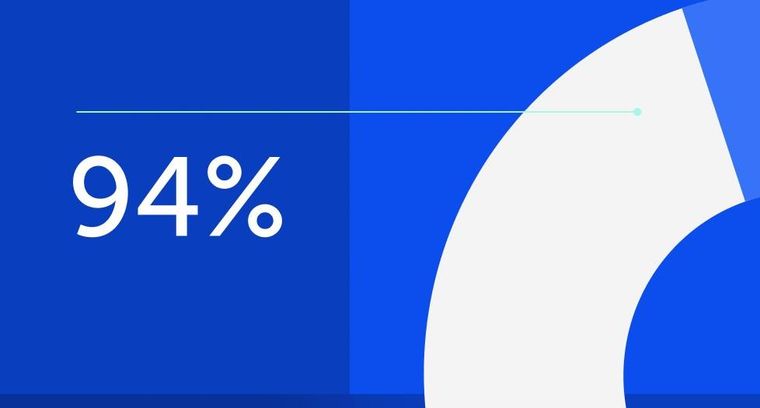
94% of researchers rate our articles as excellent or good
Learn more about the work of our research integrity team to safeguard the quality of each article we publish.
Find out more
EDITORIAL article
Front. Nucl. Eng., 07 April 2025
Sec. Radiation Measurements
Volume 4 - 2025 | https://doi.org/10.3389/fnuen.2025.1569870
This article is part of the Research TopicRadiation Detection and Measurement in Neutron Capture TherapyView all 5 articles
Editorial on the Research Topic
Radiation detection and measurement in neutron capture therapy
Boron Neutron Capture Therapy (BNCT) is now becoming popular and crucial as a new radiation therapy for cancers worldwide. BNCT can kill tumor cells by alpha and lithium particles emitted by a neutron-10B nuclear reaction. The charged particles have a range of around 10 µm, which is a similar size to a human body cell. Consequently, BNCT has a clear advantage of selectively killing tumor cells compared to other radiation therapies. In Japan BNCT systems are more and more accelerator based, not requiring building a nuclear reactor. Furthermore, Japan is the first country where BNCT is approved and covered as a cancer therapy by the public health care system. Soon, not only in Japan but also in other countries BNCT has a good chance to become one of the promising choices we can select as a reliable cancer therapy.
The other side of radiation therapy is that radiation has an adverse effect on the human body, making the detection and measurement of radiation during BNCT crucial for the introduction of the therapy on the global scale. This is however not as straightforward as for other radiation therapies, because BNCT uses neutrons, especially epi-thermal neutrons, which have otherwise not been used for clinical applications before. Additionally, high-energy gamma-rays are created at the same time, forming the mixed field of neutron, secondary neutrons and gamma-rays as well as secondary gamma- and X-rays, to which the patient is exposed during the treatment. This is completely different from other radiation therapies.
The present Research Topic includes various techniques to measure the radiation field, or part of it for BNCT. For neutrons this includes thermal energies up to several tens of MeV, and gamma-rays up to 10 MeV, which require different measurement techniques depending on the type of radiation and its energy. The physical quantities of interest are neutron source intensity, neutron flux intensity on the surface of the beam shaping assembly as well as on the patient, boron imaging, treatment effect (local boron dose), exposure doses due to neutron and gamma-ray and activation of materials in the facility. This Research Topic encompasses both theoretical and experimental studies related to BNCT. It includes the design, validation, and characterization of targeted neutron sources, both accelerator-based and reactor-based, utilizing various detectors. Additionally, the topic addresses the treatment and dosimetry aspects of BNCT within the field of nuclear medicine.
To date, four papers have been published in this Research Topic, focusing on:
(1) Boron imaging via autoradiography
(2) Neutron source intensity measurements
(3) BNCT dosimetry
(4) Neutron-induced radioactivity
Espain et al. group in Argentina has been studying boron imaging by autoradiaogaphy, which allows to predict the treatment effect, i.e., local boron dose. They have shown that a quantitative autoradiographic technique can be used to measure the boron concentration in the sample by counting the nuclear tracks on the detector. However, this method requires rapid measurements of the boron distribution after irradiation, which has so far not been possible. Thus, they developed a new methodology that allows a rapid and easy determination of boron concentration and distribution in a tissue section by simply measuring grey levels. These are later converted to optical density values, which are then correlated with boron concentration values. In order to validate the technique, consecutive sections of several tissue samples were analyzed by the conventional counting method and correlation shown. The image analysis and boron concentration estimation can be carried out in under 15 min, which is a crucial time limit for BNCT, as the boron concentration should be shorter that than the typical length of a treatment sessions. Portu’s method is expected to be well received in the boron imaging community of BNCT.
Tamaki et al. group at Osaka University, Japan, has developed the neutron intensity monitor with isomer production reaction for p-Li source. In their paper, the source neutron intensity of a p-Li neutron source was able to be determined by three foils depending on measuring purposes, in an accelerator designed for BNCT:
107Ag: Most convenient in a short measuring time.
115In: Highest measuring accuracy
189Os: Only available for backward angle
They have also pointed out that experimental tests show that the activation reaction cross section data of JENDL-A-96 should be improved for 107Ag and 115In, while IRDFF was acceptable, for real applications of BNCT.
