was 11% (range, 7–14%) and 26% (range, 21–35.0%), respectively. At a median 7 months follow-up (range, 4–26 months), no acute toxicities were seen. Acute cosmetic outcomes were excellent or good in all patients; for those patients with more than 12 months follow-up the late cosmesis outcomes were excellent or good. In conclusion, the lack of observable acute side effects and current excellent/good cosmetic outcomes is promising. We believe this suggests the CyberKnife is a suitable non-invasive radiation platform for delivering APBI with achievable normal tissue constraints.
Introduction
The American Cancer Society estimated 192,370 new breast cancers were diagnosed in 2009 (Jemal et al., 2009). Due to a widespread increase in mammography screening and improved imaging technology, many of these tumors are found early and localized to the breast with nearly 25% presenting as ductal carcinoma in situ (DCIS). Following a lumpectomy with clear margins (≥2 mm), 90% of recurrences in women who present with early disease (stage 0, I, II) have been observed to occur within 10 mm of the resection cavity (Clark et al., 1992; Vicini et al., 2003, 2004). This observation has led to an enormous interest in accelerated partial breast irradiation (APBI) for early breast cancer over the last decade. Multi-catheter (King et al., 2000; Polgar et al., 2002; Benitez et al., 2004) and balloon catheter brachytherapy (Cuttino et al., 2008; Nelson et al., 2009; Vicini et al., 2011) initially paved the way. However, because of the invasiveness of these procedures, their steep learning curve and risks of infection, lumpectomy cavity coverage with three-dimensional conformal radiotherapy (3D-CRT), and intensity-modulated radiotherapy (IMRT) have grown in popularity. Unfortunately, these external beam techniques come with a price: to assure coverage of a moving target beneath the fixed beam of a linear accelerator, the planning target volumes (PTVs) are enlarged compared to brachytherapy volumes. This PTV increase exposes more normal tissue to the effects of ionizing radiation. Although a recent presentation at the ASCO Breast Cancer Symposium reported that at a mean 36.7 months less than 14% of the 1,367 women enrolled in the 3D-CRT arm of the NSABP B-39/RTOG 0413 trial had Grade 2 or 3 fibrosis–cosmesis and fibrosis-deep connective tissue toxicities (Julian et al., 2010), other institutions on a smaller scale have not been able to duplicate the results and have published their concerns about poor cosmetic outcomes (Hepel et al., 2009; Jagsi et al., 2010).
The CyberKnife (Accuray Incorporated, Sunnyvale, CA, USA) offers an appealing APBI treatment option: non-invasive external beam radiation delivery mimicking the dosimetry of a breast brachytherapy implant. A frameless robotic stereotactic radiosurgery system, the CyberKnife provides image-guidance for continuous tracking of target motion with respiration and patient movement (Kilby et al., 2010). This image-guided tracking system allows treatments to be delivered without concerns of set-up reproducibility or patient motion. As a result, the CyberKnife tracks targets that move with respiration with great precision (Wong et al., 2007; Hoogeman et al., 2009). Thus, using the CyberKnife for APBI allows one to create a smaller PTV, resulting in less radiation exposure to adjacent normal tissue and, potentially, more agreeable cosmetic outcomes. Also, unlike brachytherapy, the CyberKnife isodose volumes easily conform to irregularly shaped cavities, are not impeded by air and fluid pockets, and are easy to constrain, thus limiting potential skin and chest wall injuries. Indeed, researchers at the University of Texas Southwestern Medical recently compared CyberKnife and 3D-CRT treatment plans. They found that APBI treatment plans achieved highly conformal target coverage and reduce the dose to nearby organs at risk relative to 3D-CRT plans (Heinzerling et al., 2010). A treatment planning study by Fox Chase reached similar conclusions for boost dose distributions produced for CyberKnife (Fan et al., 2010). Given these potential benefits, we present the technical set-up, physics planning, treatment, and early outcomes of nine patients who received APBI using the CyberKnife.
Materials and Methods
Patient Selection and Eligibility
This is an IRB approved retrospective analysis of patients treated with CyberKnife APBI at the Swedish Cancer Center. Patients eligible for CyberKnife APBI were over 45 years of age with stage 0, I, or IIA histologically confirmed invasive non-lobular carcinoma or DCIS. Axillary staging was not required for patients with DCIS. Lesion size was required to be less than or equal to 3 cm and treatable by excision. Patients with infiltrating ductal carcinoma were required to have a negative sentinel node sampling or axillary dissection by routine histologic examination (H&E). A negative pre-operative bilateral breast MRI was required to evaluate disease extent and rule out additional disease foci in other parts of the ipsilateral and contralateral breast. Additional eligibility criteria following excision or re-excision included negative inked histologic margins of at least 2 mm or better. Negative post-excision mammograms are required for DCIS tumors, if prior calcifications were seen. Patient exclusion criteria included invasive lobular or multicentric carcinoma, pregnancy, histologically confirmed positive axillary lymph nodes, collagen vascular disease, and tumors that involved the skin or that had diffuse suspicious microcalcifications on mammography. All patients were enrolled with initiation of CyberKnife treatment within 42 days of last breast surgery.
Patients seen in consultation by a radiation oncologist, refused the National Surgical Adjuvant Breast and Bowel Project B-39/Radiation Therapy Oncology Group 0413 (NSABP/RTOG) protocol (Vicini and White, 2007) and elected APBI treatment with the CyberKnife. A written informed consent was obtained for each patient regarding radiation risks. In our series, all patients except one were considered “suitable” or “cautionary” candidates as outlined in the ASTRO consensus statement for APBI (Smith et al., 2009). The one exception was 46 years of age at the time of diagnosis and tested positive for a genetic mutation of breast cancer type 1 susceptibility protein (BRCA1). She received counseling from more than one physician and more than one institution that the standard of care was whole breast irradiation. She was also informed of her risks in developing future cancers of the breast, ovaries, and colon.
Treatment Planning
Patients were treated in the supine position, head first with their arms at their side. During treatment, cavity motion was tracked using the CyberKnife Synchrony system (Accuray Incorporated, Sunnyvale, CA, USA). The Synchrony system uses a combination of fiducials implanted around the resection cavity and light emitting diodes (LEDs) placed over the ipsilateral breast, which are tracked continuously using a CCD camera. The position of the LEDs is correlated with the simultaneous position of the cavity as revealed by the fiducials in orthogonal x-rays. Based on the correlation between the position of the LEDs and the internal fiducials, a correlation model predicts the location of the tumor throughout the respiratory cycle and moves the treatment beam to remain on target with the treatment volume. The system has been shown in end-to-end phantom tests to be accurate to within 1.5 mm (Muacevic et al., 2007; Wong et al., 2007).
For this study, four to five, 2-mm gold markers (manufacturer NMPE: product number MT-NW-887-864) were used. In an earlier unpublished technical communication from the CyberKnife protocol development committee, it was shown that the commonly used titanium clips could not be tracked reliably on imaging when they were superimposed over the bony structures of the spine, humerus, or ribs. On the other hand, these bio-compatible small gold markers, with a high atomic number, allow for good contrast on imaging.
In 11 tumors, the fiducials were sutured into the cavity wall by the breast surgeon at the time of lumpectomy. One marker each identified the superior, inferior, medial, lateral, and deep margin of the cavity. CyberKnife treatment planning was generally performed 3 days before treatment which was performed 4–5 weeks post surgery. Published series show this time period has the least amount of volume change in the seroma identifying the lumpectomy cavity (Weed et al., 2004).
In one patient with bilateral tumors, interventional radiology placed fiducials under CT guidance around the lumpectomy cavity 2 weeks after lumpectomy on the contralateral side. In this case the patient was not a candidate for 3D-CRT because the lumpectomy cavity was located in the high axilla. In addition, this patient was aggressively seeking APBI and not willing to undergo any other treatment. Thus, while visualization of the lumpectomy cavity can be difficult under CT we believed this fiducial placement would be acceptable given we perform CT/MRI fusion for treatment planning. Fiducial implantation proceeded with four needles that were separately loaded, each with one fiducial. The fiducials were then placed 5–10 mm from the superior, inferior, medial, and lateral cavity wall. Treatment planning was performed 1 week after fiducial implantation to allow the fiducials to stabilize and to minimize the risk of fiducial migration. The PTV was located in the tail of the breast. At the time of treatment planning it was observed that due to the target’s lateral location, optimal coverage, and conformality of the PTV could not be achieved without depositing excessive dose in the ipsilateral lung. The CyberKnife beam set generated in the case of lateral and posterior breast tumor locations is limited due to geometric factors which prevent the creation of lateral oblique tangential beams. As a result of all these factors, the patient was encouraged and agreed to whole breast irradiation treatment in place of APBI to one lumpectomy cavity site. The site with fiducials implanted during surgery was treated with the CyberKnife.
During the treatment planning process, fiducials were located on a non-contrast CT scan of the thorax. If the lumpectomy cavity was ill-defined on CT, a non-contrast MRI of the ipsilateral breast was obtained. Both scans were acquired in the treatment position with the patient supine, arms at their sides. Patient’s torsos were elevated from the CT scanner’s couch using a 2″ Styrofoam board. As a result, their arm position on the ipsilateral breast side was more vertical, thus offering more solid angles for the CyberKnife beams to aim at the tumor without intersecting the ipsilateral arm. Care was taken to avoid breast/cavity deformation from the position of the ipsilateral arm or the MRI breast coils. The same support bra was worn for both imaging and treatment. All metal clasps and wires from the bra were removed before the planning scans were obtained. In addition, the MRI breast coils were suspended above the breast using various foamy supports to avoid deformation of the breast/cavity.
Late in our series we found that an alpha cradle better immobilized the patient’s arm during simulation and treatment. The decreased breast deformation and variability of the arm positioning resulted in more accurate CT–MR fusions. Also, faster patient set-up times were achieved. Specifically, the 3- to 5-cm thick alpha cradle, which is elevated on a Styrofoam board, serves to elevate the patient’s body and allows their arms to drop at their side below the level of the chest. All subsequent patients (n = 4) were positioned in the alpha cradle both during the simulation CT and treatment. The CT images were started at the mandible and extended several centimeters below the inframammary fold. The CT slice thickness was 1.25 mm.
For treatment planning, the CT scans and MRI images were exported to the MultiPlan treatment planning software (Accuray Incorporated, Sunnyvale, CA, USA) and fused. When an MRI was used, the lumpectomy cavity was best delineated on the T2 axial or STIR MRI images. The fiducials were best seen on the T2* gradient-echo sequence. Once the T2*-CT registration transform was obtained it was copied to the other MR sequences and used in delineation, particularly to the STIR sequence which was considered the most useful. Often the cavity could not be outlined for target identification on the CT alone due to the adjacent breast tissue density or artifact scatter from the fiducials. In such cases, both of these issues were overcome with the MRI imaging capabilities (Figure 1).