Measurement of the absolute source term is fundamentally important, especially for accelerator-based neutron sources for BNCT, as an absolute and calibrated reference for all downstream detector is needed.
(3) Dosimetry is of fundamental importance for BNCT. The Bikchurina et al. group of the Budker institute of Nuclear Physics, in Russian federation, developed various diametrical methods for accelerator-based neutron source, VITA, for BNCT. Practically, it contains four diagnostic methods, that is, compact neutron detector, cell dosimeter, promptγ-ray spectroscopy and epithermal neutron flux monitor. The compact neutron detector measures the spatial distribution of boron dose and gamma-ray dose in air and in a water phantom with a resolution of 1 mm. The cell dosimeter measures the sum of fast neutron and nitrogen doses using cell cultures irradiated with gamma-rays and mixed radiation of neutron and gamma-ray. By protecting an HPGe detector properly, it became possible to utilize the prompt γ-ray spectroscopy for real-time measurement of the boron dose during the therapy. The epithermal neutron flux monitor can measure the epithermal neutron flux. It was concluded that it is desirable to use all the developed methods in therapeutic facilities for treatment planning and evaluation of its results. These results would be valuable for other BNCT facilities as well as for VITA.
(4) Finally, Capoulat and Kreiner group for BNCT in Argentina, explored radio-activities induced by neutrons in accelerator based neutron sources for BNCT in various BNCT facilities around the world. Naturally, it is essential to minimize the production of residual radioactivity to the lowest possible level, particularly in hospitals. They assessed quantitatively the residual radioactivity in various facilities, taking into account both primary and secondary activations. The primary activation mainly arises from the interaction of the proton or deuteron beam and the neutron-producing target. The secondary activation results from neutron-induced reactions with elements exposed to neutrons in a beam shaping assembly (BSA). To assess the activations, they evaluated the representative group with respect to the target-BSA configurations. Regarding the target activation, our findings indicate that 9Be targets impinged by protons of less than 10 MeV represent the cleanest, while 7Li targets bombarded with protons lead to the highest activation levels. As for the BSA activations, the neutron energy is a crucial factor. In the case of standard BSA materials, higher neutron energy results in increasing the number of radioactive products. Additionally, his groups findings suggest that radioactivity induced by impurities and minor components in alloyed materials cannot be neglected and must be taken into account in radioactivity calculations.
In summary, this research provides a comprehensive analysis of activation of the commonly used targets and BSA materials, aimed at contributing to the optimization of accelerator-based neutron sources, especially for determination of 9Be or 7Li which is the most important issue in the BNCT community.
In the present Research Topic, we focused on measuring techniques for BNCT as few Journals to feature this field. We hope this Research Topic could become a seed to grow and enhance the research of this field. By exposing these new results to wider audiences of scientists, we hope to stimulate more research that improves technology for future BNCT facilities.
IM: Conceptualization, Writing – original draft. AW: Investigation, Supervision, Writing – review and editing. FS: Data curation, Methodology, Software, Validation, Writing – review and editing.
The author(s) declare that no financial support was received for the research and/or publication of this article.
The authors declare that the research was conducted in the absence of any commercial or financial relationships that could be construed as a potential conflict of interest.
The author(s) declared that they were an editorial board member of Frontiers, at the time of submission. This had no impact on the peer review process and the final decision.
The author(s) declare that no Generative AI was used in the creation of this manuscript.
All claims expressed in this article are solely those of the authors and do not necessarily represent those of their affiliated organizations, or those of the publisher, the editors and the reviewers. Any product that may be evaluated in this article, or claim that may be made by its manufacturer, is not guaranteed or endorsed by the publisher.
Keywords: BNCT (boron neutron capture therapy), boron imaging, neutron source, dosimetry, induced radioactivity
Citation: Murata I, Winkler A and Sato F (2025) Editorial: Radiation detection and measurement in neutron capture therapy. Front. Nucl. Eng. 4:1569870. doi: 10.3389/fnuen.2025.1569870
Received: 02 February 2025; Accepted: 20 March 2025;
Published: 07 April 2025.
Edited and reviewed by:
Sergei Dudarev, United Kingdom Atomic Energy Authority, United KingdomCopyright © 2025 Murata, Winkler and Sato. This is an open-access article distributed under the terms of the Creative Commons Attribution License (CC BY). The use, distribution or reproduction in other forums is permitted, provided the original author(s) and the copyright owner(s) are credited and that the original publication in this journal is cited, in accordance with accepted academic practice. No use, distribution or reproduction is permitted which does not comply with these terms.
*Correspondence: Isao Murata, bXVyYXRhQHNlZS5lbmcub3Nha2EtdS5hYy5qcA==
Disclaimer: All claims expressed in this article are solely those of the authors and do not necessarily represent those of their affiliated organizations, or those of the publisher, the editors and the reviewers. Any product that may be evaluated in this article or claim that may be made by its manufacturer is not guaranteed or endorsed by the publisher.
Research integrity at Frontiers
Learn more about the work of our research integrity team to safeguard the quality of each article we publish.