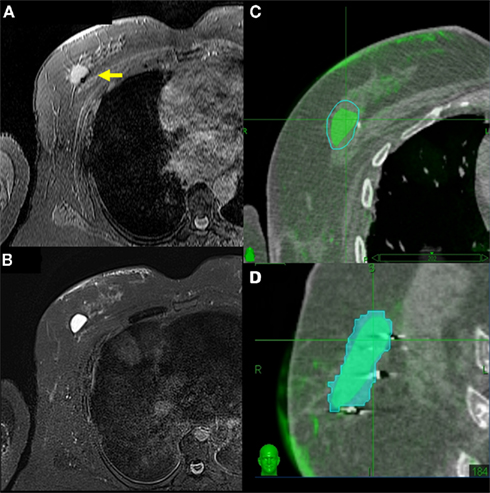
Figure 1. Example of MRI images and image fusion. (A) T2* gradient-echo MRI image, yellow arrow denotes the fiducial markers. (B) STIR MRI image showing resection cavity. (C,D) Axial and sagittal fused images.
Based upon an α/β ratio of 4.6 Gy for breast cancer tumor control, an α/β ratio of 3.4 Gy for late changes in the breast appearance (Bentzen et al., 2008a,b), and encouraging data from Dr. Silvia Formenti at New York University (Formenti, 2005) we initially selected a total dose of 30 Gy delivered in five fractions. Normal tissue dose constraints were imposed as outlined in the NSABP/RTOG Protocol (Vicini and White, 2007). Unfortunately without published data to support the use of the stereotactic platform in the treatment of APBI, patient accrual was slow due to limited re-imbursement. As a result, a decision was made to follow the NSAPB/RTOG protocol (Vicini and White, 2007) so that the dose as well as the fractionation scheme was matched. However, with a dose profile more similar to that observed with the NSABP brachytherapy arm than the 3D-CRT arm of the protocol, a second decision had to be made to expand the excision cavity volume (ECV) by 10 or 15 mm. Of interest, the multi-catheter study arm of the NSAPB/RTOG study uses a CTV defined as an expanded volume of the ECV by 15 mm and in the single catheter arm this was reduced to the ECV plus 10 mm. Based upon evidence that 90% of tumor cells remaining after lumpectomy are within 10 mm of the cavity edge (Clark et al., 1992; Vicini et al., 2003, 2004) and our experience with brachytherapy implants whereby we observed minor fluid and air pockets surrounding the device can prohibit the balloon from fully compressing adjacent breast tissue, we initially choose a CTV defined as the ECV + 10 mm. However, after treating two patients with a CTV defined as the ECV + 10 mm and documenting the exceedingly small volumes for the ipsilateral breast receiving the prescribed dose and 50% of the prescribed dose, we elected to continue our series with expanded volumes of 15 mm instead of 10 mm.
Specifically, our patient population received either 30 Gy in five fractions (group 1) or 34 Gy in 10 fractions (group 2) delivered to the PTV. Treatment was typically performed every day or within 2 weeks as needed based upon scheduling restrictions. The PTV was defined as the CTV plus a 2-mm margin. The 2-mm margin was added to accommodate for the possible tracking error of the fiducials. The CTV itself was defined as the lumpectomy cavity plus a margin of either 10 or 15 mm. For two patients in group 2, the CTV was defined as the lumpectomy cavity plus 10 mm. For all other patients the CTV was defined as the lumpectomy cavity plus 15 mm. No additional volumes were considered necessary to account for variability in day-to-day set-up or patient mobility.
All patient treatments were planned with sequential optimization on the MultiPlan System (version 8.0/3.0). The dose distribution calculations were performed using the ray-tracing algorithms with heterogeneity correction and the appropriate CT electron density model. The result of dose calculation using this simple algorithm was validated in a couple of cases using the Monte Carlo dose calculation algorithm available in version 3.5 of the treatment planning system. Excellent agreement with less than 2% difference was observed for both algorithms. Dose volume histogram (DVH) analyses were conducted to determine whether dose constraints established in the NSABP/RTOG trial could be met. In considering the NSABP/RTOG dose constraints it is important to understand that several of the NSABP constraints for 3D-CRT are in terms of volumes whereas the radiosurgery constraints are maximum point doses. Specifically, the contralateral breast, thyroid, skin, and chest wall are radiosurgery maximum point doses. On a related issue, if we looked at the range of doses and volumes within a constraint for CyberKnife versus 3D-CRT, the DVH for CyberKnife would drop off faster for the lung, thyroid, and heart (i.e., the volumes for the higher doses within the constraints are extremely small). As a result we used the NSABP dose constraints as a guide and have adapted the dose constraints as appropriate for radiosurgery maximum point doses. Furthermore, depending on the location of the lumpectomy site, particularly for very medial inner quadrant or lower inner quadrant lesions, higher contralateral breast point doses and higher volumes of heart or lung receiving the suggested dose as outlined in the national study were accepted. Lastly, while we did not contour the arms as critical structures, we did examine the arm dose for all patients during treatment planning and verified suitable arm point dose. We have now added contouring of the arms to our treatment planning protocol.
Follow-Up, Toxicity, and Cosmesis
Follow-up visits were conducted by the treating radiation oncologist 4 weeks and 6 months after completion of treatment and annually thereafter. At each visit, patients were asked to fill out the NSABP/RTOG Quality of Life Questionnaire (Vicini and White, 2007). Follow-up breast mammograms were obtained at 6 months then annually. Annual breast MRI’s start at 1 year post-treatment. As a result, patients are being imaged every 6 months. Toxicity was assessed using the National Cancer Institute’s Common Terminology Criteria for Adverse Events. Cosmesis was assessed using the four-point cosmetic rating scale of the NSABP/RTOG protocol (Vicini and White, 2007). Using this scale, an excellent outcome was defined as “minimal or no difference.” A good cosmesis was defined as “a slight difference.” Fair or poor was defined as “obvious differences…involving a quarter or less of the breast” or “as marked change…involving more than a quarter of the breast tissue,” respectively.
Results
Patients
Ten patients with 12 early breast cancers were planned for treatment using CyberKnife APBI. Table 1 provides a summary of patient and tumor characteristics for the treated patients. Two patients had bilateral tumors. Of these two patients, one patient with DCIS had a tumor less than 2 mm without necrosis and clear margins; this patient elected no treatment to that site other than close observation. For the second bilateral breast cancer patient, whole breast irradiation was used to treat an infiltrating ductal carcinoma located in the tail of the breast. As described above, this tumor site location posed a difficult target to reach with the CyberKnife without including beams that entered or exited through the ipsilateral lung.
At the time of treatment, the fiducials in two patients were in different positions relative to the cavity identified on the treatment planning CT. In these patients, cavity volume reduction had occurred as a result of fluid absorption. In both patients, a second set of scans including a CT and MRI was obtained and the targets were re-planned with the treatment scheduled 2 days later. One of these patients was able to be treated. However, the other patient’s cavity continued to shrink and CyberKnife treatment was aborted in favor of 3D-CRT. Consequently, nine patients completed APBI using the CyberKnife.
Dosimetry and Treatment
Patients received either 30 Gy in five fractions (group 1, n = 2) or 34 Gy in 10 fractions (group 2, n = 7) delivered to the PTV. In addition to prescribing to a lower isodose than recommended in the 3D-CRT arm of the national study, a field within a field was created to force the high dose region into the fluid filled lumpectomy cavity. When 34 Gy was prescribed to the PTV, the cavity wall in most cases received less than 40 Gy. During treatment planning, DVH analyses were conducted to determine whether dose constraint guidelines, as established by the NSABP/RTOG trial, could be met. Tables 2 and 3 summarize the treatment volumes for the patients in groups 1 and 2. Of note, the contralateral lung dose and heart dose were higher than the NSABP 3D-CRT guidelines and reflect the location of the lumpectomy cavity in the breast. Similarly, two patients had an extremely medial central and lower inner quadrant lumpectomy cavity resulting in a point dose calculation from the DVH of 8 and 2 Gy in the contralateral breast. For the largest contralateral breast point dose of 8 Gy, the volume of the breast that received 0.5 Gy was only 1.5%. For these patients we evaluated radiation treatment alternatives (3D-CRT or tangents) and concluded that dose to the heart, lung, and contralateral breast could be higher for 3D-CRT or tangents. In addition, the contralateral lung receiving 1.7 Gy in one patient was 19% (NSABP guidelines <15%). This patient was an outlier as the next largest volume was 6.5% and after that 1%. Likewise, the heart receiving V1.7 in one patient with right sided breast cancer was 19% (NSABP guidelines <5%). The next highest volumes were 10% for two patients and 6% for one patient; all others were below 5%. As above, these numbers are reflective of the lumpectomy location in the breast. Lastly, one patient received a 1.3-Gy maximum dose to the thyroid which was slightly higher than the NSABP/RTOG protocol constraint of less than 1 Gy.
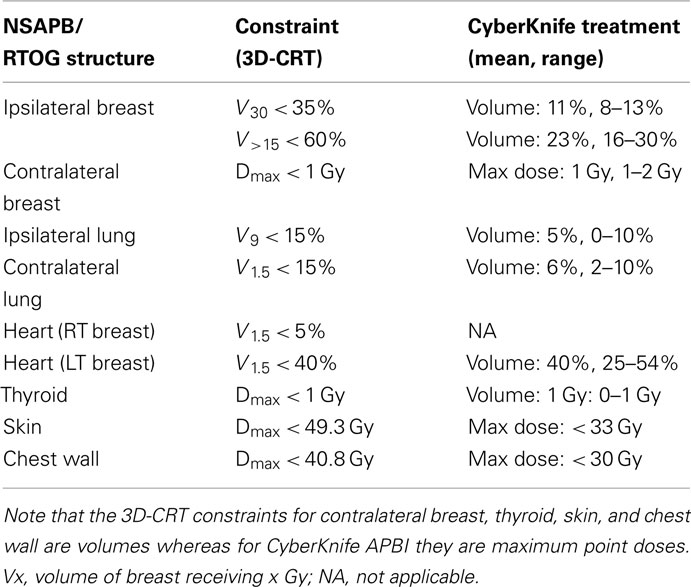
Table 2. Dose limitations for normal tissue based on the NSABP-39/RTOG 0413, 3D-CRT protocol (Vicini and White, 2007) for the patients in this series (n = 2) that received CyberKnife APBI to a total dose of 30 Gy delivered in five fractions.
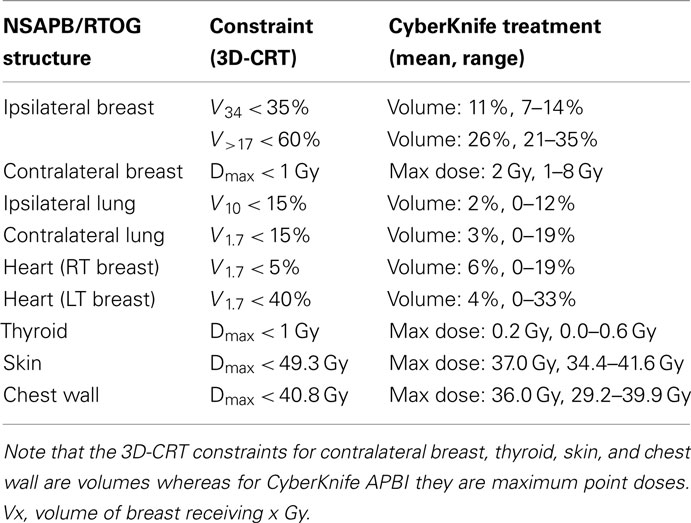
Table 3. Dose limitations for normal tissue based on the NSABP-39/RTOG 0413, 3D-CRT protocol (Vicini and White, 2007) for the patients in this series (n = 7) that received CyberKnife APBI to a total dose of 34 Gy delivered in 10 fractions.
For group 1, the mean number of beams was 155 (range, 112–119) with a PTV isodose prescription of 70% for all patients. For group 2, the mean number of beams was 157 (range, 143–182) and the mean PTV isodose prescription was 70% (range, 64–80%). Figure 2 illustrates a typical treatment plan. The mean PTV was 100 cm3 (range, 92–108 cm3) and 105 cm3 (range, 49–241 cm3) for groups 1 and 2, respectively. The mean percentage of a whole breast reference volume receiving 100 and 50% of the dose (V100 and V50) was 11% (range, 8–13%) and 23% (range, 16–30) for group 1 and 11% (range, 7–14%) and 26% (range, 21–35%) for group 2, respectively. Using the CyberKnife G4 with Iris (system version 8.5/3.5) the treatment time was approximately 60 min.
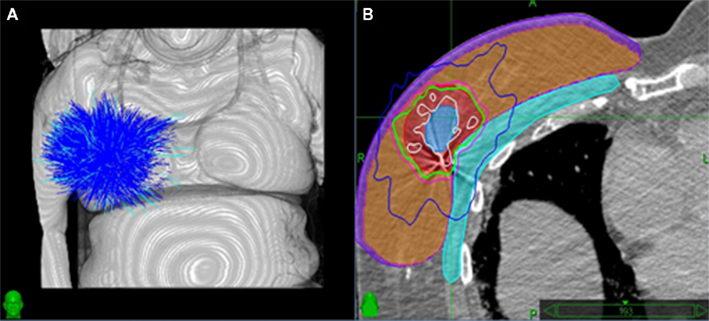
Figure 2. CyberKnife treatment planning images for a patient in the 34-Gy dose group. (A) Illustration of beam trajectories and (B) axial treatment planning image illustrating the omission of high doses to the chest wall and skin. Shown in (B) are three isodose lines at the 70, 50, and 30%. The green contour represents the planning target volume.
Toxicity, Cosmesis, and Outcome
At a median follow-up of 7 months (range, 4–26 months), minimal acute toxicities were observed. Specifically, half the patients experienced minimal to moderate fatigue following treatment lasting 2–3 weeks. However, these patients reported the same level of fatigue after surgery and at the start of their radiation. No skin, muscle, or lung toxicities were observed. All patients had increased breast edema following surgery; this became slightly worse after SBRT. For four patients, the breast edema was clinically evident 2–4 weeks after SBRT and resolved over 2 months.
The size, shape, and texture of the treated breast was compared to the breast’s original appearance after surgery and from pictures taken at the time of treatment planning. Acute cosmetic outcomes were excellent or good in all patients; for those patients with more than 12 months follow-up (n = 2) the late cosmesis outcomes remained excellent or good. No patient’s cosmetic outcome was graded as fair or poor. No breast cancer recurrence has been identified in this patient population to date. Figure 3 illustrates pre- and post-treatment images.
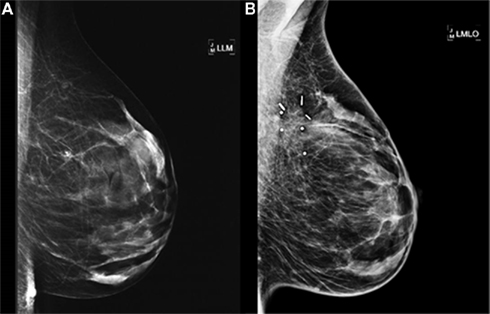
Figure 3. Pre- and post-CyberKnife APBI mammograms. (A) A left breast mammogram showing a biopsy clip in the tumor on a left lateral medial (LLM) view. (B) A left medial lateral oblique view of the same patient taken 14 months after CyberKnife APBI showing four gold fiducials (round opaque makers) and three titanium clips. The patient did not have chemotherapy or hormonal therapy post-treatment.
Discussion
Maturing data from the brachytherapy APBI experience indicate early breast cancer recurrences are rare both locally and outside the PTV (King et al., 2000; Vicini et al., 2001). Unfortunately, brachytherapy is invasive, difficult to perform, uncomfortable for the patient, and carries an infection risk. On the other hand, 3D-CRT and IMRT require larger treatment volumes for adequate coverage of the PTV. The CyberKnife offers a dose profile mimicking brachytherapy without the large PTV requirement of 3D-CRT and IMRT. We present our early experiences with CyberKnife APBI in the treatment of nine early breast cancers. At a median 7 months follow-up no patients have experienced fair/poor cosmesis and none have recurred. While this study is limited by the small number of patients, limited follow-up and retrospective nature of the study, the results to date are encouraging, but longer follow-up on a larger patient population is necessary to confirm the durability of these results.
The increased PTV volumes in the NSABP trial are now being challenged for compromising breast cosmesis (Vicini and White, 2007). Indeed, Jagsi et al. (2010) from the University of Michigan reports unacceptable outcomes in their patient population receiving IMRT when the V50 and V100 was greater than 46 and 23%, respectively. Both Jagsi and Hepel, from Tufts University, conclude that the NSAPB/RTOG trial’s normal tissue dose limitations result in a larger than acceptable number of patients developing subcutaneous fibrosis (Hepel et al., 2009; Jagsi et al., 2010). Both authors further conclude that stricter dose volume limits are encouraged for more agreeable outcomes. A more recent result from the University of Miami suggests that cosmesis is dependent on the target volume (Lewin et al., 2011). Our target volume (100 and 105 cm3 for dose groups 1 and 2, respectively) resides between those of the Jagsi study (185.8 cm3) and the University of Miami study (71.44 cm3).
In the NSABP/RTOG trial, the PTV evaluation (PTVeval) for patients receiving 3D-CRT is 25 mm or greater if penumbra is to be considered to assure coverage of the 15-mm microscopic tumor risk zone. Unlike the 3D-CRT coverage in the national trial, the CyberKnife does not require the extra 10 mm to compensate for the variability in treatment set-up and breathing motion. In addition, the low prescription isodose and high number of treatment beams used in CyberKnife APBI result in a smaller volume of normal tissue receiving a significant dose. The PTV in our patient series is similar to that seen in patients treated with multi-catheter or balloon catheter brachytherapy. Specifically, the mean ipsilateral breast volumes receiving 100 and 50% of the prescribed dose (V100, V50) in our study was less than half that allowable in the NSABP/RTOG study: 11 versus <35% and 26 versus <60% for both groups 1 and 2 (see Tables 2 and 3). Patel et al. (2007) compared the V50 and V100 of APBI with 3D-CRT verses interstitial brachytherapy. They reported both to be significantly larger for their patients’ receiving 3D-CRT than for the implant: 26 versus 12% and 52 versus 24%, respectively. Like the brachytherapy techniques, CyberKnife APBI delivers a relatively steep dose gradient outside and within the target volume with the dose maximum placed within the fluid cavity of the seroma. As such, we believe that the small treatment volumes and steep dose gradients using CyberKnife APBI will be responsible for more than acceptable cosmetic results and low toxicity in the long-term.
Conclusion
Our experience suggests that the CyberKnife is a suitable non-invasive radiation platform for delivering APBI with achievable normal tissue constraints. Although the clinical outcomes for this retrospective analysis are promising, the follow-up is too limited and the number of treated patients is too small to permit firm conclusions. Patient selection for APBI using the CyberKnife continues at our center and others worldwide including a recently opened dose escalating Phase I trial at the University of Texas Southwestern Medical Center (Timmerman, 2010). The next 3–5 years will provide additional evidence for local control and cosmetic outcomes in selected subgroups of patients with early breast cancer.
Conflict of Interest Statement
The Swedish Radiosurgery Center is a joint venture between Swedish Medical Center and the Tumor Institute Radiation Oncology Group. Dr. Vermeulen and Dr. Meier have received speaker’s honoraria from Accuray Incorporated.
Acknowledgments
We gratefully acknowledge the editorial assistance of Pam Commike, Ph.D., Accuray Incorporated. The views expressed here are entirely the authors’; Accuray did not provide assistance with data collection, compilation, or interpretation.
References
Benitez, P. R., Chen, P. Y., Vicini, F. A., Wallace, M., Kestin, L., Edmundson, G., Gustafson, G., and Martinez, A. (2004). Partial breast irradiation in breast conserving therapy by way of interstitial brachytherapy. Am. J. Surg. 188, 355–364.
Bentzen, S. M., Agrawal, R. K., Aird, E. G., Barrett, J. M., Barrett-Lee, P. J., Bliss, J. M., Brown, J., Dewar, J. A., Dobbs, H. J., Haviland, J. S., Hoskin, P. J., Hopwood, P., Lawton, P. A., Magee, B. J., Mills, J., Morgan, D. A., Owen, J. R., Simmons, S., Sumo, G., Sydenham, M. A., Venables, K., and Yarnold, J. R. (2008a). The UK standardisation of breast radiotherapy (START) Trial A of radiotherapy hypofractionation for treatment of early breast cancer: a randomised trial. Lancet Oncol. 9, 331–341.
Bentzen, S. M., Agrawal, R. K., Aird, E. G., Barrett, J. M., Barrett-Lee, P. J., Bliss, J. M., Brown, J., Dewar, J. A., Dobbs, H. J., Haviland, J. S., Hoskin, P. J., Hopwood, P., Lawton, P. A., Magee, B. J., Mills, J., Morgan, D. A., Owen, J. R., Simmons, S., Sumo, G., Sydenham, M. A., Venables, K., and Yarnold, J. R. (2008b). The UK standardisation of breast radiotherapy (START) Trial B of radiotherapy hypofractionation for treatment of early breast cancer: a randomised trial. Lancet 371, 1098–1107.
Clark, R. M., Mcculloch, P. B., Levine, M. N., Lipa, M., Wilkinson, R. H., Mahoney, L. J., Basrur, V. R., Nair, B. D., Mcdermot, R. S., Wong, C. S., and Corbett, P. J. (1992). Randomized clinical trial to assess the effectiveness of breast irradiation following lumpectomy and axillary dissection for node-negative breast cancer. J. Natl. Cancer Inst. 84, 683–689.
Cuttino, L. W., Keisch, M., Jenrette, J. M., Dragun, A. E., Prestidge, B. R., Quiet, C. A., Vicini, F. A., Rescigno, J., Wazer, D. E., Kaufman, S. A., Ramakrishnan, V. R., Patel, R., and Arthur, D. W. (2008). Multi-institutional experience using the MammoSite radiation therapy system in the treatment of early-stage breast cancer: 2-year results. Int. J. Radiat. Oncol. Biol. Phys. 71, 107–114.
Fan, J., Hayes, S., Freedman, G., Anderson, P., Li, J., Wang, L., Jin, L., Price, R., and Ma, C. (2010). Planning the breast boost: dosimetric comparison of CyberKnife, photo mini tangents, IMRT, and electron techniques. Int. J. Radiat. Oncol. Biol. Phys. 78, S3306.
Heinzerling, J. H., Ding, C., Ramirez, E., Chang, K., Anderson, J. F., Edwards, C. M., Boike, T., Rule, W., Solberg, T., and Timmerman, R. D. (2010). Comparative dose-volume analysis for CyberKnife and 3D conformal partial breast irradiation treatment of early stage breast cancer. Int. J. Radiat. Oncol. Biol. Phys. 78, S3390.
Hepel, J. T., Tokita, M., Macausland, S. G., Evans, S. B., Hiatt, J. R., Price, L. L., Dipetrillo, T., and Wazer, D. E. (2009). Toxicity of three-dimensional conformal radiotherapy for accelerated partial breast irradiation. Int. J. Radiat. Oncol. Biol. Phys. 75, 1290–1296.
Hoogeman, M., Prevost, J. B., Nuyttens, J., Poll, J., Levendag, P., and Heijmen, B. (2009). Clinical accuracy of the respiratory tumor tracking system of the cyberknife: assessment by analysis of log files. Int. J. Radiat. Oncol. Biol. Phys. 74, 297–303.
Jagsi, R., Ben-David, M. A., Moran, J. M., Marsh, R. B., Griffith, K. A., Hayman, J. A., and Pierce, L. J. (2010). Unacceptable cosmesis in a protocol investigating intensity-modulated radiotherapy with active breathing control for accelerated partial-breast irradiation. Int. J. Radiat. Oncol. Biol. Phys. 76, 71–78.
Jemal, A., Siegel, R., Ward, E., Hao, Y., Xu, J., and Thun, M. J. (2009). Cancer statistics, 2009. CA Cancer J. Clin. 59, 225–249.
Julian, T. B., Constantion, J. P., Vicini, F. A., White, J., Arthur, D. W., Kuske, R. R., Parda, D. S., Mamounas, E. P., Curran, W. J. Jr., and Wolmark, N. (2010). “Early toxicity results with 3D conformal external beam therapy (CEBT) from the NSABP B-39/RTOG 0413 accelerated partial-breast irradiation (APBI) trial,” in ASCO 2010 Breast Cancer Symposium: ASCO, San Francisco, CA.
Kilby, W., Dooley, J. R., Kuduvalli, G., Sayeh, S., and Maurer, C. R. Jr. (2010). The CyberKnife robotic radiosurgery system in 2010. Technol. Cancer Res. Treat. 9, 433–452.
King, T. A., Bolton, J. S., Kuske, R. R., Fuhrman, G. M., Scroggins, T. G., and Jiang, X. Z. (2000). Long-term results of wide-field brachytherapy as the sole method of radiation therapy after segmental mastectomy for T(is,1,2) breast cancer. Am. J. Surg. 180, 299–304.
Lewin, A. A., Derhagopian, R., Saigal, K., Panoff, J. E., Abitbol, A., Wieczorek, D. J., Mishra, V., Reis, I., Ferrell, A., Moreno, L., and Takita, C. (2011). Accelerated partial breast irradiation is safe and effective using intensity-modulated radiation therapy in selected early-stage breast cancer. Int. J. Radiat. Oncol. Biol. Phys. (in press).
Muacevic, A., Drexler, C., Wowra, B., Schweikard, A., Schlaefer, A., Hoffmann, R. T., Wilkowski, R., Winter, H., and Reiser, M. (2007). Technical description, phantom accuracy, and clinical feasibility for single-session lung radiosurgery using robotic image-guided real-time respiratory tumor tracking. Technol. Cancer Res. Treat. 6, 321–328.
Nelson, J. C., Beitsch, P. D., Vicini, F. A., Quiet, C. A., Garcia, D., Snider, H. C., Gittleman, M. A., Zannis, V. J., Whitworth, P. W., Fine, R. E., Keleher, A. J., and Kuerer, H. M. (2009). Four-year clinical update from the American Society of Breast Surgeons MammoSite brachytherapy trial. Am. J. Surg. 198, 83–91.
Patel, R. R., Becker, S. J., Das, R. K., and Mackie, T. R. (2007). A dosimetric comparison of accelerated partial breast irradiation techniques: multicatheter interstitial brachytherapy, three-dimensional conformal radiotherapy, and supine versus prone helical tomotherapy. Int. J. Radiat. Oncol. Biol. Phys. 68, 935–942.
Polgar, C., Sulyok, Z., Fodor, J., Orosz, Z., Major, T., Takacsi-Nagy, Z., Mangel, L. C., Somogyi, A., Kasler, M., and Nemeth, G. (2002). Sole brachytherapy of the tumor bed after conservative surgery for T1 breast cancer: five-year results of a phase I-II study and initial findings of a randomized phase III trial. J. Surg. Oncol. 80, 121–128; discussion 129.
Smith, B. D., Arthur, D. W., Buchholz, T. A., Haffty, B. G., Hahn, C. A., Hardenbergh, P. H., Julian, T. B., Marks, L. B., Todor, D. A., Vicini, F. A., Whelan, T. J., White, J., Wo, J. Y., and Harris, J. R. (2009). Accelerated partial breast irradiation consensus statement from the American Society for Radiation Oncology (ASTRO). Int. J. Radiat. Oncol. Biol. Phys. 74, 987–1001.
Timmerman, R. (2010). Cyberknife Partial Breast Irradiation (PBI) for Early Stage Breast Cancer. Available at: http://clinicaltrials. gov/ct2/show/NCT01162200?term=timmerman&rank=1
Vicini, F., Beitsch, P., Quiet, C., Gittleman, M., Zannis, V., Fine, R., Whitworth, P., Kuerer, H., Haffty, B., Keisch, M., and Lyden, M. (2011). Five-year analysis of treatment efficacy and cosmesis by the American Society of Breast Surgeons MammoSite Breast Brachytherapy Registry Trial in patients treated with accelerated partial breast irradiation. Int. J. Radiat. Oncol. Biol. Phys. 79, 808–817.
Vicini, F. A., Baglan, K. L., Kestin, L. L., Mitchell, C., Chen, P. Y., Frazier, R. C., Edmundson, G., Goldstein, N. S., Benitez, P., Huang, R. R., and Martinez, A. (2001). Accelerated treatment of breast cancer. J. Clin. Oncol. 19, 1993–2001.
Vicini, F. A., Kestin, L., Chen, P., Benitez, P., Goldstein, N. S., and Martinez, A. (2003). Limited-field radiation therapy in the management of early-stage breast cancer. J. Natl. Cancer Inst. 95, 1205–1210.
Vicini, F. A., Kestin, L. L., and Goldstein, N. S. (2004). Defining the clinical target volume for patients with early-stage breast cancer treated with lumpectomy and accelerated partial breast irradiation: a pathologic analysis. Int. J. Radiat. Oncol. Biol. Phys. 60, 722–730.
Vicini, F. A., and White, J. (2007). NSAPB B-39/RTOG 0413: a randomized phase III study of conventional whole breast irradiation versus partial breast irradiation for women with Stage 0, I, II Breast Cancer (Version March 13, 2007). Available at: http://www.rtog.org/ClinicalTrials/ProtocolTable/StudyDetails.aspx?study=0413 (accessed July 18, 2008).
Weed, D. W., Yan, D., Martinez, A. A., Vicini, F. A., Wilkinson, T. J., and Wong, J. (2004). The validity of surgical clips as a radiographic surrogate for the lumpectomy cavity in image-guided accelerated partial breast irradiation. Int. J. Radiat. Oncol. Biol. Phys. 60, 484–492.
Keywords: breast cancer, CyberKnife, accelerated partial breast irradiation, cosmesis
Citation: Vermeulen S, Cotrutz C, Morris A, Meier R, Buchanan C, Dawson P and Porter B (2011) Accelerated partial breast irradiation: using the CyberKnife as the radiation delivery platform in the treatment of early breast cancer. Front. Oncol. 1:43. doi: 10.3389/fonc.2011.00043
Received: 30 June 2011; Accepted: 24 October 2011;
Published online: 21 November 2011.
Edited by:
Brian Timothy Collins, Georgetown Hospital, USAReviewed by:
Jessica Hiatt, Rhode Island Hospital, USAJoel S. Greenberger, University of Pittsburgh Medical Center-Shadyside, USA
Copyright: © 2011 Vermeulen, Cotrutz, Morris, Meier, Buchanan, Dawson and Porter. This is an open-access article subject to a non-exclusive license between the authors and Frontiers Media SA, which permits use, distribution and reproduction in other forums, provided the original authors and source are credited and other Frontiers conditions are complied with.
*Correspondence: Sandra Vermeulen, Swedish Radiosurgery Center, 550 17th Avenue, Suite A-10, Seattle, WA 98122, USA. e-mail: sandra.vermeulen@swedish.org